ABSTRACT
Microplastics cause environmental problems. Biodegradable plastics have become popular because they aim to avoid such problems. However, their decomposition in the soil may have an impact. This study aims to investigate the effects of biodegradable plastics on the physicochemical properties of soil, specifically the production of CO2 and N2O in the soil and plant growth. Three kinds of biodegradable plastics in the forms of 1) nonwoven fabric sheets made of poly-lactic acid (PLA) and polybutylene-succinate (referred to hereafter as fabric), 2) laminate sheets made of polybutylene adipate terephthalate (PBAT) and pulp (hereafter laminate), and 3) drinking cups made of PLA (hereafter cup), were cut into small pieces (<5 mm), added to soil, then water-holding capacity was determined and incubated aerobically for 4 weeks at 30°C in the dark. Soil and gas samples were collected weekly to measure soil pH, nitrate-nitrogen content, CO2, and N2O productions. These plastics were also tested in a pot experiment with Komatsuna (Brassica napa var. perviridis). We tested for seed germination, plant growth, leaf color, and fresh weight at harvest. Results showed that the water retention capacity was higher in the fabric plastics as compared to the cup plastics and the control. Soil pH with the fabric plastics dropped during the initial 2 weeks of incubation, then recovered to a similar pH to the control (without plastic). Nitrate contents in the soil with laminate plastics were lower than those in the control, while CO2 production in the soil with the laminate plastics was higher than that in the control and the other plastics during the incubation period, and even higher than the one of added plastic-C. N2O was produced rapidly within 1 week of incubation in the soil with the laminate plastics, and cumulative N2O production from incubation was more than that of the control. The seed germination and plant growth tended to be suppressed in the pot experiment with the fabric and laminate plastics. The results indicate that the influence of these biodegradable plastics on soil properties, greenhouse gas production, and plant growth on the kind of plastic and the timing.
1. Introduction
Microplastics may cause environmental problems such as oceanic pollution and damage the health of marine animals (Gregory Citation1978). Biodegradable plastics have become popular, aiming to avoid such problems (Boots, Russell, and Green Citation2019). In agriculture, mulching sheets made of biodegradable plastics have become popular in Japan, and their rapid decomposition is possible because of microorganisms in the soil (NARO Citation2015). Some sanitary items such as nappies are also made of biodegradable plastics, aiming for faster decomposition in the soil after they become garbage and are dumped as land-fill or burnt. However, the decomposition of plastics in the soil may significantly impact its physical (de Souza Machado et al. Citation2018) and chemical properties (Lozano et al. Citation2021). Bi, He, and Chen (Citation2020) reported that biodegradable plastics do not influence carbon (C) and nitrogen (N) metabolisms, while Iqbal et al. (Citation2020) described a high potential risk of biodegradable plastics on nitrogen metabolisms in the soil that had been amended with them. However, little information is available for their impact on greenhouse gases such as carbon dioxide (CO2) and nitrous oxide (N2O) or their effect on plant growth (Boots, Russell, and Green Citation2019).
Amongst several greenhouse gases, CO2 and N2O are two major constituents. On the one hand, the terrestrial ecosystem is an important sink, with contributors such as forest soils that can sequester CO2. Conversely, agricultural soils are sources of N2O derived mainly from nitrogen fertilizer and manure. N2O has about 300 times higher an effect on global warming potential in its molar basis than CO2. N2O also has a destructive impact on the stratospheric ozone layer (IPCC Citation2021). This study aims to investigate the effects of biodegradable plastics on soil physicochemical properties, the production of CO2 and N2O in soil, and their effects on plant growth.
2. Materials and methods
2.1. Soil and plastics
A sandy soil (Coase-Medium Texture Gray Lowland soil) was collected from an upland field in Yokoshiba-Hikari, in the Pacific coastal region of Chiba Prefecture, Japan, with the following properties; pH(H2O): 6.78, electric conductivity (EC): 3.86 mS m−1 measured as decribed below, total carbon and nitrogen (TC and TN, measured by CN Corder; Yanaco MT700) at 9.83 and 0.90 g kg−1 NH4+ and NO3−, 0.31 and 2.75 mg N kg−1, CEC at 1.90 cmol(+) kg−1, glucosidase and urease activities at 32.7 mg p-nitrophenol kg−1 h−1 and 19.4 mg NH4+-N kg−1 h−1, determined by Eivazi and Tabatabai (Citation1988) and Tabatabai and Bremner (Citation1972), respectively. Three kinds of biodegradable plastics were amended to the soil; 1) nonwoven fabric sheets (abbreviated hereafter as fabric) made of polylactic acid (PLA) and polybutylene-succinate (PBS), 2) laminate sheets (abbreviated hereafter as laminate) made of polybutylene adipate terephthalate (PBAT) and pulp, and 3) drinking cups (abbreviated hereafter as cup) made of PLA.
2.2. Incubation experiment
The plastics were cut into small pieces (<5 mm) by hand, then mixed well into the soil at 0.2% w/w for 1) fabric and 2) laminate, 2.0% w/w for 3) cup, and 0% (no amendment) as the control. These samples (50 g each) were incubated aerobically in containers (250 mL) for 4 weeks with 50% water-holding capacity at 30℃ in the dark with 3 replicates.
2.3. Soil analysis
Water holding capacity (WHC) of the plastic-soil mixture was determined by the Hilgard method (The University of Tokyo Citation1978); the plastic-soil mixture was placed on filter paper inside a Hilgard metal dish (diameter = 5.6 cm, depth = 1 cm, volume = 25 mL) and set inside a Petri dish with shallow distilled water to moisten the sample for 1 h, then calculated based on the weight before and after this period as:
WHC = Water added to the soil sample (mL) – Water unretained in the soil sample (mL) 1 hr after water addition]/weight of oven-dried soil (d.s.)
Soil pH (H2O), and electric conductivity (EC) were measured periodically by pH/EC meter (Horiba, D-62/TOA, CM-31P), ammonium N and nitrate N contents by colorimetric methods (Anderson and Ingram Citation1989; Hayashi, Sakamoto, and Yoshida Citation1997, respectively), and cation exchange capacity (CEC) by batch extraction (Muramoto, Goto, and Ninaki Citation1992) with atomic absorption meter (Tokou Electric, ANA-182) for CEC. Another set of samples (10 g each) was incubated aerobically in a glass vial (120 mL) with a sponge stopper and was analyzed in the same way. The gas samples in the headspaces of the vials were collected on days 1, 5, 7, 14, and 28, 24 h after changing to butyl rubber stoppers to determine the gas production of CO2 and N2O concentrations by gas chromatography (GC) (Shimadzu, GC-14B), with a thermal conductivity detector (TCD) and an electron capture detector (ECD), respectively. Details of GC parameters are based on Inubushi, Wada, and Takai (Citation1984) and Inubushi, Naganuma, and Kitahara (Citation1996).
The C decomposition rate was calculated with:
[(CO2 from the plastic amended soil) – (CO2 from the control soil)]/Plastic-C
Plastic C was the assumed weight-based of the plastic C content at 51% for the fabric and laminate treatments and 48% for the cup treatment, based on the chemical formula of each compound as a raw material, respectively.
The N2O proximal emission factor was calculated as
[(N2O from the plastic amended soil) – (N2O from the control soil)]/ [(nitrate N of the amended soil) – (nitrate N of the control soil)] * 100
Since the examined plastics contained no nitrogen, we calculated this as above to estimate their impact on N2O emission, based on the influenced soil N dynamics caused by their addition.
2.4. Germination test and pot experiment
Germination test was conducted using 3 mL of plastic-water extract (1:10 ratio) and 15 seeds of Komatsuna (Brassica rapa var. perviridis) placed on filter paper inside a Petri dish with 4 replicates and incubated in a growth chamber at 20°C for 1 week under a continuously dark condition. The germination rate was calculated at the end of the incubation compared to control (distilled water) as 100%.
Another soil-plastic mixture set (200 g each) of 4 replicates was prepared for a pot experiment in the same way as the incubation experiment (2.2) and put into pots (350 mL each). Six Komatsuna seeds were planted in each pot and initially incubated for 10 days in a growth chamber at 25°C under light/dark conditions (16/8 hour cycle), watering to maintain the same moisture condition as the incubation experiment. Then the seedlings were thinned to 3 plants per pot and placed in a glass greenhouse at Chiba University’s Matsudo campus under natural light and temperature conditions during October-November, 2020 until harvest at the 4th week.
Plant height and leaf color were measured by ruler and color meter (Konica-Minolta, SPAD-502) during greenhouse cultivation and on days 21 and 28, respectively. After harvest, ammonium and nitrate N contents remaining in the soil were measured in an incubation experiment. The fresh weight of the harvested plants was also measured after removing excess water by paper towel, and the dry weight was taken after drying in an oven at 80°C for 48 hours, respectively.
2.5. Statistical analysis
All statistical analyses comparing measured parameters among all the treatments and their temporal changes were carried out in IBM SPSS Statistics 24. Multi-reference was examined by Tukey test. Differences were considered significant at a p < 0.05 significance level.
3. Results
3.1. Water holding capacity (WHC)
WHC was not significantly different among all the treatments, although it tended to be slightly higher (6%) in the fabric treatment compared to the control ().
3.2. Soil pH and EC
Soil pH with the fabric dropped about 0.5 points during the initial 2 weeks of the incubation, then recovered to almost the same pH as control (without plastic) by the end of the incubation (). Similarly, soil pH in the laminate treatment dropped about 0.4 points in the 2nd week of incubation, then recovered to a similar pH as the control by the end of incubation.
Figure 2. Changes in soil pH during incubation Error bar = S.D. (n = 3), different letters indicate significant difference (p < 0.05, Tukey).
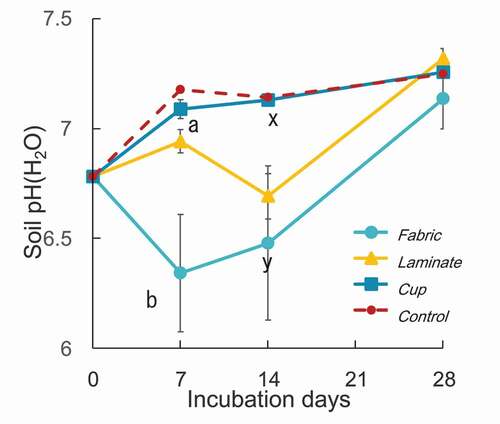
Soil EC increased gradually but similarly among the treatments during the incubation period (Figure S1).
3.3. Soil nitrate and ammonium
Nitrate content in the soil with the laminate treatment decreased to about half the level of the initial content within the first week of incubation. It remained lower than the control and other treatments until the end of the incubation period, while that of the fabric treatment increased to almost double in the 1st week and then decreased to the initial level afterward (). On the other hand, the nitrate content in the cup treatment increased to 8.7 mg N kg−1, almost 3-times higher than the initial level, and similarly to the control, throughout the incubation.
Figure 3. Changes in nitrate contents during incubation. Error bar = S.D. (n = 3), different letters indicate significant difference (p < 0.05, Tukey).
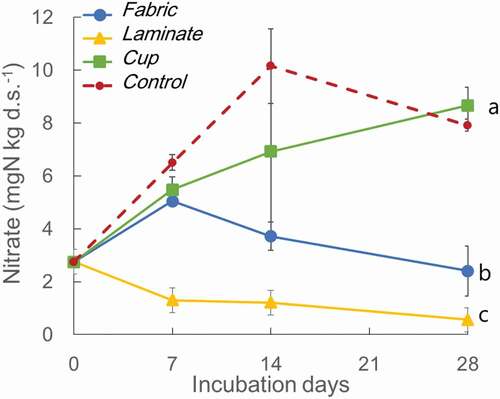
The ammonium content remained about one order of magnitude lower than the nitrate content, decreasing from about 0.31 to 0.17–0.20 mg N kg−1 during the 1st week of incubation and gradually increasing to its initial level of 0.28–0.36 mg N kg−1 by the end of the incubation period (Figure S2).
3.4. CO2 and N2O production
CO2 production increased almost constantly, that in the laminate treatment being more than 3-times higher than that of the other treatments and control during the incubation period (). There was no significant difference among the other treatments and the control.
Figure 4. Changes in cumulative CO2 production during incubation. Error bar = S.D. (n = 3), different letters indicate significant difference (p < 0.05, Tukey).
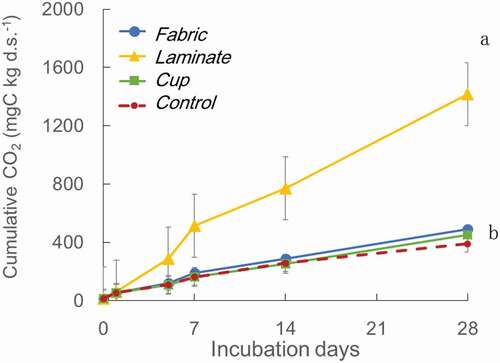
N2O increased rapidly in the laminate-treated soil within the first week of the incubation, even with no measurement on day 1. On the other hand, gradual increases were observed in the fabric treatment in the 2nd week of the incubation and in the soil containing the cup treatment and the control after the 2nd week. Cumulative N2O production by the end of the incubation was more than double in the fabric treatment compared to that of the other plastics and the control ().
3.5. Seed germination and plant growth
Seed germination tended to be less in the fabric and laminate treatments, although these had a greater variation (). Plant height increased almost constantly at the same growth rate, except with the laminate treatment, in which it stopped after the 2nd week ().
Figure 7. Changes in plant height. Error bar = S.D. (n = 3), different letters indicate significant difference (p < 0.05, Tukey).
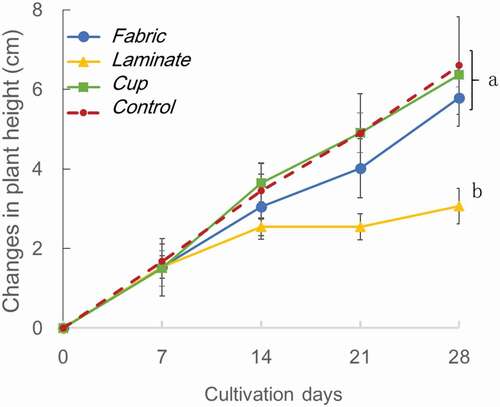
Fresh weight and dry weight were lowest with the laminate treatment, followed by the fabric treatment, while there was no significant difference between the cup treatment and the control (). There was no significance among the amount of remaining mineral N (sum of ammonium N and nitrate N) in the soil after harvest (Figure S3). At the same time, root length tended to be shorter with the laminate-treated soil (Figure S4), although no significant difference was observed in leaf color, except in the laminate treatment, which had insufficient leaf area to measure color (Figures S5, S6).
4. Discussion
4.1. Effects of plastics on soil properties
Higher water holding capacity with the fabric plastics than the cup plastics, even 10 times higher application rate than other plastics, and the control was probably due to physical properties of the fabric materials and sheet structure, similar to the laminate, although not significantly different (). This finding was similar to de Souza Machado et al. (Citation2018), who found no change with plastics. In contrast, Lozano et al. (Citation2021) reported that micro-plastics increased soil aggregation by ~18% and soil pH by ~4%, so further study is needed with more materials.
In our study, a temporal drop of soil pH by 8 ~ 10% occurred with the fabric and laminate plastic treatments (). We deduce that the decomposition of the amended plastics into things such as organic acids may have caused it, but those components were not tested. Both of those plastics had a much wider surface area than the cup plastics, despite the higher application rate of cups, and their effect on soil pH was not detected for in this incubation experiment. The temporal drop in soil pH may have inhibited N transformations such as N mineralization, nitrification, and immobilization. We did not measure nitrite, however, as the ammonium levels did not change among the treatments; nitrite oxidation may have been inhibited by the laminate and fabric treatments during incubation.
4.2. Effects of plastics on CO2 and N2O production
CO2 production in soil with the laminate plastics was much higher than that in the control and the other treatments during the incubation period, which may have been due to the effect of the laminate plastics on soil pH.
The C decomposition rate of plastics in the soil was calculated at 49%, 102%, and 4.5% for the fabric, laminate, and cup treatments, respectively. This difference may have been due to their composition, particularly that of laminate plastics, which contain pulp, mainly cellulose. Lozano et al. (Citation2021) reported that microplastic fibers impact soil enzymes (β-glucosidase, β-glucosaminidase, β-D-cellobiosidase, and phosphatase) and respiration. However, we measured soil enzyme activities such as glucosidase and urease activities only before the experiment; therefore, further investigation is necessary to measure enzymatic activity in the soil-plastic mixtures. Another possibility may be due to the effect of the amended C and their decomposition products in enhancing soil C decomposition, in a so-called “Priming effect” (Fontaine and Abbadie Citation2003). This may relate to the initial drop of soil pH in the disturbed soil, affecting the decomposition of the soil organic matter, especially with the laminate plastics.
N2O production was also high in the soil with the laminate plastics in the initial incubation stage, probably also due to the initial drop of soil pH. Since the examined plastic did not contain N, the side-effects of the amended plastic on N was estimated to be an N2O proximal emission factor, based on the 4.5–17% changes in nitrate for the fabric and laminate, respectively.
In general, N2O is produced in soil mainly through nitrification or denitrification and nitrification is a dominant process in aerobic soils (Yoshida and Alexander Citation1970). In this experiment, N2O is asumeed to be produced mostly through nitrification under aerobic conditions, although denitrification may also be possible due to high incubation temperature with C-rich plastics. Nitrification is carried out by specific soil microorganisms, namely ammonium oxidizers and nitrite oxidizers, while denitrification by rather general heterotphic bacteria and some fungi. N2O is produced in the balance and activities of these microorganisms (Inubushi and Yashima Citation2021). If nitrification is inhibited under stressed conditions such as high salinity, then N2O production increases (Inubushi, Barahona, and Yamakawa Citation1999). Amended plastic might create stress conditions during N metabolism, as observed by the drop of soil pH or by other intermediate products. Further investigation including the corresponding microorganisms is needed for more detailed examinations. In this study, methane (CH4) was not measured, as its production is dominant under anaerobic conditions. However, the future examination of CH4 and N2O production through denitrification could be considered, owing to the C-rich amendments.
4.3. Effects of plastics on seed germination and plant growth
Seed germination tended to be partially inhibited by the fabric and laminate plastics, which also suppressed the initial growth. Lower pH and other intermediates can influence germination and initial plant growth, especially the roots’ sensitive development and activities. Tanaka (Citation2002) reported that germination of rice (Oryza sativa L.) seedlings was inhibited by aliphatic acids and aromatic acids during the decomposition of wheat straw in flooded paddy soil. Therefore, the cause of changes in soil pH, such as organic acids or other compounds, should be investigated in further studies.
Boots, Russell, and Green (Citation2019) reported that microplastics derived from biodegradable PLA decreased soil pH by 0.6 points, similar to our results, but only measured once at the end of the 30-day experiment with no data about temporal changes. They also showed the suppression of biodegradable PLA on the germination and growth of perennial ryegrass (Lolium perenne), again similar to our results, and divulged other effects on ecosystem functions such as earthworm activity in the soil. Generally, plant growth is also influenced by nutrient supplies from the soil, such as soil N, but so far we could not detect significant changes of mineral N remained after harvest (Figure S3). However, there is no other long-term study so far with various plants. Thus, further long-term studies with plants other than Komatsuna are necessary.
5. Conclusion
These results from our incubation and pot experiments indicate marked influences of biodegradable plastics on soil chemical and biological properties depending on the kind of plastic and the period and timing during the investigation. These effects included greenhouse gas production, related microorganisms and changes in plant growth – even temporal, such as with an initial pH drop in the soil, N2O production, and inhibitions in plant growth. For healthy soil and ecosystems, further long-term experiments with these aspects, other forms of plastic, and different plants are required.
Supplemental Material
Download MS Word (2.3 MB)Disclosure statement
No potential conflict of interest was reported by the author(s).
Supplementary material
Supplemental data for this article can be accessed here.
Additional information
Funding
References
- Anderson, J. M., and J. S. I. Ingram. 1989. “Colorimetric Determination of Ammonium.” In Tropical Soil Biology and Fertility, edited by ISSS, 42–43. Wallingford, UK: CAB International.
- Bi, M., Q. He, and Y. Chen. 2020. “What Roles are Terrestrial Plants Playing in Global Microplastic Cycling?” Environmental Science & Technology 54: 5325–5327. doi:https://doi.org/10.1021/acs.est.0c01009.
- Boots, B., C. W. Russell, and D. S. Green. 2019. “Effects of Microplastics in Soil Ecosystems: Above and below Ground.” Environmental Science & Technology 53: 11496–11506. doi:https://doi.org/10.1021/acs.est.9b03304.
- de Souza Machado, A. A., C. W. Lau, W. Kloas, J. Bergmann, J. B. Bachelier, E. Faltin, R. Becker, A. S. Görlich, and M. C. Rillig. 2018. “Microplastics Can Change Soil Properties and Affect Plant Performance.” Environmental Science & Technology 53: 6044–6052. doi:https://doi.org/10.1021/acs.est.9b01339.
- Eivazi, F., and M. A. Tabatabai. 1988. “Glucosidases and Galactosidases in Soils.” Soil Biology & Biochemistry 20 (5): 601–606. doi:https://doi.org/10.1016/0038-0717(88)90141-1.
- Fontaine, S., and L. Abbadie. 2003. “The Priming Effect of Organic Matter: A Question of Microbial Competition?” Soil Biology & Biochemistry 35 (6): 837–843. doi:https://doi.org/10.1016/S0038-0717(03)00123-8.
- Gregory, M. R. 1978. “Accumulation and Distribution of Virgin Plastic Granules on New Zealand Beaches.” New Zealand Journal of Marine and Freshwater Research 12: 399–414. doi:https://doi.org/10.1080/00288330.1978.9515768.
- Hayashi, A., K. Sakamoto, and T. Yoshida. 1997. “A Rapid Method for Determination of Nitrate in Soil by Hydrazine Reduction Procedure.” Japanese Journal of Soil Science and Plant Nutrition 68 (3): 322–326. doi:https://doi.org/10.20710/dojo.68.3_322.
- Inubushi, K., M. A. Barahona, and K. Yamakawa. 1999. “Effect of Salts and Moisture Content on N2O Emission and Nitrogen Dynamics in Yellow Soil and Andosol in Model Experiments.” Biology and Fertility of Soils 29 (4): 401–407. doi:https://doi.org/10.1007/s003740050571.
- Inubushi, K., H. Naganuma, and S. Kitahara. 1996. “Contribution of Denitrification, Autotrophic and Heterotrophic Nitrification in Nitrous Oxide Production in Andosols.” Biology and Fertility of Soils 23 (3): 292–298. doi:https://doi.org/10.1007/s003740050173.
- Inubushi, K., H. Wada, and Y. Takai. 1984. “Easily Decomposable Organic Matter in Paddy Soil, (IV) Relationship between Reduction Process and Organic Matter Decomposition.” Soil Science and Plant Nutrition 30 (2): 189–198. doi:https://doi.org/10.1080/00380768.1984.10434682.
- Inubushi, K., and M. M. Yashima. 2021. “Mitigation of Climate Change by Nitrogen Managements in Agriculture.” In Nitrogen in Agriculture, Physiological, Agricultural and Ecological Aspects, edited by T. Ohyama and K. Inubushi, 3–14. London, UK: IntechOpen.
- IPCC. 2021. ”Climate Change 2021: The Physical Science Basis. Contribution of Working Group 1 to the Sixth Assessment Report of Intergovernmental Panel on Climate Change, AR6.” Cambridge University Press, Cambridge, United Kingdom. https://www.ipcc.ch/report/sixth-assessment-report-working-group-i/
- Iqbal, S., J. Xu, S. D. Allen, S. Khan, S. Nadir, M. S. Arif, and T. Yasmeen. 2020. “Unraveling Consequences of Soil Micro- and Nano-Plastic Pollution on Soil-Plant System: Implications for Nitrogen (N) Cycling and Soil Microbial Activity.” Chemosphere 260: 127578. doi:https://doi.org/10.1016/j.chemosphere.2020.127578.
- Lozano, Y. M., C. A. Aguilar-Trigueros, G. Onandia, S. Maas, T. Zhao, and M. C. Rillig. 2021. “Effects of Microplastics and Drought on Soil Ecosystem Functions and Multifunctionality.” Journal of Applied Ecology 58: 988–996.
- Muramoto, J., I. Goto, and M. Ninaki. 1992. “Application of Inductively Coupled Plasma Atomic Emission Spectrometry (ICP-AES) to Soil Analysis (Part 6) Rapid Analysis of Exchangeable Cations and Cation Exchange Capacity (CEC) of Soils by a Shaking Extraction Method.” Japanese Journal of Soil Science and Plant Nutrition 63: 210–215. doi:https://doi.org/10.20710/dojo.63.2_210.
- NARO. 2015. ”Biodegradable Plastic Mulch Sheet Can Be Decomposed by Soil Microorganisms, Main Research Achievements in Annual Report of NIAES, NARO.” (in Japanese). https://www.naro.affrc.go.jp/archive/niaes/sinfo/result/result32/result32_52.html
- Tabatabai, M. A., and J. M. Bremner. 1972. “Assay of Urease Activity in Soils.” Soil Biology & Biochemistry 4: 479–487. doi:https://doi.org/10.1016/0038-0717(72)90064-8.
- Tanaka, F. 2002. Formation of Aromatic Acids and Growth Inhibition of Rice (Oryza Sativa L.) Plants in Flooded Soils with Wheat Straw Added. NARC Kyushu Report. 40. 33–78. in Japanese with English summary.
- The University of Tokyo. 1978. ”Experiment of Agricultural Chemistry. 3rd ed. Tokyo, Japan: Asakura Shoten.
- Yoshida, T., and M. Alexander. 1970. “”Nitrous Oxide Formation by Nitrosomonas Europaea and Heterotrophic Microorganisms.”.” Soil Science Society of America Proceedings 34: 880–882. doi:https://doi.org/10.2136/sssaj1970.03615995003400060020x.