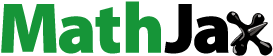
ABSTRACT
Application of bio-fertilizer with the ability to promote root development in legume plants could be beneficial, and it is vital to find an inoculation method that does not inhibit symbiosis between rhizobium and plants. However, co-inoculation of rhizobia with different microorganisms on legumes generally inhibits interdependency with rhizobia. The present study was conducted to determine the ideal inoculation method of the bio-fertilizer ‘Yume-bio’ containing Bacillus pumilus TUAT1, which has plant growth-promoting activity, without inhibiting rhizobial infection of soybean. Soybean plants were inoculated with Bradyrhizobium diazoefficiens USDA110 during the time of sowing seeds. Then, two types of ‘Yume-bio’ applications were conducted: simultaneous inoculation with the rhizobia (SI) and 1 week after inoculation the rhizobia (I). No inoculation (NI) was used as the control. Four weeks after sowing, biomass dry weight (shoot and root) and nitrogenase activity based on acetylene reduction assay (ARA) both, per plant and nodule weight increased significantly in ‘I’ treatment compared to those in ‘NI.’ However, there were no significant differences between ‘SI’ and ‘NI’ for shoot biomass and ARA per plant. Nodule numbers decreased in ‘SI’ as compared to ‘NI,’ while they were not different between ‘I’ and ‘NI.’ This study suggests that simultaneous inoculation of ‘Yume-bio’ and rhizobia inhibits nodule development, while inoculation of ‘Yume-bio’ 1 week after inoculation of rhizobia is ideal for promoting soybean growth without inhibiting rhizobium infection in soybean.
1. Introduction
Application of plant growth-promoting rhizobacteria (PGPR) as a bio-fertilizer in agricultural fields is important since this can be expected to reduce the quantities of chemical fertilizers and achieves sustainable agriculture practices. PGPRs are microorganisms inhabiting plant rhizosphere endophytically or epiphytically, and which could provide certain benefits to plant growth, such as the production of phytohormones and supplying insoluble nutrients in soil to plants by solubilizing them. Numerous studies have reported that inoculation with PGPRs improves the growth of various crops such as rice, peanut, and mungbean (Win et al. Citation2019; Dey et al. Citation2004; Nihayati, Roviq, and Lintang Citation2019).
Bacillus is a bacterial genus that has been successfully commercialized because of its ability to maintain viability, a long shelf-life in storage (in the spore form), and bear a tolerance to stress. It has been reported that cell cultures of Bacillus pumilus strain TUAT1 enhance plant growth in several types of plants, such as rice, radish, and mustard (Win et al. Citation2018; Aung et al. Citation2015). Bacillus pumilus TUAT1 strain is commercially available as the active ingredient in the ‘Yume-bio’ bio-fertilizer from Asahi Agria Co., Ltd (Tokyo, Japan). TUAT1 has previously showed beneficial effects, such as promoting better nitrogen uptake and rooting system in rice (Ngo et al. Citation2019).
Legumes have symbiotic mutualism with rhizobia to obtain nitrogen by fixing N2 from the air. Co-inoculation of rhizobia with other bacteria on legumes sometimes inhibits symbiosis with rhizobia, especially in soybean, which is an important crop cultivated worldwide. Hashami et al. (Citation2019) reported that co-inoculation of Bradyrhizobium diazoefficiens USDA110 with various soil bacteria reduced root nodule numbers in soybean by inducing defense responses. Han et al. (Citation2020) reported on the interactive relationship between Bacillus and rhizobia as well as the effect of Bacillus on rhizobia–legume symbiotic nodulation under stress and found that the Bacillus cereus group promotes and suppresses the growth of Sinorhizobia and Bradyrhizobia, respectively, and affects its colonization in nodules. Han et al. (Citation2020) stated that the rhizosphere microbiota plays an important regulatory role in rhizobia–soybean symbiosis, as well as in the plant’s adaptation to external stress.
When applying bio-fertilizers with the ability to enhance root development to legume plants, it is important to find an inoculation method that does not inhibit symbiosis between rhizobium and plants. The objective of this study was to analyze the effect of co-inoculation timings of ‘Yume-bio’ bio-fertilizer containing B. pumilus TUAT1 and rhizobium on soybeans to establish the ideal inoculation method of the biofertilizer that do not inhibit soybean rhizobial nodulation.
2. Materials and methods
2.1. Experimental design and plant growth conditions
In this study, soybean (Glycine max L.) Merr cv ‘Enrei’ was inoculated with Bradyrhizobium diazoefficiens USDA110 at the time of sowing seeds. Bradyrhizobium diazoefficiens USDA110 were cultured in YMB (Yeast Mannitol Broth) medium (Jordan Citation1984) on a shaker at 100 rpm and 28°C for 1 week before inoculation of soybean seeds. Bradyrhizobium diazoefficiens USDA110 cells were washed and diluted with N-free solution (Sugiura et al. CitationForthcoming). Soybean seeds were sterilized with 70% ethanol for 30 s, followed by 3% (v/v) NaClO for 4 min, and then washed with sterilized water. Afterward, sterilized seeds were soaked in N-free nutrient solution containing Bradyrhizobium diazoefficiens USDA110 at 108 cells/mL for 15 min with gentle shaking. Later, two seeds were sown in vermiculite (Hiruishi-tech Co., Osaka Japan) moistened with the N-free solution at 0.6 mL/g vermiculite in the pot (362 cm3). The pot was covered with aluminum foil until sprouting. One week later, one of the seedlings was cut out so that only one seedling per pot remained. The plants were watered with the sterilized N-free solution (Sugiura et al. CitationForthcoming) every 2 days to maintain the moisture at 0.6 mL/g vermiculite.
For inoculation of Yume-bio (Asahi Agria Co., Ltd., Saitama, Japan), two treatments were applied: simultaneous inoculation at sowing (SI) and 1 week after germination (I). The control group was not inoculated (NI). The experiments were performed in four biological replicates. In Yume-bio, the spore cells of Bacillus pumilus TUAT1 (107 CFU g−1) are held in microscopic pores of zeolite granules, 2 mm in diameter. Yume-bio granules are applied on the surface of vermiculite, at 2% (v/v) of the pot.
For inoculation of spore cell culture of Bacillus pumilus TUAT1, high concentration of the spore cells obtained by culturing for 72 hours in Difco sporulation medium (Nicholson and Setlow Citation1990) were washed several times with sterile water purified with reverse osmosis membrane and resuspended by sterile 0.85% (w/v) saline water. The spore culture was incubated at 65°C for 1 h to kill vegetative cells and facilitate transformation to spore cell. The numbers of cells of inoculants were confirmed by plating. One milliliter of the spore cell culture at 107 CFU/mL was applied on the base of soybean seedlings at 1 week after germination.
Plants were grown in a growth room at 25°C under a 12 h light (250 µmol s−1 m−2)/dark cycle for 4 weeks. All plants were sampled at 4 weeks after sowing to measure SPAD (soil plant analysis development) value, shoot and root fresh weight, shoot and root dry weight, nodule number, nodule weight, and nitrogenase activity.
2.2. SPAD value measurement
Leaf chlorophyll content was measured using a SPAD meter (SPAD 502 Plus, Konica Minolta, Inc., Tokyo, Japan). Values were obtained from the center of the trifoliate leaves, from the third node from the top of the plant. Values were averaged for each treatment.
2.3. Nitrogenase activity
Nitrogenase activity was measured via acetylene reduction assay (ARA) using a gas chromatograph (Shimadzu Corporation GC-2014, Kyoto, Japan) equipped with a Porapak N column (Agilent Technologies, Santa Clara, CA, USA). Roots from each plant were placed in a sealed incubation glass bin (300 mL), and 10% (v/v) air was replaced with acetylene gas. Afterward, all samples were incubated at 28°C for 60 min. Lastly, 1 mL of gas sample from each bin was injected into the gas chromatograph for analysis (Artigas et al. Citation2020).
2.4. Statistical analysis
The data were statistically analyzed using IBM SPSS (v. 23.0.0; IBM, New York, NY, USA).
3. Results
The results from SPAD parameters from ‘NI,’ ‘I,’ and ‘SI’ were 27.7, 24.2, and 26.4, respectively – not showing any significant difference among the treatments (Supplementary Figure S1). Nonetheless, biomass dry weight showed significant differences between the treatments. Inoculation of Yume-bio (‘I’ and ‘SI’) significantly increased root dry weight compared to the control ()). As for shoot dry weight, a significant difference was found only between ‘I’ and ‘NI’ and not between ‘SI’ and ‘NI’ ()).
Figure 1. Root dry weight (a) and 'shoot dry weight (b)
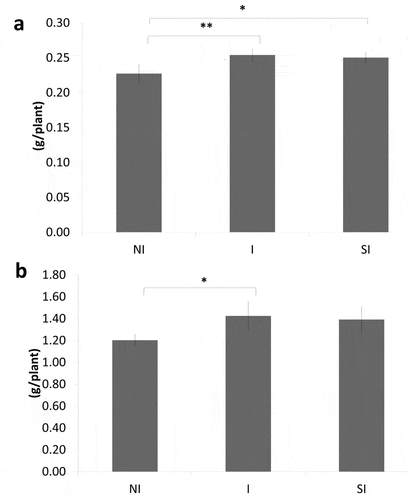
The nodule number significantly decreased in ‘SI’ treatment as compared to the control ()), indicating that simultaneous inoculation with Yume-bio inhibited infection of rhizobium into plant roots. Between ‘I’ and ‘NI,’ there was no significant difference in nodule number. There were also no significant differences in nodule fresh weight between ‘I’ and ‘SI’ when compared to ‘NI’ ()).
Figure 2. Nodule number (a) and nodule fresh weight (b)
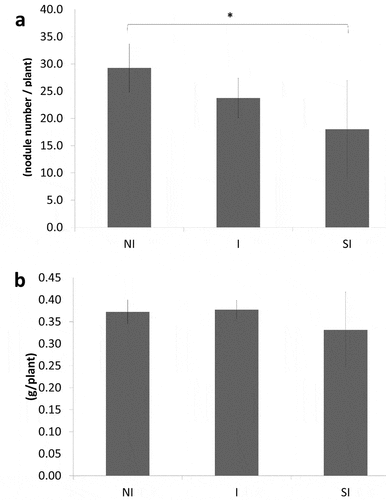
As shown in ), ARA per plant of ‘I’ treatment was 1.48 C2H4μmol/h/g which was significantly higher than the 1.03 C2H4μmol/h/g observed in ‘NI’ treatment, while there was no significant difference between ‘SI’ and ‘NI.’ The ARA per nodule weight was 4.35 C2H4 μmol/h/g with ‘I’ treatment, which was 1.6-fold significantly higher than that with ‘NI’ ()). Again, there was no significant difference in ARA per nodule weight between ‘SI’ and ‘NI.’
Figure 3. ARA per plant (a) and ARA per nodules (b)
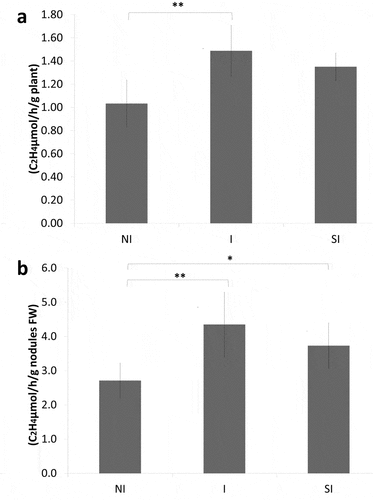
To confirm the growth promotion effect of Yume-bio on soybean plants is ascribed to the spore cells of Bacillus pumilus TUAT1 not to zeolite supporting the cells, the spore cell culture was applied to soybean plants one week after inoculating rhizobia. As shown in Supplementary Figure S2, plant biomass was increased while nodule numbers were not changed by spore cell application compared to ‘NI.’
4. Discussion
The application of the bio-fertilizer, Yume-bio, resulted in a clear increase in root dry weight in the ‘I’ and ‘SI’ treatments ()), and in shoot dry weight with the ‘I’ treatment. Previous research on rice plants from Seerat et al. (Citation2019) showed that root dry weight of ‘Hitomebore’ significantly increased with inoculation of viable spores of Bacillus pumilus TUAT1. Ngo et al. (Citation2019) also showed that inoculation of Bacillus pumilus TUAT1 cells alone in rice increased the fresh weight of shoots and roots and promoted crown root formation. Our results indicate that Bacillus pumilus TUAT1 in Yume-bio can promote the growth of soybean plants, especially in their roots, as observed in rice plants. The growth promotion effect on soybean plants was also observed by inoculating Bacillus pumilus TUAT1 spore cells, agreeing with the results in rice plant (Supplementary Figure S2).
Bacillus pumilus TUAT1 in Yume-bio showed inhibitory effects on nodule formation when inoculated at the same time as the rhizobium Bradyrhizobium diazoefficiences USDA 110 (‘SI’ treatment) ()). This may be the reason why shoot dry weight did not significantly increase in ‘SI’ when compared with the control, ‘NI.’ In Yume-bio, Bacillus pumilus TUAT spore cells are supported in zeolite. There is no report that zeolite inhibits rhizobium nodulation, rather zeolite is reported to promote rhizobium infection (Sato Citation1994); thus, it is unlikely that inhibition of nodulation by Yume-bio application is due to zeolite. As inhibitions of Bradyrhizobia infection to soybean plants were reported by simultaneous inoculation with bacterial cultures of Bacillus or other many soil bacteria (Han et al. Citation2020: Hashami et al. Citation2019), it is considered that Bacillus pumilus TUAT1strain itself inhibited nodulation by the simultaneous inoculation with the Bradyrhizobium in this study.
In a previous study by Hashami et al. (Citation2019), the number of nodules was lower in the simultaneous inoculation of Bradyrhizobium diazoefficiences USDA 110 with most of soil bacteria tested on the super-nodulation mutant NOD1-3 soybean than the inoculation of Bradyrhizobium diazoefficiences USDA 110 alone. These soil bacteria increased the mRNA accumulation of several pathogenesis-related genes in soybean roots, which are involved in defense (Hashami et al. Citation2019). Thus, simultaneous inoculation of Bacillus pumilus TUAT1 and rhizobium in our study may have also induced defense responses in soybean roots resulting in inhibition of rhizobium infection, which should be further investigated in future experiments.
In contrast, inoculation with Yume-bio 1 week after rhizobium inoculation did not inhibit nodule formation ()) and significantly increased both the root and shoot dry weights (). Furthermore, treatment ‘I’ increased nitrogen fixation activity evaluated with ARA as compared to the control (). Inhibition of nodulation was not found, indicating that infection of rhizobium had been already occurred during the first week after germination before application of Yume-bio. Thus, it was found that a suitable timing of Yume-bio inoculation was around 1 week after germination for soybean. Rhizobium inoculated on seeds was already infected in plants 1 week after germination; thus, the infection process was not inhibited by Bacillus pumilus TUAT1.
Furthermore, nitrogen fixation activity evaluated with ARA was higher with ‘I’ treatment than the ‘NI,’ even though nodule numbers and weights were not greatly different, suggesting a possibility that an inoculation of Bacillus pumilus TUAT1 supports the production of efficient nodules. Dalton et al. (Citation1986) reported that the peaks of ARA and leghemoglobin content are around 4 weeks after planting; thus, our application method of Yume-bio on soybean promotes soybean growth with maintaining high nitrogen fixation at the peak of activity. This is considered to be important to obtain a high yield as 50–60% of seed nitrogen on average is provided through biological nitrogen fixatin in soybean (Salvagiotti et al. Citation2008). In the future research, the effects of Yume-bio are needed to be tested in the field condition. In the present experiment, only Bradyrhizobium diazoefficiences USDA 110 and Bacillus pumilus TUAT1 were existed in the pots; however, numerous other bacteria live in the field soils with which Bacillus pumilus TUAT1 may compete. In addition, field conditions are not controlled as in the present experiment. To obtain the effect of Bacillus pumilus TUAT1 for stably increasing soybean yield in fields, improvement based on the preset application method is considered to be required.
Supplemental Material
Download PDF (128.3 KB)Acknowledgments
The authors would especially like to thank the Ministry of Education, Culture, Sports, Science, and Technology (MEXT) for the scholarship to R.F.M.H.
Disclosure statement
No potential conflict of interest was reported by the author(s).
Supplementary material
Supplemental data for this article can be accessed here.
Additional information
Funding
References
- Artigas, R. M. D., M. Espana, S. Lewandowska, K. Yuan, S. Okazaki, N. Ohkama-Ohtsu, and T. Yokoyama. 2020. “Phylogenetic Analysis of Symbiotic Bacteria Associated with Two Vigna Species under Different Agro-Ecological Conditions in Venezuela.” Microbes and Environments 35 (1). doi:10.1264/jsme2.ME19120.
- Aung, H. P., S. Djedidi, A. Z. Oo, Y. S. Aye, T. Yokoyama, S. Suzuki, H. Sekimoto, and S. D. Bellingrath-Kimura. 2015. “Growth and Cs-137 Uptake of Four Brassica Species Influenced by Inoculation with a Plant Growth-promoting Rhizobacterium Bacillus pumilus in Three Contaminated Farmlands in Fukushima Prefecture, Japan.” Science of the Total Environment 521: 261–269. doi:10.1016/j.scitotenv.2015.03.109.
- Dalton, D. A., S. A. Russell, F. J. Hanus, G. A. Pascoe, and H. J. Evans. 1986. “Enzymatic Reactions of Ascorbate and Glutathione that Prevent Peroxide Damage in Soybean Root Nodules.” Proceedings of the National Academy of Sciences of the United States of America 83 (11): 3811–3815. doi:10.1073/pnas.83.11.3811.
- Dey, R., K. K. Pal, D. M. Bhatt, and S. M. Chauhan. 2004. “Growth Promotion and Yield Enhancement of Peanut (Arachis hypogaea L.) By Application of Plant Growth-promoting Rhizobacteria.” Microbiological Research 159 (4): 371–394. doi:10.1016/j.micres.2004.08.004.
- Han, Q., Q. Ma, Y. Chen, B. Tian, L. X. Xu, Y. Bai, W. F. Chen, and X. Li. 2020. “Variation in Rhizosphere Microbial Communities and Its Association with the Symbiotic Efficiency of Rhizobia in Soybean.” Isme Journal 14 (8): 1915–1928. doi:10.1038/s41396-020-0648-9.
- Hashami, S. Z., H. Nakamura, N. Ohkama-Ohtsu, K. Kojima, S. Djedidi, I. Fukuhara, M. D. Haidari, H. Sekimoto, and T. Yokoyama. 2019. “Evaluation of Immune Responses Induced by Simultaneous Inoculations of Soybean (Glycine max [L.] Merr.) With Soil Bacteria and Rhizobia.” Microbes and Environments 34 (1): 64–75. doi:10.1264/jsme2.ME18110.
- Jordan, D. C. 1984. “Rhizobiaceae.” In Bergey’s Manual of Systematic Bacteriology, edited by N. R. Krieg and J. G. Holt, 234–244. Vol. 1. Baltimore: Williams and Wilkins.
- Ngo, N. P., T. Yamada, S. Higuma, N. Ueno, K. Saito, K. Kojima, M. Maeda, et al. 2019. “Spore Inoculation of Bacillus pumilus TUAT1 Strain, a Biofertilizer Microorganism, Enhances Seedling Growth by Promoting Root System Development in Rice.” Soil Science and Plant Nutrition 65 (6): 598–604. doi:10.1080/00380768.2019.1689795.
- Nicholson, W. L., and P. Setlow. 1990. “Sporulation, Germination and Outgrowth.” In Molecular Biological Methods for Bacillus, edited by C. R. Harwood and S. M. Cutting, 391–450. Chichester: Wiley.
- Nihayati, E., M. Roviq, and C. W. Lintang. 2019. “Improvement of Mungbean (Vigna radiata L) Yield through Supplementing the Plant Growth-promoting Rhizobacter (PGPR) and Mycorriza.” Eurasia Journal of Biosciences 13: 2065–2070.
- Salvagiotti, F., K. G. Cassman, J. E. Specht, D. T. Walters, A. Weiss, and A. Dobermann. 2008. “Nitrogen Uptake, Fixation and Response to Fertilizer N in Soybeans: A Review.” Field Crop Research 108 (1): 1–13. doi:10.1016/j.fcr.2008.03.001.
- Sato, T. 1994. “Chapter 9 Effects of Rhizobium Inoculation on Nitrogen Fixation and Growth of Leguminous Green Manure Crop Hairy Vetch (Vicia villosa Roth).” In Advances in Biology and Ecology of Nitrogen Fixation, edited by T. Ohyama. Intec Open. doi:10.5772/56992.
- Seerat, A. Y., T. Ookawa, K. Kojima, N. Ohkama-Ohtsu, M. Maeda, S. Djedidi, S. Habibi, H. Sekimoto, A. Abe, and T. Yokoyama. 2019. “Evaluation of the Effects of Spores and Their Heat-treated Residues from Different Bacillus Strains on the Initial Growth of Rice Plants.” Soil Science and Plant Nutrition 65 (2): 122–136. doi:10.1080/00380768.2018.1551042.
- Sugiura, H., S. Sugihara, T. Kamiya, R. M. D. Artigas, M. Miyatake, T. Fujiwara, T. Ohyama, et al. Forthcoming. “Sulfur Application Enhances Secretion of Organic Acids by Soybean Roots and Solubilization of Phosphorus in Rhizosphere.” Soil Science and Plant Nutrition. doi:10.1080/00380768.2021.1919011.
- Win, K. T., K. Okazaki, T. Ookawa, T. Yokoyama, and Y. Ohwaki. 2019. “Influence of Rice-husk Biochar and Bacillus pumilus Strain TUAT-1 on Yield, Biomass Production, and Nutrient Uptake in Two Forage Rice Genotypes.” Plos One 14 (7): 7. doi:10.1371/journal.pone.0220236.
- Win, K. T., A. Z. Oo, N. Ohkama-Ohtsu, and T. Yokoyama. 2018. “Bacillus pumilus Strain TUAT-1 and Nitrogen Application in Nursery Phase Promote Growth of Rice Plants under Field Conditions.” Agronomy-Basel 8: 10. doi:10.3390/Agronomy8100216.