ABSTRACT
Acid rain (AR) and atmospheric particulate matter (APM) are important environmental issues that often occur simultaneously in agricultural regions, the combined pollution of AR and atmospheric fine particles (PM2.5) containing heavy metals has negatively affected the growth of seedlings and has become a hot research topic. In this paper, we established exposure scenarios to simulate the AR and the cadmium-containing PM2.5 (PM2.5-Cd) pollutions. Under the scenarios, hazardous impacts of AR and PM2.5-Cd on growth, physiological and biochemical characteristics of pakchoi were evaluated. After being exposed simulated AR and PM2.5-Cd for 21 days, our results indicate that: single treatment with either AR (pH 5.5) or PM2.5-Cd, or a combined treatment of AR (pH 5.5) and PM2.5-Cd (150, 300 μg·m−3) increased the growth of pack hoi seedlings. Combined treatments with low-acidity AR (pH 3.5) and PM2.5-Cd (500 μg·m−3), significantly decreased the growth and the Soil and Plant Analyzer Development (SPAD) of pakchoi, and it also induced a significantly increase in foliar MDA content and catalase (CAT) activity, while decreasing peroxidase (POD) and superoxide dismutase (SOD) activity. The Cd concentration of leaves increased with an increasing PM2.5-Cd concentration. Correlation analysis indicated that the growth and SPAD of pakchoi were highly correlated with the foliar MDA content and enzyme activities under the combined treatments with AR and PM2.5-Cd treatment. Our results might serve as a guide to increase agricultural production and food crop safety in areas with high levels of AR and PM2.5 pollution.
1. Introduction
Acid rain (AR) has become a serious global environmental issue because of rapid worldwide agricultural and industrial development (Ma et al. Citation2021; Kochergina et al. Citation2017; Liu et al. Citation2016). The natural environment of agricultural systems in China is relatively fragile (Dolatabadian et al. Citation2013; Liang and Wang Citation2013), with issues associated with AR being most serious in southern China (Sun et al. Citation2016; Liu et al. Citation2020). The area polluted by AR accounts for more than 40% of the land area in China (You Citation2010), which is believed to have a negative impact on the safety of crop ecosystems and to be a huge threat to human life (Li et al. Citation2021; Debnath et al. Citation2018; Liu et al. Citation2019; Ren et al. Citation2018). In addition, AR can significantly improve the activity, solubility, and migration of toxic heavy metals in soil (Cui et al. Citation2017), and this combination can negatively impact the physical and chemical properties of plants, inhibiting plant growth through reduced biomass and mineral uptake, and decreasing activities of antioxidative enzymes, reducing the resistance and quality of plants (Hu et al. Citation2019; Sun et al. Citation2012; Liu et al. Citation2019; Gill, Khan, and Tuteja Citation2012).
In nature, plants are exposed to multiple stressors simultaneously. Different contaminants can show synergistic, antagonistic or additive interactions. Atmospheric particulate matter (APM) is a well-known environmental problem, with negative impacts on human health and plant growth (Sánchez-Piñero et al. Citation2021; Abdelaal et al. Citation2021). The bioavailability and toxicity of APM increase with the decreasing particle size, and 75%~90% of the heavy metals are adsorbed on to inhalable particles (Kong et al. Citation2012). Fine APM (PM2.5), especially ultrafine particulate matter with a particle size of less than PM2.5, has become a focus and difficulty of environmental research. Compared with coarser atmospheric particles, PM2.5 is characterized by a small particle, a large surface area, and strong activity. It can be easily absorbed by human and plant cells, impacting human health and plant development. Heavy metals are the main inorganic components of PM2.5 (Ayua et al. Citation2020; Liu et al. Citation2020; Liu et al. Citation2019), with 25%~40% of the heavy metals in plants originating from the APM deposition in polluted areas (Schreck et al. Citation2012; Nowack and Bucheli Citation2007). In addition, APM containing heavy metals are likely to settle on the surface of leafy vegetables, entering the leaf interior via the stomata or cuticle. Previous studies showed that plant foliage has a crucial role in the exposure, uptake, and accumulation of APM (Zha et al. Citation2018; Xiong et al. Citation2019; Singh et al. Citation2021), which can accumulate eventually in humans through the food chain, negatively impacting human health.
The average content of Cd in atmospheric dustfall in China over the past 20 years exceeds 16.5 times the value of the primary standard of Soil Environmental Quality Standard (GB15618-1995). It is adsorbed on the particles less than 1 μm in sizes (Canepari et al. Citation2008; Louie et al. Citation2005) and can be easily taken up by crops, in which it tends to accumulate within the edible part of the plant (e.g., leaves and tubers) (Gill, Khan, and Tuteja Citation2012). Pakchoi (Brassica campestris. L) is an important dietary component in China and it is widely grown in regions affected by both AR and APM deposition. The physiological and biochemical response of pakchoi to combined treatments with simulated AR and APM are remain largely unclear, although they could have important repercussions for human health.
To investigate the response of pack hoi to simulated AR with different pH values and PM2.5-Cd at different concentrations, potted plant simulation tests were established. Our aim was to determine the influences of these combined treatments on the stem length (SL), root length (RL), fresh weight (FW), water content (WT), the relative chlorophyll content (Soil and Plant Analyzer Developmen-SAPD), and Cd concentration in leaves, as well as foliar malonydiadehyde (MDA) and antioxidant enzyme activities compared with control plants. The results from this study will provide scientific insight into the combined effects of AR and heavy metal-containing PM2.5 on leafy vegetable and serve as a guide to evaluate the safety of agricultural production.
2. Materials and methods
2.1. Plant materials and treatments
Pakchoi seeds (Degao508, Henan seed co, Ltd., China) were sterilized with 1% sodium hypochlorite solution for 10 min, rinsed with double distilled water for several times and germinated in an incubator at 25 ± 1°C. Four uniform seedlings with 2 leaves were transplanted into a plastic flowerpot (diameter = 12 cm, height = 10 cm) filled with the 2.0 kg air-dried clean soil (P: 0.20 g·kg−1, N: 0.46 g·kg−1, organic matter: 11.37 g·kg−1). The yellow soil was collected from the arable layer (0–20 cm) of an agriculture field, Yaxian family farms, Linan district, Zhejiang province, China. The soil had a pH (H2O) of 6.9, organic matter content of 28.9 g·kg−1, it contained 461 mg·kg−1 of rapid available P, and 520 mg·kg−1 of slowly available K; the total N was 0.165%, the total P was 783 mg·kg−1, total K was 1.52 × 104 mg·kg−1, and the total Cd was 0.013 mg·kg−1. The pots were placed in a miniature greenhouse in a photoperiod of 16 h light/8 h dark, light intensity ranged from 1600 to 21,000 lux, at a day/night temperature of 25 ± 5°C, and a relative humidity of 60%-70%. The experiment was started once 50% of seedlings had developed four leaves. Six pots were used for each treatment group. All experiments ran for 21 days. The flowerpots were randomly switched within their treatments groups to account for differences in microclimate.
The average pH value of monthly precipitation in Hangzhou ranges from 4.78 to 5.62 (Hangzhou Muncipal Environmental Protection Bureau), with a visible damage threshold of pH 3.0 (Zhang et al. Citation2020; Liang et al. Citation2018). Therefore, we selected pH 3.5 and pH 5.5 as the treatment to test. Control rain at pH 7.0 was prepared by adding Ca2+, K2+, NH42+, Mg2+, SO42-, NO3−, F− and Cl− to deionized water. pH 3.5 and 5.5 AR were prepared by adjusting the pH of control rain by adding concentrated H2SO4 and HNO3 at a ratio of 4.7 (v/v) followed by dilution to pH 7.0, 5.5 and 3.5. The seedlings were sprayed with 15 mm AR once every 5 days, reflecting the amount of acid rain received monthly (85 mm) based on precipitation data in the Hangzhou region. The area of the flowerpots was calculated to determine the amount of rainfall needed for each mini greenhouse. The leaves of the control plants were sprayed daily with deionized water (pH 7.0).
According to the Chinese Ministry of Environmental Protection, an AQI index of 150, 300 and 500 μg·m−3, represents mild, heavy, and severe pollution, respectively. Solutions of Cd2+ (30 mg·L−1) were prepared by dissolving appropriate quantities of Cd ICP standard solution 1000 mg·L−1 (Sigma-Aldrich, USA). Aerosol generation techniques to produce high particle number and mass concentration are necessary for basic scientific investigations of particle dispersion under controlled laboratory conditions (Di, Wang, and Hua Citation2019). In the current study, an aerosol generator (9302, Shanghai Huifen company) was used to produce a polydispersed high-concentration aerosol, with a particle size of 0.01–2 μm. Based on the volume of the flowerpots and the assumption that particulate matter escape and adsorption loss did not occur during the experiment, aerosol generation of PM2.5-Cd in hermetic miniature greenhouses was performed for 144 s, 288 s and 480 s. The process was reported every four 8 h a day once every 3 days. Combined treatment with AR and PM2.5-Cd are shown in . The seedlings were sprayed with 15 mm AR once every 5 days and PM2.5-Cd deposition every 3 days were conduct simultaneously.
Table 1. Experiments design
2.2. Identification of growth index
Following a 3-week treatment period, the growth of 21-day-old pakchoi seedlings from each flowerpot was determined. The FW of each plant was measured with an electronic balance after drying with absorptive paper; the roots of each plant were cut off with scissors. Using Vernier calipers, the SL was measured from the cut root to the highest point of the leaf, and the RL from the cut part to the end of longest root. The DW of the sample was recorded after drying to a constant weight at 70°C. WT is calculated by Formula 1, moisture content (%) = (1- DW/FW) ×100.
2.3. Measurements of Cd content in leaves
Pakchoi leave samples were immersed in 20-mM EDTA solutions for 10 min to remove adsorbed metals on the foliar surface, re-washed with distilled water for at least three times, then were dried at 105°C for 30 min, and then at 60°C in an oven until completely dry (Ponce et al. Citation2015; Salazar et al. Citation2012; Mao et al. Citation2018). The washed leaves were subjected to di-acid digestion using nitric acid (HNO3) and perchloric acidin (HClO4) a ratio of 4:1 followed by heating on a hot plate at 250°C (Sharma et al. Citation2020; Zheljazkov and Nielsen Citation1996). Samples were later filtered and analyzed for Cd using Atomic Absorption Spectrophotometer (AAS) (z2000, Hitachi. Japan). The Cd concentration of each sample was expressed as mg·kg−1 DW.
2.4. Measurements of the relative content of chlorophyll
The SPAD of the pakchoi leaves was determined by SPAD-502Plus chlorophyll meter (Uddling et al. Citation2007). Before the measurement, the leaf surface of the plant was dried and prepared according to the manufacturer’s instructions. The leaves were clamped with the front end of the instrument. The level of chlorophyll was measured at the tip, middle and base of each leaf three times, with the results recorded after several seconds of exposure.
2.5. Antioxidant enzymes activities
1 g of fresh leaves from control and treated plants was ground in liquid nitrogen, added to the extract in the 1 mL kit (Soleibao Biological Technology Co., LTD, Beijng, China), homogenized and ground in a high-flux tissue grinder. After removing the glass beads, the samples were centrifuged at 8000 g at 4°C for 10 min, and a petting gun was used to remove the supernatant, which was then placed on ice. The above indices were measured in a UV spectrophotometer at a specific absorbance. All experiments were carried out at 4°C. All samples were determined by biological kits, the MDA contents was expressed as nmol/mg sample (Islam et al. Citation2016; Shi et al. Citation2005), the SOD and POD activities were expressed as units/mg protein, and the CAT activity was expressed as units/mg sample (Zhou et al. Citation2016; Wu, Zhang, and Dominy Citation2003).
2.6. Statistical analysis
All data presented are the means ± SE. The significance of differences between the treatments was analyzed by one-way analysis of variance (ANOVA), followed by a Duncan test. Two-Way ANOVA was employed to test the main effects and interaction of the simulated AR and PM2.5-Cd on the growth, SPAD, Cd concentration, foliar MDA content, and the antioxidant enzyme activities (SPSS.21.0 Inc., Chicago,Ill., USA) (Liu et al. Citation2018). Statistical program R 4.0.2 was used for correlation analysis to examine the associations between simulated AR, growth index, SPAD, Cd concentration, foliar MDA content, and antioxidant enzyme activities (Ma et al. Citation2021).
3. Results
3.1. Effects of acid rain and PM2.5-Cd on growth of pakchoi
() shows the growth of pakchoi exposed to simulated AR and PM2.5-Cd. When packhoi seedlings were treated with AR alone, compared with the control plants, the treatment of at pH5.5 and pH3.5 AR change the SL, RL, FW, DW and moisture content of pakchoi, but no significant difference compared with the control plants (p > 0.05). Under the pH5.5 AR treatment, the SL, RL, FW and DW increased by 5.77%, 7.63%, 19.29% and 9.83%, respectively, in comparison with the control. When the pH values of AR decreased to 3.5, the SL, RL, FW and DW decreased by 6.41%, 7.63%, 3.44% and 3.28%, respectively, in comparison with the control (p > 0.05).
Table 2. Effects of AR and PM2.5-Cd on growth of pakchoi
Pakchoi seedlings treated with PM2.5-Cd alone showed increased SL, RL, FW, DW and moisture content, but no difference were not significant compared with the control plants (p > 0.05). Treatments with PM2.5-Cd (300 μg·m−3), significantly increased FW and DW (by 9.51% and 21.31%, respectively), with control plants (p < 0.05).
When pakchoi seedlings were treated with pH 5.5 AR and PM2.5-Cd (300 μg·m−3), SL increased significantly (by 8.97%) compared with control plants. Under treatment with pH 5.5 AR and PM2.5-Cd (150 μg·m−3) (150 μg·m−3). RL increased significantly (by 16.03%), compared with control plants (p < 0.05). RL increased significantly (by 16.03%) compared with control plants. RL in pakchoi treated with pH 3.5 AR and PM2.5-Cd (150, 300 μg·m−3) increased by 6.78% and 0.85%, respectively, but was not significantly different compared with control plants (p > 0.05). Pakchoi seedlings treated with pH 3.5 AR and PM2.5-Cd at 150 and 300 μg·m−3 showed decreased SL, RL, FW, and DW, but were not significantly different compared with control plants. SL, RL, and FW decreased significantly (by 15.38%, 19.49%, and 9.18%, respectively) in response to pH 3.5 AR and PM2.5-Cd (500 μg·m−3), compared with control plants (p < 0.05). However, the different stress treatments had no significant effect on WT of pakchoi.
Two-way ANOVA revealed that simulated AR had significant effects on SL, RT, FW (p < 0.001) and DW (p < 0.05). The PM2.5-Cd had significant effects on SL, FW (p < 0.05) and DW (p < 0.001). In addition, simulated AR, PM2.5-Cd and their interactions had significant effects on DW.
3.2. Effects of AR and PM2.5-Cd on the relative content of chlorophyll
When pakchoi seedlings were treated with AR alone, the SPAD increased at pH 5.5 AR and decreased at pH 3.5 AR, but was not significantly different compared with the control plants (p > 0.05) ().
When treated with PM2.5-Cd alone, PM2.5-Cd (300 μg·m−3) significantly increased SPAD compared with control plants (by 7.79%) (p < 0.05). When treated with AR and PM2.5-Cd, SPAD was significantly higher in plants treated with pH 5.5 AR and PM2.5-Cd (300 μg·m−3) compared with control plants (an increase of 8.37%) (p < 0.05). However, SPAD was significantly decreased in plants treated with pH 3.5 AR and PM2.5-Cd (500 μg·m−3) (by 9.38%) (p < 0.05). Two-way ANOVA revealed that simulated AR and PM2.5-Cd impacted SPAD significantly. However, the interactive effects of AR and PM2.5-Cd on SAPD were not significant.
3.3. Effects of AR and PM2.5-Cd on Cd concentration in pakchoi leaves
Treatment with pH 5.5 and pH 3.5 AR changed the Cd concentration of pakchoi but not significantly so compared with control plants () (p > 0.05). Treatment with PM2.5-Cd alone significantly increased the Cd concentration t of leaves with increasing PM2.5-Cd concentration, with increases of 111.76%, 164.71%, and 317.64%, respectively, compared with controls. Under the combined treatment with AR and PM2.5-Cd, at the same pH value, the Cd concentration of pakchoi significantly increased with the increase in concentration of PM2.5-Cd compared with control plants (p < 0.05). However, compared with single PM2.5-Cd (500 μg·m−3) treatments, the Cd concentration decreased in plants when treated with pH 3.5 AR and PM2.5-Cd (150, 300 and 500 μg·m−3), with decreases of 5.56%, 4.45%, and 8.97%, respectively. Two-way ANOVA revealed that simulated AR and PM2.5-Cd significantly impacted the Cd concentration of pakchoi, as did the interactions of these treatments.
3.4. Effects of AR and PM2.5-Cd on foliar MDA contents
Treatment with AR increased the foliar content of MDA in pakchoi seedlings with decreasing pH, with significant differences between pH 3.5 and pH 7.0 AR (an increase of 92.27%) (). When treated with PM2.5-Cd (500 μg·m−3) alone, the MDA content increased by 89.06%, which was significantly different compared with control plants (p < 0.05). Excluding the combined treatment of AR at pH 5.5 and PM2.5-Cd (150 μg·m−3), the foliar MAD content of pakchoi were significantly increased in other combined treatments, when compared with the control plants (p < 0.05). The MDA content of pakchoi peaked under combined treatment with AR at pH 3.5 and PM2.5-Cd 500 μg·m−3, with an increase of 353.19% compared with controls. Two-way ANOVA revealed that simulated AR and PM2.5-Cd impacted MDA content significantly. In addition, the interactive effects of AR and PM2.5-Cd on MDA content were significant (p < 0.05).
3.5. Effects of AR and PM2.5-Cd on antioxidant activities of pakchoi leaves
SOD, POD and CAT activities in pakchoi under AR and PM2.5-Cd stress are shown in . When pakchoi seedlings were treated with AR alone, CAT and SOD activities increased along with decreasing pH values, with significant differences between pH 3.5 and pH 7.0 treatment groups (increased of 32.37% and 47.32%, respectively) (p < 0.05) (). Different pH values of AR had no effect on POD activity (p > 0.05) (). Under the PM2.5-Cd treatments alone, the CAT activities significantly increased compared with control plants, with increases of 43.66%, 31.43% and 97.05%, respectively (). However, POD activity was significantly decreased in PM2.5-Cd (500 μg·m−3) seedlings, with a decrease of 29.08% (). In addition, the PM2.5-Cd (500 μg·m−3) had no effect on the SOD activity of pakchoi (p > 0.05) (). When pakchoi seedlings were treated with both AR and PM2.5-Cd, the CAT activity significantly increased with the increased PM2.5-Cd concentration at the same pH value of AR (p < 0.05) (). However, POD activity significantly decreased under the combined treatments of AR at pH3.5 and PM2.5-Cd (300 and 500 μg·m−3) compared with control plants (p < 0.05), with the decreases of 32.09% and 42.94%, respectively (). SOD activity also decreased under the combined AR at pH3.5 and PM2.5-Cd (150, 300 and 500 μg·m−3) than that of the control plants (p < 0.05), with decreases of 24.95%, 24.87% and 37.88%, respectively (). Two-way ANOVA revealed that simulated AR and PM2.5-Cd impacted CAT activity significantly, whereas the interactive effects of AR and PM2.5-Cd on CAT activity were not significant. In addition, simulated AR, PM2.5-Cd and their interactions had significant effects on POD and SOD activity.
3.6. Correlation analysis
The results of Pearson’s correlation analysis between the growth index, SPAD, Cd c concentration, foliar MDA content and antioxidant enzyme activity with simulated AR and PM2.5-Cd treatments are shown in . Simulated AR pH value was positively correlated with SL, RL, FW, DW, SPAD (p < 0.001), POD and SOD (p < 0.05), and negatively correlated with MDA content (p < 0.001) and CAT activity (p < 0.05). Simulated PM2.5-Cd concentration was positively correlated with CdL, MDA content and CAT activity (p < 0.001), and negatively correlated with POD and SOD activity (p < 0.05).
Figure 5. Correlations between AR pH, PM2.5-Cd, SPAD, CdL, MDA content and antioxidant enzyme activities in leaves. CdL, Cd content in leaves.
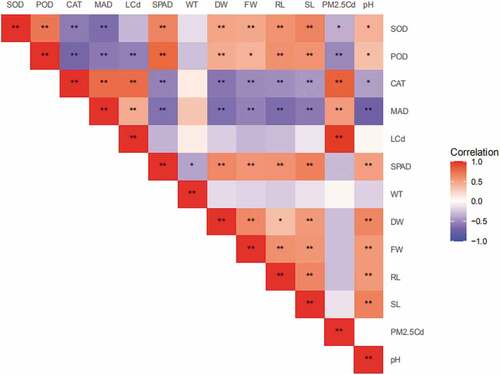
In addition, MDA content and CAT activity were negatively correlated with SL, RL, FW, DW and SPAD (p < 0.001), and both of them positive correlative with CdL (p < 0.001). MDA content was negatively correlated with POD and CAT activity (p < 0.001). POD and SOD activity was positively correlated with SL, RL, FW, DW and SPAD (p < 0.001), and POD was negatively correlated with CdL (p < 0.05).
4. Discussion
4.1. Combined pollution of AR and PM2.5-Cd on seedling growth
Plant growth reflects plant acclimatization and tolerance to environmental stress (Larcher, Larcher, and Larcher Citation2003). Although pH 3.5 is the threshold at which physiological damage to plant leaves is caused by AR (Lee and Weber Citation1979; Zhang et al. Citation2020; Liang et al. Citation2018), AR at pH 5.0 and 6.0 AR can also have adverse effects on the plant leaves (Macaulay and Enahoro Citation2015). Correlation analysis showed that AR pH was a key factor affecting the growth of plant (Pham et al. Citation2021; Yin et al. Citation2021; Singh et al. Citation2021), which explains AR with low acidity can decreased the aboveground plant growth in our study. Cd inhibits plant growth and biomass production, affects mineral uptake and protective system, and negatively interferes with essential physiological process (Li et al. Citation2015; Liu et al., Citation2017; Parmar, Kumari, and Sharma Citation2013). Previous studies have found that Cd2+ combined with AR would inhibit plant growth through reducing biomass and mineral uptake (Zhang et al. Citation2016). Our research found that the combined treatment with AR at pH 5.5 and PM2.5-Cd (500 μg·m−3) exposure would inhibit pakchoi growth, while the combined treatment with the PM2.5-Cd (500 μg·m−3) and AR at pH 3.5 significantly decreased SL, RL and FW of pakchoi (). Thus, these results indicated that the combined pollution of AR at pH 3.5 and PM2.5-Cd is more of a threat to the growth of pakchoi compared with pollution with either PM2.5-Cd or AR alone.
4.2. Combined pollution of AR and PM2.5-Cd on SPAD
Heavy metals decrease the photosynthetic rate, stomatal conductivity, and transpiration rate in plants. Chlorophyll parameters are easily affected by environmental stress and are commonly used as sensitive indicator of the photosynthesis performance (Li et al. Citation2015; Sun et al. 2016). Previous studies reported that a potentially positive impact of Cd on plant growth at a lower Cd concentration (Kumar and Knowles Citation1993; Pham et al. Citation2021), which explains PM2.5-Cd with low concentration (300 μg·m−3) had a certain positive impact on the chlorophyll content of pakchoi foliage in our study. General symptoms of heavy metal phytotoxicity were stunting and chlorosis (Ponce et al. Citation2015). In our study, the same symptoms of pakchoi was found under the combined treatment with the PM2.5-Cd (500 μg·m−3) and AR at pH 3.5 (Figure A1). The symptoms probably due to the changes in the metabolic process including photosynthesis (Mao et al. Citation2018). Decline in chlorophyll accompanied by reduced photosynthesis, was attributed to a heavy-metal induced closure of stomata (Lamoreaux and Chaney Citation1978; Stiborová et al. Citation1986). This suggests that the combination of AR at pH 3.5 and PM2.5-Cd (500 μg·m−3) resulted in stomatal closure and decreased stomatal conductance, significantly reducing the intercellular carbon dioxide concentration and, thus, directly inhibiting photosynthesis.
4.3. Combined pollution of AR and PM2.5-Cd on Cd concentraion of pakchoi
In our study, under the stress of PM2.5-Cd alone, the Cd concentration in leaves significantly increased with the increase in PM2.5-Cd concentration. However, there was no significant difference between the Cd concentration of pakchoi leaves under different pH values of AR, indicating that the effects of moderately acidic AR (pH 5.5) and a low-acidity AR (pH 3.5) on Cd levels in pakchoi were not obvious. Heavy metal uptake in leaves occurs via fat-soluble channels and water-soluble channels (Larue et al. Citation2014). In our study, the artificially imposed Cd pollution was water soluble, which is more easily absorbed by plants. Adherence mechanisms of PM2.5 in plant foliage and direct uptake by stomatal photosynthesis are the main modes of adsorption in the terrestrial part (Zhao et al. Citation2013). Most studies have indicated that PM2.5 containing heavy metals can penetrate the leaf tissue directly through the stomata (Gajbhiye et al. Citation2016; Birbaum et al. Citation2010). In our study, the Cd concentration in leaves was lower in response to the combined treatment with AR at pH 3.5 and PM2.5-Cd (500 μg·m−3) compared with the PM2.5-Cd treatment alone (), which could be attributed to the reduction of stomatal photosynthesis under combined stress of high acidity AR and high Cd concentration will affect its ability to absorb particulate matter. In addition, under the high acidity AR conditions, Cd tends to form insoluble precipitates with thiol bases synthesized from sulfur ions, which inhibit the uptake of Cd2+ in plants and affect the transport of Cd2+ in plants.
4.4. Combined pollution of AR and PM2.5-Cd on the foliar MDA content
MDA is the final decomposition product of membrane lipid peroxidation (Sanchez-Pardo, Fernandez-Pascual, and Zornoza Citation2014), and its content can be used to explain changes in plant growth and physiological functions under environmental stress (Ma et al. Citation2021). Generally, AR can damage the membrane of leaf cells and cause the accumulation of free radicals (Liu et al. Citation2019). Under the stress of AR at pH3.5, the contents of MDA in pakchoi leaves was significantly higher than the control (), indicating that those concentration of AR caused oxidative damage to pakchoi leave. Cd2+ stress can induce membrane damage, increase membrane permeability and the accumulation of free radicals in plants (Chaoui et al. Citation1997; Debnath et al. Citation2018). In our experiments, correlational analysis further supported the significant negative correlations of PM2.5-Cd and MDA content, which indicated that high concentrations of PM2.5-Cd can significantly increase oxidative damage to plant leaves (Islam et al. Citation2016). Furthermore, MDA content in leaves treated with a combination of AR at pH 3.5 and PM2.5-Cd (500 μg·m−3) was higher than in the other groups (), indicating that the combined action of AR and PM2.5-Cd increased the formation of MDA in plant leaves.
4.5. Combined pollution of AR and PM2.5-Cd on antioxidant activities
Antioxidant metabolism is an important mechanism that helps to reduce the excessive accumulation of reactive oxygen species (ROS) induced by environment stress (Chaoui et al. Citation1997; Zhang et al. Citation2015). POD, SOD, and CAT can effectively remove the free radicals induced by abiotic stress in order to protect the plant cells from to damage of abiotic stress (Ahmad et al. Citation2010). In our study, low-acidity AR (pH 3.5) significantly increased foliar CAT and SOD activity compared with control plants, suggesting that low-acidity AR has a stronger effect on foliage. Meanwhile, with increasing simulated PM2.5-Cd concentrations, foliar CAT and SOD activity also showed an upward trend. Generally, Cd2+ toxicity can increase (Srivastava et al., Citation2014) or decrease (Hassan et al., Citation2005) POD and CAT activities depending on the stress intensity (Liu et al., Citation2017). Our research showed that the increase in simulated PM2.5-Cd decreased POD and SOD activity, whereas the foliar MDA content was significantly negatively correlated with POD and SOD activity. POD and SOD activity was significantly correlated with the growth index and SPAD. This indicated that PM2.5-Cd impacts growth by affecting foliar MDA content, and POD and SOD activity. In addition, increased-pH AR and PM2.5-Cd concentration decreased SL, RL, FW, DW, and SPAD, and increased foliar MDA content and CAT activity, whereas CAT activity was significantly negative correlated with SL, RL, FW, DW and SPAD. This suggests that low-acidity AR and severe PM2.5-Cd pollution have a significant inhibitory effect on the growth of pakchoi seedlings, and might also impact the SPAD content by affecting CAT activity.
Under the combined treatments with AR and PM2.5-Cd, for POD activity, moderate pH AR (pH5.0) combined with PM2.5-Cd at 150 and 300 μg·m−3, the enzyme activity increased significantly. However, combined treatments with AR at pH3.5 and PM2.5-Cd, POD activity gradually reduced with the different concentrations of Cd. This indicating that the activities of POD were severely inhibited by the combined action of Cd and high pH AR, making plants unable to resist the stress and causing plant phenotypic changes, especially at PM2.5-Cd (500 μg·m−3). For AR with pH3.5, the change of enzyme activity was similar to that of POD, also decreased significantly combined with Cd PM2.5 at different concentrations, indicating that the plant cell membrane might be severely damaged by the AR (pH3.5) and PM2.5-Cd. Then, makes it impossible for plants to play the protective role of antioxidant enzyme system, thus affecting plant growth (). We speculated that AR (pH3.5) with PM2.5-Cd might easily cause Cd directly enter plant cells, resulting in devastating. In addition, previous researches have shown that the high catalase (CAT) activity of crop cultivars owned strong stress resistance. The results showed that its activity increased significantly at different pH of rain without or with treatment of PM2.5-Cd, meaning that high pH stress and Cd induced bad injury on plants. According to the above results, we know that different antioxidant enzymes behave differently with the combined stress of simulated AR and particulate pollutants containing PM2.5-Cd.
5. Conclusions
Combined treatments of a low pH value (pH 3.5) and PM2.5-Cd (500 μg·m−3) significantly decreased SL, RL, FW and SPAD, although the antioxidant enzymes behaved differently, with foliar MDA content and CAT activity significantly increased, whereas SOD and POD activity decreased. The Cd concentration of leaves significantly increased along with the increased PM2.5-Cd concentration, although the Cd concentration of leaves was lower in combined AR and PM2.5-Cd treatments than in single PM2.5-Cd treatments; this might be because these combined treatments induced stomatal closure and prevented heavy metals from entering the leaves. Correlation analysis indicated that SPAD of pakchoi seedlings was correlated with the foliar MDA content and enzyme activities under combined AR and PM2.5-Cd treatments. Combined stress with low-acidity AR and severe PM2.5-Cd pollution levels induced leaf tine fade and foliage yellowing. However, this complex effect could make the protection of leafy vegetables more complicated. Therefore, it is necessary to systematically study the response of leafy vegetables to stress at both the physiological and metabolic level.
Acknowledgments
We thank the Hefei Guangdian Metrology & Testing Co., LTD for detect the concentration of Cd. We are grateful to Dr. Songlin Ruan (Hangzhou Academy of Agricultural Sciences) for kind help and useful advices for data analysis. This work was financially supported by Natural science foundation of Zhejiang Province (LQ20C030007), and Jiangsu Planned Projects for Postdoctoral Research Fund (2018K064B).
Disclosure statement
No potential conflict of interest was reported by the author(s).
Additional information
Funding
References
- Abdelaal, A., E. A. Abbas, and M. S. Beheary. 2021. “Assessment of Dust Major Ions and Suspended Heavy Metal Contents in Atmospheric Particulate Matter of Port Said City, Egypt.” Arabian Journal of Geosciences 14 (15): 1419. doi:10.1007/s12517-021-07821-w.
- Ahmad, P., C. A. Jaleel, M. A. Salem, G. Nabi, and S. Sharma. 2010. “Roles of Enzymatic and Nonenzymatic Antioxidants in Plants during Abiotic Stress.” Critical Reviews in Biotechnology 30 (3): 161–175. doi:10.3109/07388550903524243.
- Ayua, T. J., A. A. Tyovenda, T. Sombo, E. Tikyaa, and T. Igbawua. 2020. “Fine Particulate Matter and Heavy Metals Pollution Status in Ambient Air of Some Selected Industrial Sites in Northern Nigeria.” J Geo Environ Pro 8 (8): 1–13. doi:10.4236/gep.2020.88001.
- Birbaum, B., M. W. J. Stark, D. Günther, L. K. Limbach, W. J. Stark, D. Günther, and L. K. Limbach. 2010. “No Evidence for Cerium Dioxide Nanoparticle Translocation in Maize Plants.” Environmental Science & Technology 44 (22): 8718–8723. doi:10.1021/es101685f.
- Canepari, S., C. Perrino, F. Olivieri, and M. Astolfi. 2008. “Characterisation of the Traffic Sources of PM through Size-segregated Sampling, Sequential Leaching and ICP Analysis.” Atmospheric Environment 42 (35): 8161–8175. doi:10.1016/j.atmosenv.2008.07.052.
- Chaoui, A., S. Mazhoudi, M. Ghorbal, and E. Ferjani. 1997. “Cadmium and Zinc Induction of Lipid Peroxidation and Effects on Antioxidant Enzyme Activities in Bean (Phaseolus Vulgaris L).” Plant Science 127 (2): 139–147. doi:10.1016/S0168-9452(97)00115-5.
- Cui, H., S. Zhang, R. Li, Q. Yi, X. Zheng, Y. Hu, and J. Zhou. 2017. “Leaching of Cu, Cd, Pb, and Phosphorus and Their Availability in the Phosphate-amended Contaminated Soils under Simulated Acid Rain.” Environmental Science and Pollution Research 24 (26): 21128–21137. doi:10.1007/s11356-017-9696-8.
- Debnath, B., M. Irshad, S. Mitra, M. Li, H. Rizwan, S. Liu, T. Pan, and D. L. Qiu. 2018. “Acid Rain Deposition Modulates Photosynthesis, Enzymatic and Non-enzymatic Antioxidant Activities in Tomato.” International Journal of Environmental Research 12 (2): 203–214. doi:10.1007/s41742-018-0084-0.
- Di, H., Z. Wang, and D. Hua. 2019. “Precise Size Distribution Measurement of Aerosol Particles and Fog Droplets in the Open Atmosphere.” Optics Express 27 (12): A890–A908. doi:10.1364/OE.27.00A890.
- Dolatabadian, A., S. A. M. M. Sanavy, A. K. Joghan, M. Majdi, A. B. Kashkooli, and A. B. Kashkooli. 2013. “The Role of Calcium in Improving Photosynthesis and Related Physiological and Biochemical Attributes of Spring Wheat Subjected to Simulated Acid Rain.” Physiology and Molecular Biology of Plants 19 (2): 189–198. doi:10.1007/s12298-013-0165-7.
- Gajbhiye, T., S. K. Pandey, K. H. Kim, J. E. Szulejko, and S. Prasad. 2016. “Airborne Foliar Transfer of PM Bound Heavy Metals in Cassia Siamea: A Less Common Route of Heavy Metal Accumulation.” Science of the Total Environment 573: 123–130. doi:10.1016/j.scitotenv.2016.08.099.
- Gill, S. S., N. A. Khan, and N. Tuteja. 2012. “Cadmium at High Dose Perturbs Growth, Photosynthesis and Nitrogen Metabolism while at Low Dose It up Regulates Sulfur Assimilation and Antioxidant Machinery in Garden Cress (Lepidium Sativum L.).” Plant Science 182: 112–120. doi:10.1016/j.plantsci.2011.04.018.
- Hassan, M.J., G. Shao, and G. Zhang. 2005.“Influence of cadmium toxicity on growth and antioidant enzyme activity in rice cultivars with different grain cadmium accumulation“ J. Plant. Nutr. 28: 1259–1270.
- Hu, X. F., A. Q. Wu, F. C. Wang, and F. S. Chen. 2019. “The Effects of Simulated Acid Rain on Internal Nutrient Cycling and the Ratios of Mg, Al, Ca, N, and P in Tea Plants of a Subtropical Plantation.” Environmental Monitoring and Assessment 191 (2): 99–113. doi:10.1007/s10661-019-7248-z.
- Islam, F., T. Yasmeen, M. S. Arif, M. Riaz, S. M. Shahzad, Q. Imran, and I. Ali. 2016. “Combined Ability of Chromium (Cr) Tolerant Plant Growth Promoting Bacteria (PGPB) and Salicylic Acid (SA) in Attenuation of Chromium Stress in Maize Plants.” Plant Physiology and Biochemistry 108: 456–467. doi:10.1016/j.plaphy.2016.08.014.
- Kochergina, Y., M. Udatny, V. Penizek, and M. Mihaljevic. 2017. “Mobility of Pb, Zn, Cu and as in Disturbed Forest Soils Affected by Acid Rain.” Ecol Environ Conserv 189 (11): 570. doi:10.1007/s10661-017-6306-7.
- Kong, S. F., B. Lu, Y. Q. Ji, X. Y. Zhao, Z. P. Bai, Y. H. Xu, Y. Liu, and H. Jiang. 2012. “Risk Assessment of Heavy Metals in Road and Soil Dusts within PM2.5, PM10 and PM100 Fractions in Dongying City, Shandong Province, China.” Journal of Environmental Monitoring 14 (3): 791–803. doi:10.1039/C1EM10555H.
- Kumar, G., and N. R. Knowles. 1993. “Changes in Lipid Peroxidation and Lipolytic and Free-radical Scavenging Enzyme Activities during Aging and Sprouting of Potato (Solanum Tuberosum) Seed-tubers.” Plant Physiology 102 (1): 115–124. doi:10.1104/pp.102.1.115.
- Lamoreaux, R. J., and W. R. Chaney. 1978. “The Effect of Cadmium on Net Photosynthesis, Transpiration and Dark Respiration of Excised Silver Maple Leaves.” Physiologia Plantarum 43 (3): 231–236. doi:10.1111/j.1399-3054.1978.
- Larcher, W., W. Larcher, and T. Larcher. 2003. Physiological Plant Ecology Berlin: Springer-Verlag.
- Larue, C., C. M. Hiram, S. Sobanska, T. Nicolas, S. Stéphanie, C. Lauric, Q. Lautent, L. Samuel, and S. Géraldine. 2014. “Fate of Pristine TiO2 Nanoparticles and Aged Paint-containing TiO2 Nanoparticles in Lettuce Crop after Foliar Exposure.” Journal of Hazardous Materials 273: 17–26. doi:10.1016/j.jhazmat.2014.03.014.
- Lee, J. J., and D. E. Weber. 1979. “The Effect of Simulated Acid Rain on Seedling Emergence and Growth of Eleven Woody Species.” Forest. Sci 25 (3): 393–398. doi:10.1093/forestscience/25.3.393.
- Li, S., W. B. Yang, T. T. Yang, Y. Chen, and W. Z. Ni. 2015. “Effects of Cadmium Stress on Leaf Chlorophyll Fluorescence and Photosynthesis of Elsholtzia argyi—A Cadmium Accumulating Plant.” International Journal of Phytoremediation 17 (1): 85–92. doi:10.1080/15226514.2013.828020.
- Li, X. L., Y. Q. Wang, Y. Zhang, Y. J. Wang, and C. M. Pei. 2021. “Response on Soil Chemical Properties and Enzyme Activity of Four Species in the Three Gorges Reservoir Area to Simulated Acid Rain.” Ecotoxicology and Environmental Safety 208: 111457. doi:10.1016/j.ecoenv.2020.111457.
- Liang, C., and W. Wang. 2013. “Antioxidant Response of Soybean Seedlings to Joint Stress of Lanthanum and Acid Rain.” Environmental Science and Pollution Research 20 (11): 8182–8191. doi:10.1007/s11356-013-1776-9.
- Liang, J., Y. I. Ye, Y. I. Peng, and M. Quan. 2018. “Effects of Simulated Acid Rain on Physiological Characteristics and Active Ingredient Content of Asparagus Cochinchinensis (Lour.) Merr.” Pak J Bot 50 (6): 2395–2399.
- Liu, H. L., J. Zhou, M. Li, Y. M. Hu, X. L. Liu, and J. Zhou. 2019. “Study of the Bioavailability of Heavy Metals from Atmospheric Deposition on the Soil-pakchoi (Brassica Chinensis L.) System.” Journal of Hazardous Materials 362: 9–16. doi:10.1016/j.jhazmat.2018.09.032.
- Liu, L., X. Zhang, S. Wang, W. Zhang, and X. Lu. 2016. “Bulk Sulfur (S) Deposition in China.” Atmospheric Environment 135: 41–49. doi:10.1016/j.atmosenv.2016.04.003.
- Liu, X., B Zhang, W. Zhao, W. Ling, D. Xie, W. Huo, Y. Wu, and J. Zhang. 2017. “Comparative Effects of Sulfuric and Nitric Acid Rain on Litter Decomposition and Soil Microbial Community in Subtropical Plantation of Yangtze River Delta Region.” Science of the Total Environment 601-602: 669–678. doi:10.1016/j.scitotenv.2017.05.151.
- Liu, X., Z. Fu, B. Zhang, Z. Lu, M. Meng, J. Lin, J. Zhuang, G. G. Wang, and J. Zhang. 2018. “Effects of Sulfuric, Nitric, and Mixed Acid Rain on Chinese Fir Sapling Growth in Southern China.” Ecotoxicology and Environmental Safety 175: 118–127. doi:10.1016/j.ecoenv.2018.04.071.
- Liu, Z. Y., J. Z. Zhou, J. Zhang, Y. B. Mao, X. Huang, and G. R. Qian. 2020. “Evaluation for the Heavy Metal Risk in Fine Particulate Matter from the Perspective of Urban Energy and Industrial Structure in China: A Meta-analysis.” Journal of Cleaner Production 244: 118597–118637. doi:10.1016/j.jclepro.2019.118597.
- Louie, P. K. K., J. Chow, L. Chen, J. Waston, G. Leung, and D. Sin. 2005. “PM2.5 Chemical Composition in Hong Kong: Urban and Regional Variations.” Science of the Total Environment 338 (3): 267–281. doi:10.1016/j.scitotenv.2004.07.021.
- Ma, S., X. Liu, Z. Jia, M. Meng, J. Zhang, Q. Ren, L. Zhai, B. Zhang, Y. Zhang, and J. Zhang. 2021. “Response of Quercus Acutissima Foliage to Different Types of Simulated Acid Rain.” Atmospheric Pollution Research 12 (7): 101112–1011120. doi:10.1016/j.apr.2021.101112.
- Macaulay, B., and G. Enahoro. 2015. “Effects of Simulated Acid Rain on the Morphology, Phenology and Dry Biomass of a Local Variety of Maize (Suwan-1) in Southwestern Nigeria. Environ.” Environmental Monitoring and Assessment 187 (10): 622–635. doi:10.1007/s10661-015-4844-4.
- Mao, F., G. J. Nan, M. Cao, Y. Q. Gao, L. Y. Guo, X. X. Meng, and G. D. Yang. 2018. “The Metal Distribution and the Change of Physiological and Biochemical Process in Soybean and Mung Bean Plants under Heavy Metal Stress.” International Journal of Phytoremediation 20 (11): 1113–1120. doi:10.1080/15226514.2017.1365346.
- Nowack, B., and T. D. Bucheli. 2007. “Occurrence, Behavior and Effects of Nanoparticles in the Environment.” Environmental Pollution 150 (1): 5–22. doi:10.1016/j.envpol.2007.06.006.
- Parmar, P., N. Kumari, and V. Sharma. 2013. “Structural and Functional Alterations in Photosynthetic Apparatus of Plants under Cadmium Stress.” Botanical Studies 54 (1): 45–51. doi:10.1186/1999-3110-54-45.
- Pham, H. T., A. T. Nguyen, A. T. Do, and L. Hens. 2021. “Impacts of Simulated Acid Rain on the Growth and the Yield of Soybean (Glycine Max (L.) Merr.) In the Mountains of Northern Vietnam.” Sustainability 13 (9): 4980–4995. doi:10.3390/SU13094980.
- Ponce, S. C., C. Prado, E. Pagano, F. Prado, and M. Rosa. 2015. “Effect of Solution pH on the Dynamic of Biosorption of Cr (VI) by Living Plants of Salvinia Minima.” Ecological Engineering 74: 33–41. doi:10.1016/j.ecoleng.2014.09.117.
- Ren, X., J. Zhu, H. Liu, X. Xu, and C. Liang. 2018. “Response of Antioxidative System in Rice (Oryza Sativa) Leaves to Simulated Acid Rain Stress.” Ecotoxicology and Environmental Safety 148: 851–856. doi:10.1016/j.ecoenv.2017.11.046.
- Salazar, M. J., J. H. Rodriguez, G. L. Nieto, and M. N. Pignata. 2012. “Effects of Heavy Metal Concentrations (Cd, Zn and Pb) in Agricultural Soils near Different Emission Sources on Quality, Accumulation and Food Safety in Soybean (Glycine Max (L.) Merrill).” Journal of Hazardous Materials 233-234 (10): 244–253. doi:10.1016/j.jhazmat.2012.07.026.
- Sanchez-Pardo, B., M. Fernandez-Pascual, and P. Zornoza. 2014. “Copper Microlocalisation and Changes in Leaf Morphology, Chloroplast Ultrastructure and Antioxidative Response in White Lupin and Soybean Grown in Copper Excess.” Journal of Plant Research 127 (1): 119–129. doi:10.1007/s10265-013-0583-1.
- Sánchez-Piñero, J., J. Moreda-Piñeiro, C. Moscoso-Pérez, V. Fernándezgonzález, D. Prada-Rodríguez, and P. López-Mahía. 2021. “Development and Validation of a Multi-pollutant Method for the Analysis of Polycyclic Aromatic Hydrocarbons, Synthetic Musk Compounds and Plasticizers in Atmospheric Particulate Matter (PM2.5).” Talanta Open 4: 1–13. doi:10.1016/J.TALO.2021.100057.
- Schreck, E., Y. Foucault, G. Sarret, Cécillon L, Castrec-Rouelle M, G. Uzu, and C. Dumat. 2012. “Metal and Metalloid Foliar Uptake by Various Plant Species Exposed to Atmospheric Industrial Fallout: Mechanisms Involved for Lead.” Science of the Total Environment 427-428: 253–262. doi:10.1016/j.scitotenv.2012.03.051.
- Sharma, P., P. Yadav, C. Ghosh, and B. Singh. 2020. “Heavy Metal Capture from the Suspended Particulate Matter by Morus Alba and Evidence of Foliar Uptake and Translocation of PM Associated Zinc Using Radiotracer (65zn).” Chemosphere 254: 126863. doi:10.1016/j.chemosphere.2020.126863.
- Shi, S., G. Wang, Y. Wang, L. Zhang, and L. Zhang. 2005. “Protective Effect of Nitric Oxide against Oxidative Stress under ultraviolet-B Radiation.” Nitric Oxide-Biol Ch 13 (1): 1–9. doi:10.1016/j.niox.2005.04.006.
- Singh, S., B. Pandey, L. B. Roy, S. Shekhar, and R. K. Singh. 2021. “Tree Responses to Foliar Dust Deposition and Gradient of Air Pollution around Opencast Coal Mines of Jharia Coalfield, India: Gas Exchange, Antioxidative Potential and Tolerance Level.” Environmental Science and Pollution Research 28 (7): 8637–8651. doi:10.1007/s11356-020-11088-1.
- Srivastava, R.K., P. Pandey, R. Rajpoot, A. Rani, and R.S., Dubey. 2014. Cadmium and lead interactive effects on oxidative stress and antioxidative responses in rice seedlings. Protoplasma 251, 1047–1065.
- Stiborová, M., M. Doubravova, A. Březinova, and A. Friedrich. 1986. “Effect of Heavy Metal Ions on Growth and Biochemical Characteristics of Photosynthesis of Barely (Hordeum Vulgare L.).” Photosynthetica 20 (4): 418–425. doi:10.4141/cjas90-109.
- Sun, J., H. Hu, Y. Li, L. Wang, Q. Zhou, and X. Huang. 2016a. “Effects and Mechanism of Acid Rain on Plant Chloroplast ATP Synthase.” Environmental Science and Pollution Research 23 (18): 18296–18306. doi:10.1007/s11356-016-7016-3.
- Sun, Z., L. Wang, M. Chen, L. Wang, C. Liang, Q. Zhou, and X. Huang. 2012. “Interactive Effects of Cadmium and Acid Rain on Photosynthetic Light Reaction in Soybean Seedlings.” Ecotoxicology and Environmental Safety 79: 62–68. doi:10.1016/j.ecoenv.2011.12.004.
- Uddling, J., J. Gelang-Alferdsson, K. Piikki, and H. Pleijel. 2007. “Evaluating the Relationship between Leaf Chlorophyll Concentration and SPAD-502 Chlorophyll Meter Readings.” Photosynthesis Research 91 (1): 37–46. doi:10.1007/s11120-006-9077-5.
- Wu, F., G. Zhang, and P. Dominy. 2003. “Four Barley Genotypes Respond Differently to Cadmium: Lipid Peroxidation and Activities of Antioxidant Capacity.” Environmental and Experimental Botany 50 (1): 67–78. doi:10.1016/S0098-8472(02)00113-2.
- Xiong, T., T. Zhang, C. Dumat, S. Sobanska, and V. Dappe. 2019. “Airborne Foliar Transfer of Particular Metals in Lactuca Sativa L.: Translocation, Phytotoxicity, and Bioaccessibility.” Environmental Science and Pollution Research 26 (4): 1–15. doi:10.1007/s11356-018-3084-x.
- Yin, P., Y. J. Peng, C. C. Song, X. J. Liu, J. Huang, and X. Lv. 2021. “Effects of Simulated Acid Rain on Growth of Lolium Perenne.” IOP Conference Series: Earth Env Sci 714: 4–09. doi:10.1088/1755-1315/714/2/022078.
- You. 2010. Improvement of China’s Air Pollution (Sulphur Dioxide and Acid Rain) Control and Countermeasures by Introducing Emissions Trading System. Canada: Dalhousie University.
- Zha, Y., Y. L. Zhang, Z. L. Ma, J. Tang, and K. Sun. 2018. “Distribution, Seasonal Variations and Ecological Risk Assessment of Polycyclic Aromatic Hydrocarbons in Foliar Dust of Nanjing, China. B.” Bulletin of Environmental Contamination and Toxicology 100 (4): 560–569. doi:10.1007/s00128-018-2287-7.
- Zhang, C., X. Yi, X. Gao, M. Wang, and C. Shen. 2020. “Physiological and Biochemical Responses of Tea Seedlings (Camellia Sinensis) to Simulated Acid Rain Conditions.” Ecotoxicology and Environmental Safety 192: 110315. doi:10.1016/j.ecoenv.2020.110315.
- Zhang, X., L. Wang, A. Zhou, Q. Zhou, and X. Huang. 2016. “Alterations in Cytosol Free Calcium in Horseradish Roots Simultaneously Exposed to lanthanum(III) and Acid Rain.” Ecotoxicology and Environmental Safety 126: 62–70. doi:10.1016/j.ecoenv.2015.12.014.
- Zhang, X., Y. Du, L. Wang, Q. Zhou, X. Huang, Z. Sun, and K. Wu. 2015. “Combined Effects of Lanthanum (Iii) and Acid Rain on Antioxidant Enzyme System in Soybean Roots.” PLoS One 10 (7): 134546–134561. doi:10.1371/journal.pone.0134546.
- Zhao, C. X., Y. J. Wang, Y. Q. Wang, and H. L. Zhao. 2013. “Interactions between Fine Particulate Matter (PM2.5) And Vegetation: A Review.” Chinese J Ecol 32 (8): 2203–2210. doi:10.13292/j.1000-4890.2013.0423. (in. Chinese).
- Zheljazkov, V. D., and N. E. Nielsen. 1996. “Effect of Heavy Metals on Peppermint and Cornmint.” Plant and Soil 178 (1): 59–66. doi:10.1007/BF00011163.
- Zhou, D., Y. Ning, J. Liu, D. Jie, and Y. Liu. 2016. “Effects of Oxidative Stress Reaction for the Eisenia Fetida with Exposure in Cd2+.” Environmental Science and Pollution Research 23 (21): 21883–21893. doi:10.1007/s11356-016-7422-6.