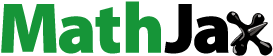
ABSTRACT
Nitrogen cycle, a most important elemental cycle in earth ecosystem, is carried out by three major microbial processes including nitrogen fixation, nitrification and denitrification. Nitrification is particularly important in agricultural soil ecosystems because it is involved in nitrogen loss, groundwater pollution by nitrogen leaching, and greenhouse gas nitrous oxide emission. Recent genomic, metagenomic and physiological analysis of nitrifying microorganisms gives new insights into their ecology and functions in soils. In the last decade, there have been a number of important findings regarding nitrification. The discovery of nitrifying archaea and complete nitrifying bacteria has overturned the conventional theory that nitrification is driven by ammonia-oxidizing and nitrite-oxidizing bacteria. Each of the newly discovered nitrifying microorganisms has unique properties, for example, a different affinity for ammonia. These nitrifying microorganisms have been shown to actually play important roles in nitrification in agricultural soils. Soil conditions and agricultural practices such as fertilization and tillage have been shown to influence the contribution of each nitrifying microorganism to the activity of nitrification. In addition, nitrification inhibition technologies have been developed to prevent the loss of nitrogen fertilizer and the of nitrous oxide emission. This review provides an overview of recent researches on the diversity and characteristics of nitrifying microorganisms, soil factors affecting their ecology in soil, their involvement in nitrous oxide emissions, and nitrification control technologies.
1. Introduction
In recent decades, anthropogenic nitrogen input, either directly or indirectly, has dramatically increased due to agricultural and industrial activities. The high-production agricultural systems rely on large fertilizer inputs to sustain productivity. Global inputs of N fertilizer and N fixation by crops are currently estimated to exceed the natural N inputs to terrestrial systems by biological N2 fixation (Fowler et al. Citation2013). Anthropogenic nitrogen input disturbs the nitrogen cycle in the earth, which exceeds planetary boundaries (Steffen et al. Citation2015). Therefore, we need to improve the efficiency of anthropogenic nitrogen utilization in crop production while reducing the negative effects of anthropogenic nitrogen input on the environment (Galloway et al. Citation2008).
The type and amount of nitrogen fertilizer have a significant impact on the microbial community structure involved in nitrification, denitrification, and nitrogen fixation. Nitrifying microorganisms are greatly influenced by nitrogen fertilizers because they obtain energy from ammonia oxidation or nitrite oxidation (Norton and Ouyang Citation2019). Nitrification is directly involved in the emission of the greenhouse gas nitrous oxide, nitrate contamination of freshwater resources, eutrophication, and loss of nitrogen from agricultural fields (Robertson et al. Citation2013; Norton and Ouyang Citation2019; Prosser et al. Citation2020). Therefore, we need to have a better understanding of nitrification processes to improve the utilization efficiency of nitrogen fertilizers and minimize negative impact of nitrification on the environment.
Since the discovery of nitrifying microorganisms by Sergei Winogradsky at the end of the 19th century (Winogradsky Citation1891), nitrification, which is the oxidation of ammonia to nitrate via nitrite, has been considered a two-step microbial reaction process performed by two distinct functional groups: ammonia-oxidizing bacteria (AOB) and nitrite-oxidizing bacteria (NOB). However, metagenomic analysis revealed the existence of a novel group of archaea that can oxidize ammonia in several environments (Treusch et al. Citation2005), and since then a number of pure cultures of ammonia-oxidizing archaea have been isolated from the various several environments including soil and ocean (Könneke et al. Citation2005; Lehtovirta-Morley Citation2018). Recently, it was found that a new complete ammonia oxidation (comammox) bacterium, Nitrospira, can oxidize ammonia to nitrate in a single microorganism (Daims et al. Citation2015; van Kessel et al. Citation2015). Thus, knowledge about nitrifying microorganisms is rapidly increasing. In this review, we focus on the latest findings on the following five microbial groups: (1) autotrophic ammonia oxidizing bacteria (AOB), (2) autotrophic nitrite oxidizing bacteria (NOB), (3) complete ammonia oxidizing bacteria (Comammox), (4) autotrophic ammonia oxidizing archaea (AOA), and (5) heterotrophic nitrifiers. We also briefly describe anaerobic ammonia oxidation (anammox), which plays an important role in the dynamics of ammonia in the soil. Then, we discuss the characteristics of these microorganisms and the reactions that they mediate in the soil. Finally, we introduce the control technology for them, referring to recent research results obtained using advanced methods such as metagenomics and genomics.
2. Diversity of nitrifiers
2.1. Autotrophic ammonia oxidizing bacteria (AOB)
Ammonia oxidizing bacteria (AOB) are classified into the classes Betaproteobacteria or Gammaproteobacteria. Nitrosomonas and Nitrosospira have been found in various environments such as soils, oceans, lakes, and activated sludges (Prosser and Embley Citation2002). Nitrosospira (encompassing Nitrosolobus and Nitrosovibrio) (Head et al. Citation1993; Stephen et al. Citation1996) is the dominant AOB genus in agricultural and forest soils (Kowalchuk and Stephen Citation2001). In contrast, γ-AOB of the Nitrosococcus genus, including N. oceani, N. watosonii, and N. halophilus, have been found in saline or hyper-saline aquatic environments such as oceans and salt lakes (Campbell et al. Citation2011). Recently, gammaproteobacterial AOB were isolated from the strongly acidic soil of the tea fields. The isolated γ-AOB, Candidatus Nitrosoglobus terrae, is acid-tolerant and non-halophilic, whose genome is considerably smaller than those of other γ-AOB and lacks several genes associated with salt tolerance that are unnecessary for survival in soil (Hayatsu et al. Citation2017). The potential role of Ca. Nitrosoglobus in wastewater treatment systems has recently been demonstrated (Wang et al. Citation2021b). The acid-tolerant γ-AOB, Ca. Nitrosacidococcus tergens, was also isolated from bioreactor cultivation using an acidic air biofilter (Picone et al. Citation2020).
In soil ecosystems, β-AOB, Nitrosospira, and Nitrosomonas are two dominant genera of terrestrial AOB, as revealed by culture-and metagenome-based investigations (Kowalchuk and Stephen Citation2001; Jiang et al. Citation2014). The lineages of β-AOB are defined and can be divided into clusters 0 to 8 and cluster 0 to 13 based on 16S rRNA gene sequences (Norton Citation2011) and ammonia monooxygenase subunit A (amoA) sequences, respectively, from pure cultures and a variety of environments (Avrahami and Conrad Citation2003). Each β-AOB cluster responds to various environmental factors, such as soil pH (Nicol et al. Citation2008), temperature (Fierer et al. Citation2009), land use (Fierer et al. Citation2009) and fertilization (Lin et al. Citation2018; Yang et al. Citation2020). Different AOB species have functional differentiation and do not equally contribute to nitrification in soil ecosystems. For example, Nitrosospira cluster 8a played a significant role in the nitrification in acidic soil under long-term inorganic and organic fertilization (Lin et al. Citation2018). The dominant AOB community structure was changed from Nitrosospira cluster 2 to cluster 3 by treatment with biochar (He et al. Citation2018).
Nitrous oxide gas (N2O) is generated by two metabolic pathways, ammonia oxidation and nitrifier-denitrification but the mechanisms involved in this process are not completely understood. In AOB, ammonia monooxygenase (AMO) oxidizes ammonia to hydroxylamine (NH2OH), which is subsequently converted to nitrite by hydroxylamine dehydrogenase (HAO) under oxic conditions. Nitrous oxide is formed during NH2OH oxidation by chemical abiotic or biochemical reactions (Prosser et al. Citation2020; Stein Citation2019). Intermediate compounds such as NH2OH, nitrite, and NO produced during biological ammonia oxidation are converted to nitrous oxide by an abiotic reactions (Liu et al. Citation2017). On the other hand, there are two biochemical N2O production pathways. One is called nitrifier-denitrification, in which nitrite (NO2−) is reduced to NO and N2O by nitrite reductase (NIR) and NO reductase (NOR), respectively, under O2 limitation and low pH conditions (Shaw et al. Citation2006; Wrage-Mönnig et al. Citation2018). The second is the HAO-mediated incomplete oxidation of NH2OH to NO, which is subsequently reduced to N2O by NO reductase (Kozlowski, Price, and Stein Citation2014). A recent study revealed a novel pathway for N2O production in N. europaea through the oxidation of NH2OH by cytochrome P460 (Caranto and Lancaster Citation2017; Caranto, Vilbert, and Lancaster Citation2016).
2.2. Autotrophic nitrite oxidizing bacteria (NOB)
Nitrite is an important intermediate in many nitrogen metabolism processes mediated by microbes, including nitrification and denitrification. Moreover, nitrite is reactive and toxic to some microorganisms and eukaryotes. Therefore, NOB have a significant regulatory function in the nitrogen cycle and microbial ecology in the soil. Nitrite oxidizers are a phylogenetically diverse functional group and classified into seven genera within four phylum in parentheses: Nitrobacter (α-proteobacteria), ‘Candidatus Nitrotoga’ (β-proteobacteria), Nitrococcus (γ-proteobacteria), Nitrospira (Nitrospirae), ‘Nitrolancetus’ (Chloroflexi), Nitrospina (Nitrospinae) and ‘Candidatus Nitromaritima’ (Nitrospinae) (Daims, Lücker, and Wagner Citation2016). NOB are widely distributed in natural and urban environments, including seawater, freshwater systems, sewage treatment plants, and various soil ecosystems (Daims et al. Citation2011).
Nitrobacter, Nitrospira, Candidatus Nitrotoga, Nitrospina, ‘Nitrolancetus’ and Nitrococcus have been identified in the soil by metagenomic analysis (Wang et al. Citation2021a). Since the abundance of Nitrobacter and Nitrospira is higher than those of the other four genera and they are more responsive to agricultural management, the two genera are considered to be the more important functional players in the NOB community in soil (Han et al. Citation2018). Nitrite oxidation kinetic analysis of six pure cultures, including three Nitrobacter and three Nitrospira species, showed that Nitrospira spp. are adapted to a highly substrate-limited environment and that Nitrobacter spp. have affinity to a wide range of substrates and an advantage under nitrite-rich environments (Nowka, Daims, and Spieck Citation2015). Therefore, Nitrobacter is commonly found in nitrogen-rich environments such as wastewater. In tillage/no-tillage agricultural soil with high nitrogen availability, potential nitrite oxidation exhibited a strong positive correlation with the abundance of Nitrobacter and a weak negative correlation with the abundance of Nitrospira-like NOB (Attard et al. Citation2010). Fertilization of lodgepole pine and spruce stands in forest soil increased Nitrobacter-like NOB abundance but did not affect Nitrospira-like NOB abundance. Nitrobacter-like NOB abundance correlated with potential nitrification activity and soil nitrate concentration, but no such correlations were observed for Nitrospira-like NOB (Wertz, Leigh, and Grayston Citation2012). In the paddy field soil, Nitrobacter-like NOB preferred the rhizosphere compartment and responded positively to increased nitrogen levels, whereas Nitrospira-like NOB were dominant in surface soil, which is a nutrient-limited environment (Ke et al. Citation2013). Both Nitrospira and Nitrobacter-like NOB contributed to the nitrite-oxidation process in red soil, and these communities were shaped by pH, total nitrogen, and soil organic carbon (Han et al. Citation2018). Ca. Nitrotoga are distributed in various environments, including soil and sediment, and has been enriched and characterized for its genome and physiology (Kitzinger et al. Citation2018; Ishii et al. Citation2017, Citation2020). Ca. Nitrotoga can tolerate lower nitrite and oxygen concentrations (Nowka, Daims, and Spieck Citation2015). However, further studies are needed to clarify the role of Ca. Nitrotoga in soil nitrification.
2.3. Complete ammonia oxidizing bacteria (Comammox)
Complete nitrification has been known to be mediated by AOB and NOB for over a century (Costa, Pérez, and Kreft Citation2006). However, a century after the discovery of AOB and NOB, AOA archaea were discovered as a second major group of ammonia oxidizers (Könneke et al. Citation2005). Recently, it was discovered that a single microorganism belonging to the genus Nitrospira can mediate complete nitrification of ammonia to nitrate via nitrite, a process termed as comammox (complete oxidation of ammonia to nitrate) (Daims et al. Citation2015; van Kessel et al. Citation2015). The thermodynamic feasibility of the comammox process was predicted by Costa, Pérez, and Kreft (Citation2006). Comammox yields more energy (EquationEquation 1(1)
(1) ) than does ammonia oxidation to nitrite (EquationEquation 2
(2)
(2) ) and nitrite oxidation to nitrate (EquationEquation 3
(3)
(3) ). Thus, comammox is more advantageous than other nitrifying bacteria and archaea in environments with low substrate concentrations (Costa, Pérez, and Kreft Citation2006).
The Nitrospira genus is widely distributed in both natural and engineered ecosystems (Daims et al. Citation2011) and is divided in six sublineages based on the DNA sequences of 16 rRNA genes (Lebedeva et al. Citation2011). All known comammox organisms belong to Nitrospira sublineage II (Lawson and Lücker Citation2018; Takahashi et al. Citation2020). Canonical NOB in sublineage II lacks the activity and genes involved in ammonia oxidation.
Nitrospira inopinata in pure culture have a higher substrate affinity for ammonia than AOA and AOB in soil, as the Km of N. inopinata for NH3 (63 nM) is 4- to 2500-fold lower than that of AOB; thus, comammox bacteria could have a competitive advantage in soil with a very low ammonia concentration (Kits et al. Citation2017). On the other hand, in comparison with AOA and especially AOB, N. inopinata produces N2O at lower levels (Kits et al. Citation2019). Comammox are distributed in various environments (Shi et al. Citation2020) and significantly contribute to soil nitrification (Osburn and Barrett Citation2020).
2.4. Autotrophic ammonia oxidizing archaea (AOA)
AOA belong to the phylum Thaumarchaeota and are divided into five main clusters: Nitrososphaera, Nitrosocosmicus (previously Nitrososphaera-sister lineage), Nitrosocaldus, Nitrosotalea, and Nitrosopumilus (Pester et al. Citation2012). Recently, Alves et al. (Citation2018) reconstructed a robust and highly resolved archaeal amoA gene phylogeny and defined a novel multilevel taxonomy (Alves et al. Citation2018). After the isolation of Nitrosopumilus maritimus from seawater, many AOA have been isolated from various environments. Nitrososphaera viennensis was isolated from soil at pH 8.0, and their optimal growth pH was 7.5 (Tourna et al. Citation2011; Stieglmeier et al. Citation2014a). Ca. Nitrosotalea devanaterra is an obligately acidophilic AOA that grows in the pH range of 4.0 to 5.5 (Lehtovirta-Morley et al. Citation2011, Citation2016b). Members of the Nitrosotalea lineage are widely distributed and abundant in acidic soils (Gubry-Rangin et al. Citation2011).
Kinetic analysis revealed a higher affinity of AOA to NH3 compared with that of AOB isolated from soil samples. Thus, growth and ammonia-oxidizing activity of AOA are more competitively advantageous than are those of AOB under lower ammonia concentration conditions (Martens-Habbena et al. Citation2009). Indeed, Nitrosopumilus maritimus SCM1 revealed the lowest reported Km value (~3 nM) for NH3 among all the cultured ammonia oxidizers. However, most recently, this substantially higher affinity of AOA to NH3 in comparison with that of AOB has been questioned by several reports (Kits et al. Citation2017), as the affinity of several isolated AOA for ammonia was not significantly different from that of AOB.
AOA significantly contribute to N2O production in various environments, based on the AOA amoA abundance in environments and the N2O isotopic signature (Santoro et al. Citation2011; Jung et al. Citation2014). However, N2O yields (N2O-N per NO3-N produced) from AOA cultures are generally lower than those from AOB culture (Hink et al. Citation2017, Citation2018). In AOA, N2O is considered to be produced via abiotic reactions because there is no genomic or physiological evidence for the enzymatic production of N2O through NH3 oxidation (Kozlowski et al. Citation2016; Stieglmeier et al. Citation2014b). However, Jung et al. (Citation2019) indicated that Nitrosocosmicus oleophilus might be able to enzymatically denitrify nitrite to N2O at low pH conditions (Jung et al. Citation2019).
2.5. Heterotrophic nitrification
Heterotrophic nitrification is generally defined as the oxidation of inorganic and organic reduced forms of nitrogen, to nitrite or nitrate, by a wide range of eukaryotes (mostly fungi) and heterotrophic bacteria (De Boer and Kowalchuk Citation2001). Heterotrophic nitrification is not linked to energy conservation (i.e., ATP production), and its cell-based rates are significantly lower than those in cultivated autotrophic nitrifiers (Verstraete and Focht Citation1977). However, heterotrophic nitrification might have an important role in soils under particular conditions that are unfavorable for autotrophic nitrifiers (Li et al. Citation2018b). Little is known about the prevalence and diversity of heterotrophic nitrifiers in different soil environments. In some cases, heterotrophic nitrification has a low efficiency in oxidizing ammonia to nitrite or nitrate. Some heterotrophic nitrification organisms metabolize organic nitrogen to produce nitrite and nitrate (Islam, Chen, and White Citation2007). It has also been shown that this process can produce nitrous oxide (Zhang et al. Citation2018; Zhang, Mueller, and Cai Citation2015).
Generally, 15N-labeled NH4+, NO3−, were applied with C2H2, which are specific inhibitors of denitrification and autotrophic nitrification at different concentrations, to distinguish the relative contributions of heterotrophic nitrification, autotrophic nitrification, and denitrification to total NO3− and NO2 production. In most cases, heterotrophic nitrification refers to the oxidation of organic N compounds to NO3− (Killham Citation1990; Zhang, Mueller, and Cai Citation2015). This type of heterotrophic nitrification occurs commonly in forest soils that have low soil pH and high soil C/N ratio (Huygens et al. Citation2008; Zhu et al. Citation2015). In the coniferous forest soil, heterotrophic nitrification is the dominant NO3− production pathway and contributes with 95% of the total nitrification (Zhang et al. Citation2013). Heterotrophic nitrification is an important pathway involved in N2O emission from the soil (Lan et al. Citation2020; Zhang et al. Citation2018). The contribution of heterotrophic nitrification to N2O emission is more significant than is autotrophic nitrification in acidic (Stange et al. Citation2013) and neutral soils (Müller et al. Citation2014).
2.6. Environmental factors affecting nitrification
2.6.1. Occurrence of AOB, AOA, and comammox bacteria in agricultural soils
The abundance, community structure, and relative contributions to nitrification of AOA, AOB, and comammox are influenced by correlated physical and chemical factors such as pH, ammonia concentration, temperature, and water content in soils (Taylor et al. Citation2012; Giguere et al. Citation2015; Li et al. Citation2019a). Since the discovery of AOA (Könneke et al. Citation2005), extensive research has been conducted to elucidate the contribution of AOA and AOB to nitrification and their niche specialization in soil ecosystems (Prosser and Nicol Citation2012; Lehtovirta-Morley Citation2018). These studies show, in many cases, that AOA is often more abundant than AOB in soils (Clark et al. Citation2020; Leininger et al. Citation2006; Schleper and Nicol Citation2010; He et al. Citation2012). Since the abundance of AOB, AOA, and comammox varies greatly depending on the soil management and soil conditions, it is necessary to confirm the relationship between the abundance of AOB, AOA, and comammox and the nitrification activity by referring to the original paper. Specific inhibitors of AOA and AOB and stable isotope probing techniques have been used to directly link the activities of AOA and AOB to the total nitrification activity in soil (Taylor et al. Citation2013). Although there is not enough information on the ecology of comammox organisms, there has been increasing numbers of reports on their abundance and their contribution to nitrification in various environments (Li et al. Citation2019a; Osburn and Barrett Citation2020). According to these reports, comammox has been detected in many soils, and their abundance may even exceed that of AOB. The abundance of comammox amoA genes was comparable to that of AOA and AOB amoA genes, suggesting that comammox is also involved in nitrification activity in soil ecosystems. Given that nitrification is considered to be driven by AOA and AOB, if the contribution of comammox to nitrification becomes clear, it will be necessary to reconsider the nitrogen dynamics and nitrogen fertilization techniques in arable soil. This review outlines the relationship between these nitrifying bacteria and some important environmental factors.
2.6.2. Soil pH
Soil pH is one of the most important factors affecting activity, population, and diversity of nitrifying microorganisms (Li et al. Citation2018b; Aigle, Prosser, and Gubry-Rangin Citation2019). Nitrification activity was observed in a wide range of soil pH values (Booth, Stark, and Rastetter Citation2005). The substrate of ammonia monooxygenase Amo, which mediates the first step of ammonia oxidation in autotrophic ammonia oxidizer, is ammonia rather than ammonium (Suzuki, Dular, and Kwok Citation1974). Ammonia concentration decreases exponentially with decreasing pH due to the ionization of ammonia to ammonium. Therefore, the low ammonia availability at low pH is a major factor limiting AOB growth in acidic soils. However, many studies have shown the contribution of AOB to nitrification activity in acidic soils (Aigle, Prosser, and Gubry-Rangin Citation2019; De Boer, Gunnewiek, and Laanbroek Citation1995; Allison and Prosser Citation1991; Long et al. Citation2012; Jiang and Bakken Citation1999; Petersen et al. Citation2012; Wertz, Leigh, and Grayston Citation2012). Several reports indicate that the contribution of AOA to nitrification activity is more important than that of AOB in acidic soil (Hu, Xu, and He Citation2014; Li et al. Citation2018b; He et al. Citation2020; Li et al. Citation2019b). AOA amoA gene abundance decreased with increasing soil pH, while AOB amoA gene abundance increased with increasing pH (Nicol et al. Citation2008). A biogeographical study on AOA and AOB showed that their distribution was driven by soil pH, and the AOA/AOB ratio decreased with an increase in soil pH in 65 soil samples collected from different regions (Hu et al. Citation2013b).
The discovery of the acidophilic ammonia oxidizer, Nitrosotalea devanaterra (Lehtovirta-Morley et al. Citation2011, Citation2014, Citation2016b; Yang et al. Citation2020) has significantly expanded our understanding of nitrification in acidic environments because AOB and AOA isolated from the environment before the discovery was neutrophilic and not able to grow below pH 6.5. Moreover, an acid-tolerant ammonia-oxidizing gamma proteobacterium, Candidatus Nitrosoglobus terrae, was isolated from strongly acidic tea field soils (Hayatsu et al. Citation2017). Recently, an enrichment culture of acid-adapted comammox Nitrospira was obtained from the tea field acidic soil from which Candidatus Nitrosoglobus terrae was isolated (Takahashi et al. Citation2020), suggesting that comammox Nitrospira may also play an important role in acidic soils. The possibility that acidophilic or acid-tolerant nitrifying bacteria are still overlooked should be considered.
The significant contribution of heterotrophic nitrification has been observed in restricted to natural or semi-natural ecosystems, particularly acidic coniferous forest soils (Killham Citation1990; Li et al. Citation2018b). As a typical example, in soils from coniferous forests, fungal heterotrophic nitrification was responsible for most of the nitrification activity (Zhu et al. Citation2015). Besides coniferous forest soil, significant heterotrophic nitrification activity was detected in acidic arable (Zhang et al. Citation2018, Citation2020) and tea field soils (Yokoyama et al. Citation2012) although not the primary nitrifier.
2.6.3. Ammonia concentration
AOA, AOB, and comammox co-exist in various soils, including agricultural soils (Pjevac et al. Citation2017; Liu et al. Citation2019b; Li et al. Citation2019a; Wang et al. Citation2020). The concentration of ammonia, which is an energy source of autotrophic ammonia oxidizers, is one of the most important environmental factors influencing niche differentiation (Prosser and Nicol Citation2012). Numerous studies including field and microcosm experiments determined the effect of ammonia concentration on AOA and AOB, indicating that AOA and AOB were more abundant in conditions with low and high ammonia concentrations, respectively (Prosser and Nicol Citation2012). Although the application of mineral nitrogen fertilizer alters the abundance and diversity of AOB, its effect on AOA is not significant in several agricultural soils (Ouyang et al. Citation2016; Ai et al. Citation2013). On the other hand, AOA abundance increased with ammonia supplementation through mineralization of compost manure or soil organic matter (Levičnik-Höfferle et al. Citation2012; Liu et al. Citation2019a; Clark et al. Citation2020). These studies indicate that AOA and AOB prefer low concentrations of ammonia generated by mineralization of organic N and high concentrations of inorganic ammonia, respectively (Ouyang et al. Citation2016; Ouyang, Norton, and Stark Citation2017).
There are few reports on the effects of ammonia on abundance and diversity of comammox, since these bacteria were discovered only a few years ago. Stable isotope probing experiments revealed that the abundance of comammox Nitrospira clade A was significantly higher than that of AOA and AOB and might contribute to nitrification in the tested agricultural soils amended with N fertilizers (Li et al. Citation2019a). Comammox Nitrospira clade B also appears to play an active role in nitrification and to outcompete AOB at low concentrations of ammonia supplied from mineralized organic nitrogen (Wang et al. Citation2019). The abundance of comammox Nitrospira clade A was significantly increased by the addition of nitrogen fertilizers and was significantly suppressed by nitrification inhibitors, suggesting their potential contribution to nitrification in the soil (Li et al. Citation2020). Soil pH-dependent ammonia availability, rather than soil N content itself, determines the niche separation of comammox Nitrospira and canonical nitrifiers in forest ecosystems under long-term N deposition (Shi et al. Citation2018).
Differences in affinity for ammonia are a major factor in determining the dominant ammonia oxidizers and their community composition (Prosser and Nicol Citation2012). All AOA had two- or three-fold higher affinity for NH3 than did AOB based on the Km values obtained from N. maritimus SCM1 (Martens-Habbena et al. Citation2009). Thus, AOA grow favorably under low ammonia conditions and becomes the dominant ammonia oxidizer in various soils. However, recently, important studies have been opposed these interpretations. Nitrosocosmicus, which was isolated from arable soil and was proposed as a new cluster, is adapted to high ammonia concentrations (Lehtovirta-Morley et al. Citation2016a; Sauder et al. Citation2017). AOA have a wide range of affinity for ammonia, and its affinity for ammonia is similar to that of AOB belonging to Nitrosomonas cluster 6a (Kits et al. Citation2017). On the other hand, the affinity for ammonia of the comammox Nitrospira inopinata was lower than that of marine AOA but higher than that of AOA isolated from non-marine environments (Kits et al. Citation2017). The affinity of ammonia oxidizers for ammonia is more diverse than previously considered. Further isolation of ammonia oxidizers and physiological studies are necessary to evaluate the niche separation of AOA, AOB, and comammox Nitrospira by ammonia concentration.
2.6.4. Temperature
Most of the pure cultured AOB strains isolated from soils have optimum growth at temperatures between 25 and 30°C (Jiang and Bakken Citation1999). Although enriched or pure cultured AOA from various environments have optimum growth at temperatures ranging from 25 to 74°C, Nitrosotalea devanaterra (Lehtovirta-Morley et al. Citation2011) and Nitrosocosmicus franklandus (Lehtovirta-Morley et al. Citation2016a) isolated from soil have optimal growth at 25°C.
The effect of temperature on the community structure and activity of AOA and AOB has been investigated in soil samples (Tourna et al. Citation2008; Taylor et al. Citation2017). The contribution of AOA to nitrification increases with increasing temperature, and the optimum temperature for AOA growth is higher than that for AOB growth (Ouyang, Norton, and Stark Citation2017). Szukics et al. (Citation2010) revealed that the abundance of AOB increased with increasing soil temperature and that the abundance of AOA decreased in a pristine forest soil (Szukics et al. Citation2010). Soil nitrification activities of eight soil samples from different sites were assessed across a temperature gradient ranging from 4 to 42°C, and the results showed that the optimal temperatures for AOA growth were 12°C greater than those for AOB growth (Taylor et al. Citation2017).
2.6.5. Moisture and aeration
Since oxygen is an essential substrate for energy production by the oxidation of ammonia or nitrite, oxygen concentration has a great influence on the ecology of nitrifying bacteria. Nitrifiers have a wide range of affinity for oxygen, and AOA have the highest affinity, followed by AOB and NOB. Km for oxygen of AOA Candidatus Nitrososphaera sp. JG1 and Candidatus Nitrosoarchaeum koreensis MY1 isolated from soils was 4.67 µM and 10.38 µM, respectively (Kim et al. Citation2012; Jung et al. Citation2011). The Km for oxygen of AOB Nitrosomonas oligotropha NL7 and Nitrosomonas europaea C-31 was 76.3 µM and 186 µM, respectively (Park and Noguera Citation2007; Park et al. Citation2010).
2.7. Microbial N2O emission
Nitrification-related pathways, bacterial denitrification, and fungal denitrification are major pathways that generate N2O in soil ecosystems. N2O is produced by nitrifiers mainly through two pathways: the oxidation of NH2OH by AOB and the abiotic reaction of nitric oxide (NO) (Lehtovirta-Morley Citation2018), namely nitrifier nitrification. Another pathway is nitrifier denitrification, in which NO2− is reduced to N2O by AOB (Wrage-Mönnig et al. Citation2018). In hydroxylamine oxidation, the N2O production potential of AOB is much higher than that of AOA and comammox at the culture experiments (Kits et al. Citation2019; Prosser et al. Citation2020). On the other hand, AOB can also produce N2O under low oxygen concentrations through nitrifier denitrification, the reduction of NO2− to N2O via NO, by the NO2− and NO reductases, a process that was thought to be restricted to AOB (Kozlowski, Kits, and Stein Citation2016; Wrage-Mönnig et al. Citation2018). AOA and comammox bacteria lack NO reductase genes (Daims et al. Citation2015; Kits et al. Citation2019; Kim et al. Citation2011; Spang et al. Citation2012; Abbas et al. Citation2019).
The relative contribution of AOA and AOB to N2O production has been investigated by quantification of amoA genes, transcripts, and/or specific inhibitors, such as the AOA-specific inhibitors, PTIO (Shen et al. 2013) and simvastatin (Zhao et al. Citation2020), and the AOB specific inhibitor, 1-octyne (Hink, Nicol, and Prosser Citation2017) to selectively inhibit and distinguish them. The relative contribution of AOA and AOB to N2O generation was evaluated in soils with different ammonia concentrations using microcosm experiments and selective inhibitors (Hink, Nicol, and Prosser Citation2017; Wang et al. Citation2016) indicating that AOB, not AOA, dominate in both N2O production and nitrification in soils amended with ammonium. AOB-dominated N2O production has also been reported in several soils, including the bioenergy crop soil (Meinhardt et al. Citation2018; Pannu et al. Citation2019), urea fertilized soil (Chen et al. Citation2020) and paddy soils (Fu et al. Citation2020). On the other hand, Giguere et al. (Citation2017) reported no significant differences in contribution of N2O production between AOA and AOB in non-cropped Oregon soils (Giguere et al. Citation2017). AOA-dominated N2O production has been observed depending on conditions such as urea levels. Duan et al. (Citation2019) indicated that the dominant contribution of AOA and AOB to N2O production was affected by the levels of urea application to the vegetable fields (Duan et al. Citation2019). Addition of urea at conventional or lower application levels induced higher AOB-dependent N2O production than AOA, while excess urea application led to higher AOA-dependent N2O production than AOB.
2.8. Control of nitrification
N2O emissions and nitrogen leaching can be prevented by controlling nitrification. Nitrogen in agricultural fields has been managed through several indirect and direct nitrification control methods, such as controlled-release N fertilizers, nitrification inhibitors, and urease inhibitors (Norton and Ouyang Citation2019). Nitrification inhibitors, which are the most effective nitrification control method, directly inhibit the conversion of ammonium to nitrite by AOB by suppressing AMO activity and reducing the risk of nitrate leaching and N2O emissions (Subbarao et al. Citation2006; Beeckman, Motte, and Beeckman Citation2018). Meta-analyses have reported that the application of nitrification inhibitors significantly reduces dissolved inorganic N leaching and N2O emission (Akiyama, Yan, and Yagi Citation2010; Qiao et al. Citation2015; Xia et al. Citation2017). The N2O mitigation effects of 2-chloro-6-(trichloromethyl) pyridine (nitrapyrin), dicyandiamide (DCD), and 3,4-dimethylepyrazole phosphate (DMPP), which are widely used in agriculture, were evaluated as 50%, 30%, and 50%, respectively (Akiyama, Yan, and Yagi Citation2010). On the other hand, the application of nitrification inhibitors with nitrogen fertilizer or animal urine could potentially increase NH3 emission in some soils (Qiao et al. Citation2015). DCD residues have been detected in New Zealand milk products due to DCD spray application in pastures (Pal, McMillan, and Saggar Citation2016). Nitrapyrin was detected in water samples from streams across Iowa (Woodward et al. Citation2019). Therefore, from the viewpoint of environmental pollution, nitrification inhibitors should be fully considered before its use in the agricultural field.
The efficacy of nitrification inhibitors can be influenced by various environmental factors and, thus, vary across soils (Subbarao et al. Citation2006). In the case of DMPP, DMPP-amended urea fertilizer had no effect on N2O emission of Australian commercial crops (Nauer et al. Citation2018), whereas DMPP reduced N2O from 37% to 75% in vegetable soils (Scheer et al. Citation2014; Lam et al. Citation2018). Four contrasting arable soils including gray desert soil, alluvial paddy soil, loess formed paddy soil, and red soil in China were studied to understand the effects of soil properties on the efficacy of DMPP (Fan et al. Citation2019). DMPP significantly inhibited nitrification and reduced N2O emissions, with an inhibitory rate ranging from 41.7% in red soil to 90.0% in gray desert soil. Reduction of N2O emissions by DMPP is correlated with AOB abundance, which is a major contributor to N2O emission rather than AOA. This study concluded that DMPP effectively inhibits nitrification by suppressing the abundance of AOB across soil types, and abiotic factors play a more important role than biotic factors in soil N2O emissions. The response of AOA, AOB, and comammox to nitrification inhibitors has been evaluated using pasture and arable soils (Li et al. Citation2020). Nitrapyrin, DMPP, DCD, and allylthiourea markedly inhibited AOB abundance in both soils. Furthermore, the three nitrification inhibitors, except for DMPP, effectively suppressed AOA abundances in both soils. Comammox bacteria respond differently to the four nitrification inhibitors in both soils. The authors speculated that the different responses may be due to the different soil properties that can influence the absorption and degradation of nitrogen inhibitors. Moreover, AOA, AOB, and comammox bacteria have different susceptibilities to DMPP, nitrapyrin, and DCD, as evaluated using stable isotope labeling methods (Zhou et al. Citation2020).
Most commercially available synthetic nitrification inhibitors target and inhibit AMO, which mediates the first step of ammonia oxidation. However, AMO has not been purified so far, and there is limited information on this enzyme, such as a three-dimensional structure, which is necessary for the development of more effective nitrification inhibitors. Nishigaya et al. (Citation2016) proposed the development of nitrification inhibitors targeting HAO (Nishigaya, Fujimoto, and Yamazaki Citation2016). The three-dimensional structure of HAO was determined based on structure-guided drug design using the information from 3D structures of the target molecule and the target-ligand complex for drug discovery. Alkyl- and aryl-hydrazine derivatives can irreversibly inactivate HAO by covalent modification at the active site of the enzyme (Logan and Hooper Citation1995). One of these derivatives, phenylhydrazine hydrochloride, effectively inhibits ammonia oxidization in soil slurry (Wu et al. Citation2012) and soil incubation experiments (Yang et al. Citation2017).
Biological nitrification inhibition (BNI) is defined as the ability of certain plant roots to produce and release nitrification inhibitors to suppress soil-nitrifier activity (Subbarao et al. Citation2015). Screening for BNI potential has been performed on various crops, and BNI ability was found for many crops such as rice, wheat, and forage grass (Sun et al. Citation2016; Nuñez et al. Citation2018; O’Sullivan et al. Citation2016). Recently, several plants, such as Megathyrsus maximus and Brachiaria hybrid cv., were shown to be able to suppress not only soil nitrification but also N2O emission (Byrnes et al. Citation2017; Villegas et al. Citation2020). BNI holds promise as a strategy to conserve N in nutrient-poor environments (Subbarao et al. Citation2013).
3. Anammox
Anammox, discovered by Mulder et al. (Mulder et al. Citation1995) and Van de Graaf et al. (Van de Graaf et al. Citation1996) is a microbial process that oxidizes NH4+ to N2 with NO2− as an electron acceptor. The anammox process consists of three steps: NO reduction from NO2−, hydrazine synthesis from NO and NH4+, and N2 gas dehydration from hydrazine (Jetten et al. Citation2009). Anammox has been detected in various environments, including marine, freshwater, and estuarine sediments, oxygen-depleted zones, wetland soils, and riparian and agricultural soil (Nie et al. Citation2019). The occurrence and activity of anammox bacteria have also been detected in paddy soils, and the anammox process has been reported to contribute ~37% to N2 production in soil (Zhu et al. Citation2011). Furthermore, the anammox process is an important nitrogen loss pathway in paddy fields.
3.1. Anammox bacteria
Strous et al. (Citation1999) obtained anammox bacterial suspensions (99.6% pure) physically separated from an enrichment culture through density-gradient centrifugation (Strous et al. Citation1999). The bacterium was responsible for anammox and CO2 assimilation by incubating cell suspensions with ammonium, nitrite, and CO2 under anaerobic conditions and was named Candidatus Brocadia anammoxidans and classified into the phylum Planctomycetes (Jetten et al. Citation2001; Strous et al. Citation1999). Although no pure cultures have been obtained, there are at least five candidate genera of anammox bacteria classified into the phylum Planctomycetes: Candidatus Brocadia, Candidatus Kuenenia, Candidatus Anammoxoglobus, Candidatus Jettenia and Candidatus Scalindua (Kuenen Citation2008). Candidatus Scalindua has been detected in marine environments and the other genera have mainly been sampled from freshwater activated sludge.
Essential functional genes for nitrogen transformation related to the anammox process are cytochrome cd1-type NO-forming nitrite reductase (nirS), copper-containing NO-forming nitrite reductase (nirK), hydrazine synthase (hzs), and hydrazine dehydrogenase/ hydroxylamine dehydrogenase (hdh/hao). Neither nirS nor nirK was found in Ca. Brocadia sinica and related genomes (Lawson et al. Citation2017; Oshiki et al. Citation2015; Speth et al. Citation2016; Zhao et al. Citation2019). The hzs genes (hazA, hzsB, and hzsC) are unique for anammox bacteria and, thus, have been used as specific biomarkers. The hzsA and hzsB genes have often been used for the quantification of the abundance of anammox bacteria in environmental samples.
3.2. Anammox in soil environment
The anammox bacteria are more diverse in soil than in seawater water columns, as evidenced by molecular biology techniques (Humbert et al. Citation2010; Nie et al. Citation2019; Sonthiphand, Hall, and Neufeld Citation2014). Brocadia was most commonly detected in soil, followed by Kuenenia (Nie et al. Citation2019). The community composition of anammox bacteria in soil is affected by soil depth, fertilization treatment, agricultural land use, and cultivation history (Nie et al. Citation2019). Candidatus Jettenia and Candidatus Brocadia have been enriched from a nitrogen-loaded peat soil (Hu et al. Citation2011), and Candidatus Anammoxoglobus and Candidatus Jettenia have been enriched from a flooded paddy soil (Hu et al. Citation2013a) with 40–50% of the enrichment cultures.
Anammox potential in soil samples has been detected using 15N tracer techniques. These techniques have been used to detect the anammox process in various environments by the addition of 15NH4+ and 14NOx− or 14NH4+ and 15NOx− to produce 15+14N2 (m/z 29) consisting of one N from NH4+ and the other N from NO2−, which can be distinguished from N2 from the denitrification process. To date, many studies have reported anammox potential in natural and agricultural soils. The anammox process was detected in an enrichment culture from a nitrogen-loaded peat soil (Hu et al. Citation2011) and was detected directly in paddy soil samples (Sato et al. Citation2012; Zhu et al. Citation2011).
Anammox activity is most frequently detected in paddy soils, which presents superior anammox in comparison with other soils (Nie et al. Citation2019). Anammox activity is higher in oxic-anoxic interfaces in wetlands loaded with nitrogen fertilizer, suggesting the occurrence of coupled nitrification-anammox-denitrification (Nie et al. Citation2019). Anammox activity has also been detected in brackish natural environments such as wetland sediments (Zhao et al. Citation2021) and mangrove sediments (Amano et al. Citation2011; Li et al. Citation2018a). The anammox activity and hazA abundance of rice rhizosphere in paddy soil were higher than those in bulk soil (Nie et al. Citation2015). Thus, the rhizosphere in paddy soil could provide the environment to enable the occurrence of anammox activity.
Anammox potential was also detected in agricultural soil, such as vegetable soil (Long et al. Citation2013; Shen et al. Citation2015, Citation2017). Ammonium concentration in soil correlates with the abundance and activity of anammox bacteria (Shen et al. Citation2013a, Shen et al. Citation2015, Citation2017). On the other hand, there is also a report that nitrate concentration is negatively correlated with anammox abundance (Qin et al. Citation2020). Further studies are needed to elucidate the relationship between N content in arable soil and the abundance and activity of anammox bacteria. Anammox activity was also detected in temperate upland forest soil, and the contribution of anammox activity to total N2 production was 1–7% in mixed forest (Xi et al. Citation2016). Total soil C and N, NO3− concentration and soil water content were positively correlated with the N2 production rates by anammox plus codenitrification (Xi et al. Citation2016). Upland forests may contribute less to N2 production by anammox than do N-loaded farmland soils. In acidic red soil, anammox rate was correlated with NO3−, and hzsB and anammox bacterial 16S rRNA gene abundances were correlated with total nitrogen (Wu et al. Citation2018). The contribution of the anammox process to N2 production ranged from less than 1% (Bai et al. Citation2015) to 53% (Wu et al. Citation2018) in natural and agricultural soil samples. Because anammox occurs under anaerobic conditions, anammox tends to be more active in wet soil where oxygen is scarce. Anammox activity is often correlated with the concentration of ammonia or nitrate in the soil, indicating that the supply of inorganic nitrogen has a great influence on anammox (Abbas et al. Citation2020).
4. Conclusions
In the last 15 years, there has been several breakthroughs regarding nitrifying microorganisms. For more than 100 years, nitrification has been thought to be performed only by AOB and NOB. The discovery of AOA and comammox overturned this theory, and these nitrifying microorganisms have been identified in various environments such as oceans and soils and have been shown to play an important role in the nitrogen cycle. On the other hand, the classical nitrifying microorganisms have been found to be more diverse, as shown by the classification of novel γ-AOB and NOBs into various phyla. In this review, we have outlined the diversity and functional characteristics of these novel and classic nitrifying microorganisms and presented published studies on the relationship between these nitrifying microorganisms and environmental factors in the soil. Genome and metagenome analyses have provided important and remarkable insights into the ecology and function of nitrifying microorganisms. However, more researches considering physiology, biochemistry, and enzymology of nitrifying microorganisms are needed for the development of technologies to control these organisms in different environments.
Disclosure statement
No potential conflict of interest was reported by the author(s).
Additional information
Funding
References
- Abbas, T., H. Zhou, Q. Zhang, Y. Li, Y. Liang, H. Di, and Y. Zhao. 2019. “Anammox Co-fungi Accompanying Denitrifying Bacteria are the Thieves of the Nitrogen Cycle in Paddy-wheat Crop Rotated Soils.” Environment International 130: 104913. doi:10.1016/j.envint.2019.104913.
- Abbas, T., Q. Zhang, X. Zou, M. Tahir, D. Wu, S. Jin, and H. Di. 2020. “Soil Anammox and Denitrification Processes Connected with N Cycling Genes Co-supporting or Contrasting under Different Water Conditions.” Environment International 140: 105757. doi:10.1016/j.envint.2020.105757.
- Ai, C., G. Liang, J. Sun, X. Wang, P. He, and W. Zhou. 2013. “Different Roles of Rhizosphere Effect and Long-term Fertilization in the Activity and Community Structure of Ammonia Oxidizers in a Calcareous Fluvo-aquic Soil.” Soil Biology & Biochemistry 57: 30–42. doi:10.1016/j.soilbio.2012.08.003.
- Aigle, A., J. I. Prosser, and C. Gubry-Rangin. 2019. “The Application of High-throughput Sequencing Technology to Analysis of amoA Phylogeny and Environmental Niche Specialisation of Terrestrial Bacterial Ammonia-oxidisers.” Environmental Microbiome 14 (1): 3. doi:10.1186/s40793-019-0342-6.
- Akiyama, H., X. Yan, and K. Yagi. 2010. “Evaluation of Effectiveness of Enhanced‐efficiency Fertilizers as Mitigation Options for N2O and NO Emissions from Agricultural Soils: Meta-analysis.” Global Change Biology 16 (6): 1837–1846. doi:10.1111/j.1365-2486.2009.02031.x.
- Allison, S. M., and J. I. Prosser. 1991. “Urease Activity in Neutrophilic Autotrophic Ammonia-oxidizing Bacteria Isolated from Acid Soils.” Soil Biology & Biochemistry 23 (1): 45–51. doi:10.1016/0038-0717(91)90161-C.
- Alves, R. J. E., B. Q. Minh, T. Urich, A. von Haeseler, and C. Schleper. 2018. “Unifying the Global Phylogeny and Environmental Distribution of Ammonia-oxidising Archaea Based on amoA Genes.” Nature Communications 9 (1): 1–17. doi:10.1038/s41467-018-03861-1.
- Amano, T., I. Yoshinaga, T. Yamagishi, C. Van Thuoc, S. Ueda, K. Kato, Y. Sako, and Y. Suwa. 2011. “Contribution of Anammox Bacteria to Benthic Nitrogen Cycling in a Mangrove Forest and Shrimp Ponds, Haiphong, Vietnam.” Microbes and Environments 26: 1–6. doi:10.1264/jsme2.ME10150.
- Attard, E., F. Poly, C. Commeaux, F. Laurent, A. Terada, B. F. Smets, S. Recous, and X. Le Roux. 2010. “Shifts between Nitrospira‐and Nitrobacter‐like Nitrite Oxidizers Underlie the Response of Soil Potential Nitrite Oxidation to Changes in Tillage Practices.” Environmental Microbiology 12 (2): 315–326. doi:10.1111/j.1462-2920.2009.02070.x.
- Avrahami, S., and R. Conrad. 2003. “Patterns of Community Change among Ammonia Oxidizers in Meadow Soils upon Long-term Incubation at Different Temperatures.” Applied and Environmental Microbiology 69 (10): 6152–6164. doi:10.1128/AEM.69.10.6152-6164.2003.
- Bai, R., D. Xi, J. Z. He, H. W. Hu, Y. T. Fang, and L. M. Zhang. 2015. “Activity, Abundance and Community Structure of Anammox Bacteria along Depth Profiles in Three Different Paddy Soils.” Soil Biology & Biochemistry 91: 212–221. doi:10.1016/j.soilbio.2015.08.040.
- Beeckman, F., H. Motte, and T. Beeckman. 2018. “Nitrification in Agricultural Soils: Impact, Actors and Mitigation.” Current Opinion in Biotechnology 50: 166–173. doi:10.1016/j.copbio.2018.01.014.
- Booth, M. S., J. M. Stark, and E. Rastetter. 2005. “Controls on Nitrogen Cycling in Terrestrial Ecosystems: A Synthetic Analysis of Literature Data.” Ecological Monographs 75 (2): 139–157. doi:10.1890/04-0988.
- Byrnes, R. C., J. Nùñez, L. Arenas, I. Rao, C. Trujillo, C. Alvarez, J. Arango, F. Rasche, and N. Chirinda. 2017. “Biological Nitrification Inhibition by Brachiaria Grasses Mitigates Soil Nitrous Oxide Emissions from Bovine Urine Patches.” Soil Biology & Biochemistry 107: 156–163. doi:10.1016/j.soilbio.2016.12.029.
- Campbell, M. A., P. S. G. Chain, H. Dang, A. F. El Sheikh, J. M. Norton, N. L. Ward, B. B. Ward, and M. G. Klotz. 2011. “Nitrosococcus Watsonii Sp. Nov., A New Species of Marine Obligate Ammonia-oxidizing Bacteria that Is Not Omnipresent in the World’s Oceans: Calls to Validate the Names ‘Nitrosococcus Halophilus’ and ‘Nitrosomonas Mobilis.’” FEMS Microbiology Ecology 76 (1): 39–48. doi:10.1111/j.1574-6941.2010.01027.x.
- Caranto, J. D., A. C. Vilbert, and K. M. Lancaster. 2016. “Nitrosomonas Europaea Cytochrome P460 Is a Direct Link between Nitrification and Nitrous Oxide Emission.” Proceedings of the National Academy of Sciences 113 (51): 14704–14709. doi:10.1073/pnas.1611051113.
- Caranto, J. D., and K. M. Lancaster. 2017. “Nitric Oxide Is an Obligate Bacterial Nitrification Intermediate Produced by Hydroxylamine Oxidoreductase.” Proceedings of the National Academy of Sciences 114 (31): 8217–8222. doi:10.1073/pnas.1704504114.
- Chen, Z., Q. Wang, J. Zhao, Y. Chen, H. Wang, J. Ma, P. Zou, and L. Bao. 2020. “Restricted Nitrous Oxide Emissions by Ammonia Oxidizers in Two Agricultural Soils following Excessive Urea Fertilization.” Journal of Soils and Sediments 20 (3): 1502–1512. doi:10.1007/s11368-019-02479-0.
- Clark, D. R., B. A. McKew, L. F. Dong, G. Leung, A. J. Dumbrell, A. Stott, H. Grant, D. B. Nedwell, M. Trimmer, and C. Whitby. 2020. “Mineralization and Nitrification: Archaea Dominate Ammonia-oxidising Communities in Grassland Soils.” Soil Biology & Biochemistry 143: 107725. doi:10.1016/j.soilbio.2020.107725.
- Costa, E., J. Pérez, and J.-U. Kreft. 2006. “Why Is Metabolic Labour Divided in Nitrification?” Trends in Microbiology 14 (5): 213–219. doi:10.1016/j.tim.2006.03.006.
- Daims, H., E. V. Lebedeva, P. Pjevac, P. Han, C. Herbold, M. Albertsen, N. Jehmlich, M. Palatinszky, J. Vierheilig, and A. Bulaev. 2015. “Complete Nitrification by Nitrospira Bacteria.” Nature 528 (7583): 504–509. doi:10.1038/nature16461.
- Daims, Damis, H., S. Lücker, D. L. Paslier, and M. Wagner. 2011. “Diversity, Environmental Genomics, and Ecophysiology of Nitrite‐oxidizing Bacteria.” In Nitrification, edited by B. B. Ward, D. J. Arp and M. G. Klotz. Washinton DC: ASM Press.
- Daims, H., S. Lücker, and M. Wagner. 2016. “A New Perspective on Microbes Formerly Known as Nitrite-oxidizing Bacteria.” Trends in Microbiology 24 (9): 699–712. doi:10.1016/j.tim.2016.05.004.
- De Boer, W., and G. A. Kowalchuk. 2001. “Nitrification in Acid Soils: Micro-organisms and Mechanisms.” Soil Biology & Biochemistry 33 (7–8): 853–866. doi:10.1016/S0038-0717(00)00247-9.
- De Boer, W., P. A. K. Gunnewiek, and H. J. Laanbroek. 1995. “Ammonium-oxidation at Low pH by a Chemolithotrophic Bacterium Belonging to the Genus Nitrosospira.” Soil Biology & Biochemistry 27 (2): 127–132. doi:10.1016/0038-0717(94)00157-V.
- Duan, P., C. Fan, Q. Zhang, and Z. Xiong. 2019. “Overdose Fertilization Induced Ammonia-oxidizing Archaea Producing Nitrous Oxide in Intensive Vegetable Fields.” Science of the Total Environment 650: 1787–1794. doi:10.1016/j.scitotenv.2018.09.341.
- Fan, X., C. Yin, H. Chen, M. Ye, Y. Zhao, T. Li, S. A. Wakelin, and Y. Liang. 2019. “The Efficacy of 3, 4-dimethylpyrazole Phosphate on N2O Emissions Is Linked to Niche Differentiation of Ammonia Oxidizing Archaea and Bacteria across Four Arable Soils.” Soil Biology & Biochemistry 130: 82–93. doi:10.1016/j.soilbio.2018.11.027.
- Fierer, N., K. M. Carney, M. C. Horner-Devine, and J. P. Megonigal. 2009. “The Biogeography of Ammonia-oxidizing Bacterial Communities in Soil.” Microbial Ecology 58 (2): 435–445. doi:10.1128/AEM.01324-10.
- Fowler, D., M. Coyle, U. Skiba, M. A. Sutton, J. N. Cape, S. Reis, L. J. Sheppard, et al. 2013. “The Global Nitrogen Cycle in the Twenty-first Century.” Philosophical Transactions of the Royal Society B: Biological Sciences 368 (1621): 20130164. doi:10.1098/rstb.2013.0164.
- Fu, Q., R. Xi, J. Zhu, H. W. Hu, Z. Xing, and J. Zuo. 2020. “The Relative Contribution of Ammonia Oxidizing Bacteria and Archaea to N2O Emission from Two Paddy Soils with Different Fertilizer N Sources: A Microcosm Study.” Geoderma 375: 114486. doi:10.1016/j.geoderma.2020.114486.
- Galloway, J. N., A. R. Townsend, J. W. Erisman, M. Bekunda, Z. Cai, J. R. Freney, L. A. Martinelli, S. P. Seitzinger, and M. A. Sutton. 2008. “Transformation of the Nitrogen Cycle: Recent Trends, Questions, and Potential Solutions.” Science 320 (5878): 889–892. doi:10.1126/science.1136674.
- Giguere, A. T., A. E. Taylor, D. D. Myrold, and P. J. Bottomley. 2015. “Nitrification Responses of Roil Ammonia‐oxidizing Archaea and Bacteria to Ammonium Concentrations.” Soil Science Society of America Journal 79 (5): 1366–1374. doi:10.2136/sssaj2015.03.0107.
- Giguere, A. T., A. E. Taylor, Y. Suwa, D. D. Myrold, and P. J. Bottomley. 2017. “Uncoupling of Ammonia Oxidation from Nitrite Oxidation: Impact upon Nitrous Oxide Production in Non-cropped Oregon Soils.” Soil Biology & Biochemistry 104: 30–38. doi:10.1016/j.soilbio.2016.10.011.
- Gubry-Rangin, C., B. Hai, C. Quince, M. Engel, B. C. Thomson, P. James, M. Schloter, R. I. Griffiths, J. I. Prosser, and G. W. Nicol. 2011. “Niche Specialization of Terrestrial Archaeal Ammonia Oxidizers.” Proceedings of the National Academy of Sciences 108 (52): 21206–21211. doi:10.1073/pnas.1109000108.
- Han, S., L. Zeng, X. Luo, X. Xiong, S. Wen, B. Wang, W. Chen, and Q. Huang. 2018. “Shifts in Nitrobacter-and Nitrospira-like Nitrite-oxidizing Bacterial Communities under Long-term Fertilization Practices.” Soil Biology & Biochemistry 124: 118–125. doi:10.1016/j.soilbio.2018.05.033.
- Hayatsu, M., K. Tago, I. Uchiyama, A. Toyoda, Y. Wang, Y. Shimomura, T. Okubo, F. Kurisu, Y. Hirono, and K. Nonaka. 2017. “An Acid-tolerant Ammonia-oxidizing γ-proteobacterium from Soil.” The ISME Journal 11 (5): 1130–1141. doi:10.1038/ismej.2016.191.
- He, J. Z., J. P. Shen, L. M. Zhang, and H. J. Di. 2012. “A Review of Ammonia-oxidizing Bacteria and Archaea in Chinese Soils.” Frontiers in Microbiology 3: 296. doi:10.3389/fmicb.2012.00296.
- He, L., Y. Bi, J. Zhao, C. M. Pittelkow, X. Zhao, S. Wang, and G. Xing. 2018. “Population and Community Structure Shifts of Ammonia Oxidizers after Four-year Successive Biochar Application to Agricultural Acidic and Alkaline Soils.” Science of the Total Environment 619: 1105–1115. doi:10.1016/j.scitotenv.2017.11.029.
- He, Z. Y., J. P. Shen, L. M. Zhang, H. J. Tian, B. Han, H. J. Di, and J. Z. He. 2020. “DNA Stable Isotope Probing Revealed No Incorporation of 13CO2 into Comammox Nitrospira but Ammonia-oxidizing Archaea in a Subtropical Acid Soil.” Journal of Soils and Sediments 20 (3): 1297–1308. doi:10.1007/s11368-019-02540-y.
- Head, I. M., W. D. Hiorns, T. M. Embley, A. L. J. McCarthy, and J. R. Saunders. 1993. “The Phylogeny of Autotrophic Ammonia-oxidizing Bacteria as Determined by Analysis of 16S Ribosomal RNA Gene Sequences.” Journal of General Microbiology 139 (6): 1147–1153. doi:10.1099/00221287-139-6-1147.
- Hink, L., C. Gubry-Rangin, G. W. Nicol, and J. I. Prosser. 2018. “The Consequences of Niche and Physiological Differentiation of Archaeal and Bacterial Ammonia Oxidisers for Nitrous Oxide Emissions.” The ISME Journal 12 (4): 1084–1093. doi:10.1038/s41396-017-0025-5.
- Hink, L., G. W. Nicol, and J. I. Prosser. 2017. “Archaea Produce Lower Yields of N2O than Bacteria during Aerobic Ammonia Oxidation in Soil.” Environmental Microbiology 19 (12): 4829–4837. doi:10.1111/1462-2920.13282.
- Hink, L., P. Lycus, C. Gubry-Rangin, Å. Frostegård, G. W. Nicol, J. I. Prosser, and L. R. Bakken. 2017. “Kinetics of NH3‐oxidation, NO‐turnover, N2O‐production and Electron Flow during Oxygen Depletion in Model Bacterial and Archaeal Ammonia Oxidisers.” Environmental Microbiology 19 (12): 4882–4896. doi:10.1111/1462-2920.13914.
- Hu, B. L., D. Rush, E. van der Biezen, P. Zheng, M. Van Mullekom, S. Schouten, J. S. S. Damste, A. J. Smolders, M. S. Jetten, and B. Kartal. 2011. “New Anaerobic, Ammonium-oxidizing Community Enriched from Peat Soil.” Applied and Environmental Microbiology 77 (3): 966–971. doi:10.1128/AEM.02402-10.
- Hu, B. L., L. D. Shen, S. Liu, C. Cai, T. T. Chen, B. Kartal, H. R. Harhangi, et al. 2013a. “Enrichment of an Anammox Bacterial Community from a Flooded Paddy Soil.” Environmental Microbiology Reports 5 (3): 483–489. doi:10.1111/1758-2229.12038.
- Hu, H. W., L. M. Zhang, Y. Dai, H. J. Di, and J. Z. He. 2013b. “pH-dependent Distribution of Soil Ammonia Oxidizers across a Large Geographical Scale as Revealed by High-throughput Pyrosequencing.” Journal of Soils and Sediments 13 (8): 1439–1449. doi:10.1007/s11368-013-0726-y.
- Hu, H. W., Z. H. Xu, and J. Z. He. 2014. “Ammonia-oxidizing Archaea Play a Predominant Role in Acid Soil Nitrification.” In Advances in Agronomy, edited by Sparks D. L., 261-302. Amsterdam: Elsevier.
- Humbert, S., S. Tarnawski, N. Fromin, M. P. Mallet, M. Aragno, and J. Zopfi. 2010. “Molecular Detection of Anammox Bacteria in Terrestrial Ecosystems: Distribution and Diversity.” The ISME Journal 4 (3): 450–454. doi:10.1038/ismej.2009.125.
- Huygens, D., P. Boeckx, P. Templer, L. Paulino, O. Van Cleemput, C. Oyarzún, C. Müller, and R. Godoy. 2008. “Mechanisms for Retention of Bioavailable Nitrogen in Volcanic Rainforest Soils.” Nature Geoscience 1 (8): 543–548. doi:10.1038/ngeo252.
- Ishii, K., H. Fujitani, K. Soh, T. Nakagawa, R. Takahashi, and S. Tsuneda. 2017. “Enrichment and Physiological Characterization of a Cold-adapted Nitrite-oxidizing Nitrotoga Sp. From an Eelgrass Sediment.” Applied ƒand Environmental Microbiology 83 (14). doi:10.1128/AEM.00549-17.
- Ishii, K., H. Fujitani, Y. Sekiguchi, and S. Tsuneda. 2020. “Physiological and Genomic Characterization of a New ‘Candidatus Nitrotoga’isolate.” Environmental Microbiology 22 (6): 2365–2382. doi:10.1111/1462-2920.15015.
- Islam, A., D. Chen, and R. E. White. 2007. “Heterotrophic and Autotrophic Nitrification in Two Acid Pasture Soils.” Soil Biology & Biochemistry 39 (4): 972–975. doi:10.1016/j.soilbio.2006.11.003.
- Jetten, M. S. M., L. van Niftrik, M. Strous, B. Kartal, J. T. Keltjens, and H. J. M. Op den Camp. 2009. “Biochemistry and Molecular Biology of Anammox Bacteria.” Critical Reviews in Biochemistry and Molecular Biology 44 (2–3): 65–84. doi:10.1080/10409230902722783.
- Jetten, M. S. M., M. Wagner, J. Fuerst, M. C. van Loosdrecht, G. Kuenen, and M. Strous. 2001. “Microbiology and Application of the Anaerobic Ammonium Oxidation (‘anammox’) Process.” Current Opinion in Biotechnology 12 (3): 283–288. doi:10.1016/S0958-1669(00)00211-1.
- Jiang, H., L. Huang, Y. Deng, S. Wang, Y. Zhou, L. Liu, and H. Dong. 2014. “Latitudinal Distribution of Ammonia-oxidizing Bacteria and Archaea in the Agricultural Soils of Eastern China.” Applied and Environmental Microbiology 80 (18): 5593–5602. doi:10.1128/AEM.01617-14.
- Jiang, Q. Q., and L. R. Bakken. 1999. “Comparison of Nitrosospira Strains Isolated from Terrestrial Environments.” FEMS Microbiology Ecology 30 (2): 171–186. doi:10.1111/j.1574-6941.1999.tb00646.x.
- Jung, M. Y., R. Well, D. Min, A. Giesemann, S. J. Park, J. G. Kim, S. J. Kim, and S. K. Rhee. 2014. “Isotopic Signatures of N2O Produced by Ammonia-oxidizing Archaea from Soils.” The ISME Journal 8 (5): 1115–1125. doi:10.1038/ismej.2013.205.
- Jung, M. Y., S. J. Park, D. Min, J. S. Kim, W. I. C. Rijpstra, J. S. S. Damsté, G. J. Kim, E. Madsen, and S. K. Rhee. 2011. “Enrichment and Characterization of an Autotrophic Ammonia-oxidizing Archaeon of Mesophilic Crenarchaeal Group I. 1a from an Agricultural Soil.” Applied and Environmental Microbiology 77 (24): 8635–8647. doi:10.1128/AEM.05787-11.
- Jung, M.-Y., J.-H. Gwak, L. Rohe, A. Giesemann, J.-G. Kim, R. Well, E. L. Madsen, C. W. Herbold, M. Wagner, and S.-K. Rhee. 2019. “Indications for Enzymatic Denitrification to N2O at Low pH in an Ammonia-oxidizing Archaeon.” The ISME Journal 13 (10): 2633–2638. doi:10.1038/s41396-019-0460-6.
- Ke, X., R. Angel, Y. Lu, and R. Conrad. 2013. “Niche Differentiation of Ammonia Oxidizers and Nitrite Oxidizers in Rice Paddy Soil.” Environmental Microbiology 15 (8): 2275–2292. doi:10.1111/1462-2920.12098.
- Killham, K. 1990. “Nitrification in Coniferous Forest Soils.” Plant and Soil 128 (1): 31–44. doi:10.1007/BF00009394.
- Kim, B. K., M. Y. Jung, D. S. Yu, S. J. Park, T. K. Oh, S. K. Rhee, and J. F. Kim. 2011. “Genome Sequence of an Ammonia-oxidizing Soil archaeon,“Candidatus Nitrosoarchaeum Koreensis” MY1.” Journal of Bacteriology 193 (19): 5539–5540. doi:10.1128/JB.05717-11.
- Kim, J. G., M. Y. Jung, S. J. Park, W. I. C. Rijpstra, J. S. Sinninghe Damsté, E. L. Madsen, D. Min, J. S. Kim, G. J. Kim, and S. K. Rhee. 2012. “Cultivation of a Highly Enriched Ammonia‐oxidizing Archaeon of Thaumarchaeotal Group I. 1b from an Agricultural Soil.” Environmental Microbiology 14 (6): 1528–1543. doi:10.1111/j.1462-2920.2012.02740.x.
- Kits, K. D., C. J. Sedlacek, E. V. Lebedeva, P. Han, A. Bulaev, P. Pjevac, A. Daebeler, et al. 2017. “Kinetic Analysis of a Complete Nitrifier Reveals an Oligotrophic Lifestyle.” Nature 549 (7671): 269–272. doi:10.1038/nature23679.
- Kits, K. D., M. Y. Jung, J. Vierheilig, P. Pjevac, C. J. Sedlacek, S. Liu, C. Herbold, et al. 2019. “Low Yield and Abiotic Origin of N2O Formed by the Complete Nitrifier Nitrospira Inopinata.” Nature Communications 10 (1): 1–12. doi:10.1038/s41467-019-09790-x.
- Kitzinger, K., H. Koch, S. Lücker, C. J. Sedlacek, C. Herbold, J. Schwarz, A. Daebeler, A. J. Mueller, M. Lukumbuzya, and S. Romano. 2018. “Characterization of the First “Candidatus Nitrotoga” Isolate Reveals Metabolic Versatility and Separate Evolution of Widespread Nitrite-oxidizing Bacteria.” MBio 9 (4): e01186–18. doi:10.1128/mBio.01186-18.
- Könneke, M., A. E. Bernhard, R. José, C. B. Walker, J. B. Waterbury, and D. A. Stahl. 2005. “Isolation of an Autotrophic Ammonia-oxidizing Marine Archaeon.” Nature 437 (7058): 543–546. doi:10.1038/nature03911.
- Kowalchuk, G. A., and J. R. Stephen. 2001. “Ammonia-oxidizing Bacteria: A Model for Molecular Microbial Ecology.” Annual Reviews in Microbiology 55 (1): 485–529. doi:10.1146/annurev.micro.55.1.485.
- Kozlowski, J. A., J. Price, and L. Y. Stein. 2014. “Revision of N2O-producing Pathways in the Ammonia-oxidizing Bacterium Nitrosomonas Europaea ATCC 19718.” Applied and Environmental Microbiology 80 (16): 4930–4935. doi:10.1128/AEM.01061-14.
- Kozlowski, J. A., K. D. Kits, and L. Y. Stein. 2016. “Comparison of Nitrogen Oxide Metabolism among Diverse Ammonia-oxidizing Bacteria.” Frontiers in Microbiology 7: 1090. doi:10.3389/fmicb.2016.01090.
- Kozlowski, J. A., M. Stieglmeier, C. Schleper, M. G. Klotz, and L. Y. Stein. 2016. “Pathways and Key Intermediates Required for Obligate Aerobic Ammonia-dependent Chemolithotrophy in Bacteria and Thaumarchaeota.” The ISME Journal 10 (8): 1836–1845. doi:10.1038/ismej.2016.2.
- Kuenen, J. G. 2008. “Anammox Bacteria: From Discovery to Application.” Nature Reviews. Microbiology 6 (4): 320–326. doi:10.1038/nrmicro1857.
- Lam, S. K., H. Suter, R. Davies, M. Bai, A. R. Mosier, J. Sun, and D. Chen. 2018. “Direct and Indirect Greenhouse Gas Emissions from Two Intensive Vegetable Farms Applied with a Nitrification Inhibitor.” Soil Biology & Biochemistry 116: 48–51. doi:10.1016/j.soilbio.2017.10.008.
- Lan, T., R. Liu, H. Suter, O. Deng, X. Gao, L. Luo, Yuan, C. Wang, and D. Chen. 2020. “Stimulation of Heterotrophic Nitrification and N2O Production, Inhibition of Autotrophic Nitrification in Soil by Adding Readily Degradable Carbon.” Journal of Soils and Sediments 20 (1): 81–90. doi:10.1007/s11368-019-02417-0.
- Lawson, C. E., and S. Lücker. 2018. “Complete Ammonia Oxidation: An Important Control on Nitrification in Engineered Ecosystems?” Current Opinion in Biotechnology 50: 158–165. doi:10.1016/j.copbio.2018.01.015.
- Lawson, C. E., S. Wu, A. S. Bhattacharjee, J. J. Hamilton, K. D. McMahon, R. Goel, and D. R. Noguera. 2017. “Metabolic Network Analysis Reveals Microbial Community Interactions in Anammox Granules.” Nature Communications 8: 15416. doi:10.1038/ncomms15416.
- Lebedeva, E. V., S. Off, S. Zumbrägel, M. Kruse, A. Shagzhina, S. Lücker, F. Maixner, A. Lipski, H. Daims, and E. Spieck. 2011. “Isolation and Characterization of a Moderately Thermophilic Nitrite-oxidizing Bacterium from a Geothermal Spring.” FEMS Microbiology Ecology 75 (2): 195–204. doi:10.1111/j.1574-6941.2010.01006.x.
- Lehtovirta-Morley, L. E. 2018. “Ammonia Oxidation: Ecology, Physiology, Biochemistry and Why They Must All Come Together.” FEMS Microbiology Letters 365 (9): fny058. doi:10.1093/femsle/fny058.
- Lehtovirta-Morley, L. E., C. Ge, J. Ross, H. Yao, G. W. Nicol, and J. I. Prosser. 2014. “Characterisation of Terrestrial Acidophilic Archaeal Ammonia Oxidisers and Their Inhibition and Stimulation by Organic Compounds.” FEMS Microbiology Ecology 89 (3): 542–552. doi:10.1111/1574-6941.12353.
- Lehtovirta-Morley, L. E., J. Ross, L. Hink, E. B. Weber, C. Gubry-Rangin, C. Thion, J. I. Prosser, and G. W. Nicol. 2016a. “Isolation of ‘Candidatus Nitrosocosmicus Franklandus,’ a Novel Ureolytic Soil Archaeal Ammonia Oxidiser with Tolerance to High Ammonia Concentration.” FEMS Microbiology Ecology 92 (5): fiw057. doi:10.1093/femsec/fiw057.
- Lehtovirta-Morley, L. E., K. Stoecker, A. Vilcinskas, J. I. Prosser, and G. W. Nicol. 2011. “Cultivation of an Obligate Acidophilic Ammonia Oxidizer from a Nitrifying Acid Soil.” Proceedings of the National Academy of Sciences 108 (38): 15892–15897. doi:10.1073/pnas.1107196108.
- Lehtovirta-Morley, L. E., L. A. Sayavedra-Soto, N. Gallois, S. Schouten, L. Y. Stein, J. I. Prosser, and G. W. Nicol. 2016b. “Identifying Potential Mechanisms Enabling Acidophily in the Ammonia-oxidizing Archaeon ‘Candidatus Nitrosotalea Devanaterra.’” Applied and Environmental Microbiology 82 (9): 2608–2619. doi:10.1128/AEM.04031-15.
- Leininger, S., T. Urich, M. Schloter, L. Schwark, J. Qi, G. W. Nicol, J. I. Prosser, S. C. Schuster, and C. Schleper. 2006. “Archaea Predominate among Ammonia-oxidizing Prokaryotes in Soils.” Nature 442 (7104): 806–809. doi:10.1038/nature04983.
- Levičnik-Höfferle, Š., G. W. Nicol, L. Ausec, I. Mandić-Mulec, and J. I. Prosser. 2012. “Stimulation of Thaumarchaeal Ammonia Oxidation by Ammonia Derived from Organic Nitrogen but Not Added Inorganic Nitrogen.” FEMS Microbiology Ecology 80 (1): 114–123. doi:10.1111/j.1574-6941.2011.01275.x.
- Li, C., H. W. Hu, Q. L. Chen, D. Chen, and J. Z. He. 2019a. “Comammox Nitrospira Play an Active Role in Nitrification of Agricultural Soils Amended with Nitrogen Fertilizers.” Soil Biology & Biochemistry 138: 107609. doi:10.1016/j.soilbio.2019.107609.
- Li, C., H. W. Hu, Q. L. Chen, D. Chen, and J. Z. He. 2020. “Growth of Comammox Nitrospira Is Inhibited by Nitrification Inhibitors in Agricultural Soils.” Journal of Soils and Sediments 20 (2): 621–628. doi:10.1007/s11368-019-02442-z.
- Li, P., S. Li, Y. Zhang, H. X. Cheng, H. Zhou, L. Qiu, and X. Diao. 2018a. “Seasonal Variation of Anaerobic Ammonium Oxidizing Bacterial Community and Abundance in Tropical Mangrove Wetland Sediments with Depth.” Applied Soil Ecology 130: 149–158. doi:10.1016/j.apsoil.2018.06.009.
- Li, Y., R. Xi, W. Wang, and H. Yao. 2019b. “The Relative Contribution of Nitrifiers to Autotrophic Nitrification across a pH-gradient in a Vegetable Cropped Soil.” Journal of Soils and Sediments 19 (3): 1416–1426. doi:10.1007/s11368-018-2109-x.
- Li, Y., S. J. Chapman, G. W. Nicol, and H. Yao. 2018b. “Nitrification and Nitrifiers in Acidic Soils.” Soil Biology & Biochemistry 116: 290–301. doi:10.1016/j.soilbio.2017.10.023.
- Lin, Y., G. Ye, J. Luo, H. J. Di, D. Liu, J. Fan, and W. Ding. 2018. “Nitrosospira Cluster 8a Plays a Predominant Role in the Nitrification Process of a Subtropical Ultisol under Long-term Inorganic and Organic Fertilization.” Applied and Environmental Microbiology 84 (18): e01031–18. doi:10.1128/AEM.01031-18.
- Liu, H., H. Pan, H. Hu, Z. Jia, Q. Zhang, Y. Liu, J. Xu, H. Di, and Y. Li. 2019a. “Autotrophic Archaeal Nitrification Is Preferentially Stimulated by Rice Callus Mineralization in a Paddy Soil.” Plant and Soil 445 (1–2): 55–69. doi:10.1007/s11104-019-04164-0.
- Liu, S., P. Han, L. Hink, J. I. Prosser, M. Wagner, and N. Bruggemann. 2017. “Abiotic Conversion of Extracellular NH2OH Contributes to N2O Emission during Ammonia Oxidation.” Environmental Science & Technology 51 (22): 13122–13132. doi:10.1021/acs.est.7b02360.
- Liu, T., Z. Wang, S. Wang, Y. Zhao, A. L. Wright, and X. Jiang. 2019b. “Responses of Ammonia-oxidizers and Comammox to Different Long-term Fertilization Regimes in a Subtropical Paddy Soil.” European Journal of Soil Biology 93: 103087. doi:10.1016/j.ejsobi.2019.103087.
- Logan, M. S. P., and A. B. Hooper. 1995. “Suicide Inactivation of Hydroxylamine Oxidoreductase of Nitrosomonas Europaea by Organohydrazines.” Biochemistry 34 (28): 9257–9264. doi:10.1021/bi00028a039.
- Long, A., J. Heitman, C. Tobias, R. Philips, and B. Song. 2013. “Co-occurring Anammox, Denitrification, and Codenitrification in Agricultural Soils.” Applied and Environmental Microbiology 79 (1): 168–176. doi:10.1128/AEM.02520-12.
- Long, X., C. Chen, Z. Xu, R. Oren, and J. Z. He. 2012. “Abundance and Community Structure of Ammonia-oxidizing Bacteria and Archaea in a Temperate Forest Ecosystem under Ten-years Elevated CO2.” Soil Biology & Biochemistry 46: 163–171. doi:10.1016/j.soilbio.2011.12.013.
- Martens-Habbena, W., P. M. Berube, H. Urakawa, J. R. de la Torre, and D. A. Stahl. 2009. “Ammonia Oxidation Kinetics Determine Niche Separation of Nitrifying Archaea and Bacteria.” Nature 461 (7266): 976–979. doi:10.1038/nature08465.
- Meinhardt, K. A., N. Stopnisek, M. W. Pannu, S. E. Strand, S. C. Fransen, K. L. Casciotti, and D. A. Stahl. 2018. “Ammonia‐oxidizing Bacteria are the Primary N2O Producers in an Ammonia‐oxidizing Archaea Dominated Alkaline Agricultural Soil.” Environmental Microbiology 20 (6): 2195–2206. doi:10.1111/1462-2920.14246.
- Mulder, A., A. A. Van de Graaf, L. A. Robertson, and J. G. Kuenen. 1995. “Anaerobic Ammonium Oxidation Discovered in a Denitrifying Fluidized Bed Reactor.” FEMS Microbiology Ecology 16 (3): 177–183. doi:10.1016/0168-6496(94)00081-7.
- Müller, C., R. J. Laughlin, O. Spott, and T. Rütting. 2014. “Quantification of N2O Emission Pathways via a 15N Tracing Model.” Soil Biology & Biochemistry 72: 44–54. doi:10.1016/j.soilbio.2014.01.013.
- Nauer, P. A., B. J. Fest, L. Visser, and S. K. Arndt. 2018. “On-farm Trial on the Effectiveness of the Nitrification Inhibitor DMPP Indicates No Benefits under Commercial Australian Farming Practices.” Agriculture, Ecosystems & Environment 253: 82–89. doi:10.1016/j.agee.2017.10.022.
- Nicol, G. W., S. Leininger, C. Schleper, and J. I. Prosser. 2008. “The Influence of Soil pH on the Diversity, Abundance and Transcriptional Activity of Ammonia Oxidizing Archaea and Bacteria.” Environmental Microbiology 10 (11): 2966–2978. doi:10.1111/j.1462-2920.2008.01701.x.
- Nie, S., G. B. Zhu, B. Singh, and Y. G. Zhu. 2019. “Anaerobic Ammonium Oxidation in Agricultural Soils-synthesis and Prospective.” Environmental Pollution 244: 127–134. doi:10.1016/j.envpol.2018.10.050.
- Nie, S., H. Li, X. Yang, Z. Zhang, B. Weng, F. Huang, G. B. Zhu, and Y. G. Zhu. 2015. “Nitrogen Loss by Anaerobic Oxidation of Ammonium in Rice Rhizosphere.” The ISME Journal 9 (9): 2059–2067. doi:10.1038/ismej.2015.25.
- Nishigaya, Y., Z. Fujimoto, and T. Yamazaki. 2016. “Optimized Inhibition Assays Reveal Different Inhibitory Responses of Hydroxylamine Oxidoreductases from Beta-and Gamma-proteobacterial Ammonium-oxidizing Bacteria.” Biochemical and Biophysical Research Communications 476 (3): 127–133. doi:10.1016/j.bbrc.2016.05.041.
- Norton, J. M. 2011. Diversity and environmental distribution of ammonia‐oxidizing bacteria, In Nitrification, edited by B. B. Ward, D. J. Arp and M. G. Klotz. Washinton DC: ASM Press.
- Norton, J. M., and Y. Ouyang. 2019. “Controls and Adaptive Management of Nitrification in Agricultural Soils.” Frontiers in Microbiology 10: 1931. doi:10.3389/fmicb.2019.01931.
- Nowka, B., H. Daims, and E. Spieck. 2015. “Comparison of Oxidation Kinetics of Nitrite-oxidizing Bacteria: Nitrite Availability as a Key Factor in Niche Differentiation.” Applied and Environmental Microbiology 81 (2): 745–753. doi:10.1128/AEM.02734-14.
- Nuñez, J., A. Arevalo, H. Karwat, K. Egenolf, J. Miles, N. Chirinda, G. Cadisch, et al. 2018. “Biological Nitrification Inhibition Activity in a Soil-grown Biparental Population of the Forage Grass, Brachiaria Humidicola.” Plant and Soil 426 (1–2): 401–411. doi:10.1007/s11104-018-3626-5.
- O’Sullivan, C. A., I. R. P. Fillery, M. M. Roper, and R. A. Richards. 2016. “Identification of Several Wheat Landraces with Biological Nitrification Inhibition Capacity.” Plant and Soil 404 (1–2): 61–74. doi:10.1007/s11104-016-2822-4.
- Osburn, E. D., and J. E. Barrett. 2020. “Abundance and Functional Importance of Complete Ammonia-oxidizing Bacteria (Comammox) versus Canonical Nitrifiers in Temperate Forest Soils.” Soil Biology & Biochemistry 145: 107801. doi:10.1016/j.soilbio.2020.107801.
- Oshiki, M., K. Shinyako-Hata, H. Satoh, and S. Okabe. 2015. “Draft Genome Sequence of an Anaerobic Ammonium-oxidizing Bacterium, ‘Candidatus Brocadia Sinica.’” Genome Announcements 3 (2): e00267–15. doi:10.1128/genomeA.00267-15.
- Ouyang, Y., J. M. Norton, and J. M. Stark. 2017. “Ammonium Availability and Temperature Control Contributions of Ammonia Oxidizing Bacteria and Archaea to Nitrification in an Agricultural Soil.” Soil Biology & Biochemistry 113: 161–172. doi:10.1016/j.soilbio.2017.06.010.
- Ouyang, Y., J. M. Norton, J. M. Stark, J. R. Reeve, and M. Y. Habteselassie. 2016. “Ammonia-oxidizing Bacteria are More Responsive than Archaea to Nitrogen Source in an Agricultural Soil.” Soil Biology & Biochemistry 96: 4–15. doi:10.1016/j.soilbio.2016.01.012.
- Pal, P., A. M. S. McMillan, and S. Saggar. 2016. “Pathways of Dicyandiamide Uptake in Pasture Plants: A Laboratory Study.” Biology and Fertility of Soils 52 (4): 539–546. doi:10.1007/s00374-016-1096-6.
- Pannu, M. W., K. A. Meinhardt, A. D. Bertagnolli, S. C. Fransen, D. A. Stahl, and S. E. Strand. 2019. “Nitrous Oxide Emissions Associated with Ammonia‐oxidizing Bacteria Abundance in Fields of Switchgrass with and without Intercropped Alfalfa.” Environmental Microbiology Reports 11 (5): 727–735. doi:10.1111/1758-2229.12790.
- Park, B. J., S. J. Park, D. N. Yoon, S. Schouten, J. S. S. Damsté, and S. K. Rhee. 2010. “Cultivation of Autotrophic Ammonia-oxidizing Archaea from Marine Sediments in Coculture with Sulfur-oxidizing Bacteria.” Applied and Environmental Microbiology 76 (22): 7575–7587. doi:10.1128/AEM.01478-10.
- Park, H. D., and D. R. Noguera. 2007. “Characterization of Two Ammonia‐oxidizing Bacteria Isolated from Reactors Operated with Low Dissolved Oxygen Concentrations.” Journal of Applied Microbiology 102 (5): 1401–1417. doi:10.1111/j.1365-2672.2006.03176.x.
- Pester, M., T. Rattei, S. Flechl, A. Gröngröft, A. Richter, J. Overmann, B. Reinhold‐Hurek, A. Loy, and M. Wagner. 2012. “amoA‐based Consensus Phylogeny of Ammonia‐oxidizing Archaea and Deep Sequencing of amoA Genes from Soils of Four Different Geographic Regions.” Environmental Microbiology 14 (2): 525–539. doi:10.1111/j.1462-2920.2011.02666.x.
- Petersen, D. G., S. J. Blazewicz, M. Firestone, D. J. Herman, M. Turetsky, and M. Waldrop. 2012. “Abundance of Microbial Genes Associated with Nitrogen Cycling as Indices of Biogeochemical Process Rates across a Vegetation Gradient in Alaska.” Environmental Microbiology 14 (4): 993–1008. doi:10.1111/j.1462-2920.2011.02679.x.
- Picone, N., A. Pol, R. Mesman, M. A. H. J. van Kessel, G. Cremers, A. H. van Gelder, T. A. van Alen, M. S. M. Jetten, S. Lücker, and H. J. M. Op den Camp. 2020. “Ammonia Oxidation at pH 2.5 By a New Gammaproteobacterial Ammonia-oxidizing Bacterium.” The ISME Journal 15: 1150–1164. doi:10.1038/s41396-020-00840-7.
- Pjevac, P., C. Schauberger, L. Poghosyan, C. W. Herbold, M. A. H. J. Van Kessel, A. Daebeler, M. Steinberger, M. S. M. Jetten, S. Lücker, and M. Wagner. 2017. “AmoA-targeted Polymerase Chain Reaction Primers for the Specific Detection and Quantification of Comammox Nitrospira in the Environment.” Frontiers in Microbiology 8: 1508. doi:10.3389/fmicb.2017.01508.
- Prosser, J. I., and G. W. Nicol. 2012. “Archaeal and Bacterial Ammonia-oxidisers in Soil: The Quest for Niche Specialisation and Differentiation.” Trends in Microbiology 20 (11): 523–531. doi:10.1016/j.tim.2012.08.001.
- Prosser, J. I., L. Hink, C. Gubry‐Rangin, and G. W. Nicol. 2020. “Nitrous Oxide Production by Ammonia Oxidizers: Physiological Diversity, Niche Differentiation and Potential Mitigation Strategies.” Global Change Biology 26 (1): 103–118. doi:10.1111/gcb.14877.
- Prosser, J. I., and T. M. Embley. 2002. “Cultivation-based and Molecular Approaches to Characterisation of Terrestrial and Aquatic Nitrifiers.” Antonie Van Leeuwenhoek 81 (1–4): 165–179. doi:10.1023/A:1020598114104.
- Qiao, C., L. Liu, S. Hu, J. E. Compton, T. L. Greaver, and Q. Li. 2015. “How Inhibiting Nitrification Affects Nitrogen Cycle and Reduces Environmental Impacts of Anthropogenic Nitrogen Input.” Global Change Biology 21 (3): 1249–1257. doi:10.1111/gcb.12802.
- Qin, H., H. Deng, C. Han, and W. Zhong. 2020. “Anammox Bacterial Abundance and Biodiversity in Greenhouse Vegetable Soil are Influenced by High Nitrate Content.” Pedosphere 30 (3): 343–351. doi:10.1016/S1002-0160(18)60023-2.
- Robertson, G. P., T. W. Bruulsema, R. J. Gehl, D. Kanter, D. L. Mauzerall, C. A. Rotz, and C. O. Williams. 2013. “Nitrogen–climate Interactions in US Agriculture.” Biogeochemistry 114 (1–3): 41–70. doi:10.1007/s10533-012-9802-4.
- Santoro, A. E., C. Buchwald, M. R. McIlvin, and K. L. Casciotti. 2011. “Isotopic Signature of N2O Produced by Marine Ammonia-oxidizing Archaea.” Science 333 (6047): 1282–1285. doi:10.1126/science.1208239.
- Sato, Y., H. Ohta, T. Yamagishi, Y. Guo, T. Nishizawa, M. H. Rahman, H. Kuroda, et al. 2012. “Detection of Anammox Activity and 16S rRNA Genes in Ravine Paddy Field Soil.” Microbes and Environments 27 (3): 316–319. doi:10.1264/jsme2.me11330.
- Sauder, L. A., M. Albertsen, K. Engel, J. Schwarz, P. H. Nielsen, M. Wagner, and J. D. Neufeld. 2017. “Cultivation and Characterization of Candidatus Nitrosocosmicus Exaquare, an Ammonia-oxidizing Archaeon from a Municipal Wastewater Treatment System.” The ISME Journal 11 (5): 1142–1157. doi:10.1038/ismej.2016.192.
- Scheer, C., D. W. Rowlings, M. Firrel, P. Deuter, S. Morris, and P. R. Grace. 2014. “Impact of Nitrification Inhibitor (DMPP) on Soil Nitrous Oxide Emissions from an Intensive Broccoli Production System in Sub-tropical Australia.” Soil Biology & Biochemistry 77: 243–251. doi:10.1016/j.soilbio.2014.07.006.
- Schleper, C., and G. W. Nicol. 2010. “Ammonia-oxidising Archaea–physiology, Ecology and Evolution.” Advances in Microbial Physiology 57: 1–41. doi:10.1016/B978-0-12-381045-8.00001-1.
- Shaw, L. J., G. W. Nicol, Z. Smith, J. Fear, J. I. Prosser, and E. M. Baggs. 2006. “Nitrosospira Spp. Can Produce Nitrous Oxide via a Nitrifier Denitrification Pathway.” Environmental Microbiology 8 (2): 214–222. doi:10.1111/j.1462-2920.2005.00882.x.
- Shen, L. D., H. S. Wu, X. Liu, and J. Li. 2017. “Vertical Distribution and Activity of Anaerobic Ammonium-oxidising Bacteria in a Vegetable Field.” Geoderma 288: 56–63. doi:10.1016/j.geoderma.2016.11.007.
- Shen, L. D., H. S. Wu, Z. Q. Gao, X. H. Xu, T. X. Chen, S. Liu, and H. X. Cheng. 2015. “Occurrence and Importance of Anaerobic Ammonium-oxidising Bacteria in Vegetable Soils.” Applied Microbiology and Biotechnology 99 (13): 5709–5718. doi:10.1007/s00253-015-6454-z.
- Shen, L. D., S. Liu, L. P. Lou, W. P. Liu, X. Y. Xu, P. Zheng, and B. L. Hu. 2013a. “Broad Distribution of Diverse Anaerobic Ammonium-oxidizing Bacteria in Chinese Agricultural Soils.” Applied and Environmental Microbiology 79 (19): 6167–6172. doi:10.1128/AEM.00884-13.
- Shen, T., M. Stieglmeier, J. Dai, T. Urich, and C. Schleper. 2013b. “Responses of the Terrestrial Ammonia-oxidizing Archaeon Ca. Nitrososphaera Viennensis and the Ammonia-oxidizing Bacterium Nitrosospira Multiformis to Nitrification Inhibitors.” FEMS Microbiology Letters 344 (2): 121–129. doi:10.1111/1574-6968.12164.
- Shi, X., H. W. Hu, J. Wang, J. Z. He, C. Zheng, X. Wan, and Z. Huang. 2018. “Niche Separation of Comammox Nitrospira and Canonical Ammonia Oxidizers in an Acidic Subtropical Forest Soil under Long-term Nitrogen Deposition.” Soil Biology & Biochemistry 126: 114–122. doi:10.1016/j.soilbio.2018.09.004.
- Shi, Y., Y. Jiang, S. Wang, X. Wang, and G. Zhu. 2020. “Biogeographic Distribution of Comammox Bacteria in Diverse Terrestrial Habitats.” Science of the Total Environment 717: 137257. doi:10.1016/j.scitotenv.2020.137257.
- Sonthiphand, P., M. W. Hall, and J. D. Neufeld. 2014. “Biogeography of Anaerobic Ammonia-oxidizing (Anammox) Bacteria.” Frontiers in Microbiology 5: 399. doi:10.3389/fmicb.2014.00399.
- Spang, A., A. Poehlein, P. Offre, S. Zumbrägel, S. Haider, N. Rychlik, B. Nowka, C. Schmeisser, E. V. Lebedeva, and T. Rattei. 2012. “The Genome of the Ammonia‐oxidizing C Andidatus N Itrososphaera Gargensis: Insights into Metabolic Versatility and Environmental Adaptations.” Environmental Microbiology 14 (12): 3122–3145. doi:10.1111/j.1462-2920.2012.02893.x.
- Speth, D. R., M. H. In ‘t Zandt, S. Guerrero-Cruz, B. E. Dutilh, and M. S. Jetten. 2016. “Genome-based Microbial Ecology of Anammox Granules in a Full-scale Wastewater Treatment System.” Nature Communications 7: 11172. doi:10.1038/ncomms11172.
- Stange, C. F., O. Spott, H. Arriaga, S. Menéndez, J. M. Estavillo, and P. Merino. 2013. “Use of the Inverse Abundance Approach to Identify the Sources of NO and N2O Release from Spanish Forest Soils under Oxic and Hypoxic Conditions.” Soil Biology & Biochemistry 57: 451–458. doi:10.1016/j.soilbio.2012.10.006.
- Steffen, W., K. Richardson, J. Rockström, S. E. Cornell, I. Fetzer, E. M. Bennett, R. Biggs, S. R. Carpenter, W. De Vries, and C. A. De Wit. 2015. “Planetary Boundaries: Guiding Human Development on a Changing Planet.” Science 347: 6223. doi:10.1126/science.1259855.
- Stein, L. Y. 2019. “Insights into the Physiology of Ammonia-oxidizing Microorganisms.” Current Opinion in Chemical Biology 49: 9–15. doi:10.1016/j.cbpa.2018.09.003.
- Stephen, J. R., A. E. McCaig, Z. Smith, J. I. Prosser, and T. M. Embley. 1996. “Molecular Diversity of Soil and Marine 16S rRNA Gene Sequences Related to Beta-subgroup Ammonia-oxidizing Bacteria.” Applied and Environmental Microbiology 62 (11): 4147–4154. doi:10.1128/aem.62.11.4147-4154.1996.
- Stieglmeier, M., A. Klingl, R. J. E. Alves, S. K. M. R. Rittmann, M. Melcher, N. Leisch, and C. Schleper. 2014a. “Nitrososphaera Viennensis Gen. Nov., Sp. Nov., An Aerobic and Mesophilic, Ammonia-oxidizing Archaeon from Soil and a Member of the Archaeal Phylum Thaumarchaeota.” International Journal of Systematic and Evolutionary Microbiology 64: 2738–2752. doi:10.1099/ijs.0.063172-0.
- Stieglmeier, M., M. Mooshammer, B. Kitzler, W. Wanek, S. Zechmeister-Boltenstern, A. Richter, and C. Schleper. 2014b. “Aerobic Nitrous Oxide Production through N-nitrosating Hybrid Formation in Ammonia-oxidizing Archaea.” The ISME Journal 8 (5): 1135–1146. doi:10.1038/ismej.2013.220.
- Strous, M., J. A. Fuerst, E. H. M. Kramer, S. Logemann, G. Muyzer, K. T. Van de Pas-Schoonen, R. Webb, J. G. Kuenen, and M. S. M. Jetten. 1999. “Missing Lithotroph Identified as New Planctomycete.” Nature 400 (6743): 446–449. doi:10.1038/22749.
- Subbarao, G. V., K. L. Sahrawat, K. Nakahara, I. M. Rao, M. Ishitani, C. T. Hash, M. Kishii, D. G. Bonnett, W. L. Berry, and J. C. Lata. 2013. “A Paradigm Shift Towards Low-nitrifying Production Systems: The Role of Biological Nitrification Inhibition (BNI).” Annals of Botany 112 (2): 297–316. doi:10.1080/07352680600794232.
- Subbarao, G. V., O. Ito, K. L. Sahrawat, W. L. Berry, K. Nakahara, T. Ishikawa, T. Watanabe, K. Suenaga, M. Rondon, and I. M. Rao. 2006. “Scope and Strategies for Regulation of Nitrification in Agricultural Systems—challenges and Opportunities.” Critical Reviews in Plant Sciences 25 (4): 303–335. doi:10.1080/07352680600794232.
- Subbarao, G. V., T. Yoshihashi, M. Worthington, K. Nakahara, Y. Ando, K. L. Sahrawat, I. M. Rao, J. C. Lata, M. Kishii, and H. J. Braun. 2015. “Suppression of Soil Nitrification by Plants.” Plant Science 233: 155–164. doi:10.1016/j.plantsci.2015.01.012.
- Sun, L., Y. Lu, F. Yu, H. J. Kronzucker, and W. Shi. 2016. “Biological Nitrification Inhibition by Rice Root Exudates and Its Relationship with Nitrogen‐use Efficiency.” New Phytologist 212 (3): 646–656. doi:10.1111/nph.14057.
- Suzuki, I., U. Dular, and S. C. Kwok. 1974. “Ammonia or Ammonium Ion as Substrate for Oxidation by Nitrosomonas Europaea Cells and Extracts.” Journal of Bacteriology 120 (1): 556–558. doi:10.1128/jb.120.1.556-558.1974.
- Szukics, U., G. Abell, V. Hödl, B. Mitter, A. Sessitsch, E. Hackl, and S. Zechmeister-Boltenstern. 2010. “Nitrifiers and Denitrifiers Respond Rapidly to Changed Moisture and Increasing Temperature in a Pristine Forest Soil.” FEMS Microbiology Ecology 72 (3): 395–406. doi:10.1111/j.1574-6941.2010.00853.x.
- Takahashi, Y., H. Fujitani, Y. Hirono, K. Tago, Y. Wang, M. Hayatsu, and S. Tsuneda. 2020. “Enrichment of Comammox and Nitrite-oxidizing Nitrospira from Acidic Soils.” Frontiers in Microbiology 11: 1737. doi:10.3389/fmicb.2020.01737.
- Taylor, A. E., A. T. Giguere, C. M. Zoebelein, D. D. Myrold, and P. J. Bottomley. 2017. “Modeling of Soil Nitrification Responses to Temperature Reveals Thermodynamic Differences between Ammonia-oxidizing Activity of Archaea and Bacteria.” The ISME Journal 11 (4): 896–908. doi:10.1038/ismej.2016.17.
- Taylor, A. E., L. H. Zeglin, T. A. Wanzek, D. D. Myrold, and P. J. Bottomley. 2012. “Dynamics of Ammonia-oxidizing Archaea and Bacteria Populations and Contributions to Soil Nitrification Potentials.” The ISME Journal 6 (11): 2024–2032. doi:10.1038/ismej.2012.51.
- Taylor, A. E., N. Vajrala, A. T. Giguere, A. I. Gitelman, D. J. Arp, D. D. Myrold, L. A. Sayavedra-Soto, and P. J. Bottomley. 2013. “Use of Aliphatic N-alkynes to Discriminate Soil Nitrification Activities of Ammonia-oxidizing Thaumarchaea and Bacteria.” Applied and Environmental Microbiology 79 (21): 6544–6551. doi:10.1128/AEM.01928-13.
- Tourna, M., M. Stieglmeier, A. Spang, M. Könneke, A. Schintlmeister, T. Urich, M. Engel, M. Schloter, M. Wagner, and A. Richter. 2011. “Nitrososphaera Viennensis, an Ammonia Oxidizing Archaeon from Soil.” Proceedings of the National Academy of Sciences 108 (20): 8420–8425. doi:10.1073/pnas.1013488108.
- Tourna, M., T. E. Freitag, G. W. Nicol, and J. I. Prosser. 2008. “Growth, Activity and Temperature Responses of Ammonia‐oxidizing Archaea and Bacteria in Soil Microcosms.” Environmental Microbiology 10 (5): 1357–1364. doi:10.1111/j.1462-2920.2007.01563.x.
- Treusch, A. H., S. Leininger, A. Kletzin, S. C. Schuster, H. Klenk, and C. Schleper. 2005. “Novel Genes for Nitrite Reductase and Amo‐related Proteins Indicate a Role of Uncultivated Mesophilic Crenarchaeota in Nitrogen Cycling.” Environmental Microbiology 7 (12): 1985–1995. doi:10.1111/j.1462-2920.2005.00906.x.
- Van de Graaf, A. A., P. de Bruijn, L. A. Robertson, M. S. M. Jetten, and J. G. Kuenen. 1996. “Autotrophic Growth of Anaerobic Ammonium-oxidizing Micro-organisms in a Fluidized Bed Reactor.” Microbiology 142 (8): 2187–2196. doi:10.1099/13500872-142-8-2187.
- van Kessel, M. A. H. J., D. R. Speth, M. Albertsen, P. H. Nielsen, H. J. M. Op den Camp, B. Kartal, M. S. M. Jetten, and S. Lücker. 2015. “Complete Nitrification by a Single Microorganism.” Nature 528 (7583): 555–559. doi:10.1038/nature16459.
- Verstraete, W., and D. D. Focht. 1977. “Biochemical Ecology of Nitrification and Denitrification.” In Advances in Microbial Ecology, edited by Nelson K. E. Berlin Heidelberg: Springer.
- Villegas, D., A. Arevalo, J. Nuñez, J. Mazabel, G. Subbarao, I. Rao, J. De Vega, and J. Arango. 2020. “Biological Nitrification Inhibition (BNI): Phenotyping of a Core Germplasm Collection of the Tropical Forage Grass Megathyrsus Maximus under Greenhouse Conditions.” Frontiers in Plant Science 11: 820. doi:10.3389/fpls.2020.00820.
- Wang, Q., L. M. Zhang, J. P. Shen, S. Du, L. L. Han, and J. Z. He. 2016. “Nitrogen Fertiliser-induced Changes in N2O Emissions are Attributed More to Ammonia-oxidising Bacteria Rather than Archaea as Revealed Using 1-octyne and Acetylene Inhibitors in Two Arable Soils.” Biology and Fertility of Soils 52 (8): 1163–1171. doi:10.1007/s00374-016-1151-3.
- Wang, X., S. Wang, Y. Jiang, J. Zhou, C. Han, and G. Zhu. 2020. “Comammox Bacterial Abundance, Activity, and Contribution in Agricultural Rhizosphere Soils.” Science of the Total Environment 727: 138563. doi:10.1016/j.scitotenv.2020.138563.
- Wang, X., Y. Wang, F. Zhu, C. Zhang, P. Wang, and X. Zhang. 2021a. “Effects of Different Land Use Types on Active Autotrophic Ammonia and Nitrite Oxidizers in Cinnamon Soils.” Applied and Environmental Microbiology 87 (12): e00092–21. doi:10.1128/AEM.00092-21.
- Wang, Z., M. Zheng, J. Meng, Z. Hu, G. Ni, A. G. Calderon, H. Li, H. De Clippeleir, A. Al-Omari, and S. Hu. 2021b. “Robust Nitritation Sustained by Acid-tolerantammonia-oxidizing Bacteria.” Environmental Science & Technology. doi:10.1021/acs.est.0c05181.
- Wang, Z., Y. Cao, X. Zhu-Barker, G. W. Nicol, A. L. Wright, Z. Jia, and X. Jiang. 2019. “Comammox Nitrospira Clade B Contributes to Nitrification in Soil.” Soil Biology & Biochemistry 135: 392–395. doi:10.1016/j.soilbio.2019.06.004.
- Wertz, S., A. K. K. Leigh, and S. J. Grayston. 2012. “Effects of Long-term Fertilization of Forest Soils on Potential Nitrification and on the Abundance and Community Structure of Ammonia Oxidizers and Nitrite Oxidizers.” FEMS Microbiology Ecology 79 (1): 142–154. doi:10.1111/j.1574-6941.2011.01204.x.
- Winogradsky, S. 1891. “The Formation of Nitrates in the Process of Nitrification.” Annales de l’Institut Pasteur 5: 577–616.
- Woodward, E. E., D. W. Kolpin, W. Zheng, N. L. Holm, S. M. Meppelink, P. J. Terrio, and M. L. Hladik. 2019. “Fate and Transport of Nitrapyrin in Agroecosystems: Occurrence in Agricultural Soils, Subsurface Drains, and Receiving Streams in the Midwestern US.” Science of the Total Environment 650: 2830–2841. doi:10.1016/j.scitotenv.2018.09.387.
- Wrage-Mönnig, N., M. A. Horn, R. Well, C. Müller, G. Velthof, and O. Oenema. 2018. “The Role of Nitrifier Denitrification in the Production of Nitrous Oxide Revisited.” Soil Biology & Biochemistry 123: A3–A16. doi:10.1016/j.soilbio.2018.03.020.
- Wu, J., Y. Hong, X. He, L. Jiao, X. Wen, S. Chen, G. Chen, et al. 2018. “Anaerobic Ammonium Oxidation in Acidic Red Soils.” Frontiers in Microbiology 9: 2142. doi:10.3389/fmicb.2018.02142.
- Wu, Y., Y. Guo, X. Lin, W. Zhong, and Z. Jia. 2012. “Inhibition of Bacterial Ammonia Oxidation by Organohydrazines in Soil Microcosms.” Frontiers in Microbiology 3: 10. doi:10.3389/fmicb.2012.00010.
- Xi, D., R. Bai, L. Zhang, and Y. Fang. 2016. “Contribution of Anammox to Nitrogen Removal in Two Temperate Forest Soils.” Applied and Environmental Microbiology 82 (15): 4602–4612. doi:10.1128/AEM.00888-16.
- Xia, L., S. K. Lam, D. Chen, J. Wang, Q. Tang, and X. Yan. 2017. “Can Knowledge‐based N Management Produce More Staple Grain with Lower Greenhouse Gas Emission and Reactive Nitrogen Pollution? A Meta‐analysis.” Global Change Biology 23 (5): 1917–1925. doi:10.1111/gcb.13455.
- Yang, W., Y. Wang, K. Tago, S. Tokuda, and M. Hayatsu. 2017. “Comparison of the Effects of Phenylhydrazine Hydrochloride and Dicyandiamide on Ammonia-oxidizing Bacteria and Archaea in Andosols.” Frontiers in Microbiology 8: 2226. doi:10.3389/fmicb.2017.02226.
- Yang, X. D., K. Ni, Y. Z. Shi, X. Y. Yi, L. F. Ji, L. F. Ma, and J. Y. Ruan. 2020. “Heavy Nitrogen Application Increases Soil Nitrification through Ammonia-oxidizing Bacteria Rather than Archaea in Acidic Tea (Camellia Sinensis L.) Plantation Soil.” Science of the Total Environment 717: 137248. doi:10.1016/j.scitotenv.2020.137248.
- Yokoyama, K., K. Jinnai, Y. Sakiyama, and M. Touma. 2012. “Contribution of Fungi to Acetylene-tolerant and High Ammonia Availability-dependent Nitrification Potential in Tea Field Soils with Relatively Neutral pH.” Applied Soil Ecology 62: 37–41. doi:10.1016/j.apsoil.2012.07.008.
- Zhang, J., C. Mueller, and Z. Cai. 2015. “Heterotrophic Nitrification of Organic N and Its Contribution to Nitrous Oxide Emissions in Soils.” Soil Biology & Biochemistry 84: 199–209. doi:10.1016/j.soilbio.2015.02.028.
- Zhang, Y., J. Zhang, T. Meng, T. Zhu, C. Müller, and Z. Cai. 2013. “Heterotrophic Nitrification Is the Predominant NO3− Production Pathway in Acid Coniferous Forest Soil in Subtropical China.” Biology and Fertility of Soils 49 (7): 955–957. doi:10.1007/s00374-012-0772-4.
- Zhang, Y., S. Dai, X. Huang, Y. Zhao, J. Zhao, Y. Cheng, Z. Cai, and J. Zhang. 2020. “pH-induced Changes in Fungal Abundance and Composition Affects Soil Heterotrophic Nitrification after 30 Days of Artificial pH Manipulation.” Geoderma 366: 114255. doi:10.1016/j.geoderma.2020.114255.
- Zhang, Y., W. Zhao, Z. Cai, C. Müller, and J. Zhang. 2018. “Heterotrophic Nitrification Is Responsible for Large Rates of N2O Emission from Subtropical Acid Forest Soil in China.” European Journal of Soil Science 69 (4): 646–654. doi:10.1016/j.soilbio.2019.107562.
- Zhao, J., M. O. Bello, Y. Meng, J. I. Prosser, and C. Gubry-Rangin. 2020. “Selective Inhibition of Ammonia Oxidising Archaea by Simvastatin Stimulates Growth of Ammonia Oxidising Bacteria.” Soil Biology & Biochemistry 141: 107673. doi:10.1016/j.soilbio.2019.107673.
- Zhao, Q., J. Bai, Y. Gao, G. Zhang, Q. Lu, and J. Jia. 2021. “Heavy Metal Contamination in Soils from Freshwater Wetlands to Salt Marshes in the Yellow River Estuary, China.” Science of the Total Environment 774: 145072. doi:10.1016/j.scitotenv.2021.145072.
- Zhao, Y., Y. Feng, L. Chen, Z. Niu, and S. Liu. 2019. “Genome-centered Omics Insight into the Competition and Niche Differentiation of Ca. Jettenia and Ca. Brocadia Affiliated to Anammox Bacteria.” Applied Microbiology and Biotechnology 103 (19): 8191–8202. doi:10.1007/s00253-019-10040-9.
- Zhou, X., S. Wang, S. Ma, X. Zheng, Z. Wang, and C. Lu. 2020. “Effects of Commonly Used Nitrification Inhibitors—dicyandiamide (DCD), 3, 4-dimethylpyrazole Phosphate (DMPP), and Nitrapyrin—on Soil Nitrogen Dynamics and Nitrifiers in Three Typical Paddy Soils.” Geoderma 380: 114637. doi:10.1016/j.geoderma.2020.114637.
- Zhu, G., S. Wang, Y. Wang, C. Wang, N. Risgaard-Petersen, M. S. Jetten, and C. Yin. 2011. “Anaerobic Ammonia Oxidation in a Fertilized Paddy Soil.” The ISME Journal 5 (12): 1905–1912. doi:10.1038/ismej.2011.63.
- Zhu, T., T. Meng, J. Zhang, W. Zhong, C. Müller, and Z. Cai. 2015. “Fungi-dominant Heterotrophic Nitrification in a Subtropical Forest Soil of China.” Journal of Soils and Sediments 15 (3): 705–709. doi:10.1007/s11368-014-1048-4.