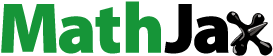
ABSTRACT
This study investigated the long-term (31 years) effects of mineral slag fertilizer (fused magnesium phosphate [FMgP] and calcium silicate [CaSi]) application on changes in the main soil chemical properties, including pH, electrical conductivity (EC), available phosphorus (P), soil organic carbon (SOC), and total nitrogen (TN) contents in paddy soils. Soil samples were obtained from six fertilizer treatments [(1) Nitrogen, phosphorus, and potassium chemical fertilizer only (CF); (2) CF + mineral slag fertilizer (CF+); (3) CF + rice straw (RS); (4) CF + rice straw + mineral slag fertilizer (RS+); (5) CF + rice straw compost (CM); and (6) NPK + rice straw compost + mineral slag fertilizer (CM+)] at five depth soils (0–5, 5–10, 10–15, 15–20, and 20–25 cm). An anaerobic incubation experiment was performed at 30°C to determine carbon (C) decomposition and nitrogen (N) mineralization potentials at every 2-week interval (0, 2, 4, 6, and 8 weeks). The results indicated that compared to non-mineral slag additions, both EC and available P increased by 36.6–136.6% in mineral slag addition treatments. Still, only compost addition mostly influenced pH values; meanwhile, SOC and TN exhibited an increase (~23.8%) in compost addition treatments but a reduction (~12.7%) in rice straw treatments by applying mineral slag fertilizer. Mineral slag addition reduced the C decomposition potential but enhanced N mineralization potential observed by the first-order reaction kinetic models. Our study suggested that mineral slag fertilizers affected the soil organic matter and its mineralization potentials.
1. Introduction
Long-term experiments (LTEs) in continuous-cropping systems with various agronomic management practices provide a helpful method to determine ecosystem’s productivity and sustainability and verify how environmental factors influence agroecosystems and future global climate change (Rasmussen, Albrecht, and Smiley Citation1998). LTEs can provide full observation of dynamics of soil properties, composition, and function across time scales for decades to further evaluate soil fertility and productivity and to predict the responses of soil carbon (C) to future climate change (Richter et al. Citation2007; Hopkins et al. Citation2009).
Changes in soil properties, such as pH, electrical conductivity (EC), available phosphorus (P), soil organic carbon (SOC), total nitrogen (TN) affected by different long-term inorganic fertilizers, and organic matter management practices have been reported in numerous studies (Smith et al. Citation1997; Grant et al. Citation2001; Blair et al. Citation2006; Dimassi et al. Citation2013; Kumar et al. Citation2014; Cheng et al. Citation2016). Meanwhile, several long-term studies on continuous lowland rice cropping (twice or three times per year) were reported from the Philippines (Pampolino et al. Citation2008), China (Dong et al. Citation2012; Yan, Wang, and Yang Citation2007), and India (Mandal et al. Citation2007; Nayak et al. Citation2008; Tripathi et al. Citation2014) in humid–subtropical regions. Additionally, there are LTEs on rice, and upland crop systems are in the same regions; however, most of the data generated are limited to the trends of grain yield (Dawe et al. Citation2000; Yadav, Dwivedi, and Pandey Citation2000; Tirol-Padre et al. Citation2007; Mao et al. Citation2015). In temperate regions, there are many single rice LTEs conducted in Japan; however, few reports are regarded in the changes in pH, EC, available P, SOC, and TN from single rice LTEs in the temperate regions. Moreover, it is unclear whether the long-term applications of CF combined with mineral slag fertilizers or organic matter can affect C sequestration and nitrogen (N) balance in temperate rice paddies.
Mineral slag fertilizers have wide applications in crops (Grzebisz Citation2013; Chand, Paul, and Kumar Citation2015; Das et al. Citation2019). For example, magnesium fertilizers can enhance the yields of potato, sugar beet (Orlovius and McHoul Citation2015), and wheat (Ceylan et al. Citation2016). Calcium silicate slag improved 23–25% yields of rice and sugarcane (Anderson, Jones, and Snyder Citation1987). A recent meta-analysis of 570 pair observations showed that magnesium fertilization enhanced crop yield in most production systems (Wang et al. Citation2019). Mineral slag fertilizers enhanced root growth, pH value and nutrient supply (Das et al. Citation2019). For example, Ca, Mg, and SiO2 slags can increase mineral CO2 sequestration, and Fe slag mitigates CH4 and N2O emissions (Furukawa and Inubushi Citation2002; Inubushi Citation2021). Calcium silicate helps sugarcane against foliar disease (Raid, Anderson, and Ulloa Citation1992) and rice against drought stress (Yang, Howe, and Golden Citation2018); meanwhile, some mineral fertilizers, such as Magnesium have been harmful to soil ammonium for many crop plants several decades ago (Mulder Citation1956). Several studies have analyzed the effects of fused magnesium phosphate and slag silicate fertilizer on soil quality and crop production in Japan (Nakagawa, Kitamoto, and Tange Citation1955; Sasaki et al. Citation2013, Citation2014). For example, Sasaki et al. (Citation2014) showed that the application of slag silicate could enhance rice plant biomass; however, the effects differed from field to field. Additionally, Sasaki et al. (Citation2013) suggested that Si supply in soil extract increased with the application rate of silicate slag fertilizer. Despite several studies on mineral slag fertilizers on crops, there are rare reports on the long-term effects of mineral slag fertilizer on the chemical properties of paddy soils, especially the interaction of slag fertilizer with inorganic fertilizer (chemical fertilizers) or organic matter (like rice straw and compost) in the paddy field. Therefore, this study aimed to investigate the changes in soil pH, EC, available P, SOC, and TN contents and their C decomposition and N mineralization potentials after long-term (31 years) applications of two types of mineral slag fertilizers (FMgP [fused magnesium phosphate] and CaSi [calcium silicate slag]) with organic and inorganic fertilizer applications in a single rice paddy in the cold temperate regions of Japan.
2. Materials and methods
2.1. Site location and experimental design
The LTE field in this study was located at the Yamagata Integrated Agricultural Research Center, Yamagata, Japan (38°15ʹN, 140°15ʹE). More information on LTE was well described in our previous studies (Cheng et al. Citation2016, Citation2017; Nguyen-Sy et al. Citation2020). In this study, soil samples were obtained from six plots of 100 m2 under different fertilization: [(1) Nitrogen, phosphorus, and potassium chemical fertilizer only (CF); (2) CF + mineral slag fertilizer (CF+); (3) CF + rice straw (RS); (4) CF + rice straw + mineral slag fertilizer (RS+); (5) CF + rice straw compost (CM); and (6) NPK + rice straw compost + mineral slag fertilizer (CM+)]. More detailed information on fertilizer application is illustrated in .
Table 1. Details of applications of inorganic fertilizers, organic matter and mineral slags in six treatments
Rice straw was left yearly on the soil surface at 6 ton·ha−1 after harvest and during the following winter in both RS and RS+ treatments. The rice straw was removed after harvest leaving only the stubbles in the CF, CF+, CM, and CM+ treatments. The rice straw compost was incorporated into the CM and CM+ treatments at 10 ton·ha−1. Rice straw and rice straw compost were incorporated into the plowed layer (0–15 cm) during land preparation. Mineral slag fertilizers and NPK fertilizers were broadcast before soil puddling and rice transplanting, respectively. Average C and N contents and their application rates in each plot were well described in our previous study (Cheng et al. Citation2016).
2.2. Soil sampling and analysis
Soil samples were obtained in November 2012, after 31 years of continuous rice cultivation. The sampling depth of the soil was 25 cm, where rice roots were mainly observed. Nine soil cores were obtained from each of the six plots and three cores were thoroughly mixed to make up one composite sample for soil analysis. Three samples were then obtained for each treatment plot. All soil samples were air-dried, sieved (<2 mm), and stored under the same conditions (at room temperature) for further analysis.
Air-dried soil was used to measure the SOC and TN contents by dry combustion using a CN-900 Analyzer (Sumika Chemical Analysis Service, Japan). Soil pH and EC were measured in a 1:2.5 (soil/water ratio, w/v) mixture using a glass electrode pH meter (D-51, Horiba, Kyoto, Japan) and an EC meter (Cond-meter DS-51, Horiba, Kyoto, Japan). The available P was measured by the Truog method extracted using 0.002 N H2SO4 solutions.
2.3. Anaerobic incubation experiment
Anaerobic incubation was performed to investigate the soil C decomposition and N mineralization. Each soil sample (5-g on an oven-dried basis) was weighed into a 68-mL serum bottle and amended with 10-mL distilled water. Each bottle was capped with a butyl rubber stopper with an aluminum seal, and the air inside was replaced with pure N2 gas. The soil samples in the sealed bottles were incubated at 30°C. After 2, 4, 6, and 8 weeks of incubation, soil samples were removed from the incubator to measure CO2 and CH4 production. The CO2 and CH4 concentrations in the headspace of each bottle were deduced by gas chromatography using GC-8A and GC-2014 (Shimadzu, Kyoto, Japan) with thermal conductivity detector and flame ionization detector, respectively. The C decomposition was approximated from the sum of CO2 and CH4 production. After the gases were measured, the incubated soil was immediately extracted using a 20-ml 10% KCl solution by shaking for 30 min on a reciprocal shaker. The N mineralization was calculated as the amount of ammonium-nitrogen (NH4+-N) in the soil after anaerobic incubation minus the amount of NH4+-N in air-dried soil. The NO3–N in air-dried soil was denitrified to N2 during anaerobic incubation, and there was no NO3–N in the submerged soil during anaerobic incubation.
2.4. Data calculations and model analysis
Since there were no plot replications for each treatment, we did not obtain statistical analysis in this paper. All data from the three pseudo-replicates were used only for calculating the average value for each treatment. Data for total 0–25 cm depth in all treatments was calculated from each of the five layers by arithmetic mean. SigmaPlot for Windows v. 10 was used to generate the C decomposition (CO2 + CH4) time curve by the first-order reaction model and to approximate the C decomposition potential (C0) as EquationEquation (1)(1)
(1) , which was well discussed by (Cheng et al. Citation2016; Kautsar et al. Citation2020).
where Dec C was the decomposed C as the sum of CO2 and CH4 production. Co was the amount of C decomposition potential (g C·kg−1). Kc was the C decomposition rate constant (wk−1) and t was the incubation time (wk).
While the N mineralization time curve was obtained from the first-order reaction model to calculate the N mineralization potential (No) as described by EquationEquation (2)(2)
(2) :
where Min N was mineralized N as the net NH4+-N production. No was the amount of N mineralization potential (g N·kg−1). Kn was the N mineralization rate constant (wk−1), and t was the incubation time (wk).
3. Results
3.1. Changes in soil pH and EC
Soils that were applied with rice straw (RS and RS+) and compost (CM and CM+) had lower pH and higher EC than soil samples in chemical fertilizer treatments (CF and CF+). In comparing pH values in treatments with and without mineral slag addition, only CF+ exhibited higher pH values than CF. However, RS and CM with mineral slag addition had similar pH values in the corresponding treatment without slag addition ()). Except for the 20–25 cm layer, there were no clear changes in pH among the top layers from 0–5 to 15–20 cm ()). For those of EC, all mineral slag fertilizer addition treatments had higher EC values than non-mineral slag treatments. Interestingly, the EC had similar trends for all mineral and non-mineral slag addition treatments in the order of CF < RS < CM and CF + < RS + < CM + ()). Except for 20–25 cm layer, there were higher EC values in the layer from 10 to 20 cm than 0–10 cm for all mineral slag addition treatments ()).
3.2. Changes in soil-available P
All mineral slag addition treatments had higher available P concentration (46.5−55.8 mg·kg−1) in all soil layers than the corresponding treatments without mineral slag addition ranging from 20.6 to 32 mg kg−1 ( and (b)). For non-mineral slag addition treatments, both RS and CM application treatments had higher available P than the CF treatment. However, for the mineral slag addition treatments, the mean available P concentration was in the order of CM+ > CF+ > RS+ in 0‒25 cm soil depth. Mostly, the available P concentration decreased with soil depths ()). Unexpectedly, CF+ had the highest available P concentration in 0–5 soil depth and had similar available P concentration with RS+ in both 5‒10 cm and 15‒20 cm soil depths.
3.3. Changes in SOC and TN contents
No changes occurred in SOC and TN contents in chemical fertilizer treatments with (CF+) and without mineral slag (CF) addition. For the non-mineral slag addition shift, the total amount of SOC increased by 21.0% and 10.8%, while TN increased 19.7% and 10.6% in RS and CM, respectively, relative to the CF treatment. However, mineral slag addition reduced SOC and TN contents of 13.2% and 12.2% in rice straw amendment treatments (RS versus RS+) and an increase of 23.2% and 24.3% in compost addition treatments (CM versus CM+), respectively () and 5(a)). Meanwhile, SOC of mineral slag addition treatments increased by 3.9% and 33.9%, while TN increased by 1.6% and 33.5% in RS+ and CM+, respectively, to the CF+ treatment () and 5(a)). The highest SOC and TN at 0 − 25 cm soil depth were observed in CM+ treatments that supplied 512-kg·ha−1 year−1 organic C and 39.5-kg·ha−1·year−1 organic N from 10-ton·ha−1 compost, plus FMgP and CaSi (0.3 and 1.5 Mg·ha−1). Conversely, the lowest SOC and TN induced CF and CF+ treatments, which did not supply rice straw or compost. In all treatments, the SOC and TN contents decreased with soil depth () and 5(b)). Compared to non-mineral slag addition, the mineral slag addition increased the SOC and TN rates (C/N), with the highest C/N in RS+ ()). Except for 20–25 cm layer, C/N increased with soil depth in all treatments ()).
3.4. C decomposition and N mineralization potentials
The changes in C decomposition and N mineralization in total 0–25 cm layer were fitted with the first-order reaction model (); however, the values of Co,No,Kc, and Kn varied among six treatments (). The value of Co was higher in rice straw treatments (RS and RS+) and lower in chemical fertilizer treatments (CF and CF+). The Co ratio of non-mineral/mineral slag addition was >1.0, indicating that slag addition reduced C decomposition potentials. Conversely, those of No ratio <1 indicated that mineral slag additions enhanced nitrogen mineralization potential. The Co in non-mineral slag addition treatments (CF, RS, and CM) was higher than those of mineral slag addition treatments (CF+, RS+, and CM+).
Table 2. The best parameters obtained from the 8-week anaerobic incubation experiment at 30°C for measuring the C decomposition potential (Co) and N mineralization potential (No) for the soil samples from different long-term inorganic fertilizer application treatments at 0−25 cm soil depth. The curves were simulated using the first-order reaction model as
The C decomposition rate (Kc) ranged from 0.377 to 0.814 wk−1, which showed the highest in CM treatment and lowest in RS+ treatments, while Kn ranged from 0.365 to 0.685 wk−1, which showed the lowest in CF+ and highest in CM treatment. The Co/No and Kc/Kn ranged from 3.16 to 4.82 and from 0.75 to 1.39, respectively (). The ratio of Co/No is lower than the C/N ratio of bulk soil, which ranged from 13.3 to 13.6.
4. Discussion
4.1. The effect of long-term mineral slags additions on soil pH, EC, and available P
As expected, pH decreased with the rice straw and rice straw compost applications in this study. Long-term application of organic matter was proposed to produce organic acid, and as a result, decreases the pH (Dong et al. Citation2012; Cheng et al. Citation2016). However, in this study, mineral slag addition enhanced pH in CF and CM treatments rather than RS treatments (). Previous studies indicated that mineral slag applications (including Mg, Ca, and Si fertilizers) greatly impacted soil pH in several crops (Chand, Paul, and Kumar Citation2015; Das et al. Citation2019; Gwon et al. Citation2018). For example, the application of Mg fertilizer also increased soil pH after 4–12 weeks compared to non-mineral slag fertilizer application treatments in corn-growing experiments (Härdter, Rex, and Orlovius Citation2004). Similarly, Sasaki et al. (Citation2013) suggested that silicate slightly enhanced soil pH (0–0.2 unit) in nine soil types in Yamagata Prefecture, Japan. There was a positive correlation between labile Si and pH. Two inversed factors mainly regulated the changes in pH in our experiment: the enhancement caused by FMgP and CaSi and the decline induced by organic matter application. In CF and CF+ treatments, the rice straw was removed, and only stubble remained in the paddy field, resulting in the pH being more affected by FMgP and CaSi. Interestingly, there was no difference in the pH between RS and RS+; however, since the optimal pH for soil microorganism activity has been ranging from 5–8 in most agricultural soils (Smith and Doran Citation1996), we can speculate that long-term application of mineral slag could play an essential role in preventing soil pH decline.
Meanwhile, the changes in EC are more pronounced than in pH; therefore, it is reasonable that soil EC depicts the salt concentration of the soil and can be naturally increased using slag application rates. Additionally, rice straw and rice straw compost applications played similarly essential roles in mineral absorption storage in soil, making its higher EC values more than non-mineral slag addition treatments. Our result agreed with previous studies (Cheng et al. Citation2016; Das et al. Citation2019), confirming that soil EC increased by rice straw, compost applications, and slag addition (Si, Ca, P) could enhance nutrient supply. As the slag fertilizers contain P (FMgP), it is logical that the available P concentration in CF+, RS+, and CM+ treatments are considered to be higher than those in CF, RS, and CM treatments. Moreover, the P in compost application is more available than in conventional CF as reported (Sikora and Enkiri Citation2003). In this experiment, long-term compost application of >30 years led to an increase in available P. This result conformed with that of Ghosh et al. (Citation2012), who reported that organic amendment also enhances soil nutrients such as available P. Alternatively, Anderson, Snyder, and Warren (Citation2008) reported that available P was unlikely to be influenced by the addition of calcium silicate slag, the increase in available P by mineral slag additions in this experiment should be contributed by FMgP, not by CaSi.
4.2. The effect of long-term mineral slag additions on SOC and TN contents
The changes in SOC and TN contents were similar among all treatments; however, the changed patterns were different from EC and the available P in this study. Compared to chemical fertilizer treatments (CF and CF+), rice straw (RS and RS+) and compost applications (CM and CM+) exhibited an increase in SOC and TN contents with the highest in CM+ treatment. The effects of long-term exogenous organic matter applications on the improvement of SOC and TN contents and compositions were reported in rice paddy ecosystems (Röing, Andrén, and Mattsson Citation2005; Tong et al. Citation2009; Zhang et al. Citation2012; Cheng et al. Citation2016, Citation2017; Takakai et al. Citation2020a, Citation2020b). Nguyen et al. (Citation2020) also reported that SOC was significantly higher and TN was significantly lower in the long-term application of rice straw in rice paddies than that in cow dung compost. However, there are rarely comparative studies on the combined effects of CF, organic matter, and mineral slag additions on SOC and TN contents changes. We discovered that mineral slag additions did not alter SOC (TN) content in chemical fertilizer treatments (CF and CF+). It was argued that SOC mineralization is associated with silicate minerals and subsequent precipitation of stable carbonate minerals like Ca, Mg, and Fe slag fertilizer (Das et al. Citation2019; Wang et al. Citation2019); however, few reports focus on the effects of the mineral slag additions on carbon sequestration in cropping systems (Das et al. Citation2019). Our discovery showed that without organic matter fertilizer addition, slag fertilizers did not affect SOC (TN) content in bulk soil but reduced in rice straw addition but increased in compost addition, which could be attributed to fast decomposition of RS (compared with CM) and positive priming effect of RS-derived C decomposition induced by mineral slag additions (). Therefore, it is not advisable to incorporate slag mineral fertilizers with RS in northeastern Japan with temperate climate. Still, the co-applications of mineral slag with compost are favorable to improving soil organic matter content. The C/N ratios in all treatments remained stable with the ranges from 13.3 to 13.6 because the changes in SOC are relative to changes in TN during all treatments as addressed above.
4.3. The effect of long-term mineral slag additions on C decomposition and N mineralization
In this study, C decomposition and N mineralization potentials were obtained from 8 weeks of anaerobic incubation at 30°C. The data exhibited a high correlation between C decomposition and N mineralization potentials (Co and No), which fits well with the first-order reaction model (). The Co/No ratios varied from 3.16 to 4.82 in this study () were close to the soil microbial biomass C/N ratio in the paddy soil (Shibahara and Inubushi Citation1995). The low Co/No ratio indicated that easily decomposed organic matter was mainly obtained from soil microbial biomass and protein (Rillig et al. Citation2007; Cheng et al. Citation2016). Findings revealed that the mineral slag additions increased Co (from 6% to 43%) but relatively decreased No (from 6% to 13%) compared to non-mineral slag addition treatments (). The Kc and Kn represent the speeds of microbial C decomposition and N mineralization processes. We discovered that mineral slag addition vastly reduced both Kc and Kn in rice straw and compost addition treatments. The reduced rate constants of Kc were 80% and 108%, much higher than those changes in Kn with 39% and 32% in rice straw and compost treatments, respectively. Unexpectedly, mineral slag addditions had inconsistent effects on SOC and TN contents, as well as their decompositionability, which was probably attributed to the rapid depletion of labile C and N such as dissolve organic C and microbial biomass C during rice growth season induced by mineral slag. Therefore, more attention should be paid to the responses of in situ C decomposition and mineralization as well as microbial community in paddy fields under long-term mineral slag addition condition before flooding of each rice season.
Figure 7. The changes in carbon (C) decomposition (a) and nitrogen (N) mineralization (b) at the 0–25 cm soil depth under anaerobic incubation at 30°C for 8 weeks. Both were simulated by the first-order reaction model as .
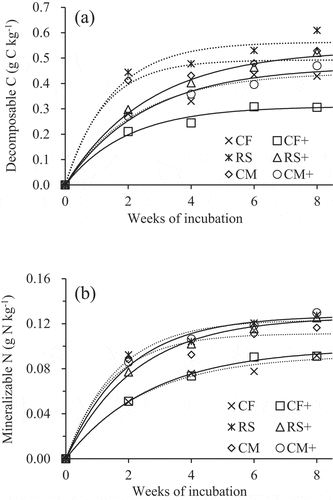
Studies on the application of a single or double first-order reaction model to estimate the N mineralization process in submerged paddy soils were reported in various studies (Inubushi, Wada, and Takai Citation1985; Ando, Aragones, and Wada Citation1992; Purwanto et al. Citation2005; Cheng et al. Citation2016; Kautsar et al. Citation2020). However, there is no report that can be used to compare the C decomposition and N mineralization as affected by mineral slag addition, especially in LTEs. Our previous study revealed that C decomposition and N mineralization were synchronous without mineral slag addition when applying rice straw and compost (Cheng et al. Citation2016). In this study, mineral slag fertilizers improved N mineralization in all pair treatments, suggesting that it is an optimum way to improve soil fertility by combining exogenous organic matter (i.e., straw and manure) application with the mineral slag fertilizers.
5. Conclusion
The 31 years of mineral slag addition changed soil properties in 0–25 cm of rice paddy soil layers. Our results revealed that pHs were unchanged by mineral slag addition in rice straw (RS versus RS+) treatments but increased in chemical fertilizer (CF versus CF+) and compost addition (CM versus CM+) treatments. However, mineral slag addition increased EC (by 36.6–78.7%) and available P (by 74.4–136.6%) compared to their pair treatments of CF, RS, and CM. The SOC and TN contents did not have a difference between CF and CF+, but slightly decreased in RS+ (11.2–13.2%) compared to RS and strongly increased in CM+ (23.4–25.0%) compared to CM treatment, respectively. Our results suggested that mineral slag addition could reduce C decomposition potential but resulting in the enhancement of N mineralization potentials. However, their C decomposition/N mineralization rates decreased as affected by mineral slag addition when applying organic matter to paddy rice systems. Therefore, results from this study can provide vital information to optimize CF and organic matter applications with the combination of mineral slag for sustaining soil fertility and for modeling C and N dynamics in paddy rice systems.
Acknowledgments
We thank Yamagata Integrated Agricultural Research Center for providing soil samples, and members of Soil Science and Plant Nutrition Lab, Faculty of Agriculture, Yamagata University for soil chemical analyses.
Disclosure statement
No potential conflict of interest was reported by the author(s).
Additional information
Funding
References
- Anderson, D. L., D. B. Jones, and G. H. Snyder. 1987. “Response of a Rice-Sugarcane Rotation to Calcium Silicate Slag on Everglades Histosolsl.” Agronomy Journal 79: 531–535. doi:https://doi.org/10.2134/agronj1987.00021962007900030026x.
- Anderson, D. L., G. H. Snyder, and J. D. Warren. 2008. “Availability of Phosphorus in Calcium Silicate Slag.” Communications in Soil Science and Plant Analysis 23 (9–10): 907–918. doi:https://doi.org/10.1080/00103629209368638.
- Ando, H., R. C. Aragones, and G. Wada. 1992. “Mineralization Pattern of Soil Organic N of Several Soils in the Tropics.” Soil Science and Plant Nutrition 38 (2): 227–234. doi:https://doi.org/10.1080/00380768.1992.10416485.
- Blair, N., R. D. Faulkner, A. R. Till, and P. R. Poulton. 2006. “Long-Term Management Impacts on Soil C, N and Physical Fertility.” Soil and Tillage Research 91 (1–2): 30–38. doi:https://doi.org/10.1016/j.still.2005.11.002.
- Ceylan, Y., U. B. Kutman, M. Mengutay, and I. Cakmak. 2016. “Magnesium Applications to Growth Medium and Foliage Affect the Starch Distribution, Increase the Grain Size and Improve the Seed Germination in Wheat.” Plant and Soil 406 (1–2): 145–156. doi:https://doi.org/10.1007/s11104-016-2871-8.
- Chand, S., B. Paul, and M. Kumar. 2015. “An Overview of Use of Linz-Donawitz (LD) Steel Slag in Agriculture.” Current World Environment 30 (33): 975–984. doi:https://doi.org/10.12944/cwe.10.3.29.
- Cheng, W., A. T. Padre, C. Sato, H. Shiono, S. Hattori, A. Kajihara, M. Aoyama, K. Tawaraya, and K. Kumagai. 2016. “Changes in the Soil C and N Contents, C Decomposition and N Mineralization Potentials in a Rice Paddy after Long-Term Application of Inorganic Fertilizers and Organic Matter.” Soil Science and Plant Nutrition 62 (2): 212–219. doi:https://doi.org/10.1080/00380768.2016.1155169.
- Cheng, W., A. T. Padre, H. Shiono, C. Sato, T. Nguyen-Sy, K. Tawaraya, and K. Kumagai. 2017. “Changes in the pH, EC, Available P, SOC and TN Stocks in a Single Rice Paddy after Long-Term Application of Inorganic Fertilizers and Organic Matters in a Cold Temperate Region of Japan.” Journal of Soils and Sediments 17 (7): 1834–1842. doi:https://doi.org/10.1007/s11368-016-1544-9.
- Das, S., G. W. Kim, H. Y. Hwang, P. P. Verma, and P. J. Kim. 2019. “Cropping with Slag to Address Soil, Environment, and Food Security.” Frontiers in Microbiology 10: 1320. doi:https://doi.org/10.3389/fmicb.2019.01320.
- Dawe, D., A. Dobermann, P. Moya, S. Abdulrachman, B. Singh, P. Lal, S. Y. Li, et al. 2000. “How Widespread are Yield Declines in Long-Term Rice Experiments in Asia?” Field Crops Research 66 (2): 175–193. doi:https://doi.org/10.1016/s0378-4290(00)00075-7.
- Dimassi, B., J. P. Cohan, J. Labreuche, and B. Mary. 2013. “Changes in Soil Carbon and Nitrogen following Tillage Conversion in a Long-Term Experiment in Northern France.” Agriculture, Ecosystems & Environment 169: 12–20. doi:https://doi.org/10.1016/j.agee.2013.01.012.
- Dong, W. Y., X. Y. Zhang, H. M. Wang, X. Q. Dai, X. M. Sun, W. W. Qiu, and F. T. Yang. 2012. “Effect of Different Fertilizer Application on the Soil Fertility of Paddy Soils in Red Soil Region of Southern China.” PLoS ONE 7 (9): e44504. doi:https://doi.org/10.1371/journal.pone.0044504.
- Furukawa, Y., and K. Inubushi. 2002. “Feasible Suppression Technique of Methane Emission from Paddy Soil by Iron Amendment.” Nutrient Cycling in Agroecosystems 64: 193–201. doi:https://doi.org/10.1023/A:1021150831735.
- Ghosh, S., B. Wilson, S. Ghoshal, N. Senapati, and B. Mandal. 2012. “Organic Amendments Influence Soil Quality and Carbon Sequestration in the Indo-Gangetic Plains of India.” Agriculture, Ecosystems & Environment 156: 134–141. doi:https://doi.org/10.1016/j.agee.2012.05.009.
- Grant, R. F., N. G. Juma, J. A. Robertson, R. C. Izaurralde, and W. B. McGill. 2001. “Long-Term Changes in Soil Carbon under Different Fertilizer, Manure, and Rotation.” Soil Science Society of America Journal 65 (6): 205–214. doi:https://doi.org/10.2136/sssaj2001.1872a.
- Grzebisz, W. 2013. “Crop Response to Magnesium Fertilization as Affected by Nitrogen Supply.” Plant and Soil 368 (1–2): 23–39. doi:https://doi.org/10.1007/s11104-012-1574-z.
- Gwon, H. S., M. I. Khan, M. A. Alam, S. Das, and P. J. Kim. 2018. “Environmental Risk Assessment of Steel-Making Slags and the Potential Use of LD Slag in Mitigating Methane Emissions and the Grain Arsenic Level in Rice (Oryza Sativa L.).” Journal of Hazardous Materials 353: 236–243. doi:https://doi.org/10.1016/j.jhazmat.2018.04.023.
- Härdter, R., M. Rex, and K. Orlovius. 2004. “Effects of Different Mg Fertilizer Sources on the Magnesium Availability in Soils.” Nutrient Cycling in Agroecosystems 70: 249–259. doi:https://doi.org/10.1007/s10705-005-0408-2.
- Hopkins, D. W., I. S. Waite, J. W. McNicol, P. R. Poulton, A. J. Macdonald, and A. G. O’Donnell. 2009. “Soil Organic Carbon Contents in Long-Term Experimental Grassland Plots in the UK (Palace Leas and Park Grass) Have Not Changed Consistently in Recent Decades.” Global Change Biology 15 (7): 1739–1754. doi:https://doi.org/10.1111/j.1365-2486.2008.01809.x.
- Inubushi, K., H. Wada, and Y. Takai. 1985. “Easily Decomposable Organic Matter in Paddy Soil (VI). Kinetics of Nitrogen Mineralization in Submerged Soils.” Soil Science and Plant Nutrition 31: 563–752. doi:https://doi.org/10.1080/00380768.1985.10557464.
- Inubushi, K. 2021. “Sustainable Soil Management in East, South and Southeast Asia.” Soil Science and Plant Nutrition 67 (1): 1–9. doi:https://doi.org/10.1080/00380768.2020.1835431.
- Kautsar, V., W. Cheng, K. Tawaraya, S. Yamada, K. Toriyama, and K. Kobayashi. 2020. “Carbon and Nitrogen Stocks and Their Mineralization Potentials are Higher under Organic than Conventional Farming Practices in Japanese Andosols.” Soil Science and Plant Nutrition 66 (1): 144–151. doi:https://doi.org/10.1080/00380768.2019.1705739.
- Kumar, S., T. Nakajima, E. G. Mbonimpa, S. Gautam, U. R. Somireddy, A. Kadono, R. Lal, R. Chintala, R. Rafique, and N. Fausey. 2014. “Long-Term Tillage and Drainage Influences on Soil Organic Carbon Dynamics, Aggregate Stability and Corn Yield.” Soil Science and Plant Nutrition 60 (1): 108–118. doi:https://doi.org/10.1080/00380768.2013.878643.
- Mandal, B., B. Majumder, P. K. Bandyopadhyay, G. C. Hazra, A. Gangopadhyay, R. N. Samantaray, A. K. Mishra, J. Chaudhury, M. N. Saha, and S. Kundu. 2007. “The Potential of Cropping Systems and Soil Amendments for Carbon Sequestration in Soils under Long-Term Experiments in Subtropical India.” Global Change Biology 13 (2): 357–369. doi:https://doi.org/10.1111/j.1365-2486.2006.01309.x.
- Mao, X. L., X. L. Xu, K. P. Lu, G. Gielen, J. F. Luo, L. Z. He, A. Donnison, et al. 2015. “Effect of 17 Years of Organic and Inorganic Fertilizer Applications on Soil Phosphorus Dynamics in a Rice–Wheat Rotation Cropping System in Eastern China.” Journal of Soils and Sediments 15 (9): 1889–1899. doi:https://doi.org/10.1007/s11368-015-1137-z.
- Mulder, E. G. 1956. “Nitrogen-Magnesium Relationships in Crop Plants.” Plant and Soil 7: 341–376. doi:https://doi.org/10.1007/BF01394322.
- Nakagawa, M., A. Kitamoto, and I. Tange. 1955. “Effect of Fused Magnesium Phosphate on Paddy Grown on ‘Akiochi’ Soil with Particular Reference to Phosphorus and Silicon.” Soil Science and Plant Nutrition 1 (1): 27–28. doi:https://doi.org/10.1080/00380768.1955.10434359.
- Nayak, P., D. Patel, B. Ramakrishnan, A. K. Mishra, and R. N. Samantaray. 2008. “Long-Term Application Effects of Chemical Fertilizer and Compost on Soil Carbon under Intensive Rice–Rice Cultivation.” Nutrient Cycling in Agroecosystems 83 (3): 259–269. doi:https://doi.org/10.1007/s10705-008-9217-8.
- Nguyen-Sy, T., W. G. Cheng, S. M. Kimani, H. Shiono, R. Sugawara, K. Tawaraya, T. Watanabe, and K. Kumagai. 2020. “Stable Carbon Isotope Ratios of Water-Extractable Organic Carbon Affected by Application of Rice Straw and Rice Straw Compost during a Long-Term Rice Experiment in Yamagata, Japan.” Soil Science and Plant Nutrition 66 (1): 125–132. doi:https://doi.org/10.1080/00380768.2019.1708209.
- Nguyen, T. T., Y. Sasaki, K. Kakuda, and H. Fujii. 2020. “Comparison of Paddy Soil Fertility under Conventional Rice Straw Application versus Cow Dung Compost Application in Mixed Crop–Livestock Systems in a Cold Temperate Region of Japan.” Soil Science and Plant Nutrition 66 (1): 106–115. doi:https://doi.org/10.1080/00380768.2019.1677445.
- Orlovius, K., and J. McHoul. 2015. “Effect of Two Magnesium Fertilizers on Leaf Magnesium Concentration, Yield, and Quality of Potato and Sugar Beet.” Journal of Plant Nutrition 38 (13): 2044–2054. doi:https://doi.org/10.1080/01904167.2014.958167.
- Pampolino, M. F., E. V. Laureles, H. C. Gines, and R. J. Buresh. 2008. “Soil Carbon and Nitrogen Changes in Long-Term Continuous Lowland Rice Cropping.” Soil Science Society of America Journal 72 (3): 798–807. doi:https://doi.org/10.2136/sssaj2006.0334.
- Purwanto, B. H., A. Watanabe, S. J. Foh, K. Kakuda, and H. Ando. 2005. “Kinetic Parameters of Gross N Mineralization of Peat Soils as Related to the Composition of Soil Organic Matter.” Soil Science and Plant Nutrition 51 (1): 109–115. doi:https://doi.org/10.1111/j.1747-0765.2005.tb00013.x.
- Raid, R. N., D. L. Anderson, and M. F. Ulloa. 1992. “Influence of Cultivar and Amendment of Soil with Calcium Silicate Slag on Foliar Disease Development and Yield of Sugar-Cane.” Crop Protection 11: 84–88. doi:https://doi.org/10.1016/0261-2194(92)90085-J.
- Rasmussen, P. E., S. L. Albrecht, and R. W. Smiley. 1998. “Soil C and N Changes under Tillage and Cropping Systems in Semi-Arid Pacific Northwest Agriculture.” Soil and Tillage Research 47 (3–4): 197–205. doi:https://doi.org/10.1016/s0167-1987(98)00106-8.
- Richter, D. D., M. Hofmockel, M. A. Callaham, D. S. Powlson, and P. Smith. 2007. “Long-Term Soil Experiments: Keys to Managing Earth’s Rapidly Changing Ecosystems.” Soil Science Society of America Journal 71 (2): 266–279. doi:https://doi.org/10.2136/sssaj2006.0181.
- Rillig, M. C., B. A. Caldwell, H. A. B. Wösten, and P. Sollins. 2007. “Role of Proteins in Soil Carbon and Nitrogen Storage: Controls on Persistence.” Biogeochemistry 85 (1): 25–44. doi:https://doi.org/10.1007/s10533-007-9102-6.
- Röing, K., O. Andrén, and L. Mattsson. 2005. “Long-Term Management Effects on Plant N Uptake and Topsoil Carbon Levels in Swedish Long-Term Field Experiments: Cereals and Ley, Crop Residue Treatment and Fertilizer N Application.” Acta Agriculturae Scandinavica 55 (1): 16–22. doi:https://doi.org/10.1080/09064710510008739.
- Sasaki, S. M., K. Kakuda, Y. Sasaki, and H. Ando. 2013. “Effect of Slag Silicate Fertilizer on Dissolved Silicon in Soil Solution Based on the Chemical Properties of Gleysols.” Soil Science and Plant Nutrition 59 (2): 271–277. doi:https://doi.org/10.1080/00380768.2012.763022.
- Sasaki, S. M., K. Kakuda, Y. Sasaki, and H. Ando. 2014. “Effects of Slag Silicate Fertilizer on Silicon Content of Rice Plants Grown in Paddy Fields on the Shounai Plain, Yamagata, Japan.” Soil Science and Plant Nutrition 60 (5): 708–721. doi:https://doi.org/10.1080/00380768.2014.936305.
- Shibahara, F., and K. Inubushi. 1995. “Measurements of Microbial Biomass C and N in Paddy Soils by the Fumigation-Extraction Method.” Soil Science and Plant Nutrition 41 (4): 681–689. doi:https://doi.org/10.1080/00380768.1995.10417018.
- Sikora, L. J., and N. K. Enkiri. 2003. “Availability of Poultry Litter Compost P to Fescue Compared with Triple Super Phosphate.” Soil Science 168 (3): 192–199. doi:https://doi.org/10.1097/01.ss.0000058891.60072.35.
- Smith, J. L., and J. W. Doran. 1996. Measurement and Use of pH and Electrical Conductivity for Soil Quality Analysis. SSSA Special Publications: Soil Science Society of America, USA. doi: https://doi.org/10.2136/sssaspecpub49.c10.
- Smith, P., J. U. Smith, D. S. Powlson, W. B. McGill, J. R. M. Arah, O. G. Chertov, K. Coleman, et al. 1997. “A Comparison of the Performance of Nine Soil Organic Matter Models Using Datasets from Seven Long-Term Experiments.” Geoderma 81 (1–2): 153–225. doi:https://doi.org/10.1016/s0016-7061(97)00087-6.
- Takakai, F., K. Hatakeyama, M. Nishida, O. Nagata, T. Sato, and Y. Kaneta. 2020b. “Effect of the Long-term Application of Organic Matter on Soil Carbon Accumulation and GHG Emissions from a Rice Paddy Field in a Cool-temperate Region, Japan-II. Effect of Different Compost Applications.” Soil Science and Plant Nutrition 66 (1): 96–105. doi:https://doi.org/10.1080/00380768.2019.1681881.
- Takakai, F., Y. Kominami, S. Ohno, and O. Nagata. 2020a. “Effect of the Long-term Application of Organic Matter on Soil Carbon Accumulation and GHG Emissions from a Rice Paddy Field in a Cool-temperate Region, Japan. I. Comparison of Rice Straw and Rice Straw Compost.” Soil Science and Plant Nutrition 66 (1): 84–95. doi:https://doi.org/10.1080/00380768.2019.1609335.
- Tirol-Padre, A., J. K. Ladha, A. P. Regmi, A. L. Bhandari, and K. Inubushi. 2007. “Organic Amendments Affect Soil Parameters in Two Long-Term Rice-Wheat Experiments.” Soil Science Society of America Journal 71 (2): 442–452. doi:https://doi.org/10.2136/sssaj2006.0141.
- Tong, C. L., H. A. Xiao, G. Y. Tang, H. Q. Wang, T. P. Huang, H. A. Xia, S. J. Keith, Y. Li, S. L. Liu, and J. S. Wu. 2009. “Long-Term Fertilizer Effects on Organic Carbon and Total Nitrogen and Coupling Relationships of C and N in Paddy Soils in Subtropical China.” Soil and Tillage Research 106 (1): 8–14. doi:https://doi.org/10.1016/j.still.2009.09.003.
- Tripathi, R., A. K. Nayak, P. Bhattacharyya, A. K. Shukla, M. Shahid, R. Raja, B. B. Panda, S. Mohanty, A. Kumar, and V. K. Thilagam. 2014. “Soil Aggregation and Distribution of Carbon and Nitrogen in Different Fractions after 41 Years Long-Term Fertilizer Experiment in Tropical Rice–Rice System.” Geoderma 213: 280–286. doi:https://doi.org/10.1016/j.geoderma.2013.08.031.
- Wang, Z., M. U. Hassan, F. Nadeem, L. Wu, F. Zhang, and X. Li. 2019. “Magnesium Fertilization Improves Crop Yield in Most Production Systems: A Meta-Analysis.” Frontier in Plant Science 10: 1727. doi:https://doi.org/10.3389/fpls.2019.01727.
- Yadav, R. L., B. S. Dwivedi, and P. S. Pandey. 2000. “Rice-Wheat Cropping System: Assessment of Sustainability under Green Manuring and Chemical Fertilizer Inputs.” Field Crops Research 65 (1): 15–30. doi:https://doi.org/10.1016/s0378-4290(99)00066-0.
- Yan, D. Z., D. J. Wang, and L. Z. Yang. 2007. “Long-Term Effect of Chemical Fertilizer, Straw, and Manure on Labile Organic Matter Fractions in a Paddy Soil.” Biology and Fertility of Soils 44 (1): 93–101. doi:https://doi.org/10.1007/s00374-007-0183-0.
- Yang, R., J. A. Howe, and B. R. Golden. 2018. “Calcium Silicate Slag Reduces Drought Stress in Rice (Oryza Sativa L.).” Journal of Agronomy and Crop Science 205 (4): 353–361. doi:https://doi.org/10.1111/jac.12327.
- Zhang, W. J., M. G. Xu, X. J. Wang, Q. H. Huang, J. Nie, Z. Z. Li, S. L. Li, S. W. Hwang, and K. B. Lee. 2012. “Effects of Organic Amendments on Soil Carbon Sequestration in Paddy Fields of Subtropical China.” Journal of Soils and Sediments 12 (4): 457–470. doi:https://doi.org/10.1007/s11368-011-0467-8.