ABSTRACT
The chitin-hydrolyzed product LMC60 contains low-molecular-weight chitin (LMwC) with an average molecular weight of approximately 3,000 and sodium chloride formed by neutralization process. LMwC and a soil amendment with LMC60 (SLMC60) showed suppressive effects on plant diseases caused by pathogenic microbes. The spectrum of plant diseases against which SLMC60 was effective is however distinct from that of chitin, implying the unique effects of SLMC60 on plants and soil microorganisms. As a part of the study to elucidate the cause of the spectrum difference between chitin and SLMC60, we report here not only the degradation profile but also the antifungal property and microbial community structure in LMC60-added soil, in comparison with those in chitin-added soil and soil without supplement. Both LMC60-added and chitin-added soils inhibited the growth of a pathogenic Fusarium oxysporum strain, compared with the soil without supplement. The declining trend in the amount of LMC60 and chitin, and the patterns of ammonium accumulation, indicated equivalent degradation profiles of LMC60 and chitin at 25°C. The number of culturable bacteria increased in soil with LMC60 and chitin, but the number of culturable fungi and content of ergosterol, which is specific to fungal cells, increased only in the soil with LMC60. The effects of LMC60 and chitin on bacterial and fungal community structures were clearly distinguishable, as follows: The relative abundance of three bacterial families (Bacillaceae, Paenibacillaceae, and Alcaligenaceae) increased significantly only with LMC60. LMC60 increased the relative abundance of the family Promicrosporaceae earlier than chitin. In terms of fungi, Fusarium increased in the presence of LMC60 and chitin, the Mortierella population expanded considerably only with chitin, and Colletotrichum and Malbranchea increased only with LMC60. These effects of LMC60 on soil microorganisms which are distinct from those of chitin might suggest its spectrum of plant disease suppression.
1. Introduction
Chitin, a polymer of N-acetyl-D-glucosamine (GlcNAc), is found in the shells of crabs and shrimps as well as in the cell walls of fungi and cuticles of insects. The amendment of soil with chitin has been shown to suppress plant-pathogenic fungi (Mitchell and Alexander Citation1962; Buxton, Khalifa, and Ward Citation1965; Khalifa Citation1965; Henis, Sneh, and Katan Citation1967; Sneh and Henis Citation1972; Van Eck Citation1978; Murakami et al. Citation2009) and nematodes (Mian et al. Citation1982; Sarathchandra et al. Citation1996). It is presumed that chitinolytic microorganisms, which are capable of hydrolyzing the chitinous hyphae of pathogenic fungi, increased their numbers and/or activities in response to the chitin added (Cretoiu et al. Citation2013). Alternatively, secondary responders to the added chitin confer pathogen suppression (Cretoiu et al. Citation2013). Chitin-oligosaccharides, which might be produced during the degradation of chitin, are known elicitors that initiate a plant-innate immune response when in contact with the surface of the plant (Shibuya and Minami Citation2001). Chitin thus affects both soil microorganisms and plants, and supposedly leads to the suppression and mitigation of plant diseases.
LMC60, which has been developed by Yaizu Suisankagaku Industry (Shizuoka, Japan), contains about 60% (w/w) of low-molecular-weight chitin (LMwC) with an average molecular weight of about 3,000 and sodium chloride, which is formed during the neutralization process after acid hydrolysis of chitin. Foliar application of LMwC showed suppressive effect on bacterial spot of tomato (Kadota et al. Citation2010). A soil amendment with LMC60 (SLMC60), which consists of LMC60 and dextrin [approximately 60% (w/w)], suppressed cabbage yellow disease (Kadota et al. Citation2004; Kadota and Nagasaka Citation2008). The suppressive effects of SLMC60 on yellows and clubroot of cabbage were enhanced by combination with nonpathogenic Fusarium (Kadota and Nagasaka Citation2008). Since LMwC showed disease suppressive effect, it is supposed that the disease suppressive effect of SLMC60 is caused by LMwC in LMC60 (Kadota et al. Citation2010). Interestingly, the suppressive effect on cabbage yellow disease caused by Fusarium oxysporum f. sp. conglutinans was observed when SLMC60, but not chitin nor chitin-oligosaccharides, was used (Kadota et al. Citation2004). The result implies SLMC60 exhibits unique effects on the plant, the pathogen, and/or soil microorganisms. However, the functional mechanism of SLMC60 is unclear. The elucidation of the functional mechanism of SLMC60 would help in developing novel soil amendments.
In this report, as a part of the study to elucidate the functional mechanism of the unique disease suppressive effect of SLMC60 which contains LMC60 and dextrin, we focused on LMC60 because of its easy availability in the manufacturing process. We first evaluated the growth inhibition effect of soil treated with LMC60 against a pathogenic F. oxysporum strain to evaluate the disease suppressive effect and then investigated the degradation profile of LMC60 in an upland soil using an incubation study. We then assessed the number of culturable bacteria and fungi, the amount of ergosterol which is specifically present in fungal cells, and the bacterial and fungal community structures in LMC60 or chitin-supplemented upland soil, in order to evaluate the relative effects of LMC60 and chitin on soil microorganisms.
2. Materials and methods
2.1. Preparation of soil samples
The surface soil of Brown Forest soil (Light Clay) in an upland field at the Center for Education and Research, Field Sciences (lat. 34.905502 north; long. 138.272157 east), Faculty of Agriculture, Shizuoka University, was sampled in 2013 (Kumeta et al. Citation2018). After air-drying at room temperature, the soil sample was sieved through 2 mm meshes. The physicochemical properties of the dried soil are shown in . Chitin powder was purchased from FUJIFILM Wako Chemical. LMC60 was supplied from Yaizu Suisankagaku Industry. To 157 g of the air-dried sieved soil in a box (H 6 cm x W 12 cm x L 17 cm), 10 g of sterilized chitin or LMC60 powder [final ca. 60 g kg−1] was added and mixed. We selected 60 g kg−1 chitin as the optimal amount based on the quantitative range of the method used to measure the amount of chitin added to soil using thermogravimetry (Kumeta et al. Citation2018), although this amount is practically too much for use in plant cultivation. Water content was kept at 60% of maximum water holding capacity. The box was covered with a lid with pine halls and incubated at 25°C. For analyses, soil was taken from 10 distinctive points in the box, mixed, and stored in plastic tubes at −80°C.
Table 1. Selected physicochemical properties of the air-dried Brown Forest soil examined in this study and also used in our previous report (Kumeta et al. Citation2018)
2.2. Evaluation of the ability of soil to inhibit the growth of Fusarium oxysporum
Growth inhibition activity of soil against a plant pathogen F. oxysporum was assayed by co-cultivating indigenous soil microorganisms and a pathogenic fungus (Mitsuboshi et al. Citation2016) with some modifications. A piece of colony (about 5 mm × 5 mm) of F. oxysporum f. sp. lycopersici race 1 (Tomita et al. Citation2013) on a potato dextrose agar plate was placed in the center of YPMG agar medium (peptone-yeast extract medium; yeast extract 3 g, peptone 5 g, beef extract 3 g, glucose 10 g, agar 15 g, and distilled water 1 L; pH 7.0) (Anonymous Citation1975) mixed with a dilution series of a soil suspension (10−1 to 10−6). After an approximately one-week cultivation, the size of the colonies formed on the plates were expressed as area occupancies, which were calculated as the ratio of areas of the F. oxysporum colony to that of the Petri dishes (9 cm-diameter). The experiment was done in triplicate for each of soil samples prepared in duplicate.
2.3. Thermogravimetric measurement of LMC60 and chitin contents
To prepare the standard curves for determining chitin and LMC60 contents in soil, a portion of the soil whose water content was adjusted to 60% of maximum water holding capacity was mixed with chitin powder or LMC60 powder with a desired content (0–108 g kg−1), placed into a microtube, and dried at 105°C overnight. After the dried soil was ground to a powder in a mortar, approximately 70 mg of each of the ground soil samples was loaded into an aluminum pan (5.0 mm diameter × 2.5 mm depth) and vaporized (heating rate = 5°C min−1, from room temperature to 500°C) in a nitrogen atmosphere with a flow rate of 200 mL min−1 by using a thermogravimetry system (TG-DTA2020S/MS, Brucker) whose balance capacity, measurement range, and sensitivity were 1.0 g, ± 200 mg, and ± 0.1 mg, respectively. Rates of decline in soil weight (RDSW) (mg min−1) were calculated, based on the thermogravimetric data. Measurements were repeated three times and the average and standard deviation were calculated. For the chitin measurement, local maximum RDSW values around 385°C were used to prepare the standard curve of chitin content, as reported previously (Kumeta et al. Citation2018). In the case of LMC60, the changes in weight from 200 to 370°C were plotted to establish the standard curve.
A portion of the soil incubated with or without chitin or LMC60 was placed into a microtube and dried at 105°C overnight. The dried soil was ground in a mortar, loaded into an aluminum pan and vaporized as described above. Chitin and LMC60 contents were determined based on the local maximum RDSW around 385°C and the changes in weight from 200 to 370°C, respectively.
2.4. Measurement of (GlcNAc)n (n = 1-5) in LMC60
The contents of N-acetylglucosamine (GlcNAc), N,N’-diacetylchitobiose [(GlcNAc)2], N,N’,N”-triacetylchitotriose [(GlcNAc)3], N,N’,N”, N”’-tetraacetylchitotetraose [(GlcNAc)4], and N,N’,N”, N”’, N”“-pentaacetylchitopentaose [(GlcNAc)5] in LMC60 were measured by high-performance liquid chromatography with UV detection at 215 nm (SPD-20A, Shimadzu) and a normal phase column 4.6 mm × 250 mm (Inertsil NH2 3 μm, GL Scienc), as reported previously (Iinuma et al. Citation2018). (GlcNAc)n (n = 1–5) were separated under isocratic conditions {acetonitrile/water = 65/35 (v v−1)} at a flow rate of 1.0 mL min−1according to. GlcNAc and (GlcNAc)n (n = 2–5), which were purchased from FUJIFILM Wako Pure Chemical and SEIKAGAKU, respectively, were used as standards. (GlcNAc)n (n = 1–5) were identified and measured based on their corresponding retention times and peak areas.
2.5. Measurement of ammonium content
To measure ammonium nitrogen (NH4+-N), 1 g of each soil sample was suspended in 10 mL of 2 mol L−1 potassium chloride (KCl), shaken at 250 rpm for 1 h, and centrifuged at 3,000 rpm for 1 min. The supernatants were subjected to assays. NH4+-N content was measured by the indophenol method.
2.6. Chitinase and N-acetylglucosaminidase assay
Two grams of each soil sample were suspended in 10 mL of 0.1 mol L−1 potassium phosphate buffer (pH 7.0), shaken at 250 rpm for 30 min, and centrifuged at 3,500 rpm for 10 min. The supernatant was passed through one sheet of filter paper and subjected to enzyme assays to measure chitinolytic activities. Chitinase activity was measured by using 4-methylumbelliferyl-N,N',N''-triacetylchitotorioside [4MU-(GlcNAc)3] (Sigma-Aldrich) according to a previously described method (Miyashita, Fujii, and Sawada Citation1991). N-acetylglucosaminidase activity was evaluated using 4-methylumbelliferyl-N-acetyl-β-D-glucosamine (4MU-GlcNAc) (Sigma-Aldrich) as the substrate, as reported previously (Saito et al. Citation2013). One unit of chitinase or N-acetylglucosaminidase activity was defined as the amount of enzyme that liberated 1 × 10−6 mol of 4-metylumbelliferone from the corresponding substrate in 1 min at 37°C.
2.7. Counting the number of culturable microorganisms
To estimate the number of culturable microorganisms in soil, 1 g of soil sample was suspended in sterilized distilled water and shaken for 10 min. The suspension supernatant was diluted with sterilized distilled water and spread on agarized media: rose-bengal streptomycin agar medium [0.1% weight/volume (w/v) potassium dihydrogen phosphate (KH2PO4), 0.025% (w/v) magnesium sulfate (MgSO4), 0.5% (w/v) tryptone peptone (BD), 1.0% (w/v) glucose, 0.0033% (w/v) rose-bengal, 30 ppm streptomycin (FUJIFILM Wako Pure Chemical), and 1.5% (w/v) agar (pH 6.8)] for fungal count, and YG (yeast extract-glucose) agar medium [0.1% (w/v) glucose, 0.1% (w/v) yeast extract (BD), 0.03% (w/v) dipotassium hydrogen phosphate (K2HPO4), 0.02% (w/v) KH2PO4, 0.01% (w/v) MgSO4, and 1.5% (w/v) agar (pH 6.8)] for bacterial count. Following incubation at 25°C for 3 days on rose-bengal streptomycin medium and for 7 days on YG medium, colony numbers were counted. The Mann–Whitney U test was used to evaluate significant differences (P < 0.01) in the colony forming unit (CFU) of bacteria and fungi.
2.8. Measurement of ergosterol content in soil
Fungal biomass was evaluated by measuring ergosterol content in soil. One gram of soil sample was added into a 15-mL plastic tube with 0.5 g of each of two types of glass beads (AS ONE) whose diameters were around 200 and 800 μm, respectively. Following the addition of 1 mL of methanol, the plastic tube was mixed on a shaker for 1 h at 250 rpm and then allowed to stand for 15 min. The supernatant was transferred to a new 1.5-mL plastic tube, and centrifuged at 5°C for 10 min at 9,000 × g. The supernatant was filtered by a membrane with a 0.2 μm pore size and subjected to high-performance liquid chromatography. Ergosterol was separated through a reverse phase column (C18, 4.6 mm × 250 mm, 5 μm) and detected by absorbance at 282 nm. Ergosterol, which was purchased from FUJIFILM Wako Pure Chemical, served as the standard. Ergosterol was detected and quantified based on the corresponding retention time and peak area. The Mann–Whitney U test was used to evaluate significant differences (P < 0.01) in the ergosterol content in soil.
2.9. Analysis of microbiomes
DNA was extracted from 0.4 g of each soil sample using a FastDNA SPIN Kit for Soil (MP Biomedicals), according to a previously reported method (Takada-Hoshino and Matsumoto Citation2004). Extracted DNA was used for PCR, which was performed to amplify the V4 region of 16S rRNA genes for analyzing bacterial community structure and the fungal ITS2 region, with ExTaq DNA polymerase (Takara Bio). To amplify the V4 region, the primers 515F (5ʹ-GTGCCAGCMGCCGCGGTAA-3ʹ) and 806R (5ʹ-GGACTACHVGGGTWTCTAAT-3ʹ), which contain sequencer adopter regions (Caporaso et al. Citation2011), were used, whereas the primers gITS7 (5ʹ-GTGARTCATCGARTCTTTG-3ʹ) (Ihrmark et al. Citation2012) and ITS4 (5ʹ-TCCTCCGCTTATTGATATGC-3ʹ) were used for the fungal ITS2 region. PCR products, whose specific amplification was checked by agarose gel electrophoresis, were purified using AMpure XP (Beckman Coulter). PCR fragments were subjected to paired-end sequencing using an Illumina MiSeq platform (Illumina, San Diego, CA, USA) at Fasmac (Atsugi, Japan) or the Bioengineering Lab. (Sagamihara, Japan). Sequences were quality filtered and analyzed with the Quantitative Insights Into Microbial Ecology 2 (QIIME2) pipeline (version 2018.8 [Bolyen et al. Citation2019]). The remaining sequences (see Tables S1 and S2for the detailed number of sequences) were clustered into OTUs at the 97% level and classified using the Greengenes 13–8 reference database (McDonald et al. Citation2012), as implemented in QIIME2. Close relatives of representative OTUs were identified by BLASTn analysis and a phylogenetic analysis using the SILVA database (SILVA SSU Ref NR_128 database) and backbone tree (tree_SSURefNR99_1200slv_128) in the ARB program for sequence analysis (Ludwig et al. Citation2004). The diversity indexes H’ and D for bacteria and fungi were calculated based on the relative abundance of OTUs whose values exceeded 1%. The Mann–Whitney U test was used to evaluate significant differences (P < 0.01) in the abundance of bacteria and fungi at the level of family and genus, and also in the values of H’ and D indexes. Illumina sequencing data were deposited in the DDBJ/ENA/GenBank database under BioSample IDs SAMD00257607-00257625, SAMD00257628-00257629, and SAMD00257632-SAMD00257645.
3. Results
3.1. Evaluation of inhibitory effects of chitin- or LMC60-added soil on the growth of Fusarium oxysporum
To evaluate the disease suppressive ability of soils supplemented with chitin or LMC60, we investigated the growth inhibition of the soils on a pathogenic F. oxysporum strain. As already reported by Mitsuboshi et al. (Citation2016), almost no growth of F. oxysporum colonies was observed on plates with dilutions of soil from 10−1 to 10−3 (). The incubation for 0–45 d did not significantly affect the growth inhibitory effect of the soil without supplementation (, left). When the soils were not incubated (at 0 d), neither the chitin- nor LMC60-supplementation affected the inhibitory effect of the soil (, left). However, the soils incubated for 3 d with LMC60 and chitin indicated enhanced growth-inhibitory effect on plates with dilutions from 10−4 to 10−6 (, middle). These results suggested that neither chitin nor LMC60 directly inhibited the growth of this fungus. The growth-inhibitory effect of the LMC60-supplemented soil seemed to be still slightly enhanced in the soil sample incubated for 11 d, but not in chitin-supplemented soil (, right). In the soil samples incubated for 45 and 60 d the growth-inhibitory effects were comparable with each other regardless the addition of LMC60 and chitin (, dark-gray and black symbols).
Figure 1. Growth of Fusarium oxysporum f. sp. lycopersici race 1 on an agar medium inoculated with each of the incubated upland soil samples. Area occupancies indicate the rates of sizes of colonies to that of the F. oxyssporum strain on the agar medium without soil suspension. (A) Effect of inoculation of upland soil incubated with LMC60 (middle) or chitin (right) or without supplement (left) for 0 d (white symbols), 3 d (striped symbols), 11 d (light-gray symbols), 45 d (dark-gray symbols) and 60 d (black symbols) at 25°C. (B) Effect of inoculation of upland soil incubated with LMC60 (triangles) or chitin (squares) or without supplement (circles) for 0 d (left), 3 d (middle), and 11 d (right) at 25°C. The panels A and B show the same data rearranged. Averages of triplicate measurements for the duplicate samples are plotted. Error bars indicate the maximum and minimum contents of the duplicated soil samples
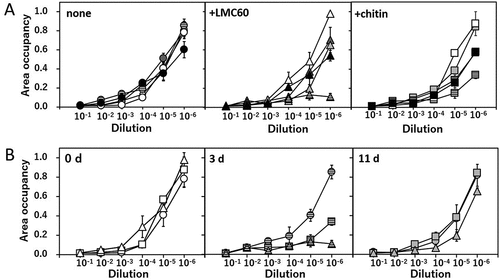
3.2. Analysis of LMC60
We previously reported the application of thermogravimetory (TG) for measuring chitin content in soil (Kumeta et al. Citation2018). To examine whether TG can also be applied for the determination of LMC60 content in soil, we investigated the thermogravimetric profile of LMC60 and compared it with that of chitin powder. Unlike the pyrolysis of chitin which took place at around 385°C, the weight of LMC60 declined from 200 to 370°C (). The higher pyrolysis temperature of chitin suggests that LMC60 does not contain highly polymerized chitin chains which can be found in powdered chitin. The broad pyrolysis temperature range of LMC60 implies that LMC60 contains chitin chains with varying chain length. As the broad pyrolysis temperature of LMC60 (200–370°C) suggest the presence of GlcNAc and (GlcNAc)2, whose pyrolysis temperatures are 200 and 240°C (Kumeta et al. Citation2018), the contents of GlcNAc, (GlcNAc)2, and higher oligomers of LMC60 were measured by HPLC. The result indicated that LMC60 contains about 0.5% (w/w) each of (GlcNAc)n (n = 1–5) ().
Table 2. Contents of (GlcNAc)n (n = 1–5) in LMC60
Figure 2. (A) Thermogravimetric profile of an upland soil supplemented with chitin or LMC60. Alteration of relative weight (%) (normal lines) and rates of decline in weight (dashed line) are plotted. The content of each supplement added to soil was set to 60 g kg−1. (B) Alteration of relative weight (normal lines) and rate of decline in weight (dashed lines) with or without LMC60. The content of LMC60 added to soil (g kg−1) is also indicated. The two straight lines indicate 200 and 370°C. (C) Relationship between the change in weight from 200–370°C and LMC60 content. The averages of triplicate measurements are plotted. Error bars indicate standard deviations. The result of linear approximation is shown by a formula with its determination coefficient
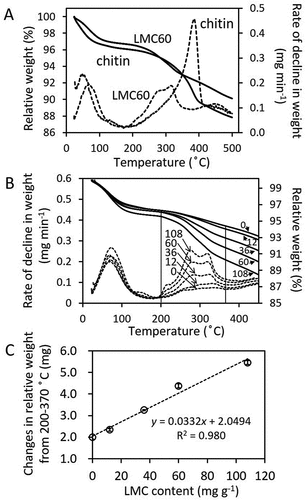
Based on the thermogravimetric profile of soil with LMC60 (), we used the ratio of the weights (%) between the declining weights from 200 to 370°C and the initial weight, in order to prepare a standard curve to measure the amount of LMC60 in soil. The declining weight values and the amount of LMC60 added to soil were strongly and positively correlated (R2 = 0.980) (), even more so in the case of chitin (R2 = 0.996) (Kumeta et al. Citation2018).
3.3. Degradation profiles of chitin and LMC60
The degradation of LMC60 was investigated in an incubated upland soil. The pattern of reduction in the amount of LMC was comparable with that of chitin (). The accumulation of ammonia/ammonium, which would be generated by LMC60 degradation, in the LMC60-treated soil was similar to or slightly slower than that in the chitin-treated soil (). These data demonstrate that the degradation profile of LMC60 in the incubated upland soil was similar to that of chitin.
Figure 3. Degradation of chitin and LMC60 in an upland soil. (A) Amount of LMC60 and chitin in incubated upland soil. (B) Amount of ammonium in incubated upland soil supplemented with chitin or LMC60. (C) Activities of chitinolytic enzymes. Circles, control soil (without LMC60 or chitin); triangles, soil supplemented with LMC60; squares, soil supplemented with chitin. In panel C, chitinase activity evaluated with 4MU-(GlcNAc)3 is indicated by open symbols while N-acetylglucosaminidase activity assayed with 4MU-GlcNAc is shown by closed symbols. In panel A, the results of exponential approximation are shown by formulas with the determination coefficients. Soil samples were prepared in duplicate and the average contents are shown. Error bars indicate the maximum and minimum contents of the duplicated soil samples
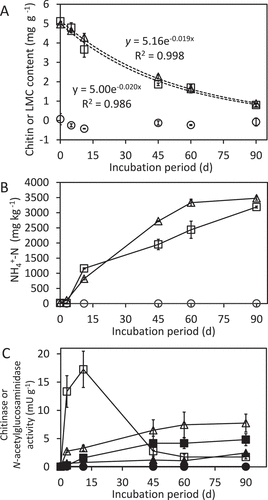
3.4. Chitinase and N-acetylglcosminidase activity
In the presence of chitin, chitinase activity seemed to be transient because activity increased until 11 d, then decreased (), as was previously observed in upland soil to which chitin had been added (Kumeta et al. Citation2018). In contrast, chitinase activity gradually increased until 60 d in the LMC60-supplemented upland soil, although maximum activity was about half of that of chitin-supplemented soil. The increasing patterns of N-acetylglucosaminidase (GlcNAcase) activity in the presence of chitin or LMC60 were similar to that of chitinase activity in the LMC60-supplemented soil. GlcNAcase activity was lower in LMC60-supplemented soil than in chitin-supplemented soil.
3.5. Effects of chitin and LMC60 on the population of microorganisms
To investigate the effects of LMC60 on soil microorganisms, we first counted the number of culturable soil bacteria and fungi on agar media. Both LMC60 and chitin increased the number of culturable bacteria 10–20 times more than soil without any supplementation (). The increase in the presence of LMC60 was slightly earlier than that with chitin. Chitin did not increase the number of culturable fungi whereas LMC60 significantly did (P < 0.01) (). To confirm the increase in fungi in the presence of LMC60, ergosterol content was measured. Ergosterol content increased in the presence of LMC60 but did not change in soil with or without chitin (). The profile of the increase in ergosterol content induced by LMC60 appeared to coincide well with the number of culturable fungi. We thus conclude that the addition of LMC60 increased fungal biomass in the incubated upland soil. The decrease in ergosterol content observed at 60 and 90 days was milder than that of fungal count (). Ergosterol might have persisted in the dead hyphae of fungi in soil, but its levels may have decreased slowly following biotic and abiotic degradation.
Figure 4. Number of culturable bacteria (A), fungi (B), and ergosterol content (C) in incubated upland soil without supplements (white bars), with LMC60 (gray bars), and with chitin (black bars). Soil samples were prepared in duplicate and the number of culturable microbes in both soil samples are indicated. Averages of triplicate counts are plotted. Error bars indicate standard deviations
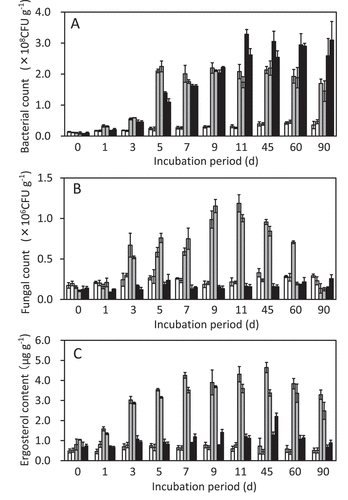
3.6. Effects of LMC60 on bacterial community structure
The relative abundance of Strepmycetaceae and Xanthomonadaceae families increased considerably in the presence of either chitin or LMC60 (, orange and green parts, respectively). Streptomcetaceae includes the genus Streptomyces, which is a well-known chitin-degrader in soil environments, increased earlier than Xanthomonadaceae. The family Promicromonosporaceae, including the genus Cellulosimicrobium, also increased in the LMC60- and chitin-supplemented soils (, red parts). The timing of the increases in the LMC60- and chitin-supplemented soils were different, however. In the chitin-supplemented soil, the proportion of Promicromonosporaceae increased to 7% and 17% at 60 d, whereas Promicromonosporaceae increased to 6% and 10% even by 3 d and the population was maintained until 60 d (9%) in the LMC60-supplemented soil. A significant increase of Alcaligenaceae was also observed in the LMC60- and chitin-supplemented soils, although the amount of increase was much larger in the LMC60-supplemented soil than in the chitin-supplemented soil (, purple parts). A significant increase of the Bacillaceae family was only observed in the presence of LMC60 (, blue parts). The relative ratio of Bacillaceae was 18 or 19, 2 or 6, and 2 or 5% at 3, 11, and 60 d, respectively, in the presence of LMC60, whereas the ratio was less than 1% in the presence of chitin. Paenibacillaceae appeared to increase only in LMC60-supplemented soil but the change was not statistically significant. The effects of LMC60 on soil bacteria were thus distinguishable from those of chitin, especially on the Bacillaceae, Promicromonosporaceae, and Alcaligenaceae families, although the effects on Streptomyhcetaceae and Xanthomonadaceae families were similar.
Figure 5. Abundance ratio of bacterial families in upland soil incubated with LMC60 or chitin. Classes accounting for less than 0.01 (1%) were classified as ‘other bacteria.’ Red, Promicromonosporaceae; orange, Streptomycetaceae; blue, Bacillaceae; sky blue, Paenibacillaceae; purple, Alcaligenaceae; green, Xanthomonadaceae
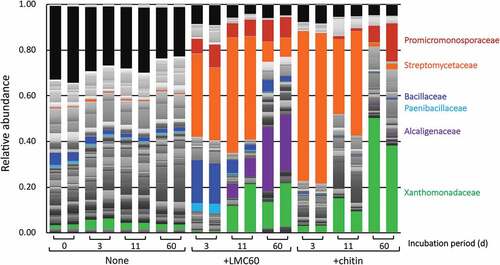
The H’ and D indexes of the LMC60- and chitin-supplemented soils were significantly lower than those of soil without supplements (P < 0.01) (), indicating that bacterial diversity was reduced following the addition of LMC60 and chitin. The indexes also suggested that diversity was reduced more rapidly in soil to which chitin, rather than LMC60, was added.
Table 3. Diversity indexes (H’ and D) in LMC60- and chitin-supplemented soils
3.7. Effects of LMC60 on fungal community structure
Amplicon sequencing that targeted the ITS region revealed that in the presence of chitin, the genus Mortierella, which increases in chitin-amended soil and exhibits chitin-degrading activity (Okafor Citation1966; De Boer et al. Citation1999), increased drastically by day 3 (, sky blue parts). In contrast, the addition of LMC60 significantly decreased the abundance of Mortierella. The genera Colletotrichum and Malbranchea increased significantly only in LMC60-supplemented soil whereas chitin did not affect the relative abundance of these genera (, green and purple parts, respectively). The ratio of Fusarium increased significantly in the LMC60-supplemented soil (, red parts) at 3, 11, and 60 d, whereas in the chitin-supplemented soil, a significant increase in Fusarium was observed at 11 and 60 d, but not at 3 d. The fungal genera Fusarium, Collectotrichum and Malbranchea, whose relative abundance increased in the LMC60-supplemented soil, are all Ascomycota. We conclude that the addition of LMC60 increased the fungal population, especially Ascomycota.
Figure 6. Fungal community structure in upland soil incubated with LMC60 or chitin. Classes accounting for less than 0.01 (1%) were classified as ‘other fungi.’ Sky blue, Mortierella; red, Fusarium; green, Colletotrichum; purple, Malbranchea.
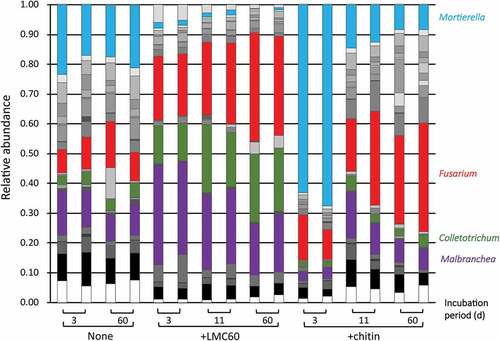
The H’ and D indexes of the LMC60-supplemented soils were significantly lower than those of soil without supplements (P < 0.01) (), indicating that fungal diversity was reduced after the addition of LMC60. Chitin drastically affected those indexes at 3 d, lowering fungal diversity, although not significantly at 11 and 60 d. The effects of LMC60 and chitin on these indexes suggest that fungal diversity was consistently lowered by LMC, and tentatively even more so by chitin.
4. Discussion
LMC60 contains approximately 10% (w/w) sodium chloride in addition to LMwC of an average molecular weight of 3,000, which corresponds to a 14–15 degree of polymerization of GlcNAc. In this study, we compared the effects on microorganisms of LMC60 and chitin in an incubated upland soil, in order to elucidate the microbiological backgrounds of the disease-suppressive effect of the soil amendment with LMC60 (SLMC60) (Kadota et al. Citation2004, Citation2010; Kadota and Nagasaka Citation2008), whose spectrum seems similar to, but distinguishable from, that of chitin (Kadota et al. Citation2004, Citation2010; Kadota and Nagasaka Citation2008). SLMC60 contained approximately 60% (w/w) dextrin other than LMC60. Although we in this study demonstrated the distinctive effects of LMC60 on soil microorganisms, the effects of dextrin, which remains to be elucidated, should be considered, to discuss the spectra of disease-suppressive effects of SLMC60 and chitin. However, it is valuable to evaluate the effects of LMC60 on soil microorganisms because the soil supplemented with LMC60 inhibited the growth of a pathogenic F. oxysporum strain more strongly than the soil without supplement did (). In addition to LMwC, LMC60 contains approximately 10% (w/w) sodium chloride, which would also affect activity of soil microorganisms. We state here again that we evaluated the net effects of LMC60 (LMwC and sodium chloride) on soil microorganisms. We are now obtaining desalted LMC60 which contains only LMwC in order to evaluate the degradation and effects on soil microorganism of LMwC.
In this study, we indicated that LMC60 contains 0.5% (w/w) of each (GlcNAc)n (n = 1–5) (), and that pyrolysis of LMC60 was observed at 200–370°C (). Considering that the pyrolysis temperatures of GlcNAc, (GlcNAc)2, and chitin were about 200, 240, and 385°C, respectively (Kumeta et al. Citation2018), the broad pyrolysis temperature range of LMC60 is reasonable and suggests the presence of chitin chains of varying chain length.
The degradation profiles of LMC60 and chitin were comparable in the incubated upland soil (). The shapes of broad pyrolysis temperature range of LMC60 () did not seem to significantly alter during the degradation process, although the size of the broad peak of the pyrolysis was getting smaller along the degradation (data not shown). This suggests that the components of LMC60 were evenly degraded regardless the chain length of chitin. On the other hand, peaks corresponding to LMwC was not detected during the degradation process of chitin in soil not only in this study (data not shown) but also in our previous report (Kumeta et al. Citation2018). These implied that LMwC which should be generated during chitin-degradation in soil was rapidly degraded further and consumed by microorganisms. LMC60 continuously lowered bacterial and fungal diversity but more mildly than chitin (). Chitin impacted microorganisms at 3 d more strongly than LMC60, but diversity seemed to recover earlier in chitin-supplemented soil than in LMC60-supplemented soil (, ). These milder and more continuous effects of LMC60 than chitin on soil microorganisms may be attributed to the presence of chitin chains of varying chain length in LMC60. LMC60 and chitin showed many different effects on soil microorganisms as above, but in total, the degradation profiles of LMC60 and chitin were similar. It is unclear why LMC60 and chitin indicated similar degradation profile in the incubated soil, in spite that profiles of chitinolytic enzyme activity and microbial community structure were distinctive from each other. Physicochemical factors such as pH and EC, which were not measured in this study, might have been altered differently in the LMC60 and chitin-supplemented soils, and might have affected microbial activity and community structure. Sodium chloride in LMC60 might have affected. Further series of experiment have to be conducted to elucidate the reason for the similar degradation profile and distinctive effects on chitinolytic enzyme activity and microbial community structure of LMC60 and chitin.
We previously reported that the addition of chitin to soil immediately increased the population of bacteria, genus Streptomyces, which is the main decomposer of chitin in soil environments (Iwasaki, Ichino, and Saito Citation2020). As the population of Streptomyces decreased over time, the populations of Lysobacter, Pseudoxanthomonas, Cellulosimicrobium, Streptosporandium, and Nonomuraea increased (Iwasaki, Ichino, and Saito Citation2020). We obtained a similar result in this study: As Streptomycetaceae decreased, the Xanthomonadaceae and Promicromonosporaceae families, to which Lysobacter and Pseudoxanthomonas, and Celllulosimicrobium, respectively, belong, increased in the chitin-supplemented soil (). Interestingly, in the LMC60-supplemented soil, an increase of Promicromonosporaceae was observed even at 3 d, in contrast to the chitin-supplemented soil. This is not easily attributed to LMwC in LMC60 because LMC60 also contains sodium chloride (see above). However, the specific increase of Bacillaceae in LMC60-supplemented soil could at least partially be attributed to the presence of 0.5% (w/w) GlcNAc in LMC60 (), because GlcNAc supplementation has been reported to significantly increase Bacillaceae (Hui et al. Citation2020; Shimoi et al. Citation2020).
In the chitin-supplemented soil, Mortierella increased considerably at 3 d (). Mortierella has been reported to be consistently enriched in biosolarized soils with chitin amendment (Randall et al. Citation2020), and is the dominant soil-suppressive agent against vanilla Fusarium wilt disease and might serve as an indicator and enhancer of Fusarium wilt disease suppression in vanilla (Xiong et al. Citation2017). In contrast, the relative abundance of Mortierella decreased in response to LMC60 (). LMC60 significantly increased the fungal population, especially Colletotrichum and Malbranchea, in the incubated soil ( and ). This effect of LMC60 should be due to one or both of the components of LMC60: LMwC and sodium chloride, but a definitive conclusion cannot be made at this moment. In the LMC60-supplemented soil, the Ascomycota genera Malbranchea, Colletotrichum, and Fusarium increased (). Malbranchea species are thermophilic strains and there is little information on the plant pathogenicity of Malbranchea. In contrast, Colletotrichum species are known plant pathogens that cause destructive diseases in a wide variety of fruit crops (Dowling et al. Citation2020; López-Moral et al. Citation2020 for review). In the case of Fusarium species, which are known plant pathogens, nonpathogenic Fusarium has been reported to suppress Fusarium wilt diseases via saprophytic competition, induction of systematic resistance in the plant host, and parasitic competition (Mazzola Citation2002 for review). The application of a nonpathogenic Fusarium strain with SLMC60 significantly enhanced the disease-suppressive effect against cabbage yellow disease and cabbage clubroot disease which are caused by F. oxysporum f. sp. conglutinans and Plasmodiophora brassicae, respectively (Kadota and Nagasaka Citation2008). LMwC in SLMC60 might be a good nutrient for the supplemented and indigenous populations of Fusarium, and the Fusarium population might compete for nutrients with the pathogen F. oxysporum f. sp. conglutinans. Colletotrichum and Malbranchea, which might increase following the addition of SLMC60, thereby also might contribute to the suppression of cabbage yellow disease.
The similarities and differences of the microbiological backgrounds of LMC60- and chitin-supplemented soils, which have been revealed by this study, simply provide hints to elucidate the mechanisms by which SLMC60 and chitin suppress plant diseases, either similarly, or differently. We are now planning to investigate the chemical characteristics of LMwC, which is the main component of LMC60, and its effects on soil organisms.
Acknowledgments
The authors appreciate assistance by Mai Shizukuda and Tomoyasu Nishizawa at Ibaraki University with ergosterol measurements, Masahiro Higuchi at Yaizu Suisankagaku Industry for providing LMC60, and Yusuke Wakikawa and Kazuo Hayakawa at Advanced Instrumental Analysis Center, SIST, for their support in thermogravimetric analysis.
Disclosure statement
This study was initiated after Yaizu Suisankagaku Industry (YSK) introduced the disease suppression effect of SLMC60 to Akihiro Saito (AS). YSK provided AS with LMC60 and related information.
Additional information
Funding
References
- Anonymous. 1975. “Appendix, I. Composition of Medium and Preparation Method.”431–443 In Soil Microbiological Society of Japan (Ed.), Experimental Methods in Soil Microbiology. Yokendo, Tokyo: In Japanese
- Bolyen, E., J. R. Rideout, M. R. Dillon, N. A. Bokulich, C. C. Abnet, G. A. Al-Ghalith, H. Alexander, et al. 2019. “Reproducible, Interactive, Scalable and Extensible Microbiome Data Science Using QIIME 2.” Nature Biotechnology 37 (8): 852–857. doi:10.1038/s41587-019-0209-9.
- Buxton, E. W., O. Khalifa, and V. Ward. 1965. “Effect of Soil Amendment with Chitin on Pea Wilt Caused by Fusarium oxysporum f. pisi.” Annals of Applied Biology 55 (1): 83–88. doi:10.1111/j.1744-7348.1965.tb07870.x.
- Caporaso, J. G., C. L. Lauber, W. A. Walters, D. Berg-lyons, C. A. Lozupone, P. J. Turnbaugh, N. Fierer, and R. Knight. 2011. “Global Patterns of 16S rRNA Diversity at a Depth of Millions of Sequences per Sample.” Proceedings of the National Academy of Sciences of the United States of America. 108:4516–4522. doi:10.1073/pnas.1000080107.
- Cretoiu, M. S., G. W. Korthals, J. H. M. Visser, and J. D. van Elsas. 2013. “Chitin Amendment Increases Soil Suppressiveness toward Plant Pathogens and Modulates the Actinobacterial and Oxalobacteraceal Communities in an Experimental Agricultural Field.” Applied and Environmental Microbiology 79 (17): 5291–5301. doi:10.1128/AEM.01361-13.
- De Boer, W., S. Gerards, P. J. A. Klein Gunnewiek, and R. Modderman. 1999. “Response of the Chitinolytic Microbial Community to Chitin Amendments of Dune Soils.” Biology and Fertility of Soils 29: 170–177. doi:10.1007/s003740050541.
- Dowling, M., N. Peres, S. Villani, and G. Schnabel. 2020. “Managing Colletotrichum on Fruit Crops: A “Complex” Challenge.” Plant Disease 104 (9): 2301–2316. doi:10.1094/PDIS-11-19-2378-FE.
- Henis, Y., B. Sneh, and J. Katan. 1967. “Effect of Organic Amendments on Rhizoctonia and Accompanying Microflora in Soil.” Canadian Journal of Microbiology 13 (6): 643–649. doi:10.1139/m67-085.
- Hui, C., H. Jiang, B. Liu, R. Wei, Y. Zhang, Q. Zhang, Y. Liang, and Y. Zhao. 2020. “Chitin Degradation and the Temporary Response of Bacterial Chitinolytic Communities to Chitin Amendment in Soil under Different Fertilization Regimes.” Science of the Total Environment 705: 136003. doi:10.1016/j.scitotenv.2019.136003.
- Ihrmark, K., I. T. M. Bödeker, K. Cruz-Martinez, H. Friberg, A. Kubartova, J. Schenck, Y. Strid, et al. 2012. “New Primers to Amplify the Fungal ITS2 Region - Evaluation by 454-sequencing of Artificial and Natural Communities.” FEMS Microbiology Ecology 82 (3): 666–677. doi:10.1111/j.1574-6941.2012.01437.x.
- Iinuma, C., A. Saito, T. Ohnuma, E. Tenconi, A. Rosu, S. Colson, Y. Mizutani, et al. 2018. “NgcESco Acts as a Lower-Affinity Binding Protein of an ABC Transporter for the Uptake of N,N′-Diacetylchitobiose in Streptomyces coelicolor A3(2).” Microbes and Environments 33 (3): 272–281. doi:10.1264/jsme2.ME17172.
- Iwasaki, Y., T. Ichino, and A. Saito. 2020. “Transition of the Bacterial Community and Culturable Chitinolytic Bacteria in Chitin-treated Upland Soil: From Streptomyces to Methionine-auxotrophic Lysobacter and Other Genera.” Microbes and Environments 35 (1). doi:10.1264/jsme2.ME19070.
- Kadota, I., and A. Nagasaka. 2008. “Use of Host Resistance to Pathogens Induced by Chitin and Microbes for Plant Disease Control. (In Japanese).” Research Journal of Food and Agriculture 31: 20–23.
- Kadota, I., H. Okada, H., . T. Yoshida, Y. Matahira, and S. Toyoda 2004. “Plant Disease Controlling Agent and Plant Disease Controlling Method Using the Agent.” Japan Patent 2004-323460, November 18.
- Kadota, I., Y. Yoshida, Y. Matahira, and K. Hanada. 2010. “Control Effect of Bacterial Spot and Bacterial Canker of Tomato by Foliar Application of the Materials Including Low Molecular Chitin. (In Japanese)” Annual Report of the Society of Plant Protection of North Japan 61: 71–75.
- Khalifa, O. 1965. “Biological Control of Fusarium Wilt of Peas by Organic Soil Amendments.” Annals of Applied Biology 56 (1): 129–137. doi:10.1111/j.1744-7348.1965.tb01222.x.
- Kumeta, Y., K. Inami, K. Ishimaru, Y. Yamazaki, R. Sameshima-Saito, and A. Saito. 2018. “Thermogravimetric Evaluation of Chitin Degradation in Soil: Implication for the Enhancement of Ammonification of Native Organic Nitrogen by Chitin Addition.” Soil Science and Plant Nutrition 64 (4): 512–519. doi:10.1080/00380768.2018.1457408.
- López-Moral, A., C. Agustí-Brisach, M. Lovera, O. Arquero, and A. Trapero. 2020. “Almond Anthracnose: Current Knowledge and Future Perspectives.” Plants (Basel) 9 (8): 945. doi:10.3390/plants9080945.
- Ludwig, W., O. Strunk, R. Westram, L. Richter, H. Meier, B. A. Yadhukumar, T. Lai, S. Steppi, G. Jobb, and W. Föster. 2004. “ARB: A Software Environment for Sequence Data.” Nucleic Acids Research 32 (4): 1363–1371. doi:10.1093/nar/gkh293.
- Mazzola, M. 2002. “Mechanisms of Natural Soil Suppressiveness to Soilborne Diseases.” Antonie Van Leeuwenhoek 81 (1/4): 557–564. doi:10.1023/A:1020557523557.
- McDonald, D., M. N. Price, J. Goodrich, E. P. Nawrocki, T. Z. DeSantis, A. Probst, G. L. Andersen, R. Knight, and P. Hugenholtz. 2012. “An Improved Greengenes Taxonomy with Explicit Ranks for Ecological and Evolutionary Analyses of Bacteria and Archaea.” The ISME Journal 6 (3): 610–618. doi:10.1038/ismej.2011.139.
- Mian, J. H., G. Godoy, R. A. Shelby, R. Rodriguez-Kabana, and G. Morgan-Jones. 1982. “Chitin Amendments for Control of Meloidogyne arenaria in Infested Soil.” Nematropica 12: 71–84.
- Mitchell, R., and M. Alexander. 1962. “Microbiological Processes Associated with the Use of Chitin for Biological Control.” Soil Science Society of America Journal 26 (6): 556–558. doi:10.2136/sssaj1962.03615995002600060013x.
- Mitsuboshi, M., Y. Kioka, K. Noguchi, and S. Asakawa. 2016. “An Evaluation Method for the Suppression of Pathogenic Fusarium oxysporum by Soil Microorganisms Using the Dilution Plate Technique.” Microbes and Environments 31 (3): 307–313. doi:10.1264/jsme2.ME16052.
- Miyashita, K., T. Fujii, and Y. Sawada. 1991. “Molecular Cloning and Characterization of Chitinase Genes from Streptomyces lividans 66.” Journal of General Microbiology 137 (9): 2065–2072. doi:10.1099/00221287-137-9-2065.
- Murakami, H., Y. Kuroyanagi, S. Tsushima, and Y. Shishido. 2009. “Effects of Application of Organic Materials on the Development of Clubroot Disease Caused by Plasmodiophora brassicae (In Japanese).” Soil Microorganisms 63: 3–8.
- Okafor, N. 1966. “Estimation of the Decomposition of Chitin in Soil by the Method of Carbon Dioxide Release.” Soil Science 102 (2): 140–142. doi:10.1097/00010694-196608000-00010.
- Randall, T. E., J. D. Fernandez-Bayo, D. R. Harrold, Y. Achmon, K. V. Hestmark, T. R. Gordon, J. J. Stapleton, C. W. Simmons, J. S. VanderGheynst, and V. Gupta. 2020. “Changes of Fusarium oxysporum f. sp. Lactucae Levels and Soil Microbial Community during Soil Biosolarization Using Chitin as Soil Amendment.” PLoS ONE 15 (5): e0232662. doi:10.1371/journal.pone.0232662.
- Saito, A., H. Ebise, Y. Orihara, S. Murakami, Y. Sano, A. Kimura, Y. Sugiyama, A. Ando, T. Fujii, and K. Miyashita. 2013. “Enzymatic and Genetic Characterization of the DasD Protein Possessing N -acetyl-β- D -glucosaminidase Activity in Streptomyces coelicolor A3(2).” FEMS Microbiology Letters 340 (1): 33–40. doi:10.1111/1574-6968.12069.
- Sarathchandra, S. U., R. N. Watson, N. R. Cox, M. E. di Menna, J. A. Brown, G. Burch, and F. J. Neville. 1996. “Effects of Chitin Amendment of Soil on Microorganisms, Nematodes, and Growth of White Clover (Trifolium repens L.) And Perennial Ryegrass (Lolium perenne L.).” Biology and Fertility of Soils 22 (3): 221–226. doi:10.1007/BF00382516.
- Shibuya, N., and E. Minami. 2001. “Oligosaccharide Signalling for Defence Responses in Plant.” Physiological and Molecular Plant Pathology 59 (5): 223–233. doi:10.1006/pmpp.2001.0364.
- Shimoi, Y., D. Honma, A. Kurematsu, Y. Iwasaki, Y. Kotsuchibashi, Y. Wakikawa, and A. Saito. 2020. “Effects of Chitin Degradation Products N-acetylglucosamine and N,Nʹ -diacetylchitobiose on Chitinase Activity and Bacterial Community Structure in an Incubated Upland Soil.” Soil Science and Plant Nutrition 66 (3): 429–437. doi:10.1080/00380768.2020.1767488.
- Sneh, B., and Y. Henis. 1972. “Production of Antifungal Substances Active against Rhizoctonia solani in Chitin-amended Soil.” Phytopathology 62 (6): 595–600. doi:10.1094/Phyto-62-595.
- Takada-Hoshino, Y., and N. Matsumoto. 2004. “An Improved DNA Extraction Method Using Skim Milk from Soils that Strongly Adsorb DNA.” Microbes and Environments 19 (1): 13–19. doi:10.1264/jsme2.19.13.
- Tomita, M., A. Kikuchi, M. Kobayashi, M. Yamaguchi, S. Ifuku, S. Yamashoji, A. Ando, and A. Saito. 2013. “Characterization of Antifungal Activity of the GH-46 Subclass III Chitosanase from Bacillus circulans MH-K1.” Antonie Van Leeuwenhoek 104 (5): 737–748. doi:10.1007/s10482-013-9982-5.
- Van Eck, W. H. 1978. “Autolysis of Chlamydospores of Fusarium Solani F. Sp. Cucurbitae in Chitin and Laminarin Amended Soils.” Soil Biology and Biochemistry 10 (2): 89–92. doi:10.1016/0038-0717(78)90076-7.
- Xiong, R., R. Li, Y. Ren, C. Liu, Q. Zhao, H. Wu, A. Jousset, and Q. Shen. 2017. “Distinct Roles for Soil Fungal and Bacterial Communities Associated with the Suppression of Vanilla Fusarium Wilt Disease.” Soil Biology and Biochemistry 107: 198–207. doi:10.1016/j.soilbio.2017.01.010.