ABSTRACT
Potassium (K) fertilizers have been widely used to promise agricultural production, yet rice (Oryza sativa L.) showed healthy growth for long periods under K-deficient conditions. These findings imply that rice could take up minerals-bound K as non-exchangeable K. When the weathering of primary silicate minerals occurs in the rhizosphere of rice, the rice takes up not only dissolved K but also dissolved silicic acid (SiO2). We grew three rice cultivars with different Si-uptake abilities (Hokuriku 193, Oochikara, and Lsi-1) in granitic Fluvisol under K- and Si-deficient conditions to quantitatively reveal the significance of the Si-uptake by rice for the mineral weathering in the rhizosphere. As a result, the Si-uptake quantity by rice was positive linearly correlated with the amount of Al accumulation in soil, suggesting a substance containing a certain ratio of Si and Al was decomposed by rice. The K:Al:Si molar ratio of the decomposed substance by rice was 0.2:1:2.6, and the ratio is suggestive of silicate minerals such as K-feldspar. Because silicate minerals such as K-feldspar (e.g., KAlSi3O8) contain a large amount of aluminum (Al), Al release alongside K ions and silicic acid in their weathering. Dissolved Al is highly active and can be stabilized by combining with organic matter (OM) or silicic acid in the rhizosphere. The Si-uptake levels and mineral decomposing abilities of rice affected the variety of the accumulated substances (i.e., Al hydroxy oxide with OM, clay minerals) in the soil after cultivation. In particular, the Al hydroxy oxide with OM accumulates as persistent soil organic carbon. Thus, it appears that the Si-uptake abilities of plants have a great influence on the weathering of minerals and their products.
1. Introduction
Potassium (K) is one of the main essential nutrients in plants and is exclusively sourced from geological materials. It is mainly mined as sylvanite (KCl + NaCl) and is used to produce fertilizer products (Manning Citation2010), which are important in sustainable agriculture.
Mineral soils contain 0.04–3% K, but 90–98% of this is considered to be incorporated into the crystal lattice structure of minerals and so is not directly available for plant uptake (Zörb, Senbayram, and Peiter Citation2014). The forms of K in the soil are classified into four groups: water-soluble, exchangeable, fixed between mineral layers such as mica, and structural components of silicate minerals such as feldspar (Sparks and Huang Citation1985). Water-soluble K is directly available for plants, and exchangeable K is electrostatically bound to the surfaces of clay minerals and/or OM and can also be readily used by plants. However, fixed K and structural K are considered to be supplied slowly or be unavailable for plants due to the very slow dissolution rate of silicate minerals.
However, some reports that many plant species can take up K from silicate minerals (Manning Citation2010; Weerasuriya, Pushpakumara, and Cooray Citation1993). Especially, rice can not only take up fixed K in minerals but also facilitate the release of SiO2 from minerals (Sugiyama and Ae Citation2000). Therefore, we focused on rice-induced mineral weathering that releases K and Si from silicate minerals. Field experiments have been performed to evaluate soil fertility for rice (Oryza sativa L.) cultivation in terms of the major nutrient elements nitrogen (N), phosphorus (P), and K over 70–80 years at several agricultural experiments stations in Japan. According to those results, rice plants treated with NP fertilizers had almost identical yields to those treated with NPK fertilizers (Shiota, Sano, and Okimura Citation1984; Momii and Izawa Citation2007). Thus, it appears that rice can take up K from a source other than soil available K and irrigation water, and the fixed K and structural K in silicate minerals is one of the potential sources.
If minerals are decomposed by rice to release K+, then silicon (Si) and aluminum (Al) will also be released into the soil. It will result in Al accumulating in the rhizosphere because Al is less taken up by rice plants (approximately 0.3 g m−2 year−1, Kusa et al.Citation2021). However, residual Al in cultivated soils have not been measured quantitatively and there is also little quantitative evidence of the supply of unexchangeable K from soils for plant cultivation. Recently, Kusa et al.(Citation2021) reported that Al accumulation occurred in the soil during paddy rice cultivation for 11 years and this accumulation rate was much higher than those of the general (mainly proton-promoted) weathering of minerals. The same authors also suggested that carbon (C) accumulation with Al (hydroxy) oxide was found in the paddy soil. Furthermore, Hobara et al.(Citation2016) reported that in a cultivation of the soil using fresh volcanic ash, more active Al accumulated in the rhizosphere of rice plants with high Si uptake than in those with low Si uptake. These previous reports imply that variation in Si uptake by rice can affect the mineral weathering after cultivation. However, these results were based on data from volcanic soil, which is easily weathered due to the presence of fine-grained volcanic glass. If a similar phenomenon has happened in mineral soils, such as those of granitic origin, then rice cultivation may be one of the factors that are responsible for weathering of silicate minerals.
In this study, we grew three rice cultivars with different Si-uptake abilities (Hokuriku 193, Oochikara, and Lsi-1) in granitic Fluvisol under the restricted supply of K and Si (no fertilizer application) to clarify a relationship between the Si-uptake abilities of rice and their influence to form the accumulating substances in the soil.
2. Materials and methods
2.1. Field experiment
Three rice cultivars were grown in paddy fields with granitic gray lowland soil (Fluvisols) in Otsu, Shiga, Japan (35°0′N, 136°0′E). The following cultivars were selected based on their Si uptake abilities: the forage rice cultivar Hokuriku 193, which can take up large amounts of Si (67–100 g m−2); the edible rice cultivar Oochikara, which can take up intermediate amounts of Si (40–60 g m−2); and Lsi-1 which is the parent mutant of Oochikara, lacks one of the Si transporters in Oochikara (Ma et al. Citation2002) and so can only take up low amounts of Si (20 g m−2). All three cultivars were grown without fertilizer from June 12 to September 29, 2017, and from May 20 to October 2, 2018 (planting to harvesting). After harvesting, soil samples were collected from the field. The stubble of each rice plant and associated soil was dried at 40°C for 1 week and then struck with a wooden hammer. Any soil remaining on the roots was then collected as ‘rhizosphere soil’ using a brush and passed through a 2-mm sieve. Soil samples were also obtained from corners of an uncultivated field adjacent to each cultivar and used as ‘control soil'.
2.2. Pot experiment
The same Fluvisol that was present in the experimental fields was used in the pot experiment after being dried and passed through a 2-mm sieve. Seeds of the rice cultivars Hokuriku 193, Oochikara, and Lsi-1 were washed with a detergent containing 0.5% sodium hypochlorite and sandwiched between 2cm × 2cm × 0.5cm urethane foam. They were then soaked in distilled water and germinated in an incubator at 30°C. The young seedlings attached to the urethane foam were transplanted into 300-mL polyethylene pots filled with 500 g of soil on May 20, 2019, at a density of 5 seedlings per pot with three replicate pots per cultivar, and cultivated for 101 days without fertilizer. During cultivation, distilled water was constantly added. The rice was harvested on August 29, 2019, and soil samples were collected from the entire pot. Each soil sample was then dried and any roots were removed with tweezers. Unplanted soil that had been subjected to the same water management as the planted soil in the pots was collected as ‘control soil'.
2.3. Chemical analysis of the soil and irrigation water
Exchangeable K in the soil was measured using the 0.01 M ammonium acetate (NH₄CH₃CO₂) extraction method. Dried soil (1 g) was added to 20mL of 0.01 M NH₄CH₃CO₂ solution and shaken for 1 h, following which the K content was measured with an ion meter (LAQUA twin K+; Horiba, Japan). Dissolved Si was obtained from the soil by phosphate buffer extraction (pH 6.2, 0.04 M phosphate buffer shaken for 24 h), following which the Si content of the extract was measured by inductively coupled plasma atomic emission spectroscopy (ICP-AES: iCap7400 Duo, Thermo, Waltham, USA) after filtration (ADVANTEC No.6). The exchangeable K and dissolved Si of the soil before cultivation in each experiment were used for elemental balance calculations.
The chemical forms of Al in the soil were analyzed using a five-step sequential extraction method based on the adsorption strength between Al and OM. This method used 1 M potassium chloride (KCl), 0.5 M copper chloride (CuCl2), 0.1 M sodium pyrophosphate (Na4O7P2), 0.2 M ammonium oxalate [(NH4)2C2O4], and 0.5 M sodium hydroxide (NaOH) solutions, respectively (Dai et al. Citation2011). The contents of Al in the respective extracts were then determined by ICP-AES. These fractions included Alk (from KCl), which is composed of Al3+ ions; AlCu (from CuCl2) and Alpp (from Na4O7P2), which are weakly and strongly bonded with OM, respectively; Alox [from (NH4)2C2O4], which is equivalent to the allophane form of Al; and AlNa (from NaOH), which is the crystalline form of Al (Dai et al. Citation2011). The dissolved organic carbon contents of the CuCl2 and Na4O7P2 extracts (Ccu and Cpp, respectively) were also measured with a dissolved organic carbon analyzer (TOC-L; Shimadzu, Kyoto, Japan).
Irrigation water samples were collected once a week during the cultivation in 2017 (from Jun 11 to September 29) and the K and Si contents were measured by ICP-AES. To calculate the irrigation water supply for Si and K, the average concentrations (Si: 2.14 mg L−1, K: 0.71 mg L−1) were used. We assumed that irrigation water was supplied at a rate of 15,000 Mg ha−1 year−1 (Tanji Citation1998) for an elemental balance calculation.
To calculate the balances between rice uptake and the soil supply for Si and K, the available soil depth in the fields and the bulk density of the soil were assumed to be 0.1 m and 1 g cm−3, respectively.
2.4. Chemical analysis of plant samples
After harvest, the rice plants were dried and ground. The resulting powders were then digested with a mixture of nitrate and hydrogen peroxide (Oda Citation2004), and the K contents of the digested solutions were measured by ICP-AES. The silica (SiO2) contents of the samples were obtained by the combustion method, which involved heating the samples for 2 h at 200°C and 19 h at 750°C and then measuring the dry ash weights. The K and Si uptakes by the rice plants were determined from the contents of each element and the dry matter weights. The resulting SiO2 contents were converted to Si to calculate the Si uptake by rice.
2.5. Statistical analysis
All statistical analyses were performed using Kaleida Graph version 4.5 (HURINKS Inc., Tokyo, Japan).
3. Results and discussion
3.1. Balances of K and Si between uptake by rice and the supply from soil and irrigation water
In 2017, Hokuriku 193 and Oochikara had dry matter weights of 2560 g m−2 and 1591 g m−2, respectively (), which were similar to the standard levels of rice growth in Japan (Furuhata Citation2013; Uehara et al. Citation2003), whereas Lsi-1 (1086 gm−2) had approximately 60% of the dry matter production of Oochikara. Both Hokuriku 193 and Oochikara grew as well under the restricted supply of K and Si (no fertilizer application) as they did under standard cultivation conditions in the field experiment. In 2018, Hokuriku 193 (1548 g m−2) and Oochikara (917 g m−2) had approximately 60% of their dry matter production in 2017, respectively. The amount of K-uptake by rice was greater than that of supply from the soil and irrigation water for Hokuriku 193 and Oochikara, however, the balance was not negative for Lsi-1. Whereas, the amount of Si-uptake by rice was greater than that of supply from the soil and irrigation water for all three cultivars in 2017 and 2018 ().
Table 1. Yield and potassium (K) and silicon (Si) uptakes and balances in rice (Oryza sativa) plants during the field experiment. (means ± standard deviations)
In the pot experiment, the dry matter weight of Hokuriku 193 was 17.3 g pot−1. The dry matter weight of Lsi-1 (13.6 g pot−1) was almost identical to that of Oochikara (14.0 g pot−1). For K and Si, the uptakes by rice (K: 70-100 mg pot−1, Si: 370-650 mg pot−1) exceeded their supplies from soil (K: 30 mg pot−1, Si: 80 mg pot−1), and their balances were negative for all cultivars ().
Table 2. Yield and potassium (K) and silicon (Si) uptakes and balances in rice (Oryza sativa) plants during the pot experiment. (means ± standard deviations)
Based on the above results, rice plants also grew normally under the restricted supply of K and Si (no fertilizer application) in the field and the pot experiment. The rice would take up K and Si from an unknown source other than soil available K or Si. The only source of K and Si was minerals containing K in the pot experiment.
3.2. Accumulation of Al in the soil
The amounts of Alpp were higher in the rhizosphere soils than that in the respective control soils after the cultivation of Hokuriku 193 in 2017 and 2018 and Oochikara in 2017 (). By contrast, the Alpp of the rhizosphere in soils grown by Oochikara in 2018 and Lsi-1 decrease but both of Alox and AlNa of these soils increased, which took up less Si (Oochikara in 2018: 39.5 g m−2; Lsi-1: 19.9 g m−2) than Hokuriku 193 in 2017 and 2018 and Oochikara in 2017 (60.0-100.2 g m−2). Examination of the relationship between the uptake quantity of Si by rice and the increase in the amount of Alpp (ΔAlpp) in the rhizosphere soil compared with the control soil in the field showed that there was a positive correlation between them (r2 = 0.75, P = 0.059; ). Therefore, it is suggested that rice cultivation accelerated the decomposition (weathering) of minerals in the rhizosphere soil, resulting in the release of Al (Kusa et al. Citation2021).
Table 3. Aluminum (Al) contents of five extracts were obtained by the five-step sequential extraction of soils in the field experiment. The following extracts were obtained: Alk from potassium chloride (KCl) solution, Alcu from copper chloride (CuCl2) solution, Alpp from sodium pyrophosphate (Na4O7P2) solution, Alox from ammonium oxalate solution [(NH4)2C2O4], and AlNa from sodium hydroxide (NaOH) solution. (means ± standard deviations)
Figure 1. Relationship between silicon (Si) uptake by rice (Oryza sativa) and increases in aluminum extracted by sodium pyrophosphate (ΔAlpp) of the rhizosphere soil in the field experiment (r2 = 0.75, P = 0.059)
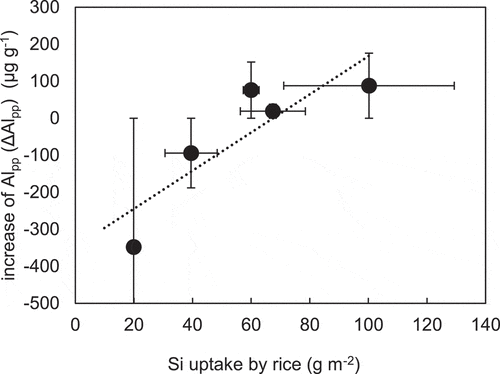
Table 4. Aluminum (Al) contents of five extracts were obtained by the five-step sequential extraction of soils in the pot experiment. (means ± standard deviations)
When there are high levels of Si-uptake in the rhizosphere of rice (> 60 g m−2: the value of Si-uptake where an increase of Alpp (ΔAlpp) is positive in ), the silicic acid that is released from the mineral can be immediately taken up by rice and the remained Al3+ ions are converted into Al hydroxide [Al(OH)n(3-n)+], which forms an Al-organo complex with surrounding OM (Alpp) (Dai et al. Citation2011). By contrast, when Si-uptake by rice levels is low, the silicic acid concentration in the rhizosphere does not decrease and Al can be recombined with Si to form silica-alumina gel, amorphous aluminosilicate, or clay (Sudo Citation1974), causing Alox and AlNa to increase and Alpp to decrease. Thus, when Al is released by mineral decomposition in the rhizosphere, the forming substance bound to Al can change depending on the silicic acid concentration in the rhizosphere, which is affected by the amount of Si-uptake by the rice and the silicic acid concentration of the irrigation water. The maximum level of accumulated Alpp in the field experiment was 88 mg kg−1 (increase in Alpp between the rhizosphere and the control soils of Hokuriku193 in 2017, ), which is also similar to that resulting from rice cultivation in volcanic soil (57 mg kg−1; Kusa et al. Citation2021). Those Al accumulation rates are much higher than those obtained by proton-promoted natural weathering (Sverdrup et al. Citation2019).
In the pot experiment, the amounts of all forms (Alk, Alcu, Alpp, Alox, and AlNa) of Al increased in the soil after cultivation for all three cultivars (). Although the soil used in the pot experiment contained same amount of dissolved Si as the field soil (16.0 g m−2), it was irrigated with distilled water and therefore had approximately 20% less Si, converted from the irrigation water supply of Si (3.2 g m−2). In addition, five rice plants were grown in each pot, resulting in more Si-uptake by rice per unit weight of soil, for example, the Si-uptake by Hokuriku 193 was 1.3 times higher in the pot soil (1.3 g kg−1) than in the field in 2018 (1.0 g kg−1). Consequently, Si concentrations in the soil were markedly lower in the pot experiments than that in the field experiment. Even cultivation of the low Si-uptake cultivar Lsi-1 resulted in an increased amount of Alpp. The Si balances in the pot experiment were from −58 to −114 g m−2 year−1 when converted to field experimental soil. Thus, mineral decomposition by rice plants was observed in the soils during only 101 days of cultivation.
The amount of released total Al in the pot experiment was highest in the soil in which Oochikara had been cultivated, even though the Si-uptake of Hokuriku 193 (0.65 g pot−1) was higher than that of Oochikara (0.45 g pot−1) (). It is possible that other elements are related to these discrepancies, though the cause of these discrepancies remains to be determined.
3.3. Accumulation of organic C in the soil
In the field experiment, the amounts of Ccu and Cpp in the rhizosphere soils increased after the cultivation of Hokuriku 193 in 2017 (), which reflects the increases for Alcu and Alpp (). However, there was no clear increase in Al with carbon in each extract for the other sample soils in field experiments. This was probably due to spatial variation of carbon materials near the active Al in the paddy field. Namely, the active Al is associated with the surrounding carbon if it exists, but if there is no surrounding carbon, it becomes a hydroxyl aluminum polymer and is evaluated as AlNa (Wada Citation1977). In the pot experiment, the total amount of Cpp in the soils also increased with Alpp after cultivation (). This simultaneous increase in Alpp and Cpp (: r2 = 0.99, P = 0.05) indicates that they formed a complex. The released hydroxyl Al from silicate minerals could bond with surrounding OM to form Al-organo complexes in the cultivated soils. Therefore, Al-organic complexes could accumulate in paddy soils for rice cultivation during a short period.
Table 5. Carbon (C) contents of two extracts were obtained by the five-step sequential extraction of soils in the field experiment. The following extracts were obtained: CCu from copper chloride (CuCl2) solution and Cpp from sodium pyrophosphate (Na4O7P2) solution. (means ± standard deviations
Table 6. Carbon (C) contents of two extracts were obtained by the five-step sequential extraction of soils in the pot experiment. (means ± standard deviations)
3.4. Identify the minerals decomposed by rice from stoichiometric analysis
The balances for Al, Si, and K between uptake by rice and the supply from soil to Hokuriku 193 in the pot experiment are shown in . Total Al (i.e., the sum of all forms) increased by 7.77 mM pot−1 during cultivation (‘c’ in ), while the amounts of Si and K uptake by rice from an unknown source, which may have been derived from decomposed minerals, were 20.35 mM pot−1 (‘b’ in ) and 1.79 mM pot−1 (‘a’ in ), respectively. Therefore, the molar ratio of K:Al:Si in the material decomposed by rice (Hokuriku 193) was 1.79:7.77:20.35 or 0.2:1:2.6 (). This proportion of K is similar to the calculated value (0.3–0.5) from the mineral mode composition of Tanakami granite (Hashimoto et al. Citation2000), which was the parent material of the experimental soil. The Al:Si ratio is close to the ratio in silicate minerals such as K-feldspar and biotite (Al:Si = 1:3), which are components of Tanakami granite, along with quartz and plagioclase. Furthermore, the estimated amount of Si that would be released by the decomposition of silicate minerals by rice (23.16 mM pot−1, c× 3) shows good agreement with the negative balance of Si (20.35 mM pot−1) which was observed in the experiment. Therefore, these results quantitatively demonstrate that rice plants take up K and Si through the decomposition of silicate minerals.
Figure 3. Estimation of decomposed mineral composition by the integrated data obtained from the pot experiment where rice (Oryza sativa ‘Hokuriku 193ʹ) was cultivated together with K and Si balance estimation and sequential extraction of soil Al. Elemental concentrations are given in mol (weight divided by atomic weight)
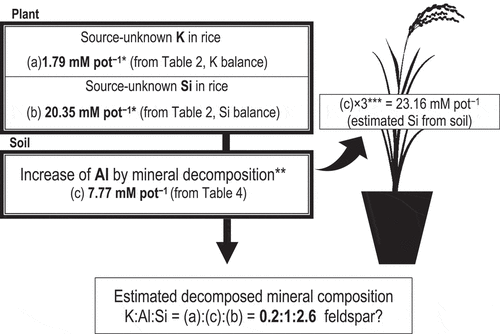
3.5. The resulting substances in the rhizosphere according to Si uptake level by plant
The results of the above pot experiment suggest that cultivated rice may decompose minerals and take up K and Si, resulting in the accumulation of Al in the soils. Differences in the amounts of elemental uptakes and mineral decomposition by rice are expected to cause some changes in the properties of the cultivated soil. For example, if K-feldspar (KAlSi3O8) is decomposed by the plants under the condition where water is present, a variety of materials will be deposited in the rhizosphere. The following typical cases could be expected to occur depending on the level of K and Si uptake by plants, in which OM is an organic matter.
Case (1): The plant takes up all K and Si in the rhizosphere, causing Alpp to increase:
4KAlSi3O8 + nH2O → 4K+ (↑plant) + 12H4SiO2(↑plant)+ 4Al3+→ 4hydroxyl Al (+ OM)
Case (2): The plant takes up K and most of the Si in the rhizosphere, causing Alox to increase:
4KAlSi3O8 + nH2O → 4K+ (↑plant) + (8–9.4)H4SiO2(↑plant) + (2.6–4)H4SiO2 + 4Al3+→2Al2O3·(SiO2)1.3–2·(5–6)H2O(allophone)
Case (3): The plant takes up K and about two-third of the Si in the rhizosphere, causing AlNa to increase
4KAlSi3O8 + nH2O → 4K+(↑plant) + 8H4SiO2(↑plant) + 4H4SiO2 + 4Al3+→ Al4Si4O10(OH)8 (kaolinite or halloysite)
Case (4): The plant takes up the only K in the rhizosphere, causing AlNa and Si to increase:
4KAlSi3O8 + nH2O → 4K+(↑plant) + 12H4SiO2 + 4Al3+ → Al4Si4O10(OH)8 (kaolinite or halloysite) + 8SiO2 (amorphous silica)
Case (5): No plants are grown in the soil (hydrolysis under geologic timescale):
3KAlSi3O8 + nH2O → 3KAl2(AlSi3)O10 (OH)2 (illite)
The formed materials in cases (1)– (5) have been interpreted as variations of elemental dissolution in the natural weathering cycle. However, the results of our experiments revealed that cases (1)–(3) progressed within a single rice crop season in the rhizosphere. Since the root activity of a plant varies locally, it is natural for multiple cases to be occurring at the same time in the rhizosphere. In the pot experiments, the cultivation of Hokuriku 193 and Oochikara led to increases in Alpp suggesting that the reaction in case (1) had mainly occurred, whereas the cultivation of Lsi-1 resulted in only small increases in Alpp and AlNa and a higher Si concentration of the NaOH extracts than those of others, suggesting that the reaction is mainly case (3) had occurred. Further, Sugiyama and Ae (Citation2000) reported an increase in soil available silicic acid after soybean cultivation under K-free fertilization conditions. Thus, reaction (4) may occur in some crops such as soybean that do not take up Si. These reactions demonstrate that the amounts of silicic acid of the rhizosphere soil and the uptake by the rice plants could affect the form of accumulated materials in the post-cultivated soil. In addition, the combination of Al(OH)n (3-n)+ with OM is expected to increase some fractions of organic C (Ccu and Cpp) of the soil. The increase in Cpp was accompanied by an increase in Alpp in the pot experiments ().
In the field experiment, when Si uptake by rice was >60 g m−2 (Hokuriku 193 in 2017), the reaction shown in case (1) would have mainly progressed, with Alpp accumulating in the rhizosphere soil (). Furthermore, increases in Cpp and Alpp of the soil were shown in the cultivation of Hokuriku 193 in 2017 (). By contrast, when Si uptake was less than 40 gm−2 (Oochikara in 2017 and Lsi-1), the reactions shown in cases (2) and (3) would have mainly proceeded in the rhizosphere, with Alox and AlNa increasing, which could contain allophones and clay minerals ().
By contrast, proton-promoted weathering cannot result in the rapid dissolution of Al from minerals except in highly acidic conditions. Because of the absence of plant uptakes of K and Si, it is also difficult for several reactions to occur at the same time with geological weathering. Therefore, the interaction between minerals and Si-uptake plants is probably important for clay mineral formation and carbon storage, which may lead to ‘soil formation'. Kusa et al. (Citation2021) reported that the mechanism of weathering by rice is chelation by the root cell wall. However, the mechanism of mineral decomposition by plants remains still unclear, so further research is required to investigate the nature of the interactions between the roots of plants and silicate minerals.
4. Conclusions
Our study was designed to quantitatively determine the changes in amounts of K, Si, Al, and C in the soil as a result of rice cultivation. We observed that normal rice growth could occur in granitic Fluvisol under the restricted supply of K and Si (no fertilizer application) (, 2), and our experiments also revealed that Al and C could be accumulated in the soil over a short period (101 days or a single cropping season). There was a positive correlation between Si-uptake quantity by rice and the Al supply from soil minerals (). Quantifications of the amount of accumulated Al in cultivated soil and the balances of K and Si between uptake by rice and the soil supply in the pot experiment suggested that silicate minerals such as K-feldspar were decomposed by rice (). Furthermore, the differences in Si uptake level by rice can cause several materials (hydroxyl Al oxide or clay minerals) to be accumulated in the post cultivated soils. Together, these results suggest that rice cultivation promotes the decomposition of silicate minerals and rice plants take up K and Si, resulting in the accumulation of Al oxide and/or clay minerals, along with the organic C that adsorbed Al. These findings enhance our understanding of the direct role of plant cultivation in weathering. Further research is required to better understand the mechanism of mineral decomposition by plants.
Acknowledgments
We are grateful to Prof. Ma (Okayama University) for sharing the Oochikara and Lsi-1 seeds. We thank Dr. D.Yoshimura, Messrs T. Seo, and T. Kira (Ryukoku University) for rice cultivation in the field. We thank to Miss S. Matsuzono, Messrs R. Monma, and Y. Murakami for sample preparation and their measurement.
Disclosure statement
No potential conflict of interest was reported by the authors.
Additional information
Funding
References
- Dai, Q.X., N.Ae, T.Suzuki, M.Rajkumar, S.Fukunaga, and N.Fujitake. 2011. “Assessment of Potentially Reactive Pools of Aluminum in Andisols Using a Five-step Sequential Extraction Procedure.” Soil Science and Plant Nutrition 57(4): 500–507. doi:10.1080/00380768.2011.598445.
- Furuhata,M. 2013. “Growth and Yield Characteristics of Rice Cultivar ‘Hokuriku 193ʹ Cultivated by Space Planting Method in the Hokuriku Distinct of Japan.” Japanese Journal of Crop Science 82((1):): 69–76. doi:10.1626/jcs.82.69.
- Hashimoto,K., Y.Kuda, T.Kutsukake, S.Nakano, H.Nishihashi, S.Nishimura, K.Sawada, K.Sugii, and G.Yoshida. 2000. “Granitic Masses around Lake Biwa, Southwest Japan: Part5. The Tanakami Granite Pluton.” Earth Science (Chikyu Kagaku) 54: 380–392.
- Hobara,S., S. Fukunaga-Yoshida, T.Suzuki, S.Matsumoto, T.Matoh, and N.Ae. 2016. “Plant Silicon Uptake Increases Active Aluminum Minerals in Root-zone Soil: Implications for Plant Influence on Soil Carbon.” Geoderma 279: 45–52. doi:10.1016/j.geoderma.2016.05.024.
- Kusa,K., M.Moriizumi, S.Hobara, M.Kaneko, S.Matsumoto, J.Kasuga, and N.Ae. 2021. “Mineral Weathering and Silicon Uptake by Rice Plant Promote Carbon Storage in Paddy.” Soil Science and Plant Nutrition 67(2): 162–170. doi:10.1080/00380768.2021.1878471.
- Ma, J.F., K.Tamai, M.Ichii, and G.F. Wu. 2002. “ARice Mutant Defective in Si Uptake.” Plant Physiology 130(4): 2111–2117. doi:10.1104/pp.010348.
- Manning, D.A. 2010. “Mineral Sources of Potassium for Plant Nutrition. A Review.” Agronomy for Sustainable Development 30(2): 281–294. doi:10.1051/agro/2009023.
- Momii,T., and T.Izawa. 2007. “The Yielding of Rice and the Effects of Each Element in Consecutive 77-year Lacking Four Elements and Fertilizing with Compost.” Japanese Journal of Crop Science 76: 288–294. doi:10.1626/jcs.76.288.
- Oda,H. 2004. “Uptake and Transport of Cd Supplied at Different Growth Stages in Hydroponically Cultured Soybean Plants.” Biomedical Research on Trace Elements 15(3): 289–291.
- Shiota,Y., K.Sano, and I.Okimura. 1984. “Short and Long-term Effect of the Successive Application of Rice Straw Compost Evaluated by Nitrogen Uptake by Rice Plants.” Research Bulletin of the Aichi-ken Agricultural Research Center 16: 43–51.
- Sparks, D.L., and P.M. Huang. 1985. “Physical chemistry of soil potassium.” Potassium in agriculture, ASA, CSSA, and SSSA Books, 201-276. doi:10.2134/1985.potassium.
- Sudo,T. 1974. Nendo koubutsugaku [Clay Mineralogy], 218–228. Tokyo: Iwanami Syoten.
- Sugiyama,M., and N.Ae. 2000. “Uptake Response of Crops to Potassium from Andosol and Potassium −bearing Minerals Applied to Andosol.” Japan Journal of Soil Science Plant Nutrition 71: 786–793.
- Sverdrup, H.U., E.Oelkers, M.Erlandsson Lampa, S.Belyazid, D.Kurz, and C.Akselsson. 2019. “Reviews and Syntheses: Weathering of Silicate Minerals in Soils and Watersheds: Parameterization of the Weathering Kinetics Module in the PROFILE and ForSAFE Models.” Biogeosciences Discussions 1–58. https://doi.org/10.5194/bg-2019-38
- Tanji,H. 1998. “Demand Estimation of Irrigation Water of Japan in the 21th Century.” Journal of Japan Society of Hydrology Water Resources 11: 757–767. doi:10.3178/jjshwr.11.757.
- Uehara,Y., A.Kobayashi, Y.Koga, H.Ohta, H.Shimizu, K.Miura, K.Fukui etal. 2003. “ ANew Rice Variety ‘Kusayutaka’.” Bulletin of National Agricultural Research Center 2:83–105.
- Wada,K. 1977. “Active Aluminum in Kuroboku Soils and Non- and Para-crystalline Clay Minerals.” Nendokagaku 17: 143–151.
- Weerasuriya, T.J., S.Pushpakumara, and P.I. Cooray. 1993. “Acidulated Pegmatitic Mica: APromising New Multi-nutrient Mineral Fertilizer.” Fertilizer Research 34(1): 67–77. doi:10.1007/BF00749962.
- Zörb,C., M.Senbayram, and E.Peiter. 2014. “Potassium in Agriculture–status and Perspectives.” Journal of Plant Physiology 171(9): 656–669. doi:10.1016/j.jplph.2013.08.008.