ABSTRACT
Stand age is a key factor for reforestation, and it has an important influence on the sustainable development of artificial forests. In order to reveal the temporal dynamics of soil active carbon (C) and nitrogen (N) in Chinese cypress artificial forests (CAFs) under different stand ages, soil samples were collected from five stands (25, 35, 45, 55, and 65 years old) in rocky mountainous of North China. The characterization of soil active C and N components, like soil microbial biomass C (MBC), microbial biomass N (MBN), dissolved organic C (DOC), dissolved organic N (DON), ammonium-N (NH4+-N) and nitrate-N (NO3−-N), as well as other soil properties were determined. The results showed that with the increase of stand age, the contents of soil MBC, MBN, DOC, DON, NH4+-N and NO3−-N tended to increase first and then decrease, and most of them reached the highest values in 45 years old. Soil active C and N components all reached peak values in June, and all of them showed the most significant variations in 0 ~ 10 cm soil layer (P < 0.01). Correlation analysis revealed that soil active C and N components showed significantly positive correlations with soil total N, total C, the number of microorganism communities and soil water content, as well as litter biomass (P < 0.01). Stepwise multiple regression analysis demonstrated that soil total N was the key controlling factor to soil active C and N components. In conclusion, with the increase of stand ages, soil active C and N components of CAFs did not accumulate all the time, indicating that stand age should be regarded as an important factor for the sustainable management of artificial forests.
1. Introduction
Soil active carbon (C) and nitrogen (N), which are frequently used as evaluating indexes of soil quality and environmental variation. Soil microbial biomass C and N (MBC, MBN), dissolved organic C and N (DOC, DON), ammonium-N (NH4+-N) and nitrate-N (NO3−-N) are the most active C and N components. They can be used as important indexes to indicate soil C and N turnover and nutrient availability (Kaiser and Guggenberger Citation2000). Although they account for a small proportion of soil C and N storage, they play a key role in the terrestrial ecological processes of C and N cycles (Sorensen et al. Citation2018; Wu et al. Citation2020a). Soil MBC and MBN are functional components of those microorganisms that regulate the decomposition of soil organic matter (SOM) and nutrient transformations (Zhao et al. Citation2017; Sorensen et al. Citation2018). Soil DOC and DON are potential sources of C and N fixation in deep soil (Monteith et al. Citation2007), as well as the main available materials and energy sources to soil microorganisms. Therefore, soil MBC, MBN, DOC, and DON all play a crucial role in the process of soil nutrient accumulation, which are of great significance to the fertility restoration of degraded soil after disturbance (Spohn et al. Citation2015). NH4+-N and NO3−-N are effective indicators of soil N bioavailability and important material basis for biochemical conversion during the N cycle.
Over the last century, excessive deforestation and long-term farming led to land degradation across China. In order to address this problem, the Chinese government implemented the Grain for Green Program by restoring cultivated lands to forestlands (Zeng et al. Citation2020). At present, the artificial forest area has ranked first in the world with 69.33 million ha in China (Kong et al. Citation2020). Nevertheless, the management of artificial forests is confronting new challenges. Long-term cultivation of pure forest has led to an increase in pests and diseases and biodiversity decline (Zhang et al. Citation2020). Stand age has become a key regulatory factor for reforestation (Jonsson et al. Citation2020). Lucas-Borja et al. (Citation2016) reported that stand age affects the distribution pattern of soil nutrients by changing forest material composition, stand structure and forest microclimate during the development of forest ecosystem. Young trees allocate more biomass to stem, branches, and leaves for growth (Porté et al. Citation2002), older trees use more water (Nosetto, Jobbagy, and Pauelo Citation2005). In addition, branches and leaves are more sensitive to stand age, which may be the important factor to influencing the yield and quality of litter (Xiang et al. Citation2021). According to previous studies, soil active C and N contents have been reported to decrease after reaching a peak value at a certain stage (Jia et al. Citation2005) or continuous increase (Susyan et al. Citation2011; Mao et al. Citation2012) or decrease (An et al. Citation2011) with increasing stand ages. These conflicting findings are believed to be closely correlated with the biological characteristics of vegetation, forest management and regional climate. We must establish a better understanding of how soil active C and N turnover respond to the changes of season and other soil properties during the development of artificial forests.
Since the 1950s, artificial afforestation has become the main measure for ecological restoration in the rocky mountainous area of North China (Kong et al. Citation2020). Chinese cypress (Platycladus orientatlis) is an evergreen coniferous tree species with strong adaptability to the environment (Feng et al., Citation2018). It is a common vegetation restoration tree in northern China. Moreover, Chinese cypress pure artificial forests (CAFs) can effectively accumulate soil nutrients and significantly improve soil quality (Chen et al. Citation2016). However, with the increase of CAFs plantation years, excessive consumption of soil nutrients by vegetation may lead to a decline in soil fertility, which is not conducive to the sustainable development of soil (Wang et al. Citation2011). Therefore, in the present study, CAFs at five typical stand stages were selected as research objects to identify the temporal dynamics of soil active C and N, which are frequently used as evaluating indexes of soil quality and environmental variation. We aim to gain a further insight into the response of soil active C and N to stand age and temporal dynamics, and finally provide scientific support for the sustainable management of artificial forests.
2. Materials and methods
2.1. Study sites and soil sampling
The study site is located at the Yellow River Forest Ecosystem Research Station of Xiaolangdi Dam (35°01’ N, 112°28’ E, 330 ~ 420 m a.s.l.), Chinese Forest Ecosystem Research Network (CFERN), Jiyuan city, Henan province, central of China. The local climate is a warm temperate continental monsoon, with four distinct seasons. It is hot and rainy in summer and cold and dry in winter. The mean annual temperature and precipitation in this area are 13.1°C and 641.7 mm, respectively, with more than 60% precipitation from June to September (Figure S1). The vegetation types are mainly drought-tolerant secondary shrubs, composed of Ziziphus jujube, Vitex negundo, and Lespedeza bicolor, and artificial forests planted after the destruction of original vegetation.
Five different stand ages of CAFs were selected on gentle slopes (10 ~ 18 °) and sunny aspects, including middle forest stage (25 years old, 25a), near-mature forest stage (35 years old, 35a), mature forest stage (45 years old, 45a; 55 years old, 55a) and overripe forest stage (65 years old, 65a). All forest stands were in close proximity (10 km apart) and had a similar slope position and aspect. According to US soil taxonomy, soils in the five stands were all classified as alfisols, with thin effective soil thickness and poor fertility and water-holding capacity. Details of sampling sites are given in .
Table 1. Characteristics of sampling sites
Evading rainy days, soil samples were collected around the middle of March, June, September, and December of 2020 on behalf of the local spring, summer, autumn, and winter. Three 10 × 10 m plots were selected in each stand. After the removal of surface litter, five soil samples within each plot were randomly obtained from three layers of 0 ~ 10, 10 ~ 20 and 20 ~ 30 cm using S-shaped sampling method. Fresh soils were sieved (2 mm) to remove small stone and roots and refrigerated at 4°C until analysis.
2.2. Analysis of soil active carbon and nitrogen
Soil MBC and MBN were determined by chloroform fumigation-extraction method (Wu et al. Citation2020b). A factor of 2.22 was used as efficiency of both microbial biomass C and N extractions (Jenkinson, Brookes, and Powlson Citation2004). Five grams of fresh soil were immersed in 25 mL of KCl (1 mol L−1) solution and shaken on an end-over-end shaker for 60 min. Then the extracts were filtered into 50-mL plastic bottles via cellulose-acetate membrane filters (0.45 μm pore size). Concentrations of DOC, DON, NH4+-N and NO3−-N in KCl extracts were measured using a continuous flow analyzer (SAN++, SKALAR, the Netherlands).
2.3. Analysis of soil physicochemical and biological properties
The quantities of three main soil microbial communities (bacteria, fungi, and actinomycete) were determined by the plate dilution method (Cai and Huang Citation2002). Soil total C (TC) and total N (TN) were measured with a soil elemental analyzer (EURO EA3000, Italy). Available N (AN), available phosphorus (AP) and available potassium (AK) contents of soil and litter were measured by using the alkali-diffusion method, ammonium acetate extraction-flame photometer method, and sodium bicarbonate extraction molybdenum-antimony anti-colorimetric method, respectively (Kong et al. Citation2020). Soil pH (air-dried soil/water, 1/2.5, w/w) was measured with a portable pH meter (PB-10, SARTORIUS, Germany). Soil water content (SWC) and litter water content (LWC) were determined by oven-drying method at 105°C and 80°C, respectively. Litter biomass (LB) was measured by the drying and weighing method. Soil particle size measurement system (Mastersizer 2000, UK) was utilized to determine soil particle composition volume fraction (classified into clay: <0.002, silt: 0.002 ~ 0.02 mm, sand: 0.02 ~ 2 mm). Soil bulk density (SBD) was analyzed by a cutting ring method. Soil porosity (SP) was detected with a soil three-phase meter (Daiki-1130, Japan). Canopy density was measured according to plant canopy analyzer (LAI-2000, LI-COR, USA).
2.4. Statistical analysis
The effects of stand age, temporal dynamic and soil layer on soil MBC, MBN, DOC, DON, NH4+-N and NO3−-N contents were examined by utilizing the principal component analysis (PCA), performed by the software CANOCO 5.0 (Biometris-Plant research international, Wageningen, the Netherlands). Pearson correlation coefficients were used to evaluate the relationship between soil active C and N components and other soil parameters. Stepwise multiple regressionanalysis was conducted to determine the main parameters influencing the soil active C and N components. Both of them were performed using the software SPSS Statistics 20 (IBM, New York, USA).
3. Results
3.1. Temporal dynamics of soil MBC and MBN under different stand ages
Stand age had significantly effect on soil MBC and MBN contents (). In the five stand ages of CAFs, the annual mean values of soil MBC and MBN contents in 35a (337 mg C kg−1, 33 mg N kg−1) and 45a (349 mg C kg−1, 34 mg N kg−1) at the layer of 0 ~ 30 cm were higher than those in 25a (181 mg C kg−1, 15 mg N kg−1) and 65a (171 mg C kg−1, 23 mg N kg−1) (). Soil MBC and MBN contents under the five stand ages of CAFs reached their highest values in June and lowest in March (). From surface layer (0 ~ 10 cm) to deep layer (20 ~ 30 cm), soil MBC and MBN contents decreased significantly by 39% and 40%, respectively ().
Figure 1. Principal component analysis (PCA) for the response of soil active C and N contents to stand age, temporal dynamic and soil layer. MBC, microbial biomass C; MBN, microbial biomass N; DOC, dissolved organic C; DON, dissolved organic N; 25a, middle forest stage (25 years old); 35a, near-mature forest stage (35 years old); 45a and 55a, mature forest stage (45 and 55 years old); 65a, overripe forest stage (65 years old); SA, TD and SL reprsent stand age, temporal dynamic and soil layer, respectively.
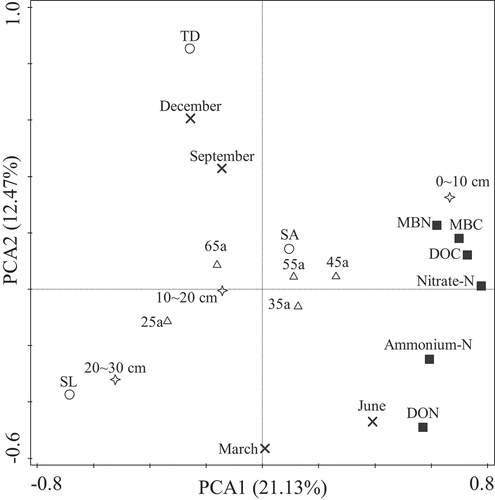
Figure 2. Temporal dynamics of soil microbial biomass C (MBC) (a) and microbial biomass N (MBN) (b) in Chinese cypress artificial forest with five stand ages. 25a, middle forest stage (25 years old); 35a, near-mature forest stage (35 years old); 45a and 55a, mature forest stage (45 and 55 years old); 65a, overripe forest stage (65 years old).
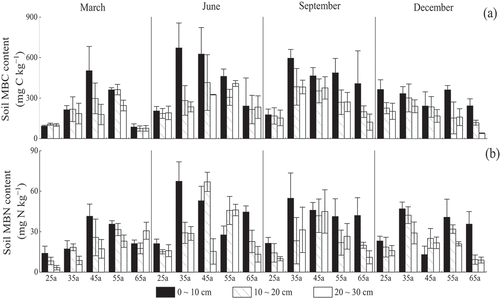
3.2. Temporal dynamics of soil DOC and DON under different stand ages
Soil DOC content had a significant correlation with stand age (). The annual mean value of soil DOC content in 45a at the layer of 0 ~ 30 cm was 1.43 and 1.31 times higher than that in 25a and 65a, respectively (). And the annual mean value of soil DON content (0 ~ 30 cm) showed the trend of 35a ≈ 45a > 55a > 65a > 25a (). Soil DOC and DON contents also had a significant temporal dynamic, attaining a peak value in June (). In the five stand ages of CAFs, DOC, and DON contents at 0 ~ 10 cm soil layer were substantially higher than those at other soil layers ().
Figure 3. Temporal dynamics of soil dissolved organic C (DOC) (a) and dissolved organic N (DON) (b) in Chinese cypress artificial forest with five stand ages. 25a, middle forest stage (25 years old); 35a, near-mature forest stage (35 years old); 45a and 55a, mature forest stage (45 and 55 years old); 65a, overripe forest stage (65 years old).
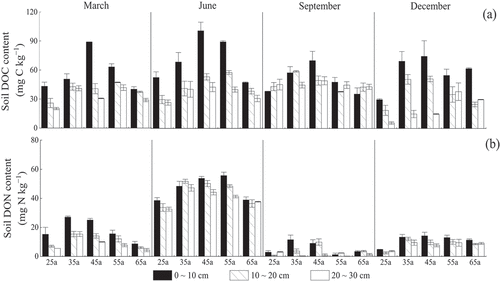
3.3. Temporal dynamics of soil NH4+-N and NO3−-N under different stand ages
Soil NO3−-N content was significantly affected by stand age (). With the increase of stand age, soil NO3−-N content showed a V-shaped trend and peaked in 45a (). Soil NH4+-N content showed high values in March and June and low values in September and December (), while soil NO3−-N content reached its peak value in June (). Furthermore, compared with the 0 ~ 10 cm soil layer, soil NH4+-N and NO3−-N contents at the 20 ~ 30 cm soil layer decreased significantly by 20% and 40%, respectively ().
Figure 4. Temporal dynamics of soil ammonium-N (NH4+-N) (a) and nitrate-N (NO3−-N) (b) in Chinese cypress artificial forest with five stand ages. 25a, middle forest stage (25 years old); 35a, near-mature forest stage (35 years old); 45a and 55a, mature forest stage (45 and 55 years old); 65a, overripe forest stage (65 years old).
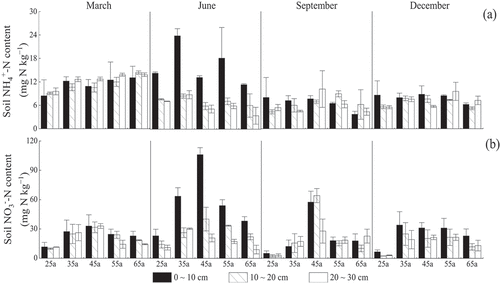
3.4. Relationships between soil active C and N components and other soil properties
The PCA was executed to disclose the effects of stand age, temporal dynamics and soil layer on soil active C and N (Matsushima, Choi, and Chang Citation2014). In the PCA biplot, PCA1 and PCA2 explained 21.13% and 12.47% variations of soil active C and N components, respectively (). PCA1 was significantly positive correlated with MBC, MBN, DOC, DON, NH4+-N, and NO3−-N contents. Stand age was located on the right side of PCA1, within the same side of soil active C and N components. Whereas temporal dynamic and soil layer were located on the left side of PCA1, implying that stand age had comparatively greater impacts on the soil active C and N components than temporal dynamic and soil layer.
In order to further explore the main influencing factors of soil active C and N components, Pearson correlation between the soil active C and N contents and soil properties was conducted (). Soil active C and N components showed positive correlations with TC, TN, AP, and LB (P < 0.01). Except for soil NH4+-N, other soil active C and N components all showed a positive correlation with the number of bacteria and fungi communities, SWC, soil clay and silt volume fractions (P < 0.01), while a negative correlation with soil sand volume fraction and LAP (P < 0.01). Except for soil NO3−-N, other soil active C and N components had significant correlations with the number of actinomycete communities (P < 0.05). In addition, soil MBC, MBN, DOC, and NO3−-N contents had strong positive correlations with LAN (P < 0.01). Soil MBN and NO3−-N contents were positively correlated with pH (P < 0.01).
Table 2. Pearson correlation coefficients between soil active C, N components, and soil properties (n = 180)
A stepwise multiple regression analysis was performed to determine the main controlling factors of soil active C and N components (). The key contributing factors of soil MBC, MBN, and DOC contents were soil TN and the number of actinomycete communities, accounting for 34%, 20%, and 44% of the variation, respectively. And soil TN was also the prime factor affecting the contents of soil DON (60%), NH4+-N (30%), and NO3−-N (70%).
Table 3. Multiple regression models for the relationship between soil active C and N components as well as soil properties (n = 180)
4. Discussion
4.1. Effects of stand ages on soil active C and N components
In the forest ecosystem, root exudates and litter residues are the primary source of soil organic matters (Zeng et al. Citation2010). In the present study, with the continuous increase of stand age, soil active C and N components (including MBC, MBN, DOC, DON, and NO3−-N) first increased and then decreased, showing the highest levels at 45a. The CAFs at 45a had a better soil physical structure (Table S1), which is not only conducive to capturing organic matter (Ma et al. Citation2014) but also promoting the growth of soil microorganism (Table S2). As revealed in this study, soil active C and N components (except for the NH4+-N) were significantly correlated with soil particle volume fraction (P < 0.01, ). The CAFs at 25a and 35a showed a fast growth rate and enhanced cell metabolism (Xiang et al. Citation2021), needing a large number of C, N to synthesize proteins and nucleic acids (Cui et al. Citation2014), thus the accumulation of soil active C and N was much less than the consumption of trees. At the middle forest stage of CAFs (25a), soil active C and N components tended to accumulate due to the enhanced quantity of above-ground tree litter () and the progressively growing root systems (Luan et al. Citation2010). While at overripe forest stage of CAFs (65a), soil active C and N components showed a downtrend due to the decrease of litter quantity and nutrient content. PCA analysis also verified that stand age at this stage had a significant negative impact on soil active C and N components (). However, soil NH4+-N was not significantly affected by stand age, which might result from the direct absorption and utilization by plants (Wang et al. Citation2015). Further, soil NH4+-N had a negative correlation with the number of bacteria and fungi communities (), implying that some soil NH4+-N might be converted to NO3−-N by microorganism (Hishi et al. Citation2014).
Table 4. Main litter properties in Chinese cypress artificial forests with five stand ages
Soil active C and N components decreased with the increase of soil layer, which was mainly due to the decomposition of litter and the release of organic matter in the soil surface (Zhou et al. Citation2021). In addition, the surface soil was loose (Table S1), and the frequency of gas and heat exchange in the surface soil layer was higher than that in the deep soil layer, which were beneficial to the activity of soil microorganisms and the turnover of soil active C and N.
4.2. Effects of temporal dynamics on soil active C and N components
In this study, soil active C and N contents fluctuated markedly in different seasons, and exhibited positive responses in June, especially soil DON and NH4+-N contents (). Temperature, precipitation, soil water content, and substrate concentration are the main factors affecting the turnover of soil active C and N components (Michel and Matzner Citation2002). Lee, Park, and Matzner (Citation2018) reported that temperature is a decisive factor in the physicochemical dissolution of soil organic matters. From March to June, the number of soil microorganism communities increased (Table S2), which could improve the decomposition rate of organic matters. It indirectly confirmed that warming and precipitation were the main reasons for the increase of soil active C and N contents from March to June (Figure S1). The soil active C and N components showed a decreasing trend from June to September, mainly due to 60% of the annual precipitation concentrated in this period (Figure S1). A large amount of concentrated precipitation caused the leaching migration of dissolved organic matter in the surface soil layer. And the flooding state limited soil microorganism activity, that is, declined the number of soil microorganism (Table S2).
In addition, soil DOC/DON had a significant increase from June to September (), which might be due to that 27% of the C in freshly fallen leaf litter can be soluble in water (Qualls and Haines Citation1991). And the loss of DON in soil was greater than that of DOC, demonstrating that plants absorbed more N and grew vigorously in this period. From September to December, the temperature dropped further, resulting in low contents of soil active C and N components. According to Verstraeten et al. (Citation2016), with the decline of temperature and precipitation, soil inorganic N decrease appropriately and can be converted to DON, which might cause a slow upward trend of DON from September to December. Consequently, temperature and precipitation could be the main driving factors of soil active C and N components turnover in the rocky mountainous area of North China.
5. Conclusion
Stand age and temporal dynamic have significant effects on soil active C and N components (i.e., MBC, MBN, DOC, DON, NH4+-N, and NO3−-N). We found that soil active C and N components generally tended to increase first and then decrease, and reached a high value in 45a, which performed as a significant seasonal variation and showed peak values in June. With the increase of soil layer, most of soil active C and N contents decreased significantly, showing a surface enrichment phenomenon. Moreover, soil TN and the number of actinomycete communities were the main controlling factors for soil MBC, MBN, and DOC contents. Furthermore, soil TN also significantly influenced DON, NH4+-N, and NO3−-N. In conclusion, stand age could be considered as a key factor in the sustainable management of artificial forests.
Supplemental Material
Download Zip (100.1 KB)Acknowledgments
The authors also thank Mr. Jia Chang-rong of the State-owned Jiyuan Nanshan forestry farm, China for providing information and help for the selection and set up of plots in the forestry farm.
Disclosure statement
No potential conflict of interest was reported by the author(s).
Supplementary material
Supplemental data for this article can be accessed here.
References
- An, R., J. Gong, X. You, Z. Ge, Q. Duan, and X. Yan. 2011. “Seasonal Dynamics of Soil Microorganisms and Soil Nutrients in Fast-growing Populus Plantation Forests of Different Ages inYili, Xinjiang, China.” Chinese Journal of Plant Ecology 35 (4): 389–401. doi:https://doi.org/10.3724/SP.J.1258.2011.00389.
- Cai, X., and J. Huang. 2002 . “Microbiology“, 333–354. 2nd ed. Beijing, China: Higher Education Press.
- Chen, J., B. Pei, H. Liu, M. Fu, E. Guo, and X. Yang. 2016. “Effects of Different Soil and Water Conservation Species on Soil Quality in Lithoid Hilly Area of North China.” Forest Resources Management 2: 81–86+97. doi:https://doi.org/10.13466/j.cnki.lyzygl.2016.02.015.
- Cui, N., X. Liu, D. Zhang, J. Zhang, Y. Liu, C. Deng, T. Ji, and Y. Chen. 2014. “The Distribution Pattern of Carbon, Nitrogen and Phosphorus and the Stoichiometry Characteristics of Pinus Massoniana Plantation in Different Ages.” Ecology and Environmental Sciences 23 (2): 188–195. doi:https://doi.org/10.16258/j.cnki.1674-5906.2014.02.023.
- Feng, Z., X. Yang, H. Liang, Y. Kong, D. Hui, J. Zhao, E. Guo, and B. Fan. 2018. “Improvements in the Root Morphology, Physiology, and Anatomy of Platycladus Orientalis Seedlings from Air-root Pruning.” HortScience 53 (12): 1750–1756. doi:https://doi.org/10.21273/HORTSCI13375-18.
- Hishi, T., R. Urakawa, N. Tashiro, Y. Maeda, and H. Shibata. 2014. “Seasonality of Factors Controlling N Mineralization Rates among Slope Positions and Aspects in Cool-temperate Deciduous Natural Forests and Larch Plantations.” Biology and Fertility of Soils 50 (2): 343–356. doi:https://doi.org/10.1007/s00374-013-0863-x.
- Jenkinson, D. S., P. C. Brookes, and D. S. Powlson. 2004. “Measuring Soil Microbial Biomass.” Soil Biology and Biochemistry 36 (1): 5–7. doi:https://doi.org/10.1016/j.soilbio.2003.10.002.
- Jia, G., J. Cao, C. Wang, and G. Wang. 2005. “Microbial Biomass and Nutrients in Soil at the Different Stages of Secondary Forest Succession in Ziwulin, Northwest China.” Forest Ecology and Management 217 (1): 117–125. doi:https://doi.org/10.1016/j.foreco.2005.05.055.
- Jonsson, M., J. Bengtsson, J. Moen, L. Gamfeldt, and T. Snäll. 2020. “Stand Age and Climate Influence Forest Ecosystem Service Delivery and Multifunctionality.” Environmental Research Letters 15 (9): 0940a8. doi:https://doi.org/10.1088/1748-9326/abaf1c.
- Kaiser, K., and G. Guggenberger. 2000. “The Role of DOM Sorption to Mineral Surfaces in the Preservation of Organic Matter in Soils.” Organic Geochemistry 31 (7–8): 711–725. doi:https://doi.org/10.1016/s0146-6380(00)00046-2.
- Kong, Y., N. L. Ma, Y. Yang, Y. Lai, Z. Feng, X. Shao, X. Xu, and D. Zhang. 2020. “Examining CO2 and N2O Pollution and Reduction from Forestry Application of Pure and Mixture Forest.” Environmental Pollution 265: 114951. doi:https://doi.org/10.1016/j.envpol.2020.114951.
- Lee, M. H., J. H. Park, and E. Matzner. 2018. “Sustained Production of Dissolved Organic Carbon and Nitrogen in Forest Floors during Continuous Leaching.” Geoderma 310:163−169. doi:https://doi.org/10.1016/j.geoderma.2017.07.027.
- Luan, J., C. Xiang, S. Liu, Z. Luo, Y. Gong, and X. Zhu. 2010. “Assessments of the Impacts of Chinese Fir Plantation and Natural Regenerated Forest on Soil Organic Matter Quality at Longmen Mountain, Sichuan, China.” Geoderma 156 (3): 228–236. doi:https://doi.org/10.1016/j.geoderma.2010.02.021.
- Lucas-Borja, M. E., J. Hedo, A. Cerdá, D. Candel-Pérez, and B. Viñegla. 2016. “Unravelling the Importance of Forest Age Stand and Forest Structure Driving Microbiological Soil Properties, Enzymatic Activities and Soil Nutrients Content in Mediterranean Spanish Black Pine (Pinus Nigra Ar. Ssp. Salzmannii) Forest.” Science of the Total Environment 562: 145–154. doi:https://doi.org/10.1016/j.scitotenv.2016.03.160.
- Ma, Y., T. R. Filley, K. Szlavecz, and M. K. McCormick. 2014. “Controls on Wood and Leaf Litter Incorporation into Soil Fractions in Forests at Different Successional Stages.” Soil Biology and Biochemistry 69: 212–222. doi:https://doi.org/10.1016/j.soilbio.2013.10.043.
- Mao, R., D. Zeng, L. Li, and Y. Hu. 2012. “Changes in Labile Soil Organic Matter Fractions following Land Use Change from Monocropping to Poplar-based Agroforestry Systems in a Semiarid Region of Northeast China.” Environmental Monitoring and Assessment 184 (11): 6845–6853. doi:https://doi.org/10.1007/s10661-011-2462-3.
- Matsushima, M. Y., W. J. Choi, and S. X. Chang. 2014. “Canada Bluejoint Foliar δ15N and δ13C Indicate Changed Soil N Availability by Litter Removal and N Fertilization in a 13-year-old Boreal Plantation.” Soil Science and Plant Nutrition 60 (2): 208–215. doi:https://doi.org/10.1080/00380768.2013.869762.
- Michel, K., and E. Matzner. 2002. “Nitrogen Content of Forest Floor Oa Layers Affects Carbon Pathways and Nitrogen Mineralization.” Soil Biology and Biochemistry 34 (11): 1807. doi:https://doi.org/10.1016/S0038-0717(02)00170-0.
- Monteith, D. T., J. L. Stoddard, C. D. Evans, H. A. Wit, M. Forsius, T. Høgåsen, A. Wilander, et al. 2007. “Dissolved Organic Carbon Trends Resulting from Changes in Atmospheric Deposition Chemistry.” Nature 450:537−541. doi:https://doi.org/10.1038/nature06316.
- Nosetto, M. D., E. G. Jobbagy, and J. M. Pauelo. 2005. “Land-use Change and Water Losses: The Case of Grassland Afforestation across a Soil Textural Gradient in Central Argentina.” Global Change Biology 11 (7): 1101–1117. doi:https://doi.org/10.1111/j.1365-2486.2005.00975.x.
- Porté, A., P. Trichet, D. Bert, and D. Loustau. 2002. “Allometric Relationship for Branch and Tree Woody Biomass of Maritime Pine (Pinus Pinaster Aït).” Forest Ecology and Management 158 (1): 71–83. doi:https://doi.org/10.1016/S0378-1127(00)00673-3.
- Qualls, R. G., and B. L. Haines. 1991. “Geochemistry of Dissolved Organic Nutrients in Water Percolating through a Forest Ecosystem.” Soil Science Society of America Journal 55 (4): 1112–1123. doi:https://doi.org/10.2136/SSSAJ1991.03615995005500040036X.
- Sorensen, P. O., A. C. Finzi, M. A. Giasson, A. B. Reinmann, and P. H. Templer. 2018. “Winter Soil Freeze-thaw Cycles Lead to Reductions in Soil Microbial Biomass and Activity Not Compensated for by Soil Warming.” Soil Biology and Biochemistry 116: 39–47. doi:https://doi.org/10.1016/j.soilbio.2017.09.026.
- Spohn, M., T. J. Novák, J. Incze, and L. Giani. 2015. “Dynamics of Soil Carbon, Nitrogen, and Phosphorus in Calcareous Soils after Land-use abandonmen—A Chronosequence Study.” Plant and Soil 401:185−196. doi:https://doi.org/10.1007/s11104-015-2513-6.
- Susyan, E. A., S. Wirth, N. D. Ananyeva, and E. V. Stolnikova. 2011. “Forest Succession on Abandoned Arable Soils in European Russia—Impacts on Microbial Biomass, Fungal-bacterial Ratio, and Basal CO2 Respiration Activity.” European Journal of Soil Biology 47 (3): 169–174. doi:https://doi.org/10.1016/j.ejsobi.2011.04.002.
- Verstraeten, A., P. Verschelde, B. D. Vos, J. Neirynck, N. Cools, P. Roskams, M. Hens, G. Louettes, S. Sleutel, and S. D. Neve. 2016. “Increasing Trends of Dissolved Organic Nitrogen (DON) in Temperate Forests under Recovery from Acidification in Flanders, Belgium.” Science of the Total Environment 553: 107–119. doi:https://doi.org/10.1016/j.scitotenv.2016.02.060.
- Wang, C., H. Xiao, L. Wang, and D. Du. 2015. “Insights into Ecological Effects of Invasive Plants on Soil Nitrogen Cycles.” American Journal of Plant Sciences 6 (1): 34–46. doi:https://doi.org/10.4236/ajps.2015.61005.
- Wang, W., L. Qiu, Y. Zu, D. Su, J. An, H. Wang, G. Zheng, W. Sun, and X. Chen. 2011. “Changes in Soil Organic Carbon, Nitrogen, pH and Bulk Density with the Development of Larch (Larix Gmelinii) Plantations in China.” Global Change Biology 17: 2657–2676. doi:https://doi.org/10.1111/j.1365-2486.2011.02447.x.
- Wu, G., X. Chen, J. Ling, F. Li, F. Li, L. Peixoto, Y. Wen, and S. Zhou. 2020a. “Effects of Soil Warming and Increased Precipitation on Greenhouse Gas Fluxes in Spring Maize Seasons in the North China Plain.” Science of the Total Environment 734: 139269. doi:https://doi.org/10.1016/j.scitotenv.2020.139269.
- Wu, H., X. Xu, W. Chen, and L. Han. 2020b. “Dissolved Organic Matter and Inorganic N Jointly Regulate Greenhouse Gases Fluxes from Forest Soils with Different Moistures during a Freeze-thaw Period.” Soil Science and Plant Nutrition 66 (1): 163–176. doi:https://doi.org/10.1080/00380768.2019.1667212.
- Xiang, W., L. Li, S. Ouyang, W. Xiao, L. Chen, P. Lei, X. Deng, Y. Zeng, J. Fang, and D. I. Forrester. 2021. “Effects of Stand Age on Tree Biomass Partitioning and Allometric Equations in Chinese Fir (Cunninghamia Lanceolata) Plantations.” European Journal of Forest Research 140: 317–332. doi:https://doi.org/10.1007/s10342-020-01333-0.
- Zeng, D., R. Mao, S. Chang, L. Jun, and D. Yang. 2010. “Carbon Mineralization of Tree Leaf Litter and Crop Residues from Poplar-based Agroforestry Systems in Northeast China: A Laboratory Study.” Applied Soil Ecology 44 (2): 133–137. doi:https://doi.org/10.1016/j.apsoil.2009.11.002.
- Zeng, L., J. Li, Z. Zhou, and Y. Yu. 2020. “Optimizing Land Use Patterns for the Grain for Green Project Based on the Efficiency of Ecosystem Services under Different Objectives.” Ecological Indicators 114: 106347. doi:https://doi.org/10.1016/j.ecolind.2020.106347.
- Zhang, Z., M. Huang, Y. Yang, and X. Zhao. 2020. “Evaluating Drought-induced Mortality Risk for Robinia Pseudoacacia Plantations along the Precipitation Gradient on the Chinese Loess Plateau.” Agric 284: 107897. doi:https://doi.org/10.1016/j.agrformet.2019.107897.
- Zhao, H., J. Sun, X. Xu, and X. Qin. 2017. “Stoichiometry of Soil Microbial Biomass Carbon and Microbial Biomass Nitrogen in China’s Temperate and Alpine Grasslands.” European Journal of Soil Biology 83: 1–8. doi:https://doi.org/10.1016/j.ejsobi.2017.09.007.
- Zhou, Z., Z. Li, K. Chen, Z. Chen, X. Zeng, H. Yu, S. Guo, et al. 2021. “Changes in Soil Physicochemical Properties and Bacterial Communities at Different Soil Depths after Long-term Straw Mulching under a No-till System.” Soil 7 (2): 595–609. doi:https://doi.org/10.5194/SOIL-7-595-2021.