ABSTRACT
The Green Revolution (GR) made a major contribution to increasing the rice yield in the Philippines from 1.3 t ha−1 in 1966 to 4.0 t ha−1 in 2018, but the changes in paddy soil fertility that occurred during this 50-year period remain unknown. We collected 37 soil samples from the plow layers of paddy fields in Luzon, Leyte, Panay, and Mindanao in the Philippines in 2016 and 2017 (the ‘2010s’) and compared their fertility-related soil properties with published data for soil samples collected from the same or nearby sampling sites in 1969 (the ‘1960s’). Paddy soils in the Philippines had relatively high exchangeable calcium (Ca) and magnesium (Mg) content, cation exchange capacity (CEC), available silicon (Si) content, and total carbon (C) and nitrogen (N) content, reflecting their indigenous pedological background. We found that the 2010s soils had a lower available N content, particularly in wetter regions, despite the high input of N fertilizers, whereas the available phosphorus (P) showed a six-fold increase from the 1960s to the 2010s. The total potassium (K) content had significantly decreased during this period, possibly due to the mining effect of intensive farming with K-deficient fertilization. The 2010s soils contained a greater silt content and higher proportion of smectite in the clay fraction, which may have been transported from upstream via irrigation water. The increase in smectite corresponded with a significant increase in the cation exchange capacity, despite the organic C and clay contents tending to decrease, and these increases in smectite and soil pH may have contributed to the increase in the available fraction of Si that had not been applied as fertilizers. These findings demonstrate that paddy soil fertility in the Philippines changed in many ways over this 50-year period, largely owing to the direct impact of GR implementation in paddy fields but also potentially due to offsite effects from upland fields. The environmental impact of the nutrient loss and soil organic matter degradation behind the fertility improvement should be assessed holistically for the sustainable development of rice paddy ecosystems. (337 words)
1. Introduction
The Green Revolution (GR) was an agricultural innovation to increase food production that started with the development of the high-yielding rice variety IR8 at the International Rice Research Institute (IRRI) in the Philippines in the 1960s (Evenson and Gollin Citation2003). Other modern varieties of rice (MVs) have been developed since then, all of which have resistance to lodging at high nitrogen (N) levels but are susceptible to pest disease and so require a high input of both chemical fertilizers and pesticides (Herdt and Capule Citation1983). These biochemical innovations were supported by mechanical improvements, such as irrigation systems and farm mechanization. These combined technologies were first adopted in the Philippines, followed by India and the other Southeast and South Asian countries from the late 1960s (Eliazer Nelson, Ravichandran, and Antony Citation2019; Tran and Kajisa Citation2006). While the GR has tripled rice yields in Asia over the last 50 years, it has been criticized for its adverse impacts on human health (e.g., pesticide-induced diseases) and the environment (e.g., soil degradation and chemical runoff beyond the areas cultivated) (Pingali Citation2012; Zeigler and Mohanty Citation2010), which remain controversial and may differ greatly depending on the social, economic, and environmental circumstances of a given country.
The Philippines experienced a large increase in paddy rice production after the start of the GR. However, while the use of chemical fertilizers greatly supported this success, their nutritional imbalances have been implicated in chemical degradation of the soil (Asio et al. Citation2009). The updated Philippine National Action Plan to combat desertification, land degradation, and drought (PNAP) reported that the long-term use of urea alone or the excessive use of N compared with other nutrients resulted in silent soil degradation known as soil mining (Department of Agriculture Citation2010). For example, macro- and micronutrient mining from the mineral phases caused by excessive N fertilization relative to other nutrients resulted in reduced yields due to deficiencies in K (Cassman, Peng, and Dobermann Citation1997) and zinc (Zn) (Yoshida, Ahn, and Forno Citation1973) in the Philippines. Furthermore, a long-term continuous cropping experiment at IRRI revealed that N deficiency caused a yield decline even with a high annual input of N fertilizers (Dobermann et al. Citation2000), which Cassman et al. (Citation1996) attributed to a decreased crop uptake of soil organic N that becomes available through the mineralization of soil organic matter. However, while these surveys suggested that paddy soils have a decreased ability to supply nutrients from both the mineral and organic phases; nationwide trends in the changes in soil fertility in the Philippines have never been fully elucidated.
Farm-level studies across a wide range of areas in the Philippines observed nutrient deficiencies in the paddy soils (Dobermann and Oberthür Citation1997; Miura and Badayos Citation1999; Palanog et al. Citation2019), but it was difficult to determine whether these were due to the fertility changes that occurred after GR implementation or the intrinsic soil properties. One effective way to clarify the long-term changes in soil fertility as a result of the GR would be to conduct a follow-up survey on paddy fields where a fertility survey had been conducted before GR implementation. An ideal research dataset for this purpose is that of Kawaguchi and Kyuma (Citation1977), who collected paddy soils from across the Philippines and other Asian countries in 1969 to comprehensively assess the soil fertility status. Follow-up surveys to clarify changes in soil fertility in response to GR implementation have already been conducted in some of the Asian countries sampled by Kawaguchi and Kyuma (Citation1977), which have shown that soil degradation has occurred in Bangladesh (Ali et al. Citation1997) whereas an improvement in fertility status has resulted in Indonesia (Darmawan et al. Citation2006) and Thailand (Yanai et al. Citation2020). Thus, the soil response to GR implementation appears to differ among countries and has not yet been fully elucidated in the Philippines.
Therefore, the objective of this study was to determine the current fertility-related soil properties in paddy fields in the Philippines and to compare these with those observed by Kawaguchi and Kyuma (Citation1977) to evaluate the long-term changes in paddy soil fertility status over the last 50 years.
2. Materials and methods
2.1. Natural setting of the Philippines
The Philippines is an archipelago of 7,109 islands that extend from 5° to 21° north and from 116° to 127° east (PHIVOLCS, Citation2020). Magmatic arcs, including 24 currently active volcanoes, have formed in most of the archipelago except for islands in the province of Palawan from the subduction of the Sunda-Eurasian plate from the west and the Philippine Sea plate from the east (Yumul et al. Citation2008). Consequently, the surface geology is mostly igneous rocks or sediments that originated from andesitic-basaltic volcaniclastic materials (Schopka, Derry, and Arcilla Citation2011).
According to the Philippine Atmospheric, Geophysical and Astronomical Services Administration, the Philippines has a tropical climate, with a mean annual temperature of 26.6°C calculated from weather stations across the archipelago but excluding the highland City of Baguio and a wide variation in annual rainfall among regions, ranging from 965 to 4,064 mm (PAGASA, Citation2020). The western half of this nation is relatively dry with a tropical monsoon (Am) climate, whereas the eastern half is more humid with a tropical rainforest (Af) climate according to the Köppen climate classification. The climate of the Philippines can be further subdivided into Climates I to IV according to the modified Corona classification (Corporal-Lodangco and Leslie Citation2017) (). Based on comparisons of the long-term changes in average precipitation between these four climate types (Manzanas et al. Citation2018), the western parts of Luzon, Mindoro, Negros, and Palawan can be classified as Climate I, which has two pronounced seasons–a pronounced rainy period from June to August and a distinctively dry period from December to February; regions along or very near to the eastern coast can be classified as Climate II, which is characterized by the absence of a dry season but has a very pronounced maximum rain period from December to February; the central part of Cagayan, Isabela, parts of Western Mindanao, and most of Eastern Palawan can be classified as Climate III, which does not have pronounced wet or dry seasons; and southern Bicol, Leyte, and the central part of Mindanao can be classified as Climate IV, which is similar to Type III but wetter. Thus, there is a climatic gradient in the order I, III, IV, and II from west to east in the Philippines.
Figure 1. Distribution of the sampling sites in the Philippines in the 1960s and 2010s.Longitudinal lines with different color indicate the borders of climatic regions according to the Corona’s classification. The areas to the west of red line associate with Climate I, those to the east of blue lines associate with Climate II, those inside of the yellow lines or green lines were Climate III and IV, respectively
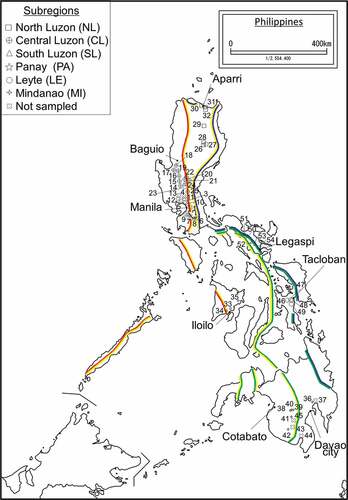
The major soil types in the Philippines are Inceptisols (48.8%), Ultisols (27.0%), Alfisols (13.2%), and Entisols (5.1%) according to the USDA Soil Taxonomy (Carating, Galanta, and Bacatio Citation2014), while Andosols occupy only 5% of the cultivated area, despite the abundance of volcanoes. In lowland areas, Entisols and Inceptisols are dominant, which have a high organic matter status, base status, available silicon (Si) content, and clay content compared with other tropical Asian lowland soils (Miura and Badayos Citation1999).
2.2. Paddy rice production in the Philippines
The average rice yield in the Philippines tripled over the past 50 years, increasing from 1.3 t ha−1 in 1966 to 4.0 t ha−1 in 2018 (FAOSTAT Citation2020), with a period of stagnation at ~2.8 t ha−1 from the 1980s to the mid-1990s (Dobermann et al. Citation2000; Umetso, Lekprichakul, and Chakravorty Citation2003). This increase was supported by several GR technologies. Many MVs that were descendants of IR8 were released and more rapidly adopted in the Philippines than in any other country, with the proportion of the total rice area planted to MVs increasing from 3% in 1966 to >75% by 1980 and 90% by 1990 (Brennan and Malabayabas Citation2011). The most popular MVs currently cultivated are reported to be the inbred varieties NSIC Rc222 and Rc216 (Glover, Kim, and Stone Citation2020). Since MVs have higher nutrient requirements than traditional rice varieties, this resulted in increasing amounts of chemical fertilizers, particularly N, being applied to paddy fields. For example, the Central Luzon Loop Survey dataset (Moya et al. Citation2015) showed that the amount of N, phosphorus (P), and K fertilizers applied increased from 9, 4, and 5 kg ha−1, respectively, in 1966 to 115, 15, and 19 kg ha−1, respectively, in 2011 during the wet season and from 20, 7, and 11 kg ha−1, respectively, in 1966 to 119, 15, and 21 in 2011 kg ha−1, respectively, in 2011 during the dry season. Wet-season crops are cultivated with or without irrigation, while dry season crops can only be cultivated with irrigation, and the irrigation ratio in the Philippines also sharply increased from approximately 30% in the early 1960s to 40% in the mid-1960s and then gradually increased to >60% in the early 1990s (Hayami and Kikuchi Citation1999). Irrigation infrastructure systems are particularly well developed in Central Luzon, Ilocos, Cagayan Valley, and Southern Tagalog (Estudillo and Otsuka Citation2006), which has enabled double- and triple-cropped rice systems to become the dominant agricultural land use in these areas.
Mechanical tools have also been adopted in the Philippines as labor-saving technologies. However, smaller machinery has been favored over larger machinery, and animals such as carabao or water buffalo (Bubalus bubalis) are still used alongside this, particularly for land preparation at the sides and corners of paddy fields (Umetso, Lekprichakul, and Chakravorty Citation2003). This small-scale mechanization and the retention of animal use can be explained by the partitioning of farms into smaller areas as they are passed on from one generation to the next (Moya et al. Citation2015).
2.3. Soil sampling and interview survey
Paddy soils at a depth of 0–15 cm were sampled in September and December 2016 and January and August 2017 from 37 locations distributed in six subregions throughout the Philippines: 7 in Cagayan Valley, 13 in Luzon Central Plain, 4 in Bicol Peninsula, 3 in Panay coastal plain, 4 in Northern Leyte, and 6 in Southern Mindanao (). These locations were at or near some of the sites where Kawaguchi and Kyuma (Citation1977) collected soil samples in 1969. Their data on the surface soils (lower boundary = 12.9 ± 3.0 cm in depth, n = 37) were used as a reference or ‘an anchor to the past’ for the evaluation of long-term changes up to the present. Since Kawaguchi and Kyuma (Citation1977) recorded how to approach their sampling sites in detail (e.g., a direction and a distance from a nearby cross road), we were able to return there or at least an adjacent field. The other 17 locations where they collected soil samples were either no longer used for rice production as a result of urbanization (i.e., Ph1–6, 9–13, and 23 in Luzon Central Plain, and Ph54 in Bicol Peninsula) or inaccessible due to travel restrictions (i.e., Ph37 and 43–45 in Mindanao). In terms of climatic region, 13 of these soils belonged to Climate I, 15 belonged to Climate III, and 9 belonged to Climate IV. No soil samples were collected from Climate II in this study.
Along with the soil sampling, an interview survey was conducted in Tagalog with local farmers who knew the details of the paddy field management in each sample field. Interview items included planting times, the cultivated area, the types of rice cultivars planted, the types of organic and inorganic fertilizers applied, and the methods of weeding, plowing, and irrigation.
2.4. Analytical methods
The soil samples were air-dried and sieved to <2 mm prior to chemical analysis. The fertility-related physicochemical properties of the samples were then investigated using the same analytical methods as Kawaguchi and Kyuma (Citation1977) in the 1960s, with the exception of total carbon (TC) and total N (TN).
Briefly, the soil pH was measured using the glass electrode method with a soil/water ratio of 1:2.5 (pH/Ion Meter F-23; HORIBA, Japan). TC and TN were determined with the dry combustion method, whereby finely ground soil samples were oven-dried at 110°C for approximately 24 h (Sparks, Citation1996) and then analyzed with an NC analyzer (Sumigraph NC-95A; Sumika Chemical Analysis Center, Japan) and a gas chromatograph (GC8A; Shimadzu, Japan). The available nitrogen (Av-N) was determined by incubating each soil sample at 40°C for 2 weeks and then extracting the Av-N with 2 mol L−1 KCl solution and evaluating the NH4+ concentration using the Kjeldahl method. Total P (TP) was determined by energy-dispersive X-ray fluorescence spectrometry (EDXRF) (XEPOS C; SPECTRO Analytical Instruments, Germany) using pressed powder pellet samples (Sparks et al. Citation1996). The available P (Av-P) was extracted from each soil sample using NH4F and HCl mixed solutions (Bray No. 2 method) and the P content of the extract was determined by colorimetry with an absorption spectrophotometer (UVmini 1240; Shimadzu, Japan) (Bray and Kurtz Citation1945). In addition, the P extracted with 0.2 mol L−1 hydrochloric acid (HCl-P) was determined by colorimetry with an absorption spectrophotometer (UVmini 1240; Shimadzu, Japan).
Exchangeable calcium (ex-Ca), magnesium (ex-Mg), K (ex-K), and sodium (ex-Na) were extracted using 1 mol L−1 neutral ammonium acetate and their levels were determined using an atomic absorption spectrophotometer (AA6200; Shimadzu, Japan). The cation exchange capacity (CEC) of the soil was determined by extracting each sample with 10% NaCl solution after extraction of the exchangeable bases and then evaluating the NH4+ concentration using the indophenol method (Sparks et al. Citation1996). The available Si (Av-Si) was extracted using 1 mol L−1 acetate buffer (pH 4.0) and the Av-Si content of the extract was assessed by colorimetry using an absorption spectrophotometer (Uvmini 1240, Shimadzu, Japan). The contents of ammonium oxalate soluble aluminum and iron (Alo and Feo) were measured by inductively coupled plasma-optional emissions spectroscopy (ICP-OES) (iCAP 7400 Duo Full MFC; Thermo Fisher Scientific, USA). P retention (Pre) was determined according to Blakemore, Searle, and Daly (Citation1987). The total contents of P, Al, Fe, Si, Ca, Mg, Na, and K were determined with an energy-dispersive X-ray fluorescence (EDXRF) spectrometer (XEPOS C; SPECTRO Analytical Instruments, Germany) using pressed powder pellet samples and by calibration with 18 standard soil samples with certified concentrations of these elements.
The particle-size distribution of the soil was determined using sieving and sedimentation methods (Klute Citation1986). Finally, the types of clay minerals in the ≤2-µm soil particles were identified by X-ray diffraction (XRD) analysis (MiniFlex 600; Rigaku, Japan). The samples were pretreated with Mg-saturation at room temperature (Mg-25°C) followed by glycerol (Mg-gly) or with K-saturation at room temperature (K-25°C) followed by heating at 350°C (K-350°C) and 550°C (K-550°C). The XRD patterns were then obtained by orienting the samples on glass slides and step-scanning these from 3 to 20 °2θ in increments of 0.02 °2θ. The relative peak area ratios of 7, 10, and 14 Å on the XRD analysis charts for Mg-25°C specimens were then calculated to evaluate the clay mineral contents.
2.5. Data comparison and statistical analyses
The analytical data published by Kawaguchi and Kyuma (Citation1977) were used as the soil samples from the 1960s. Since the TC and TN contents of these soil samples were determined using the Tyurin and Kjeldahl methods, they were multiplied by 1.2 and 1.1, respectively, to allow comparison with values obtained for the soil samples analyzed in the 2010s. These adjustment factors were determined from a preliminary experiment using the dry combustion method (data not shown).
The mean values of the soil properties of the 37 samples evaluated in both the 1960s and the 2010s were compared using paired-sample t-tests both for the overall data and the data from each region. Note that the 1960s data for the 17 urbanized or inaccessible locations were not included in the statistical analysis. In addition, the mean values of the soil properties were compared among the climatic regions using analysis of variance and Tukey’s test. In these analyses, the original data were used for pH, ex-Ca, ex-Mg, CEC, Av-Si, and the silt and clay contents, as equality of the variances was confirmed by Shapiro-Wilk test, while log-transformed data were used for all other parameters for which equality of the variances was not confirmed. All analyses were performed using the SYSTAT 13 software (SYSTAT, Chicago, IL). The significance level used here was P < 0.05.
3. Results
3.1. Properties of paddy soils in the 2010s
Summarized values for the fertility-related properties of paddy soils in the Philippines in the 2010s are provided in (), while the individual data for each sampled paddy field are listed in Table s1. The overall mean soil pH was 6.62, which is slightly acidic but close to neutral. The overall means (and ranges) for the sand, silt, and clay contents were 22.3% (2.4%–66.6%), 44.3% (24.6%–71.5%), and 33.4% (6.3%–56.9%), respectively. The overall mean CEC was 30.9 cmolc kg−1 (range, 7.9–64.6 cmolc kg−1), while the mean ex-Ca, ex-Mg, ex-K, and ex-Na contents were 18.9, 8.6, 0.40, and 0.57 cmolc kg−1, respectively. Together, these data indicate that paddy soils in the Philippines are very slightly acidic and have a clay loam texture and a relatively high CEC content, demonstrating that they are rich in exchangeable base cations.
Table 1. Mean, median, minimum, maximum, and coefficient of variation of the fertility-related properties of paddy soils in the Philippines in the 2010s (n = 37)
The overall mean TC and TN contents were 18.5 and 1.81 g kg−1, respectively, with an average C/N ratio of 10.2. The mean Av-N content was 144 mg kg−1, which corresponded to approximately 8.0% of the TN content. The mean TP content was 0.76 g kg−1, while the overall mean Av-P and HCl-P contents were 65.2 and 87.0 mg kg−1, which corresponded to approximately 8.6% and 11.4% of the TP content. The mean Av-Si content was 295 mg kg–1. The coefficient of variation (CV) was relatively high (>60%) for the Av-N, Av-P, and ex-K contents but as low as ~40% for Av-Si. The mean Alo + 1/2Feo value was 4.6 g kg−1 (range, 0.6–10.7 g kg−1). The mean Pre value was 25.0% (range, 2.3%–55.7%).
The XRD patterns of the Mg-gly clay specimens are presented in (). Most specimens exhibited a prominent peak at 1.72 nm d-spacings, which generally shifted from 1.4 nm d-spacings of the Mg-25°C specimens, indicating the presence of smectite. In addition, the peak at 1.4 nm shifted to 1.0–1.2 nm after K-saturation (K-25°C) (data not shown), indicating that vermiculite was also present in the clay fractions whereas hydroxy-interlayered minerals were almost absent. Kaolinite, which was represented by 0.7 nm d-spacings in the XRD, was the second most dominant clay mineral, whereas no other minerals were clearly detected. A few specimens exhibited neither the diffraction peak for smectite nor kaolinite (i.e., Ph48, 49, 53), suggesting the absence of crystalline aluminosilicates.
Figure 2. XRD peak patterns for the clay specimens after Mg-saturation and glycerol solvation (Mg-gly) for 2010s. A broad peak corresponding to 1.72 nm d-spacing indicate the presence of smectite. The other peaks corresponding to 1.4 nm, 1.0 nm, 0.7 nm d-spacings indicate the presence of vermiculite, micaceous mineral (illite), and kaolinite, respectively
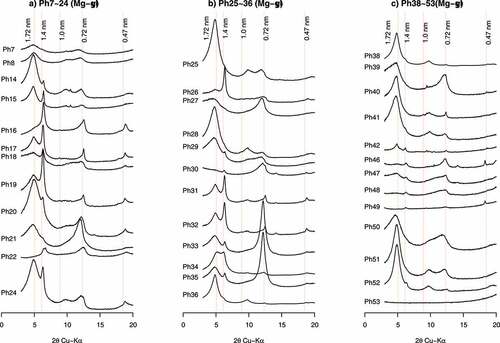
Comparison of the mean values of the fertility-related properties among the six subregions revealed that there were no significant regional differences in most of the soil properties. However, comparison of the mean values among climatic regions I, III, and IV revealed that the soils in Climate IV had significantly higher TN (p < 0.05) and Av-N (p < 0.05) contents, whereas those in Climate I had significantly higher soil pH (p < 0.05) than the soils in the other climatic regions ().
Table 2. Differences in the means of the fertility-related properties of paddy soils depending on climatic regions in the Philippines in the 2010s
3.2. Comparison of the soil properties between the 1960s and 2010s
The mean values of the soil properties in the 1960s and the 2010s are presented in (), only for those showing a significant difference between the ages, while the raw data of all the properties investigated are presented in Table s1. The mean Av-N content ()) decreased from 205 mg kg−1 to144 mg kg−1 (p < 0.05). There was a particularly large change in the mean Av-P content ()), which showed a 6.0-fold increase from 11 mg kg−1 in the 1960s to 65.2 mg kg−1 in the 2010s (p < 0.01). However, the increase in TP ()) was several times larger than that of Av-P, indicating that >80% of P accumulated in the soils was present in non-available forms. The mean HCl-P content ()) also increased from 55.2 mg kg−1 to 87.0 mg kg−1 (p < 0.01). The mean Av-Si content ()) significantly increased from 208 mg Si kg−1 to 295 mg Si kg−1 (p < 0.01). The mean silt content ()) significantly increased from 31.4% to 44.3% (p < 0.01), whereas the sand ()) and clay contents ()) significantly decreased from 28.7% to 22.3% and from 39.9% to 33.4% (p < 0.01), respectively. Finally, CEC ()) significantly increased from 24.9 cmolc kg−1 to 30.9 cmolc kg−1 (p < 0.01).
Figure 3. Changes in the selected fertility-related properties of the soils from the 1960s (closed) to the 2010s (open) in the Philippines. Paired t-test. *: p < 0.05; **: p < 0.01. Bars indicate standard errors
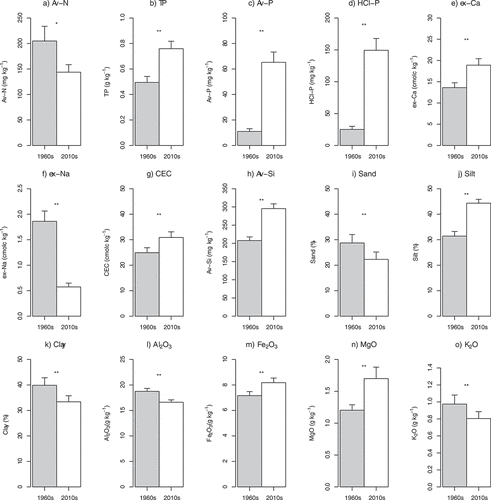
Comparisons of elemental composition revealed that total SiO2 content was not significantly different between the 1960s and the 2010s. However, Fe2O3 and MgO contents were significantly increased, whereas Al2O3 and K2O contents were decreased during these 50 years ()). All of the major elements investigated (i.e., SiO2, Al2O3, Fe2O3, CaO, MgO, and K2O) showed significant positive correlations between the 1960s and 2010s (p < 0.01) (). Although the data plots were inclined above the 1:1 line for Fe2O3 and MgO or below the line for Al2O3 and K2O, they were plotted close to the 1:1 line without a few exceptions, indicating that variations between sites appears not largely changed during these 50 years.
Figure 4. Differences between 1960s and 2010s concerning total content of SiO2 (a), Al2O3 (b), Fe2O3 (c), MgO (d), CaO (e), and K2O (f). **: p < 0.01, ***: p < 0.001
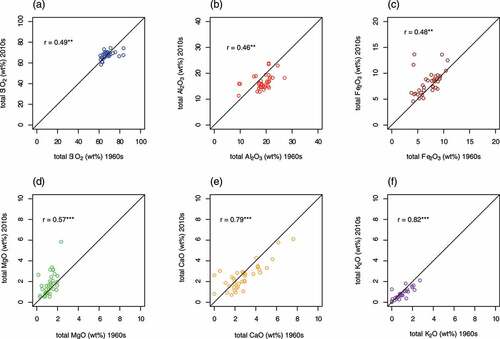
Changes in the XRD peak area ratios of 0.7-nm, 1.0-nm, and 1.4-nm minerals for the Mg-25°C specimens are presented in (). The mean peak area ratio of 1.4 nm, which was mostly derived from smectite (), significantly increased from 61.7% in the 1960s to 83.2% in the 2010s (p < 0.01). By contrast, the mean peak area ratio of the 0.7-nm mineral (i.e., kaolinite) significantly decreased from 33.8% to 16.5% (p < 0.01). Thus, the composition of the clay minerals became enriched with smectite and exhibited a decreased proportion of kaolinite over the 50-year period. These changes in clay mineralogy well corresponded to both the significant increase in Mg and Fe, major components of smectite [2:1, e.g., (Na,Ca)0.33, Al1.67 (Fe2+,Mg2+)0.33 (Si4O10) (OH)2·nH2O] and to the decrease in total Al content, major component of kaolinite [1:1, Al2Si2O5(OH)4].
3.3. Interview survey
The results of the interview survey are summarized in (). The double-cropping system was dominant in the surveyed fields, with the triple-cropping system being less common and the single-cropping system being very rare. The mean cultivated area was 2.4 ha, but this varied greatly from 0.3 to 8 ha. A total of 30 different rice cultivars were used by the farmers. However, more than 60% of the farmers surveyed cultivated Rc222 alone or multiple varieties that included Rc222 and Rc216. The mean amounts of N, P (P2O5), and K (K2O) added as inorganic fertilizers during a single crop season were 82 ± 34, 34 ± 21, and 27 ± 24 kg ha−1, respectively. Urea was applied by more than 90% of the farmers, 80% of whom used complete fertilizer (N-P2O5-K2O wt% = 14–14-14) and 50% of whom used ammonium phosphate fertilizer (16–20-0) in combination. Other types of chemical fertilizers, including K, sulfur (S), or Zn, were also applied in some cases, but this practice was not very common. More than half of the farmers applied no organic fertilizers. In many cases, weeding was performed with a herbicide, but manual weeding (i.e., by hand or machete) or a combination of herbicide use and manual weeding was also common. Approximately 60% of the farmers used a hand tractor for plowing, whereas 25% still used carabao for this purpose. More than 90% of the farmers drew irrigation water, approximately half of whom used the national irrigation system while the remainder used private shallow wells or water pumps. Thus, the representative style of farming in the surveyed fields was the small-scale double-cropping of Rc222 or Rc216 with chemical fertilizers (mainly urea and complete fertilizer), the application of herbicide, the drawing of irrigation water, and plowing with a hand tractor.
Table 3. Summarized information of the interview survey to local farmers on paddy field management (n = 37)
4. Discussion
4.1. Current soil fertilities in the Philippines and their pedological background
Kawaguchi and Kyuma (Citation1977) compared the fertilities of paddy soils among nine tropical Asian countries (Bangladesh, Myanmar, Cambodia, India, Indonesia, Malaysia, the Philippines, Sri Lanka, and Thailand) in the 1960s by using factor analysis to obtain three fertility factors [inherent potentiality (IP), organic matter and N status (OM), and available P status] from 11 soil properties (TC, TN, Av-N, TP, Av-P, HCl-P, ex-Ca + Mg, ex-K, CEC, Av-Si, and sand content) obtained from a total of 410 paddy fields. They found that paddy soils in the Philippines had the highest IP scores, which were positively correlated with ex-Ca + Mg, CEC, and Av-Si, and the second highest OM scores, which were positively correlated with TC, TN, and Av-N. The present study showed that these general trends were still present in these soils in the 2010s.
The relatively high levels of ex-Ca + Mg, CEC, and Av-Si in paddy soils in the Philippines can be explained by their indigenous pedological background, i.e., the andesitic-basaltic lithology, hot and humid climate, and lowland plain topography. Andesitic-basaltic volcanic rocks contain fine, weatherable primary minerals, such as plagioclase and augite, which weather rapidly under a tropical climate (i.e., higher temperatures with precipitation) to liberate rock-forming elements such as Ca, Mg, Si, and Al (Benedetti et al. Citation2003). While cations with low ionic potentials, such as Ca2+ and Mg2+, are solubilized in water as hydrated cations, a large proportion of the Si and almost all of the Al can be precipitated as hydroxides [e.g., Si(OH)4 and Al(OH)3] and polymerized to form secondary minerals (Sposito Citation2008). River water running through andesitic-basaltic bedrock areas dissolves relatively high concentrations of alkali, alkali-earth cations, and Si(OH)4 monomers (Ibarra et al. Citation2016), which can replenish these nutrient elements in lowland paddy soils via irrigation water. However, higher temperatures facilitate mineral crystallization, resulting in short-range ordered (SRO) minerals such as allophane and imogolite being unstable and promptly crystalizing into aluminosilicate minerals (Rasmussen, Dahlgren, and Southard Citation2010). A lower abundance of SRO minerals in the study soils was evidenced by the fact that all of the Alo + 1/2Feo and Pre values were below the diagnostic criteria for andic horizons (i.e., <20 g kg−1 and <85%, respectively). Consequently, smectite, which is a high-activity clay with a CEC of ~100 cmolc kg−1 (Środoń and McCarty Citation2008), becomes dominant in place of kaolinite in the soils on lowland plains () because this topography is favorable for maintaining a higher pH and concentrations of Si(OH)4 and Mg2+ in the soil solution (Rajmohan and Elango Citation2004). A few soils (i.e., Ph30, 48, 49), which had relatively high Alo + 1/2Feo content > 7.0 g kg−1 and Pre value >40%, and showed no specific peak of smectite in XRD () may be an intermediate pedogenic state from Andosols to Inceptisols or affected by volcanic ash deposition to a greater extent than the other locations.
In contrast to the relatively homogeneous inorganic soil properties, those relating to soil organic matter showed large variations among the different climatic regions. The TC, TN, and Av-N contents tended to increase in the order of Climate I, II, and IV, which equates to increasingly short dry periods within a year (), but were not significantly correlated with the Alo + 1/2Feo content, which is a representative indicator of the SRO mineral content in the soil. This result is inconsistent with global trends, in which the soil organic C content is weakly correlated with climatic variables and significantly correlated with variables related to SRO minerals (Rasmussen et al. Citation2018). This discrepancy may be caused by the Alo + 1/2Feo content exhibiting only small variation (CV < 50%) in contrast to the wide range of annual precipitation (965–4,064 mm) in the Philippines. Soil organic matter decomposition should be slower under continuously wet or submerged soil conditions in regions with a shorter dry season, which is likely to lead to an increase in the TC, TN, and Av-N contents (). This climatic control of soil organic matter should be taken into account when considering the C and N dynamics in paddy fields in the Philippines.
4.2. Fertilizer-induced changes in soil properties
Our comparative analysis revealed changes in the fertility-related soil properties in paddy fields in the Philippines from the 1960s to the 2010s, most of which appear to be related to chemical fertilizer application. The interview survey showed that most rice farmers at our study sites preferentially used N-dominant fertilizers over those containing other components (P and K) (), which is consistent with the national trends reported in other recent studies (Glover, Kim, and Stone Citation2020; Moya et al. Citation2015; Silva et al. Citation2017). However, this preferential use of N fertilizers does not necessarily increase the Av-N content in paddy fields because of the enhanced mineralization of soil organic matter (Dawe et al. Citation2000) and rice straw burning (Mendoza Citation2015). The accumulation of chemically stable organic N (e.g., N covalently bound to phenolic lignin residues) (Olk et al. Citation1996) has also been attributed to a decrease in Av-N contents in intensively cropped irrigated paddy fields in the Philippines. Olk et al. (Citation2006) emphasized that the chemically-stable phenolic N compounds accumulated in paddy fields under long-term submerged conditions, induced by the annual double or triple cropping systems. This theory was consistent to the trends observed in our study, particularly for soils in wetter climatic regions. The TC content had decreased from the 1960s to the 2010s for approximately half of the soils in Climate I and most of the soils in Climates III and IV ()). Similarly, the Av-N content had largely decreased over the same period ()), but the TN content had not decreased to the same degree or rather increased especially in Climate I ()), leading to a decrease in the ratio of Av-N to TN content from 7.8% to 5.9% in Climate I, 10.9% to 7.8% in Climate III, and 16.6% to 9.6% in Climate IV. Since yield issues in the Philippines are frequently associated with an insufficient uptake of mineralized soil N even when the TN content remains constant or is increased through the input of fertilizers (Olk et al. Citation2006), the application of organic fertilizer (e.g., pig or chicken manure, compost, or vermicompost), which is not very common at present (), is recommended to increase the amount of mineralizable organic N in the paddy fields (Diekmann et al. Citation1996; Quimbo, Mamaril, and Tafere Citation2014).
Figure 6. Differences in TC (a), TN (b), and Av-N (c) between 1960s and 2010s depending on the climatic regions. Each box-and-whisker shows five-number summary of a set of data: minimum, lower quartile, median, upper quartile, and maximum, whereas filled circle shows outliers
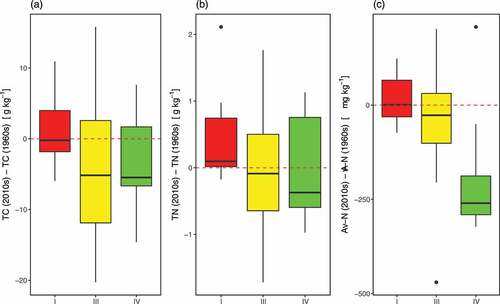
The Av-P content increased from the 1960s to the 2010s in all of the paddy fields examined, which was certainly caused by a higher input of chemical P fertilizers that occurred after the GR (Moya et al. Citation2015). Since most P fertilizers contain calcium phosphate as the active ingredient, the observed increase in ex-Ca also probably occurred as a result of P fertilizer application in most cases. Similar increasing trends in both Av-P and ex-Ca were also observed in follow-up surveys in other countries, including Indonesia (Darmawan et al. Citation2006), Thailand (Yanai et al. Citation2020), and Malaysia (Tanaka et al. Citation2020). Therefore, the accumulation of fertilized P in paddy soils is a direct impact of the GR not only in the Philippines but in many tropical Asian countries. Despite this overall increasing trend in Av-P, approximately 15% of the soils still showed an Av-P deficiency for rice production based on the criterion of IRRI (i.e., <20.0 mg P kg−1). Therefore, further input of P fertilizers in accordance with plant requirements is recommended for soils showing an Av-P deficiency. While P showed an accumulation trend, it should be noted that P also shows the highest mobility at soil pH 6–7 (Barrow Citation2017), which was typical of the paddy soils included in our study (). Therefore, elucidation of whether P applied in paddy fields is accumulated in the soils or leached with the drainage of irrigation water is critical for better land management to achieve both agricultural and environmental sustainability.
Unlike N and P, there has been insufficient fertilization of paddy soils with K to meet the demands of rice production in the Philippines and other Asian countries, resulting in K mining from the soil reserves becoming an issue (Dobermann, Cruz, and Cassman Citation1996). The progress of K mining was apparent at the study sites, with a significant decrease in the total K content from the 1960s to the 2010s ()). Furthermore, the ex-K content also showed a decreasing trend (Table s1), which was probably accelerated by the competition for ion exchange sites between K+ and Ca2+ applied as P fertilizers. To maintain the indigenous K source in the soil, it is highly recommended that the remaining stubble and straw is incorporated into the soil to improve the K balance and help conserve other soil nutrients with lower fertilization rates, such as Zn and Si (Dobermann and Fairhurst Citation2002). Rice straw is currently not incorporated but burned widely in the Philippines, which causes almost complete N loss, and considerable losses of K and other nutrients (Mendoza Citation2015).
4.3. Other factors affecting the changes in soil properties
Pearson correlation analysis showed that there were strong positive correlations between the 1960s and 2010s for the sand (r = 0.81, p < 0.001) and clay (r = 0.71, p < 0.001) contents, indicating that the soil texture had basically been maintained over this 50-year period. Nevertheless, the soil texture was shifted into more silty and less sandy (i.e., siltation) for most of the study soils from the 1960s to the 2010s. The textural modification was unexpected, as soil texture is typically stable irrespective of the farming practices used. While we first thought that mechanical plow toward deeper depth may cause textual changes in the plow layers, it would not be a main reason because siltation occurred even in the paddy fields conventionally plowed by hand or carabao (Ph20, 21). One possible source of silt particles is volcanic ash deposition. Big eruptions that occurred during this period in the Philippines were from Mount Mayon, Mount Pinatubo, and Taal Volcano (Castillo and Newhall Citation2004; Leone and Gaillard Citation1999). Paddy fields to the north of Mount Pinatubo (Ph 14–16) or the nearby field from Mount Taal (Ph7) showed relatively large increase in silt content, which may had been influenced by direct deposition of volcanic ashes. These eruptions, however, cannot be a single factor explaining the nationwide trend toward the siltation of paddy soils. Another probable source of silt particles was the transport of silt from upstream areas via irrigation water, as revealed by Magahud and Sanchez (Citation2016), who found that silt particles were more abundant in soils near the irrigation entrance than in the middle of the paddy field. It has been reported that irrigation-based soil siltation in lowland areas is enhanced by severe soil erosion in upland deforested fields (Asio et al. Citation2009). Furthermore, the rice yield in Bukidnon Province, Northern Mindanao, decreased by 27% over a period of 5 years in farms that were heavily affected by siltation of the irrigation canal followed by water shortage (Lantican, Guerra, and Bhuiyan Citation2003). Thus, soil siltation in paddy fields may have been accelerated by volcanic ash deposition and upland soil erosion, though this requires further elucidation in the future by considering land connectivity via water systems.
Although the mechanism behind the increase in smectite and decrease in kaolinite in the soils () remains elusive, this mineralogical modification largely improved the chemical soil fertility. Smectite is a dominant clay mineral in suspended sediments in river water in the Philippines (Liu et al. Citation2016), which may be incorporated into lowland paddies via irrigation water. In addition, the in situ formation of smectite in paddy soils can be promoted by a higher soil pH (Hassannezhad et al. Citation2008), possibly as a result of a decrease in the soil redox potential (Eh) followed by an increase in pH during the long flooding period caused by the increased rice-cropping times. This increase in highly active smectite increased the CEC of the paddy soils despite the decrease in clay and TC contents () and may also have contributed to the increase in Av-Si content, as smectite can support relatively high levels of Si in the soil solution. While chemically advantageous, smectite enrichment may have adverse effects on the physical properties of the soil. For instance, it may cause the formation of hard and compact layers after continuous machinery plowing, which decreases the soil porosity, and the decrease in soil organic matter content can make soil compaction even worse. Hence, the increase in smectite from the 1960s to the 2010s should be carefully evaluated to obtain an overall understanding of the physical and chemical soil properties.
5. Conclusive remarks
This follow-up survey to the study of Kawaguchi and Kyuma (Citation1977) in the Philippines clearly showed nationwide increasing trends in the Av-P content and decreases in the total K content in paddy soils. There was also a decrease in the Av-N content and the proportion of Av-N to total N content, which was more pronounced in wetter climatic regions. In addition to these changes caused by chemical fertilizer application, soil siltation and smectite enrichment were also unexpectedly observed, which were probably influenced by the transport of eroded particles from upland fields via irrigation water. These properties and total elemental composition have been considered to be stable during decades and to be available for the evidence that the sampling points were the same between two periods. Since this study clarified that even these properties could be modified by intensive agriculture, proof that the sampling points are the same should be carefully ensured by other strategies. Although the increase in smectite content contributed to an improved chemical soil fertility, such as through an increased CEC and Av-Si content, it may have adverse effects on the physical properties of the soil if combined with a lower soil organic matter content and more frequent machinery plowing. Together, these findings indicate that the efficient and balanced use of both inorganic and organic fertilizers is a feasible option for achieving sustainable rice production and environmental conservation in the Philippines over the coming decades. However, the establishment of rational soil/fertilizer management schemes for both lowland and upland fields will be a particularly challenging issue for sustainable landscape management and will require the thorough consideration of land connectivity via water systems.
Supplemental Material
Download MS Excel (67 KB)Acknowledgments
The authors would like to express their deep gratitude to Dr. Kazutake Kyuma, Emeritus Professor of Kyoto University, and Dr. Toshiyuki Wakatsuki, Emeritus Professor of Shimane University, for their constructive suggestions on this research. This research was partly funded by the Japan Society for the Promotion of Science through a Grant-in-Aid for Scientific Research (B) (overseas academic) (No. 15H05247).
Disclosure statement
No potential conflict of interest was reported by the author(s).
Supplementary material
Supplemental data for this article can be accessed here.
Additional information
Funding
References
- Ali, M. M., S. M. saheed, S. M. saheed, S. M. saheed, and S. M. Saheed. 1997. “Soil Degradation during the Period 1967–1995 in Bangladesh: I. Carbon and Nitrogen.” Soil Science and Plant Nutrition 43 (4): 863–878. doi:10.1080/00380768.1997.10414653.
- Asio, V., R. Jahn, F. Perez, I. Navarrete, and S. Abit. 2009. “A Review of Soil Degradation in the Philippines.” Annals of Tropical Research 31 (2): 69–94. doi:10.32945/atr3124.2009.
- Barrow, N. J. 2017. “The Effects of pH on Phosphate Uptake from the Soil.” Plant and Soil 410 (1–2): 401–410. doi:10.1007/s11104-016-3008-9.
- Benedetti, M. F., A. Dia, J. Riotte, F. Chabaux, M. Gerard, J. Boulegue, B. Fritz, et al. 2003. “Chemical Weathering of Basaltic Lava Flows Undergoing Extreme Climatic Conditions: The Water Geochemistry Record.” Chemical Geology 201 (1–2): 1–17. doi:10.1016/S0009-2541(03)00231-6.
- Blakemore, L. C., P. L. Searle, and B. K. Daly. 1987. “Methods for Chemical Analysis of Soils.” New Zealand Soil Bureau Scientific Report 80. doi:10.7931/DL1-SBSR-10A.
- Bray, R. H., and L. T. Kurtz. 1945. “Determination of Total, Organic, and Available Forms of Phosphorus in Soils.” Soil Science 59 (1): 39–45. doi:10.1097/00010694-194501000-00006.
- Brennan, J. P., and A. Malabayabas. 2011. “International Rice Research Institute’s Contribution to Rice Varietal Yield Improvement in South-East Asia.” 111. ACIR Impact Assessment Series, 74. http://books.irri.org/IRRI-contribution-to-rice-varietal-yield-improvement-SEA.pdf
- Carating, R. B., R. G. Galanta, and C. D. Bacatio. 2014. ““Introduction.” Chap. 1.” In The Soils of the Philippines, edited by R. B. Carating, R. G. Galanta, and C. D. Bacatio, 1–49. Netherlands: Springer.
- Cassman, K. G., A. Dobermann, P. C. Sta Cruz, G. G. Gines, M. I. Samson, J. P. Descalsota, J. M. Alcantara, M. A. Dizon, and D. C. Olk. 1996. “Soil Organic Matter and the Indigenous Nitrogen Supply of Intensive Irrigated Rice Systems in the Tropics.” Plant and Soil 182 (2): 267–278. doi:10.1007/BF00029058.
- Cassman, K. G., S. Peng, and A. Dobermann. 1997. “Nutritional Physiology of the Rice Plants and Productivity Decline of Irrigated Rice Systems in the Tropics.” Soil Science and Plant Nutrition 43 (sup1): 1101–1106. doi:10.1080/00380768.1997.11863725.
- Castillo, P. R., and C. G. Newhall. 2004. “Geochemical Constraints on Possible Subduction Components in Lavas of Mayon and Taal Volcanoes, Southern Luzon, Philippines.” Journal of Petrology 45 (6): 1089–1108. doi:10.1093/petrology/egh005.
- Corporal-Lodangco, I. L., and L. M. Leslie. 2017. “Defining Philippine Climate Zones Using Surface and High-Resolution Satellite Data.” Procedia Computer Science 114: 324–332. doi:10.1016/j.procs.2017.09.068.
- Darmawan, K. K., T. Wakatsuki, T. Wakatsuki, T. Wakatsuki, T. Wakatsuki, and T. Wakatsuki. 2006. “Effect of Green Revolution Technology during the Period 1970-2003 on Sawah Soil Properties in Java, Indonesia: II. Changes in the Chemical Properties of Soils.” Soil Science and Plant Nutrition 52 (5): 645–653. doi:10.1111/j.1747-0765.2006.00054.x.
- Dawe, D., A. Dobermann, P. Moya, S. Abdulrachman, B. Singh, P. Lal, S. Y. Li, et al. 2000. “How Widespread are Yield Declines in Long-term Rice Experiments in Asia?” Field Crop Research 66 (2): 175–193. doi:10.1016/S0378-4290(00)00075-7.
- Department of Agriculture. 2010. “The Updated Philippine National Action Plan to Combat Desertification, Land Degradation and Drought (DLDD)” http://extwprlegs1.fao.org/docs/pdf/phi152609.pdf
- Diekmann, K. H., J. C. G. Ottow, S. K. De Datta, and S. K. De Datta. 1996. “Yield and Nitrogen Response of Lowland Rice (Oryza Sativa L.) To Sesbania Rostrata and Aeschynomene Afraspera Green Manure in Different Marginally Productive Soils in the Philippines.” Biology and Fertility of Soils 21 (1–2): 103–108. doi:10.1007/BF00336000.
- Dobermann, A., P. C. S. Cruz, and K. G. Cassman. 1996. “Fertilizer Inputs, Nutrient Balance, and Soil Nutrient-supplying Power in Intensive, Irrigated Rice Systems. I. Potassium Uptake and K Balance.” Nutrient Cycling and Agroecosystems 46 (1): 1–10. doi:10.1007/BF00210219.
- Dobermann, A., and T. H. Fairhurst. 2002. “Rice Straw Management.” Better Crop International 16: 7–11.
- Dobermann, A., and T. Oberthür. 1997. “Fuzzy Mapping of Soil Fertility - A Case Study on Irrigated Riceland in the Philippines.” Geoderma 77 (2–4): 317–339. doi:10.1016/S0016-7061(97)00028-1.
- Dobermann, A. D., R. P. R. Dawe, K. G. Cassman, and K. G. Cassman. 2000. “Reversal of Rice Yield Decline in a Long-term Continuous Cropping Experiment.” Agronomy Journal 92 (4): 633–643. doi:10.2134/agronj2000.924633x.
- Eliazer Nelson, A. R. L., K. Ravichandran, and U. Antony. 2019. “The Impact of the Green Revolution on Indigenous Crops of India.” Journal of Ethnic Foods 6 (1): 1–10. doi:10.1186/s42779-019-0011-9.
- Estudillo, J. P., and K. Otsuka. 2006. “Lessons from Three Decades of Green Revolution in the Philippines.” The Developing Economies 44 (2): 123–148. doi:10.1111/j.1746-1049.2006.00010.x.
- Evenson, R. E., and D. Gollin. 2003. “Assessing the Impact of the Green Revolution, 1960 to 2000.” Science 300 (5620): 758–762. September, 2020 doi:10.1126/science.1078710.
- FAOSTAT (Food and Agriculture Organization of the United Nations). 2020.“FAOSTAT” http://www.fao.org/faostat/en/#home
- Glover, D., S. K. Kim, and G. D. Stone. 2020. “Golden Rice and Technology Adoption Theory: A Study of Seed Choice Dynamics among Rice Growers in the Philippines.” Technology in Society 60: 101227. doi:10.1016/j.techsoc.2019.101227.
- Hassannezhad, H., A. Pashaee, F. Khormali, and M. Mohammadian. 2008. “Effect of Soil Moisture Regime and Rice Cultivation on Mineralogical Characteristics of Paddy Soils of Mazandaran Province, Northern Iran, Amol.” International Jounarl of Soil Science 3 (3): 138–148. doi:10.3923/ijss.2008.138.148.
- Hayami, Y., and M. Kikuchi. 1999. “The Three Decades of Green Revolution in a Philippine Village.” Japanese Journal of Rural Economy 1: 10–24. doi:10.18480/jjre.1.10.
- Herdt, R. W., and C. Capule. 1983. “Development and Inroduction of MVs, Philippines.” In Adoption, Spread, and Production Impact of Modern Rice Varieties in Asia, edited by R. W. Herdt and C. Capule, 14–16. Philippines: Instutute of Rice Research Institute.
- Ibarra, D. E., J. K. Caves, S. Moon, D. L. Thomas, J. Hartmann, C. P. Chamberlain, and K. Maher. 2016. “Differential Weathering of Basaltic and Granitic Catchments from Concentration–discharge Relationships.” Geochimica et Cosmochimica Acta 190: 265–293. doi:10.1016/j.gca.2016.07.006.
- Kawaguchi, K., and K. Kyuma. 1977. Paddy Soils in Tropical Asia. Their Material Nature and Fertility. Vol. 258. Honolulu: University Press of Hawaii.
- Klute, A., ed. 1986. Methods of Soil Analysis Part 1 – Physical and Mineralogical Methods. 2nd ed. 1188. Wisconsin: SSSA Book series: 5. American Society of Agronomy and Soil Science Society of America.
- Lantican, M. A., L. C. Guerra, and S. I. Bhuiyan. 2003. “Impacts of Soil Erosion in the Upper Manupali Watershed on Irrigated Lowlands in the Philippines.” Paddy and Water Environment 1 (1): 19–26. doi:10.1007/s10333-002-0004-x.
- Leone, F., and J. C. Gaillard. 1999. “Analysis of the Institutional and Social Responses to the Eruption and the Lahars of Mount Pinatubo Volcano from 1991 to 1998 (Central Luzon, Philippines).” GeoJournal 49 (2): 223–238. doi:10.1023/A:1007076704752.
- Liu, Z., Y. Zhao, C. Colin, C. Colin, K. Stattegger, M. G. Wiesner, C. A. Huh, et al. 2016. “Source-to-sink Transport Processes of Fluvial Sediments in the South China Sea.” Earth-Science Reviews 153: 238–273. doi:10.1016/j.earscirev.2015.08.005.
- Magahud, J., and P. Sanchez. 2016. “Soil Properties of Major Irrigated Rice Areas in the Philippines.” Asia Life Sciences 25 (1): 291–309.
- Manzanas, R., A. Lucero, A. Weisheimer, and J. M. Gutiérrez. 2018. “Can Bias Correction and Statistical Downscaling Methods Improve the Skill of Seasonal Precipitation Forecasts?” Climate Dynamics 50 (3): 1161–1176. doi:10.1007/s00382-017-3668-z.
- Mendoza, T. 2015. “Enhancing Crop Residues Recycling in the Philippine Landscape.” In Environmental Implications of Recycling and Recycled Products, edited by S. S. Muthu, 79–100, Singapore: Springer.
- Miura, K., and R. B. Badayos. 1999. “Evaluation of Soil Fertility Status of Lowland Areas in the Philippines.” Japan Agricure Research Quarterly 33 (2): 91–96.
- Moya, P., K. Kajisa, R. Barker, S. Mohanty, F. Gascon, M. Rose, and S. Valentin. 2015. Changes in Rice Farming in the Philippines : Insights from Five Decades of a Household-level Survey.Philippines: International Rice Research Institute.
- Olk, D. C., K. G. Cassman, E. W. Randall, P. Kinchesh, L. J. Sanger, and J. M. Anderson. 1996. “Changes in Chemical Properties of Organic Matter with Intensified Rice Cropping in Tropical Lowland Soil.” European Journal of Soil Science 47 (3): 293–303. doi:10.1111/j.1365-2389.1996.tb01403.x.
- Olk, D. C., K. G. Cassman, K. S. Rohr, M. M. Anders, J. D. Mao, and J. L. Deenik. 2006. “Chemical Stabilization of Soil Organic Nitrogen by Phenolic Lignin Residues in Anaerobic Agroecosystems.” Soil Biology and Biochemistry 38 (11): 3303–3312. doi:10.1016/j.soilbio.2006.04.009.
- PAGASA (Philippine Atmospheric Geophysical and Astronomical Services Administration). 2020. “Climate” http://bagong.pagasa.dost.gov.ph/climate
- Palanog, A. D., M. I. C. Calayugan, G. I. D. Empleo, A. Amparado, M. A. I. Asilo, E. C. Arocena, P. C. S. Cruz, et al. 2019. “Zinc and Iron Nutrition Status in the Philippines Population and Local Soils.” Frontier in Nutrition 6 (Article): 81. doi:10.3389/fnut.2019.00081.
- PHIVOCS (Philippine Institute of Volcanology and Seismology) 2020. “Volcanoes of the Philippines” https://www.phivolcs.dost.gov.ph
- Pingali, P. L. 2012. “Green Revolution: Impacts, Limits, and the Path Ahead.” Proceedings of National Academy of Sciences of the United States of America 109 (31): 12302–12308. doi:10.1073/pnas.0912953109.
- Quimbo, M. C., C. P. Mamaril, and K. L. S. Tafere. 2014. “Organic versus Inorganic Management on the Yield and Soil Fertility of Irrigated Lowland Rice.” Annals of Tropical Research 36: 32–49. doi:10.32945/atr3613.2014.
- Rajmohan, N., and L. Elango. 2004. “Identification and Evolution of Hydrogeochemical Processes in the Groundwater Environment in an Area of the Palar and Cheyyar River Basins, Southern India.” Environmental Geology 46: 47–61.
- Rasmussen, C., R. A. Dahlgren, and R. J. Southard. 2010. “Basalt Weathering and Pedogenesis across an Environmental Gradient in the Southern Cascade Range, California, USA.” Geoderma 154 (3–4): 473–485. doi:10.1016/j.geoderma.2009.05.019.
- Rasmussen, C., K. Heckman, K. W. R. Wieder, M. Keiluweit, C. R. Lawrence, A. A. Berhe, J. C. Blankinship, et al. 2018. “Beyond Clay: Towards an Improved Set of Variables for Predicting Soil Organic Matter Content.” Biogeochemistry 137 (3): 297–306. October, 2020. doi10.1007/s10533-018-0424-3.
- Schopka, H. H., L. A. Derry, and C. A. Arcilla. 2011. “Chemical Weathering, River Geochemistry and Atmospheric Carbon Fluxes from Volcanic and Ultramafic Regions on Luzon Island, the Philippines.” Geochimica et Cosmochimica Acta 75 (4): 978–1002. doi:10.1016/j.gca.2010.11.014.
- Silva, J. V., P. Reidsma, A. G. Laborte, and M. K. van Ittersum. 2017. “Explaining Rice Yields and Yield Gaps in Central Luzon, Philippines: An Application of Stochastic Frontier Analysis and Crop Modelling.” European Journal of Agronomy 82: 223–241. doi:10.1016/j.eja.2016.06.017.
- Sparks, D. L., A. L. Page, P. A. Helmke, R. H. Loeppert, P. N. Soltanpour, M. A. Tabatabai, C. T. Johnston, and M. E. Sumner. 1996. Methods of Soil Analysis Part 3 – Chemical Methods. SSSA Book Series: 5. Soil Science Society of America and American Society of Agronomy, Wisconsin, U.S.A.
- Sposito, G. 2008. The Chemistry of Soils. New York, U.S.A.: Oxford University Press.
- Środoń, J., and D. K. McCarty. 2008. “Surface Area and Layer Charge of Smectite from CEC and EGME/H2O-retention Measurements.” Clays Clay Miner 56 (2): 155–174. doi:10.1346/CCMN.2008.0560203.
- Tanaka, S., H. Saito, N. Kajiwara, T. N. Paing, K. H. M. Yusoff, S. Abe, A. Nakao, and J. Yanai. 2020. “Long-term Changes in Paddy Soil Fertility in Peninsular Malaysia during 50 Years after the Green Revolution with Special Reference to Their Physiographic Environments.” Soil Science and Plant Nutrition,67: 80-88.
- Tran, T. U., and K. Kajisa. 2006. “The Impact of Green Revolution on Rice Production in Vietnam.” The Developing Economies 44 (2): 167–189. doi:10.1111/j.1746-1049.2006.00012.x.
- Umetso, C., T. Lekprichakul, and U. Chakravorty. 2003. “Efficiency and Technical Change in the Philippine Rice Sector: A Malmquist Total Factor Productivity Analysis.” American Journal of Agricultural Economics 85 (4): 943–963. doi:10.1111/1467-8276.00499.
- 2010. “The Updated Philippine National Action Plan to Combat Desertification, Land Degradation and Drought (DLDD) Department of Agriculture.”.
- Yanai, J., M. Hirose, S. Tanaka, K. Sakamoto, A. Nakao, K. Dejbhimon, A. Sriprachote, P. Kanyawongha, T. Lattirasuvan, and S. Abe. 2020. “Changes in Paddy Soil Fertility in Thailand Due to the Green Revolution during the Last 50 Years.” Soil Science and Plant Nutrition 66 (6): 889–899. in press September, 2020 doi:10.1080/00380768.2020.1814115.
- Yoshida, S., J. S. Ahn, and D. A. Forno. 1973. “Occurrence, Diagnosis, and Correction of Zinc Deficiency of Lowland Rice.” Soil Science and Plant Nutrition 19 (2): 83–93. doi:10.1080/00380768.1973.10432522.
- Yumul, G. P., C. B. Dimalanta, V. B. Maglambayarw, and E. J. Marquez. 2008. “Tectonic Setting of A Composite Terrane: A Review of the Philippine Island Arc System.” Geosciences Journal 12 (1): 7–17. doi:10.1007/s12303-008-0002-0.
- Zeigler, R. S., and S. Mohanty. 2010. “Support for International Agricultural Research: Current Status and Future Challenges.” New Biotechnology 27 (5): 565–572. doi:10.1016/j.nbt.2010.08.003.