ABSTRACT
Excess inputs of reactive nitrogen (Nr) from the atmosphere will cause disturbances to forest ecosystems, including soil and stream water acidification, plant nutrient imbalances, alterations of species compositions (such as biodiversity losses), and nitrogen (N) leaching into stream water. Central Japan (or Chubu region) has experienced both domestic and transboundary air pollution since the 1950s. Emissions of nitrogen oxides in China peaked in 2011/2012, although the peak of ammonia emissions has not been confirmed. Changes in these emissions have been reflected gradually in atmospheric N deposition rates and the biogeochemical cycle in ecosystems in central Japan. We synthetically analyzed the current N saturation situation for forest ecosystems in central Japan based on our recent publications and the latest available dataset. The stream water in the Lake Ijira catchment (IJR) on the Pacific side in Gifu Prefecture became acidified and N-saturated in the mid-1990s but has begun to recover with a decrease in nitrate (NO3–) concentration since the mid-2000s. The progress and recovery of N saturation appeared to be related to the trends of atmospheric deposition, climatic anomalies (such as the cool and drought summers in 1993/1994), and forest management. The NO3 – concentrations in stream water within the forest catchment of Kajikawa (KJK) on the Sea of Japan side in Niigata Prefecture have continued to increase, although the catchment seems to be recovering from acidification owing to a decline in atmospheric sulfur (and probably N) deposition. Finally, the NO3– concentration in stream water in KJK became higher than that in IJR, a direct inversion of the situation in the early 2000s. The recent analysis of 17O excess in NO3– estimated that the export of biologically unprocessed atmospheric NO3– via stream water was larger in KJK than in IJR. The N cycle in soil-plant systems likely does not function as expected under excess atmospheric N input (still over 10 kg ha–1 year–1), especially in KJK. In addition to atmospheric N deposition, reduced N uptake rates as coniferous forests mature, and changing precipitation amounts/patterns appear to accelerate N leaching from forest catchments in central Japan.
1. Introduction
The nitrogen (N) cycle is one of the most important earth-system processes in the concept of ‘Planetary boundaries,’ and the cycle may have already transgressed its boundary (Rockström et al. Citation2009). Production rates of reactive nitrogen (Nr) have accelerated sharply since the 1960s through the Haber-Bosch process, biological nitrogen fixation promoted via widespread cultivation, and the combustion of fossil fuels. Accordingly, the anthropogenic production rate increased approximately from 15 Tg N per year in 1860 to 165 Tg per year in 2000 (Galloway et al. Citation2003). A portion of Nr produced by human activities is released to the atmosphere and finally deposited in terrestrial ecosystems. Disturbances of forest ecosystems via excess Nr inputs from the atmosphere have been observed in the United States (Aber et al. Citation1989, Citation1998; McNulty, Aber, and Newman Citation1996; Fenn et al. Citation1998), in Europe (Grennfelt and Hultberg Citation1986; Skeffington and Wilson Citation1988; Wright et al. Citation1995), and in Asia (Fang et al. Citation2011; Chiwa et al. Citation2019); these disturbances include soil and stream water acidification, imbalances of plant nutrients, alterations to species composition (such as biodiversity losses), and N leaching into stream water.
Central Japan (or the Chubu region) has historically experienced both domestic and transboundary air pollution since the 1950s (Yamashita et al. Citation2016; Sase et al. Citation2019). In the 1960s/1970s, large amounts of sulfur (S) oxides that originated from the Chukyo Industrial Area, Japan’s largest industrial area located around Nagoya City, were deposited in forest area on the Pacific side (Ishida, Tayasu, and Takenaka Citation2015; Sase et al. Citation2019). On the Sea of Japan side, deposition amounts of non-sea salt (nss) S derived from the Asian Continent significantly increased from the mid-1990s onward and then started declining in the mid-2000s (Ohizumi et al. Citation2016). The declining S deposition trends since the mid-2000s have closely corresponded to current emission trends. Regarding Nr, emissions of nitrogen oxides (NOX) in Japan declined after the peak in the 1970s, whereas those of ammonia (NH3) reached a plateau the 1980s and have since been stable or slightly decreased. Emissions of NOX in China increased until 2011, whereas NH3 emissions have continued to increase up to present (Kurokawa and Ohara Citation2020). Deposition amounts of dissolved inorganic nitrogen (DIN), calculated as the sum of nitrate (NO3–) and ammonium (NH4+), have declined since the mid-2000s in forest catchments on the Pacific side and Sea of Japan side; this tendency partly reflects recent emission trends (Sase et al. Citation2019, Citation2021).
The Ministry of the Environment of Japan (MOEJ) has been monitoring atmospheric deposition and its effects on terrestrial ecosystems since the 1980s. Based on the monitoring data, Yamada et al. (Citation2007) first reported that acidification of stream water and lake water has occurred since the mid-1990s in the Lake Ijira catchment (IJR) located approximately 40 km from the Chukyo Industrial Area. In IJR, the annual deposition amounts of H+, nss-sulfate (SO42–), and NO3– were among the highest of all Japanese monitoring sites. The pH in surface mineral soils in IJR decreased from 4.5 in 1990 to 3.9 in 2003, and the mean annual DIN deposition was 19.2 kg ha–1 year–1 (1.37 kmol ha–1 year–1 as wet and dry deposition) (Nakahara et al. Citation2010). This deposition was significantly greater than N leaching threshold values in Asia or Europe (Fang et al. Citation2011). IJR was suggested as having become N-saturated in the mid-1990s owing to changes in biogeochemical processes triggered by the climatic anomaly in 1994, and other forest factors (defoliation or maturation of trees) and an increase in atmospheric deposition likely contributed as well (Nakahara et al. Citation2010). On the Sea of Japan side, atmospheric deposition rates and stream water chemistry have been monitored since 2002 in Kajikawa catchment (KJK) in a Japanese cedar (Cryptomeria japonica D. Don) forest for the Acid Deposition Monitoring Network in East Asia (EANET). Based on a 5-y dataset from 2002 to 2007 in KJK, the annual DIN deposition reached its highest level in Japan (18 kg ha–1 year–1 by throughfall and stemflow, TF+SF) and temporary acidification with NO3– leaching was often observed during rain events (Kamisako et al. Citation2008). In the neighboring prefectures of Gifu, Nagano, and Niigata (see ), significant long-term declining trends in river water pH occurred from the 1970s/1980s to the early 2000s in several watersheds, as this water mainly drained from granite/rhyolite rocks with a low acid neutralizing capacity (Kurita and Ueda Citation2006; Matsubara et al. Citation2009). Thus, phenomena suggesting acidification and/or N saturation have been reported in central Japan. The above studies mainly included data spanning until the early 2000s; however, anthropogenic emissions of S and Nr species have been dynamically changing since the mid-2000s as previously mentioned.
Figure 1. Locations and images of Lake Ijira catchment (IJR) and Kajikawa catchment (KJK) in central Japan (after Kamisako et al. Citation2008; Sase et al. Citation2019). Stream water samples were collected at the outlet of catchment areas (shown as the sampling points). In the case of Lake Ijira catchment (IJR), stream water samples have been collected at RW1 and RW2 since 2007 and 1988, respectively.
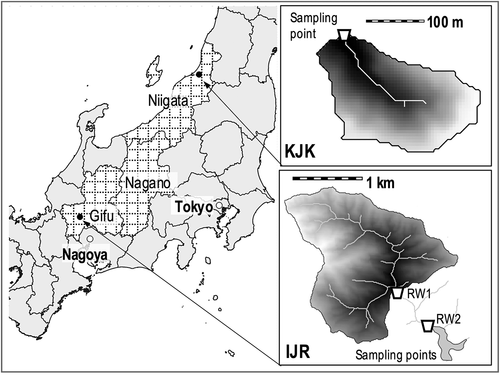
Ecosystems in both IJR and KJK sensitively responded to a reduction of atmospheric deposition in recent years, showing symptoms of recovery from acidification (Sase et al. Citation2019, Citation2021). However, the recovery from N saturation may also be regulated by other various factors, such as forest conditions and the climate. Interestingly, the NO3– concentration in stream water in KJK is still increasing (Sase et al. Citation2021). A recent analysis of 17O excess in NO3– clarified that unprocessed atmospheric NO3– was leached from both ecosystems in KJK and IJR (Nakagawa et al. Citation2018). This study’s objectives are to assess the current N saturation situation in forest areas in central Japan, and to identify factors influencing the current situation. We have synthetically analyzed N fluxes in forest ecosystems in central Japan, in conjunction with sulfate dynamics, based on our recent publications and the latest available data.
2. Survey outline in central Japan
The site descriptions and field survey methods utilized in this study are detailed in previous publications for IJR (Yamada et al. Citation2007; Nakahara et al. Citation2010; Sase et al. Citation2019) and KJK (Kamisako et al. Citation2008; Sase et al. Citation2008, Citation2012, Citation2021).
2.1. Study site
IJR (35°34ʹ N 136°42ʹ E; ) is the catchment area of the Kamagatani River (approximately 298 ha in area) inflowing to the Lake Ijira, a reservoir in Yamagata City, Gifu Prefecture, on the Pacific side. KJK (37°59ʹ N, 139°23ʹ E; ) is a small forested catchment (approximately 3.84 ha in area) in the northern part of Shibata City (formerly Kajikawa village), Niigata Prefecture, near the Sea of Japan coast. Both catchment areas are fully covered by forests. Japanese cypress (Chamaecyparis obtusa Sieb. & Zucc.), broadleaf trees, Japanese red pine (Pinus densiflora Sieb. & Zucc.), and Japanese cedar trees can be found in IJR (Nakahara et al. Citation2010; Sase et al. Citation2019), whereas most of the area in KJK is covered by planted Japanese cedars (Sase et al. Citation2021). Site characteristics, including precipitation amounts, vegetation, soil, and geology, are summarized in .
Table 1. Site characteristics of two study catchments, Lake Ijira catchment on the Pacific side and Kajikawa catchment on the Sea of Japan side
2.2. Field surveys
Stream water (SW) samples have been collected at the upstream point of Kamagatani River (RW1 in ) in IJR every two weeks since 2007 to precisely estimate material fluxes from the river, in addition to the historical quarterly sampling point since 1988 at the inflowing point to the lake (RW2 in ). Wet deposition samples and atmospheric particulate/gaseous samples have been collected since 1999 using a wet-only sampler and the filter pack method, respectively, at the Ijira EANET deposition monitoring site located within the IJR catchment area (e.g., EANET Citation2020). Data since 2000 are disclosed at the EANET website. The fluxes from dry deposition have been estimated by the inferential method based on the air concentrations and meteorological data (EANET Citation2013). Soil sampling and tree growth observations were also conducted in IJR as part of the national and/or EANET monitoring regimes.
In KJK, SW samples have been collected biweekly or monthly since 2002 at the outlet of the catchment area, where a V-notch weir was constructed to estimate flow rate and material fluxes from the stream (). Precipitation samples outside the forest canopy and throughfall (TF) and stemflow (SF) samples below forest canopy have been collected using bulk samplers in KJK. Although DIN fluxes by TF and SF in KJK may often be underestimated owing to various factors such as the uptake of ions in the tree canopy and alterations of ions via microbial activities during field storage (Sase et al. Citation2008), the fluxes by TF+SF have been compared with rates of N leaching to the stream.
2.3. Chemical analysis
The pH, electrical conductivity (EC), and concentrations of major inorganic ions (Cl−, NO3−, SO42−, Na+, K+, Ca2+, Mg2+, and NH4+) in the water samples and extract solutions from the filters for the aforementioned dry deposition were determined according to the methods described in the EANET technical manual (EANET Citation2010a, Citation2010b). Alkalinity of SW samples was measured using the pH 4.8 endpoint method (EANET Citation2010a; hereinafter referred to as ‘pH 4.8 alkalinity’). Ion concentrations were determined using ion chromatography, and concentrations of H+ ions were calculated from the pH values. The ion concentration was expressed in micromoles of charge per liter (μmolc L−1). The non-sea-salt (nss) fraction of SO42− was estimated from the concentrations of Na+ and the component ratio of seawater (EANET Citation2010a). The analysis of 17O excess (∆17O) of NO3– was applied to the rainwater, soil solution, and stream water samples collected from 2012 to 2014. The detailed analytical methods used for this part of the study are found in Nakagawa et al. (Citation2018). In this study, we focused on the NO3– concentration in SW as an indicator of the N saturation status.
2.4. Quality control and assurance and data analysis
All measurement processed was subjected to quality control and quality assurance (QA/QC) procedures specified in the technical manuals of the EANET (EANET Citation2010a, Citation2010b).
We defined a water year (WY) in IJR as the time interval from November of one year to October of the next year considering the hydrological pattern on the Pacific side. Moreover, for convenience, we identified each WY by the year in which it ended. For example, the WY from November 2013 to October 2014 is called ‘WY 2014ʹ in IJR (Sase et al. Citation2019). By comparison, a WY in KJK was defined as the time interval from June of one year to May of the next year because of the hydrological pattern on the Sea of Japan side. Each WY was identified by the year in which it began, e.g., the WY from June 2013 to May 2014 is called ‘WY 2013’ in KJK (Sase et al. Citation2021).
3. Results and discussion
3.1. Trends of atmospheric deposition
The atmospheric environment in Northeast Asia is continuously and dynamically changing. Emissions of sulfur dioxide (SO2) in China peaked in 2006 and started declining thereafter (Lu, Zhang, and Streets Citation2011), whereas domestic emissions in Japan began declining in the 1980s (Smith et al. Citation2011). Moreover, emissions of NOX in China declined after 2011, whereas NH3 emissions have continued increasing (Kurokawa and Ohara Citation2020). These emission trends in the region have been clearly reflected in the atmospheric depositions in IJR and KJK. The annual deposition amounts of SO4-S and DIN declined in both IJR and KJK, although deposition amounts of NO3-N in KJK did not show clear trends (; updated after Sase et al. Citation2019, Sase et al. Citation2021; EANET Citation2020). Deposition amounts of nss-S (considered as mainly anthropogenic S) peaked in 2006/2007, which is similar to the SO2 emission trends in China. The deposition trends of NO3-N and NH3-N were not necessarily consistent with the emissions of NOX and NH3 in China.
Figure 2. Annual atmospheric fluxes of (1) SO4–sulfur (S) and/or non-sea salt (nss) SO4–S and (2) dissolved inorganic nitrogen (DIN) in (a) Lake Ijira catchment (IJR) and (b) Kajikawa catchment (KJK) (updated with the latest data after EANET Citation2020; Sase et al. Citation2021). Wet, fluxes by wet deposition at the Acid Deposition Monitoring Network in East Asia (EANET) site; RF, fluxes by rainfall outside canopy; TF+SF, fluxes by throughfall and stemflow.
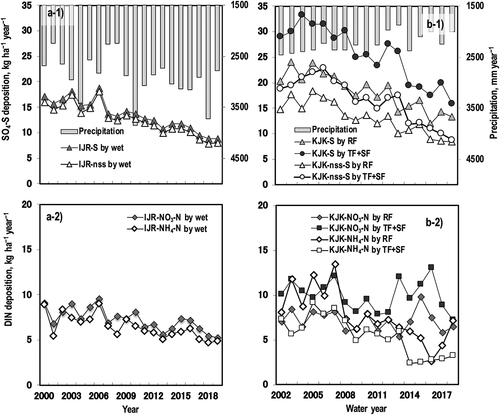
Transboundary air pollution has influenced atmospheric deposition even in IJR, as suggested by an S isotopic analysis of rainwater (Inomata et al. Citation2019; Sase et al. Citation2019, Citation2021). In KJK, nss-S deposition amounts distinctly increased in winter owing to northwesterly seasonal winds, thereby suggesting the strong influence of transboundary air pollution (Kamisako et al. Citation2008; Sase et al. Citation2008, Citation2012, Citation2021). This influence was also suggested by previous S isotopic studies (Ohizumi et al. Citation2016; Inomata et al. Citation2019). Thus, the decreasing trends of S deposition reflect those of emissions in China. Interestingly, both domestic and transboundary air pollutants may have affected DIN deposition trends. The deposition trends of NH4-N in IJR and KJK were similar to those of S, which showed relatively high values until their peak in 2006/2007 and low values in the 2010s. This suggests that NH4+ and SO42– in rainwater were transported as particulate matter (ammonium sulfate, (NH4)2SO4) at a long range. Emission reductions of NOX and NH3 in Japan in the 2000s (Kurokawa et al. Citation2013) may have also contributed to IJR’s deposition trends (Sase et al. Citation2019). In KJK, the NH4-N deposition via RF also seemed to be influenced by local emission sources because of its slightly different seasonality from those of NO3-N and S, which were mainly regulated by transboundary air pollution (Kamisako et al. Citation2008; Sase et al. Citation2008). As described previously, NOX emissions in China increased continuously until the early 2010s and then gradually decreased (Kurokawa and Ohara Citation2020). However, the NO3-N deposition in KJK did not reflect this trend and instead increased in the mid-2010s. Therefore, the deposition trends based on field observations at the study sites did not necessarily reflect the national emission trends estimated based on statistics.
Although only wet deposition data are shown for IJR in , owing to the limited contribution of dry deposition at that location (Endo et al. Citation2011), the trends of S and DIN are reasonable (Sase et al. Citation2019). The trends of S by TF+SF in KJK are also reasonable owing to the limited canopy interactions of SO42– (Sase et al. Citation2008). In contrast, because deposition amounts of NH4-N (and probably NO3-N) by TF+SF were largely influenced by canopy interactions, such as uptake on leaf surfaces (Sase et al. Citation2008), they did not provide a precise total DIN deposition. However, as the trends of DIN (NO3-N + NH4-N) showed, the deposition amounts appeared to decrease or at least stay constant at minimum (Sase et al. Citation2021).
3.2. Changes in stream water chemistry
The historical data collected in IJR since 1988 demonstrate the processes of acidification and its recovery (; after Nakahara et al. Citation2010; Sase et al. Citation2019). The pH and pH 4.8 alkalinity suddenly declined in the mid-1990s after a slight increase in the beginning of the survey. Simultaneously, significantly high concentrations of SO42– and NO3– were observed, and then the NO3– concentration increased continuously until the early 2000s. Following acidification, the pH and pH 4.8 alkalinity increased to almost their original levels in the early 2000s, and the pH continuously increased gradually.
Figure 3. Changes in (1) pH and pH 4.8 alkalinity and (2) concentrations of SO42– and NO3– in stream water in (a) Lake Ijira catchment (IJR) and (b) Kajikawa catchment (KJK) (drawn with the latest data after Sase et al. Citation2019, Citation2021). For IJR, the historical data since 1988 at RW2 are plotted.
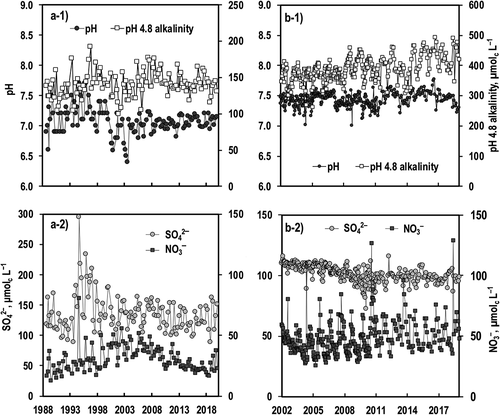
As previously mentioned, IJR has been famous for having the highest level of acid deposition in Japan (Yamada et al. Citation2007; Matsubara et al. Citation2009). The wet deposition amounts of S and N increased after 1994 and soil acidification phenomena were also reported in the 1990s (Nakahara et al. Citation2010). In these severe conditions, further climatic anomalies, the extremely cool summer in 1993, and the drought summer in 1994 triggered further alterations of biogeochemical cycles such as a decline of forest growth rates and a consequent N uptake reduction. Therefore, diminished effects from the climatic anomalies contributed to the smooth recovery from acidification and N saturation together with declining atmospheric deposition (Sase et al. Citation2019). Among other possible factors, such as the defoliation/dieback of Japanese red pine caused by pine wilt disease and the maturation of Japanese cedar stands (Nakahara et al. Citation2010), the growth of Japanese cedar stands has been improved by the thinning of trees in the mid-2000s through forest management, which appeared to contribute to the recovery through an enhanced forest N uptake (Sase et al. Citation2019). The situation in IJR suggests the importance of forest resilience against climatic events and appropriate managements in plantation forests within the context of air pollution effects.
In KJK, chronological changes in the stream water chemistry showed relatively simple recovery processes from acidification; the pH and pH 4.8 alkalinity remained relatively stable until 2006 and increased gradually thereafter, while the SO42– concentration declined substantially after 2006 (; after Sase et al. Citation2021). These phenomena are closely aligned with the trends of atmospheric deposition shown in . However, the NO3– concentration slightly declined in the beginning of the survey until 2004/2005 and then have increased continuously, even though the DIN deposition amounts did not increase in the corresponding period.
Although the SO42– concentration in stream water in KJK seemed to have sensitively responded to the atmospheric S deposition, this study’s isotopic analysis suggested that S derived from the atmospheric deposition was thoroughly homogenized via internal processes, such as absorption/desorption in soil and plant uptake rates, and then flowed into the stream water (Sase et al. Citation2021). The internal S processes sensitively responded to the atmospheric deposition in KJK. However, the NO3– concentration in stream water did not necessarily respond to the atmospheric N deposition. The thinning of Japanese cedar trees in 2002 was suggested as having contributed to an acceleration of N uptake by trees, which resulted in a decrease of NO3– concentrations until 2004/2005. However, these effects remained only for 2–3 y before an increase in the concentration was observed (Sase et al. Citation2021). Finally, the NO3– concentration in stream water in KJK recently became higher than that in IJR, representing a reversal of the relationship in the early 2000s. Through utilizing the ∆17O analysis of NO3– from 2012 to 2014 in IJR and KJK, Nakagawa et al. (Citation2018) estimated that 6.5%±1.8% and 9.4%±2.6% of the annual atmospheric NO3– inputs, respectively, flowed into stream water as the output without biological processes in plant-soil systems. This is in accordance with the relationship between NO3– concentration levels in both catchments during the study period.
As previously mentioned, both IJR and KJK appear to be in recovery from acidification with a reduction of atmospheric deposition. In Japanese lakes monitored by MOEJ, no progress of acidification was found and SO42– concentrations in lake waters declined after 2006 (Sase et al. Citation2021). A reduction of the atmospheric N deposition is expected to contribute to a decline in N leaching (e.g., Baba et al. Citation2020). However, the responses of IJR and KJK in this study differed, and there are a few possible reasons for this discrepancy. First, the maturation of trees and forest management techniques appear to have regulated NO3– concentrations in stream water in both IJR and KJK. However, the dates that these phenomena occurred slightly differed within JIR and KJK despite the ages of the main tree species in both catchments were almost identical, at around 45 y old (). Trees were thinned in both 2002–2004 and 2006–2007 in IJR after the acidification/N saturation phenomena (Sase et al. Citation2019), whereas this process was performed just once in 2002 in KJK (Sase et al. Citation2021). The thinning appears to have contributed to a recovery from acidification/N saturation in the 2000s in IJR and a tentative enhancement of the plant N uptake until 2004/2005 in KJK. A widespread maturation of trees may accelerate N saturation within forest ecosystems (Chiwa et al. Citation2012). In general, the thinning of trees may also enhance N uptake in plantations (Carlyle Citation1994). However, this phenomenon may depend on the condition and time scale. Chiwa et al. (Citation2020) reported that the thinning of trees did not influence annual N outputs from a N-saturated plantation forest in Japan in the short term. In IJR and KJK, the thinning of trees and declining atmospheric N deposition appear to have synergistically contributed to suppressing N leaching for several years, although this effect disappeared after a few years in the latter catchment. The high percentage of Japanese cedar in KJK (see ) appears to have also contributed to high N leaching, as suggested by other studies (Nishina et al. Citation2017; Watanabe et al. Citation2018; Yang and Chiwa Citation2021). The factors mentioned above may affect utilization efficiency of Nr species derived from the atmospheric deposition in plant-soil systems. Similar alterations of the biogeochemical N cycle were detected using the ∆17O analysis of NO3– (Tsunogai et al. Citation2014). Based on the research in IJR and KJK, reductions in the biological assimilation rates of NO3– in forest soils, rather than increased nitrification rates, were suggested as being responsible for the increase in the stream NO3– concentration (Nakagawa et al. Citation2018). Thus, forest conditions, such as tree growth rate and dominance of Japanese cedar trees, likely influenced N leaching from these forest catchments.
3.3. Changes in N leaching from the ecosystems
Relationships between N leaching from the ecosystems and atmospheric N deposition (input) are shown for annual mean NO3– concentrations in stream water and annual N outputs from the ecosystems in and 4b, respectively (following EANET Citation2021; Sase et al. Citation2019, Citation2021). To show changing trends dynamically, 3-y moving averages are plotted for several periods. The NO3– concentrations in IJR declined with reductions of the atmospheric N inputs, whereas those in KJK increased (). The N outputs in IJR declined first but stabilized in the middle/late-2010s, whereas those in KJK gradually increased (). Finally, the N outputs in both catchments approached the 1:1 line.
Figure 4. Relationships (a) between the atmospheric N inputs and NO3– concentrations in stream water and (b) between the atmospheric N inputs and N outputs from ecosystems in Lake Ijira catchment (IJR) and Kajikawa catchment (KJK) (EANET Citation2021). Three-year-moving averages are plotted for several periods to show chronological changes. Water year (WY) in IJR, from November to October in the next year, e.g., WY 2019 in IJR, from November 2018 to October 2019 (after Sase et al. Citation2019); WY in KJK, from June to May in the next year, e.g., WY 2018 in KJK, from June 2018 to May 2019 (after Sase et al. Citation2021).
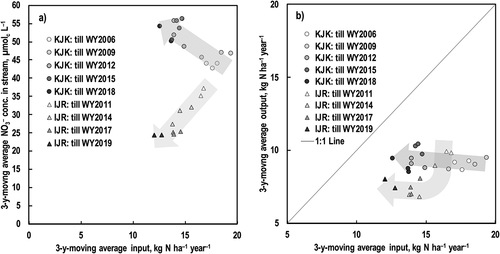
The NO3– concentrations in stream water and N outputs are largely influenced by the stream water discharge, although both the inputs and outputs may have uncertainty. A recent increase in precipitation amounts (often over 3,000 mm) in IJR and gradual declining precipitation amounts in KJK (see ) appear to have influenced the N outputs in these catchments. Owing to the monsoon climate with warm and wet summers, N leaching is controlled by hydrological processes in Asia (Ohte et al. Citation2001; Fang et al. Citation2011). NO3– concentrations in stream water significantly increased with the water discharge during rain events in IJR (Sase et al. Citation2019) and KJK (Kamisako et al. Citation2008). Moreover, changes in precipitation amounts/patterns owing to climate change, including extreme rain events (e.g., Shimpo et al. Citation2019) and/or snowfall changes (e.g., Kawase et al. Citation2020), have recently been suggested. Therefore, the effects of stream water discharge rates on NO3– concentrations should be carefully considered through accounting for possible alterations of hydrological processes caused by climate change. However, the current N inputs remain high, at over 10 kg ha–1 year–1, which is higher than the threshold values observed in previous studies; Fang et al. (Citation2011) pointed out that an increase in N leaching occurred above 5 kg ha–1 year–1 in forest catchments in China, while the threshold for forests in Europe and the United States was approximately 10 kg ha–1 year–1. The ecosystems may not mitigate effects from changing forest conditions and climate change under high N inputs.
3.4. Necessity of further studies
There are several limitations of N saturation studies in forest catchments. First, atmospheric N depositions in KJK estimated via the TF+SF method may have large uncertainties because of canopy interactions (such as uptake) and microbial N alteration during field storage; however, the method is useful for estimates of S deposition (Sase et al. Citation2008). In IJR, fluxes from wet and dry depositions have been estimated since 2007 using a wet-only sampler and the inferential method (Sase et al. Citation2019). Ideally, a similar approach should be applied to forest catchments, including KJK, for longer time. Moreover, atmospheric deposition of organic N (probably organic nitrate) should be considered to estimate N inputs more precisely (Fang et al. Citation2011), as we focused on DIN deposition in our previous studies. Researchers have also suggested that forests may simultaneously become receptors of atmospheric deposition as well as emission sources of NH3 (e.g., Hansen et al. Citation2017) and NOy (as NO + NO2 + HONO; e.g., Mushinski et al. Citation2019). Bidirectional fluxes of both reduced and oxidized Nr species should be investigated in forests to estimate the actual N budget between the atmosphere and ecosystems. Moreover, N export fluxes from denitrification should also be considered for N budgets on a catchment scale (Fang et al. Citation2015).
Long-term data on atmospheric deposition and stream water chemistry can be accumulated in these catchments. However, because of budget/manpower restrictions, data on soil and forest vegetation could not be continuously collected in this study. Because the data on tree growth and soil chemical properties were in accordance with the progress of N saturation in IJR (Nakahara et al. Citation2010), further studies on relationships between plants, soils, and atmospheric deposition may be necessary for the recovery processes in cooperation with relevant scientific communities studying forests, soils, and hydrology.
We have previously suggested that alterations of hydrological processes caused by climate change may influence recovery processes from acidification (Sase et al. Citation2019, Citation2021). Accordingly, extreme weather events, such as heavy rain events, accelerated N leaching from ecosystems in both IJR and KJK. Therefore, we suggest that the effects of extreme weather events on N leaching processes be carefully monitored.
4. Conclusion
In this study, we evaluated the status of N saturation in forest catchments in central Japan based on the atmospheric deposition (input) and the stream water discharge (output). Relatively long-term datasets over 15 y allowed us to discuss changes in N leaching with atmospheric N deposition in these forest catchments. Two forest catchments in central Japan, IJR on the Pacific side and KJK on the Sea of Japan side, have experienced both domestic and transboundary air pollution. The recent reduction of atmospheric deposition contributed to recovery from acidification in both forest catchments. However, N leaching in KJK continues to increase with the maturation of a Japanese cedar plantation. IJR continues to recover from N saturation, but its annual N outputs have slightly increased because of the recent increase in precipitation amounts. Forest conditions and changing climates appeared to control N leaching. Moreover, the current atmospheric DIN deposition amounts are still high, at over 10 kg ha–1 year–1, which will impede the catchments’ recovery from N saturation. To assess whether or not forest catchments could recover smoothly from N saturation, forest conditions and changing climatic conditions should be continuously monitored, together with atmospheric DIN deposition.
Acknowledgments
The study in IJR was conducted based on monitoring data from the Ministry of the Environment of Japan and their related research outputs. The study in KJK was conducted as one of the Network Center’s research activities for Acid Deposition Monitoring Network in East Asia (EANET). We are also grateful to the local government of Niigata Prefecture and the previous and current land managers, namely Mr. Kohei Funayama, Mr. Takeo Funayama, and Mr. Takanori Funayama, for their permission and assistance to use the selected forested area in KJK as a study site. We thank all officers, experts, and scientists in all relevant organizations.
Disclosure statement
No potential conflict of interest was reported by the author(s).
Additional information
Funding
References
- Aber, J. D., W. McDowell, K. J. Nadelhoffer, A. Magill, G. Berntson, M. Kamakea, S. McNulty, W. Gurrie, L. Rusted, and I. Fernandez. 1998. “Nitrogen Saturation in Temperate Forest Ecosystems.” BioScience 48 (11): 921–934. doi:https://doi.org/10.2307/1313296.
- Aber, J. D., K. J. Nadelhoffer, P. Steudler, and J. M. Melillo. 1989. “Nitrogen Saturation in Northern Forest Ecosystems.” BioScience 39 (6): 378–386. doi:https://doi.org/10.2307/1311067.
- Baba, M., M. Okazaki, K. Matsuda, E. Shima, F. Tachiyanagi, T. Sugiura, and K. Toyota. 2020. “Decreases in Inorganic Nitrogen Inputs and Effects on Nitrogen Saturation and Soil Acidification.” Journal of Forest Research 25 (1): 31–40. doi:https://doi.org/10.1080/13416979.2019.1705019.
- Carlyle, J. C. 1994. “Opportunities for Managing Nitrogen Uptake in Established Pinus Radiata Plantations on Sandy Soils.” New Zealand Journal of Forestry Science 24: 344–361.
- Chiwa, M., H. Haga, T. Kasahara, M. Tateishi, T. Saito, H. Kato, K. Otsuki, and Y. Onda. 2020. “Effect of Forest Thinning on Hydrologic Nitrate Exports from a N-saturated Plantation.” Journal of Forestry Research 31 (2): 387–395. doi:https://doi.org/10.1007/s11676-018-0784-5.
- Chiwa, M., N. Onikura, J. Ide, and A. Kume. 2012. “Impact of N-saturated Upland Forests on Downstream N Pollution in the Tatara River Basin, Japan.” Ecosystems 15 (2): 230–241. doi:https://doi.org/10.1007/s10021-011-9505-z.
- Chiwa, M., R. Tateno, T. Hishi, and H. Shibata. 2019. “Nitrate Leaching from Japanese Temperate Forest Ecosystems in Response to Elevated Atmospheric N Deposition.” Journal of Forest Research 24 (1): 1–15. doi:https://doi.org/10.1080/13416979.2018.1530082.
- EANET. 2010a. Technical Manual for Inland Aquatic Environment Monitoring in East Asia-2010. Niigata, Japan: Network Center for the EANET, Asia Center for Air Pollution Research.
- EANET. 2010b. Technical Manual for Wet Deposition Monitoring in East Asia-2010. Niigata, Japan: Network Center for the EANET, Asia Center for Air Pollution Research.
- EANET. 2013. Technical Manual on Dry Deposition Flux Estimation in East Asia. Niigata, Japan: Network Center for the EANET, Asia Center for Air Pollution Research.
- EANET. 2020. Data Report 2019. Network Center for the Acid Deposition Monitoring Network in East Asia (EANET). Niigata, Japan: Asia Center for Air Pollution Research.
- EANET. 2021. The Fourth Periodic Report of the State of Acid Deposition. Niigata, Japan: Network Center for the Acid Deposition Monitoring Network in East Asia (EANET), Asia Center for Air Pollution Research.
- Endo, T., H. Yagoh, K. Sato, K. Matsuda, K. Hayashi, I. Noguchi, and K. Sawada. 2011. “Regional Characteristics of Dry Deposition of Sulfur and Nitrogen Compounds at EANET Sites in Japan from 2003 to 2008.” Atmospheric Environment 45 (6): 1259–1267. doi:https://doi.org/10.1016/j.atmosenv.2010.12.003.
- Fang, Y., P. Gundersen, R. D. Vogt, K. Koba, F. Chen, X. Y. Chen, and M. Yoh. 2011. “Atmospheric Deposition and Leaching of Nitrogen in Chinese Forest Ecosystems.” Journal of Forest Research 16 (5): 341–350. doi:https://doi.org/10.1007/s10310-011-0267-4.
- Fang, Y., K. Koba, A. Makabe, C. Takahashi, W. Zhu, T. Hayashi, A. Hokari, et al. 2015. “Microbial Denitrification Dominates Nitrate Losses from Forest Ecosystems.” Proceedings of the National Academy of Sciences 112: 1470–1474. doi:https://doi.org/10.1073/pnas.1416776112.
- Fenn, M. E., M. A. Poth, J. D. Aber, J. S. Baron, B. T. Bormann, D. W. Johnson, A. D. Lemly, S. G. McNulty, D. F. Ryan, and R. Stottlemyer. 1998. “Nitrogen Excess in North American Ecosystems: Predisposing Factors, Ecosystem Responses, and Management Strategies.” Ecological Applications 8 (3): 706–733. doi:https://doi.org/10.1890/1051-0761(1998)008[0706:NEINAE]2.0.CO;2.
- Galloway, J. N., J. D. Aber, J. W. Erisman, S. P. Seitzinger, R. W. Howarth, E. B. Cowling, and B. J. Cosby. 2003. “The Nitrogen Cascade.” BioScience 53 (4): 341–356. doi:https://doi.org/10.1641/0006-3568(2003)053[0341:TNC]2.0.CO;2.
- Grennfelt, P., and H. Hultberg. 1986. “Effects of Nitrogen Deposition on the Acidification of Terrestrial and Aquatic Ecosystems.” Water, Air, and Soil Pollution 30 (3–4): 945–963. doi:https://doi.org/10.1007/978-94-009-3385-9_96.
- Hansen, K., E. Personne, C. A. Skjøth, B. Loubet, A. Ibrom, R. Jensen, L. L. Sørensen, and E. Bøgh. 2017. “Investigating Sources of Measured Forest-atmosphere Ammonia Fluxes Using Two-layer Bi-directional Modelling.” Agricultural and Forest Meteorology 237: 80–94. doi:https://doi.org/10.1016/j.agrformet.2017.02.008.
- Inomata, Y., T. Ohizumi, T. Saito, M. Morohashi, N. Yamashita, M. Takahashi, H. Sase, et al. 2019. “Estimate of Transboundary Transported Anthropogenic Sulfate Deposition in Japan by Using the Sulfur Isotopic Ratio.” Science of the Total Environment 691 :779–788. doi:https://doi.org/10.1016/j.scitotenv.2019.07.004.
- Ishida, T., I. Tayasu, and C. Takenaka. 2015. “Characterization of Sulfur Deposition over the Period of Industrialization in Japan Using Sulfur Isotope Ratio in Japanese Cedar Tree Rings Taken from Stumps.” Environmental Monitoring and Assessment 187 (7): 459. doi:https://doi.org/10.1007/s10661-015-4678-0.
- Kamisako, M., H. Sase, T. Matsui, H. Suzuki, A. Takahashi, T. Oida, M. Nakata, T. Totsuka, and H. Ueda. 2008. “Seasonal and Annual Fluxes of Inorganic Constituents in a Small Catchment of a Japanese Cedar Forest near the Sea of Japan.” Water, Air, and Soil Pollution 195 (1–4): 51–61. doi:https://doi.org/10.1007/s11270-008-9726-8.
- Kawase, H., T. Yamazaki, S. Sugimoto, T. Sasai, R. Ito, T. Hamada, M. Kuribayashi, et al. 2020. “Changes in Extremely Heavy and Light Snow-cover Winters Due to Global Warming over High Mountainous Areas in Central Japan.” Progress in Earth and Planetary Science 7 (1): 10. doi:https://doi.org/10.1186/s40645-020-0322-x.
- Kurita, H., and H. Ueda. 2006. “Long Term Decrease of pH of River and Lake Water in the Upper-most Stream Part of the Mountainous Region in Central Japan – Decrease of pH in past 30 Years in Relation with Acid Rain.” Journal of Japan Society for Atmospheric Environment 41 (2): 45–64. In Japanese with English summary. doi:https://doi.org/10.11298/taiki1995.41.2_45.
- Kurokawa, J., and T. Ohara. 2020. “Long-term Historical Trends in Air Pollutant Emissions in Asia: Regional Emission Inventory in ASia (REAS) Version 3.” Atmospheric Chemistry and Physics 20 (21): 12761–12793. doi:https://doi.org/10.5194/acp-20-12761-2020.
- Kurokawa, J., T. Ohara, T. Morikawa, S. Hanayama, G. Janssens-Maenhout, T. Fukui, K. Kawashima, and H. Akimoto. 2013. “Emissions of Air Pollutants and Greenhouse Gases over Asian Regions during 2000–2008: Regional Emission Inventory in Asia (REAS) Version 2.” Atmospheric Chemistry and Physics 13 (21): 11019–11058. doi:https://doi.org/10.5194/acp-13-11019-2013.
- Lu, Z., Q. Zhang, and D. G. Streets. 2011. “Sulfur Dioxide and Primary Carbonaceous Aerosol Emission in China and India, 1996-2010.” Atmospheric Chemistry and Physics 11 (18): 9839–9864. doi:https://doi.org/10.5194/acp-11-9839-2011.
- Matsubara, H., S. Morimoto, H. Sase, T. Ohizumi, H. Sumida, M. Nakata, and H. Ueda. 2009. “Long-term Declining Trends in River Water pH in Central Japan.” Water, Air, and Soil Pollution 200 (1–4): 253–265. doi:https://doi.org/10.1007/s11270-008-9909-3.
- McNulty, S. G., J. D. Aber, and S. D. Newman. 1996. “Nitrogen Saturation in a High Elevation New England Spruce-fir Stand.” Forest Ecology and Management 84 (1–3): 109–121. doi:https://doi.org/10.1016/0378-1127(96)03742-5.
- Mushinski, R. M., R. P. Phillips, Z. C. Payne, R. B. Abney, I. Jo, S. Fei, S. E. Pusedef, J. R. White, D. B. Rusch, and J. D. Raff. 2019. “Microbial Mechanisms and Ecosystem Flux Estimation for Aerobic NOy Emissions from Deciduous Forest Soils.” Proceedings of the National Academy of Sciences 116( 6): 2138–2145. doi:https://doi.org/10.1073/pnas.1814632116.
- Nakagawa, F., U. Tsunogai, Y. Obata, K. Ando, N. Yamashita, T. Saito, S. Uchiyama, M. Morohashi, and H. Sase. 2018. “Export Flux of Unprocessed Atmospheric Nitrate from Temperate Forested Catchments: A Possible New Index for Nitrogen Saturation.” Biogeosciences 15 (22): 7025–7042. doi:https://doi.org/10.5194/bg-15-7025-2018.
- Nakahara, O., M. Takahashi, H. Sase, T. Yamada, K. Matsuda, T. Ohizumi, H. Fukuhara, et al. 2010. “Soil and Stream Water Acidification in a Forested Catchment in Central Japan.” Biogeochemistry 97 (2–3): 141–158. doi:https://doi.org/10.1007/s10533-009-9362-4.
- Nishina, K., M. Watanabe, M. K. Koshikawa, T. Takamatsu, Y. Morino, T. Nagashima, K. Soma, and S. Hayashi. 2017. “Varying Sensitivity of Mountainous Streamwater Base-flow NO3– Concentrations to N Deposition in the Northern Suburbs of Tokyo.” Scientific Reports 7: 7701. doi:https://doi.org/10.1038/s41598-017-08111-w.
- Ohizumi, T., N. Take, Y. Inomata, H. Yagoh, T. Endo, M. Takahashi, K. Yanahara, and M. Kusakabe. 2016. “Long-term Variation of the Source of Sulfate Deposition in a Leeward Area of Asian Continent in View of Sulfur Isotopic Composition.” Atmospheric Environment 140: 42–51. doi:https://doi.org/10.1016/j.atmosenv.2016.05.057.
- Ohte, N., M. J. Mitchell, H. Shibata, N. Tokuchi, H. Toda, and G. Iwatsubo. 2001. “Comparative Evaluation on Nitrogen Saturation of Forest Catchments in Japan and Northeastern United States.” Water, Air, and Soil Pollution 130 (1/4): 649–654. doi:https://doi.org/10.1023/A:1013804728336.
- Rockström, J., W. Steffen, K. Noone, Å. Persson, I. I. I. F. S. Chapin, E. F. Lambin, T. M. Lenton, et al. 2009. “A Safe Operating Space for Humanity.” Nature 461 (7263): 472–475. doi:https://doi.org/10.1038/461472a.
- Sase, H., K. Matsuda, T. Visaratana, H. Garivait, N. Yamashita, B. Kietvuttinon, B. Hongthong, et al. 2012. “Deposition Process of Sulfate and Elemental Carbon in Japanese and Thai Forests.” Asian Journal of Atmospheric Environment 6 (4): 246–258. doi:https://doi.org/10.5572/ajae.2012.6.4.246.
- Sase, H., T. Saito, M. Takahashi, M. Morohashi, N. Yamashita, Y. Inomata, T. Ohizumi, and M. Nakata. 2021. “Transboundary Air Pollution Reduction Rapidly Reflected in Stream Water Chemistry in Forested Catchment on the Sea of Japan Coast in Central Japan.” Atmospheric Environment 248: 118223. doi:https://doi.org/10.1016/j.atmosenv.2021.118223.
- Sase, H., A. Takahashi, M. Sato, H. Kobayashi, M. Nakata, and T. Totsuka. 2008. “Seasonal Variation in the Atmospheric Deposition of Inorganic Constituents and Canopy Interactions in a Japanese Cedar Forest.” Environmental Pollution 152 (1): 1–10. doi:https://doi.org/10.1016/j.envpol.2007.06.023.
- Sase, H., M. Takahashi, K. Matsuda, K. Sato, T. Tanikawa, N. Yamashita, T. Ohizumi, et al. 2019. “Response of River Water Chemistry to Changing Atmospheric Environment and Sulfur Dynamics in a Forested Catchment in Central Japan.” Biogeochemistry 142 (3): 357–374. doi:https://doi.org/10.1007/s10533-019-00540-1.
- Shimpo, A., K. Takemura, S. Wakamatsu, H. Togawa, Y. Mochizuki, M. Takekawa, S. Tanaka, et al. 2019. “Primary Factors behind the Heavy Rain Event of July 2018 and the Subsequent Heat Wave in Japan.” SOLA 15A: 13–18. doi:https://doi.org/10.2151/sola.15A-003.
- Skeffington, R. A., and E. J. Wilson. 1988. “Excess Nitrogen Deposition: Issues for Consideration.” Environmental Pollution 54 (3–4): 159–184. doi:https://doi.org/10.1016/0269-7491(88)90110-8.
- Smith, S. J., J. Van Aardenne, Z. Klimont, R. J. Andres, A. Volke, and S. Delgado Arias. 2011. “Anthropogenic Sulfur Dioxide Emissions: 1850-2005.” Atmospheric Chemistry and Physics 11 (3): 1101–1116. doi:https://doi.org/10.5194/acp-11-1101-2011.
- Tsunogai, U., D. D. Komatsu, T. Ohyama, A. Suzuki, F. Nakagawa, I. Noguchi, K. Takagi, M. Nomura, K. Fukuzawa, and H. Shibata. 2014. “Quantifying the Effects of Clear-cutting and Strip-cutting on Nitrate Dynamics in a Forested Watershed Using Triple Oxygen Isotopes as Tracers.” Biogeosciences 11: 5411–5424. doi:https://doi.org/10.5194/bg-11-5411-2014.
- Watanabe, M. S., M. S. Hasegawa, M. K. Koshikawa, T. Takamatsu, A. Kohzu, A. Imai, and S. Hayashi. 2018. “Coniferous Coverage as Well as Catchment Steepness Influences Local Stream Nitrate Concentrations within a Nitrogen-saturated Forest in Central Japan.” Science of the Total Environment 636: 539–546. doi:https://doi.org/10.1016/j.scitotenv.2018.04.307.
- Wright, R. F., J. G. M. Roelofs, M. Bredemeier, K. Blanck, A. W. Boxman, B. A. Emmett, P. Gundersen, et al. 1995. “NITREX: Responses of Coniferous Forest Ecosystems to Experimentally Changed Deposition of Nitrogen.” Forest Ecology and Management 71 (1–2): 163–169. doi:https://doi.org/10.1016/0378-1127(94)06093-X.
- Yamada, T., T. Inoue, H. Fukuhara, O. Nakahara, T. Izuta, R. Suda, M. Takahashi, et al. 2007. “Long-term Trend in Surface Water Quality of Five Lakes in Japan.” Water, Air, and Soil Pollution: Focus 7 :259–266. doi:https://doi.org/10.1007/s11267-006-9076-8.
- Yamashita, N., H. Sase, T. Ohizumi, J. Kurokawa, T. Ohara, Y. Morino, M. Kuribayashi, et al. 2016. “Mapping the Relative Risk of Surface Water Acidification Based on Cumulative Acid Deposition over the past 25 Years in Japan.” Journal of Forest Research 21 (3): 115–124. doi:https://doi.org/10.1007/s10310-016-0523-8.
- Yang, R., and M. Chiwa. 2021. “Low Nitrogen Retention in a Japanese Cedar Plantation in a Suburban Area, Western Japan.” Scientific Reports 11 (1): 5335. doi:https://doi.org/10.1038/s41598-021-84753-1.