ABSTRACT
This comparative investigation aimed to evaluate controlling factors for the changes of chemical and microbial properties in soils taken from chemical fertilizer experiments carried out mainly in Chernozem and Andosol in Hungary and Japan, respectively. The plant available nutrient content of the soil, the stock of C and N and the soil microbial parameters of the carbon and nitrogen cycles were examined under different land-use and vegetation. In the Japanese soils, there was about 2–3 times higher organic C content than in Hungarian soils. The average CO2-production was about 1.5 times higher in Hungary soils than Japanese soils, and significantly increased (9–37%) in the fertilized treatments compared to control in all Hungarian long-term experiments. Regarding the Hungarian soils, the NPK-fertilization especially increased the nitrate and phosphorus content of soils, among the microbial parameters of the CO2-production, MBC, MBN, net nitrification, saccharase and urease activities – in most cases significantly increased – among the six microbial examined parameters. With reference to Japanese soils, the nitrogen fertilization especially increased the nitrate and phosphorus content of soils and had the highest effect on the microbiological parameters in the forest soil, where the MBC, MBN, net nitrification, saccharase and urease activities increased significantly. Only the net nitrification increased significantly in the fertilized treatments in the all Numata experiments, and generally 10–100 times higher values were found in the Japanese soils than in the Hungarian ones. The microbial activity decreased according to land use in the following order: maize>forest>grassland in Hungary soils, and forest>grassland>apple orchards in Japanese soils. These results confirm that climate conditions and human activity have important impacts on the C and N cycles of the soil–plant–atmosphere system. Based on our results, it appears that land-use and chemical fertilization resulted in a larger change in the stock of organic matter and soil microbiological processes in the Hungarian long-term experiments compared to the Japanese experiments.
1. Introduction
Ecological conditions (climate, climate change, soil-forming rock, factors and processes of soil formation, natural vegetation) are more remarkable in the long term. At the same time, by selecting the cultivated plant species/variety, agro-technical methods can be professionally applied in the hope of successful farming. The replenishment of soil mineral and organic matter have an outstanding important among agro-technical methods.
Several organic and chemical long-term fertilization experiments have shown that chemical (Kátai Citation2006; Kátai and Zsuposné Citation2015; Zhong, Yan, and Shangguan Citation2015) and microbiological properties of soil (Hopkins and Shiel Citation1996; Kátai et al. Citation2014; Simon and Tako Citation2014; Geisseler and Scow Citation2014; Luo et al. Citation2015) can significantly altered. According to these experiment results, the element cycles, energy flow, biodynamics and activity of living organisms, as well as the growth of plants are all positively influenced by a balanced nutrient supply. The dynamics of carbon (C) and nitrogen (N) in ecosystems not only contribute to environmental problems (greenhouse gas productions, global warming, acid rain, nitrogen depositions, and eutrophication), but also affect soil fertility and crop productivity, and ultimately the sustainable development of humankind (Cheng Citation2020), which should be monitored carefully and continuously.
In previous soil monitoring investigations, soil respiration, nitrogen mineralization, microbial biomass and enzymes involved in the decomposition of organic matter were the most sensitive parameters for detecting changes in soil conditions (Powlson, Xu, and Brookes Citation2017) and were commonly used as microbial indicators (Szili-Kovács, Kátai, and Takács Citation2011). In our study, the CO2 production, N mineralization and nitrification were measured for the decomposition of soil organic matter and soil N. We determined the saccharase enzyme activity as glucose from the degradation of saccharose; the urease activity as ammonium from mineralization of urea. The source of nutrients and energy for most soil microorganisms is the stock of soil organic matter. Microbial biomass is the living matter of the soil (except for roots and terrestrial animals), is largely responsible for the transformation of soil organic matter, and is the major driving force in the cycle of elements (C, N, P, and S). At the same time, microbial biomass contributes to the expansion of plant nutrient stock (Inubushi, Sakamoto, and Sawamoto Citation2015). Microbial biomass is often referred as the engine of organic matter transformation (Powlson, Xu, and Brookes Citation2017).
Soils in agricultural and other crop ecosystems have lower carbon stocks than those under natural vegetation due to land use and erosion of soil organic carbon (OC) stocks. According to Lal (Citation2020), in agro-ecosystem soils, compared to natural ecosystems, OC depletion depends on soil texture and climate conditions, which is stronger in coarser sand than in finer-textured clay soils and more pronounced in tropical ecosystems than in temperate zones.
The organic stock of soils is one of the key parameters of soil. As the organic matter found in soil is one of the sources of plant nutrients, it is therefore important to maintain soil fertility, as well as to assist with water and air management (Sikora and Stott Citation1996). In recent centuries, concentrations of carbon dioxide in the atmosphere have increased from 278 ppm to 405 ppm, which has caused a 1°C increase in mean temperature along with other greenhouse gases (IPCC Citation2014). The increasing use of nitrogen fertilizers worldwide has contributed to the gradual increase of the atmospheric concentration of nitrogenous pollutants and CO2. The effect of this contamination can be significant when acid rain and nitrogen deposits act directly on plants, as well through acidification in the soil (Matsushima, Nagano, and Inubushi Citation2010). In permanent grasslands, Katja et al. (Citation2020) showed large differences in the amount of leached nitrogen (3–50 kg N ha−1 year−1) between dry and wet years and among different soil types.
Kong et al. (Citation2013a) reported the environmental consequences of soil management by comparisons between Hungarian and Japanese soils. According to their research, land use has a significant effect on the cumulative N2O and CO2 productions in soil, and among the land uses they examined, a larger amount of gases were emitted by apple culture compared to grassland or forest soils. Sakata et al. (Citation2015) measured the N2O and CO2 productions from tropical oil palm plantations and found that soil type significantly influenced gas productions, whereas fertilization did not. Fertilization under aerobic and flooded conditions increased the amount of greenhouse gases; CO2, CH4, N2O, differently (Inubushi et al. Citation2020). Wong et al. (Citation2020) showed that agricultural soils generally contain lower TC and TN than forest-covered soils, and that land use (vegetation) greatly affects the TC and TN dynamics of the soil. According to Wong et al. (Citation2020), the nutrient content of soils has decreased as follows: forest>rubber>oil palm plantation ecosystem.
In Hungary, we have been conducting ongoing long-term experiments in crop production and fertilization for more than three decades (Kátai Citation1994, Citation2006; Kátai et al. Citation2009, Citation2014, Citation2020). These experiments have primarily focussed on the nutrient requirements of crops (N, P, and K), and subsequently, our research has extended to the soil physical, chemical and microbiological effects on intensive nutrient supply. The C and N-stock of soils directly affects the soil microbiological processes. In this study, we focussed on CO2-production, mobilization of organic material, net-nitrification and soil acidification. In Numata Environmental Horticulture and Forest Experimental Farm, Chiba University in Gunma, Japan, an artificial N deposition field experiment was conducted for 19 months to investigate the effect of N addition on soil biochemical properties under orchard garden, grassland and forest vegetation.
We conducted Hungarian-Japanese research project, aiming to investigate the effects of land use change and N addition as long-term fertilization on soil biochemical properties. The Hungarian fertilized plots received NPK, while the Japanese only N- fertilizer. In this article, we discuss how the microbial processes related to the C- and N-cycles were changed as a result of nutrient supply in different long-term experiments.
2. Materials and methods
2.1. Study sites and samplings
The Hungarian soil samples originated from Debrecen University’s Demonstration Garden (from oak forest (Experimental code: DF) and grassland (DG)): two treatments, control and high doses of fertilizers, separately: ammonium nitrate, mono-ammonium phosphate and potassium chloride), and from the long-term experiments of Debrecen-Látókép (LE) (since 1983) and of Görbeháza (GE) (since 1988). The test plant was maize (Zea mays L.) in LE and GE and two treatments (control, and high doses of ammonium nitrate, mono-ammonium phosphate and potassium chloride) were chosen for investigation. shows the treatments’ details. Soil types are Chernozem in Debrecen and Vertisol in Görbeháza, according to Word Reference Base (WRB, Word Reference Base for Soil Resources Citation2014). The Hungarian soil sampling was done on 26 March 2017in DF, DG, as well as in LE and GE. The mean annual temperature in 2016 and 2017 were 11–13°C and 10–12°C, and the annual precipitation were 699 mm and 605 mm, respectively.
Table 1. The treatments of experiments, vegetation, soil types and places of sampling
On the Numata Environmental Horticulture and Forestry Experimental Farm of Chiba University (Experimental code: NE), Gunma Prefecture, Japan (established in 1948), the control and fertilized treatments with spitted application of ammonium nitrate solution with 3 month interval (May, August and November) at the total rate equivalent to 30 kg N ha−1 year−1 in forest vegetation, grassland and an apple orchard, were started in May 2011 as for simulation of acid deposition for 19 months. Soil type is Andosols and other details as described in Inubushi and Kong (Citation2014). Soil samples were collected on 28 November 2012. The mean annual temperature was 12.7°C in the Numata region, with the precipitation 1479 mm. The soil samples were stored in a refrigerator at 5°C and microbiological parameters were measured within 10 days after sampling. The laboratorial measurements were made in three replications.
2.2. Analysis of physical, chemical and microbial properties of soils
Moisture content of soil was measured by oven drying at 105°C for 24 h, and expressed as m/m%, based on the absolute mass of dry soil. Determination method of silt and clay content (their diameter <0.02 mm) is based on Stoke’s Law governing the rate of sedimentation of particles in water. The combined ratio of clay and silt of soil is expressed as m/m% of soils. The pH value was measured in distilled water and in 1 mol L−1 potassium chloride (KCl) solution with 1:2.5 extracting ratio (Buzás Citation1988).
The analysis of NO3-N content of soils was based on the method that used Na-salicylate as a reagent (Pansu and Gantheyrou Citation2006). Determinations of the extractable phosphorus and potassium content of soils were made using a 0.1 mol L−1 ammonium lactate–acetic acid (AL) solution (Buzás Citation1988). The organic carbon (OC) and total nitrogen (TN) were determined by the Kjeldahl method (Pansu and Gantheyrou Citation2006). The CO2-production was measured by a 10-day soil incubation at 25°C (Schinner et al. Citation1996), with NaOH trapping. The microbial biomass carbon (MBC) and nitrogen (MBN) were measured by chloroform fumigation-extraction methods (Vance, Brookes, and Jenkinson Citation1987). The net nitrification rate was measured after a 14-day soil incubation at 27°C, with a reagent of Na-salicylate. Saccharase activity measuring methods was done according to Frankenberger and Johanson (Citation1983), while urease activity measurement was based on the quantitative determination of ammonia by spectrophotometric methods (640 nm) (Schinner et al. Citation1996).
2.3. Statistical evaluation
The sampling average, standard deviation and for comparison of two treatments One Sample T Test (p ≤ 0.05) were calculated, with SPSS 27.0 and Excel 2016 programs. Pearson correlation and regression analyses were used to establish relationships between soil properties.
3. Results
3.1. Physical and chemical characteristics of experimental soils
We found moisture content in the soils of Japanese experiments 1.5–2 times higher than in Hungarian soils (), which is mainly due to the higher amount of local precipitation and the higher stock of organic matter in the soils. Due to the different parent materials in the two countries the silt and clay % is much higher in the Hungarian soils (48–60%), than in Japanese soils (8–20%).
Table 2. Moisture, silt and clay contents, pH and nutrient content in experimental soils
Regarding the pH value, the Hungarian soils are nearly neutral, with the soil in the Látókép experiment being slightly acidic (LE), and soils from Debrecen (DG, DF) and Görbeháza (GE) slightly alkaline. Among Japanese soil, the pH value was nearly neutral under grassland (NG), while soils in the two other sampling places were acidic (NF, NO). In some Hungarian experiment decreased the pH in the fertilized treatments such as GE also in Japanese soils, long-term land-use change since 1948 did cause significant shift down of soil pH in NO to compare with NF and NG.
The fertilization significantly increased the nitrate, AL-soluble phosphorus and potassium content of soils in almost all soil samples, expect the potassium content of soils from Debrecen (DF and DG). Fertilization resulted in the largest change in AL-soluble phosphorus content in the LE and GE experiments. The nitrate content of the Japanese control soils was more or less similar to that of the Hungarian soils. The Japanese forest soil (NF) is very poor in phosphorus, while the other two sampling places contained substantial amounts of phosphorus, especially in the fertilized plots of the orchard soil.
3.2. Microbiological parameters related to the carbon cycle in the experimental soils
In the GE experiment, fertilization did not affect the amount of OC, while fertilization significantly increased the CO2-production, and saccharase activity (). These parameters were remarkably high in GE compared to the other Hungarian experiments. In the LE, MBC were increased significantly (42%), while in DG, CO2 production and saccharase activity were increased significantly. It is noteworthy that fertilization significantly increased the CO2 production (9–37%) and saccharase activity (1.3–2.7 times) compared to the control in all Hungarian experiments (except in LE).
Table 3. Effect of fertilization on microbial parameters of the carbon cycle in soils
The average content of OC and MBC in Japanese soils was almost three times higher than in Hungarian soils. The saccharase activity measured in the soils of the Hungarian experiments proved to be almost 50% higher, while the CO2 production proved to be 30% higher than the Japanese soils. The activity of saccharase increased significantly after fertilization in DF, DG, GE (60%), and in NF (70%) respectively.
In the soils of the Japanese experiment, a small change was observed as a result of fertilization. In the NG and NO, fertilization decreased saccharase activity, while the values of MBC increased significantly in the NF fertilized treatment (37%).
3.3. Microbiological parameters related to the nitrogen cycle in the experimental soils
Fertilization did not affect the amount of TN. The net nitrification increased significantly in the three Numata Experiments (NF, NG and NO), and generally 10–100 times higher values were found in Japanese soils than in the Hungarian ones (). Urease activity was also significantly stimulated in LE (20%) and GE (30%) by fertilization, whereas in NF of the Japanese soils (20%). A significant decrease in urease activity was observed in DF, DG, NG and NO. MBN showed a significant increase in fertilizer treatments in DF, DG, GM of Hungarian experiments and in the case of NF, but not in the others.
Table 4. Effect of fertilization on microbial parameters of the nitrogen cycle in soils
Evaluating the studied parameters of the nitrogen cycle, we can state that urease activity and net nitrification was an order of magnitude highest in the NF and NG experimental fields compared to the other ones. In the Hungarian experiments (LE, GE), fertilization mainly resulted in an increase in urease activity.
3.4. Microbial quotients and specific respiratory activity in the experimental soils
In the Japanese soils, the C/N ratio was higher than in the Hungarians (), but fertilization did not change C/N ratios, significantly. The MBC/MBN ratio varied between 6.8 and 10.9 in all Hungarian soils, while remarkably higher values (20.2–26.0) were calculated in the soils of NF and NO. Fertilization only caused a significant increase in the NF experiment. The microbial quotient (MBC/OC) represents similar ranges in the Hungarian (0.71 − 1.16%) and Japanese (0.42–1.29%) experiments ().
3.5. Correlation analysis between physical, chemical and microbial parameters of soils
Relation between physical, chemical and microbial parameters () showed that the silt and clay content of the Hungarian soils have a positive correlation with saccharase activity (r = 0.595*, p < 0.05), CO2-production (r = 0.858**, p < 0.01) and MBN values (r = 0.699*), while the silt and clay content of the Japanese soil was negatively correlated with four parameters, as follows: saccharase (r = −0.892**) and urease activity (r = −0.987**), MBC (r = −0.839**) and MBN (r = −0.678*). The silt and clay content negatively correlated with the urease activity in both the Hungarian and the Japanese soils (HUN r = −0.917**; JPN r = −0.987**). There were positive strong relationships between moisture content and urease activity (HUN r = 0.855**; JPN r = 0.929**) in both soil type. However, in Japanese soils, a positive correlation was observed between soil moisture content and MBC values (r = 0.971**) as well as medium–strong relationship saccharase activity (r = 0.636*).
Table 5. Relationships between the physical, chemical, and microbial characteristics of the experimental soils (r – values)
In the case of Japanese soils, the pH(KCl) value was in a positive correlation with saccharase activity (r = 0.831**) as well as with MBN values (r = 0.981**). In Hungarian soil the pH(KCl) was also positive correlation with the urease activity (r = 0.719**).
We proved correlation in opposite direction between nitrate content and CO2 production of experimental soils (HUN r = −0.951**; JPN r = 0.813**), while in Hungarian soil the nitrate content correlate with the urease activity (r = 0.870**). Among the soil soluble nutrient content, the AL-phosphorus correlate with the saccharase activity negatively (HUN r = −0.900**; JPN r = −0.775*), and the AL-potassium with the CO2-production positively (HUN r = 0.988**; JPN r = 0.596*) on both soil. The organic carbon content (OC) of the Hungarian soils showed positive correlations with MBC (r = 0.929**) and MBN (r = 0.874**). Regarding to Japanese soils, the CO2 production correlated with OC (r = 0.808**) and organic nitrogen (TN) (r = 0.907**).
Our results showed positive correlations between OC and CO2 production (HUN r = 0.586*; JPN r = 0.808**). The slope of the regression equation was higher in the Japanese soils, probably due to the greater organic matter content of the soil (). They were proved by the results of Inubushi and Kong (Citation2014), since the MBC/OC in Andosols was much lower than those in non-Andosols, indicating that Andosols contain more stable carbon fixed with active aluminum than in non-Andosols (Wada Citation1986; Shirato, Hakamata, and Taniyama Citation2004).
Figure 1. Relationship between organic carbon (OC) and CO2-production (Japan n = 18; Hungary n = 24).
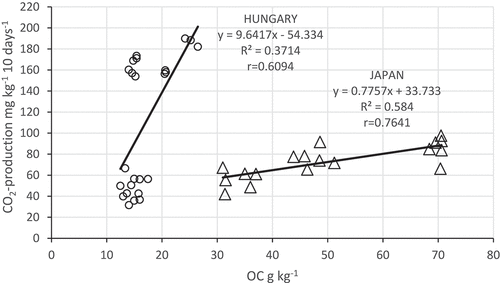
Also, positive correlations were observed between MBC and CO2 production (HUN r = 0.617*; JPN r = 0.451*; ). Other correlations were between OC and MBC content () in the soils of the two countries, but the slopes were almost the same in the two countries.
Table 6. Relationships between the microbial characteristics of the experimental soils (r – values)
Figure 2. Relationship between organic carbon (OC) and soil microbial biomass C (MBC) (Japan n = 18; Hungary n = 24).
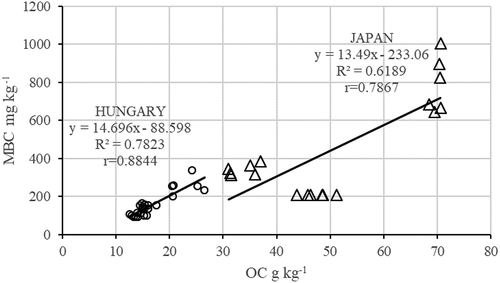
Other correlations between the microbial parameters of the Hungarian and the Japanese soils were presented in , including urease and net nitrification. also presents the relationship between MBC and saccharase enzyme activity in both Hungarian and Japanese soils.
Figure 3. Relationship between soil microbial biomass C (MBC) and saccharase enzyme activity (Japan n = 18; Hungary n = 24).
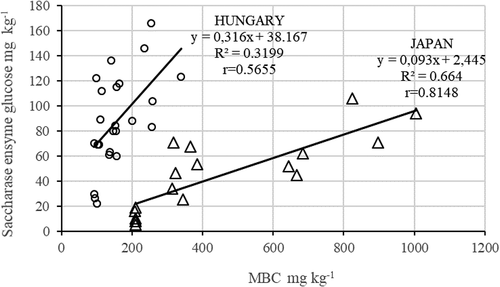
It might be an evidence the closer or moderate correlation between OC and CO2 production, between OC and MBC, or saccharase activity and MBC. We consider it is remarkable that our results from several experimental fields and large differences can be observed between the correlations of the parameters of Hungarian and Japanese soils. Although the relationship system might be evident, it is important to notice that the same relationship cannot be observed in case of elements of the N cycle (TN and nitrate-nitrogen; ON and MBN; urease activity and MBN).
4. Discussion
A comparative study of the carbon and nitrogen cycle parameters was performed on soils taken from the Hungarian and Japanese long-term fertilization experiments to evaluate influencing factors of land use and nutrient management on soil physicochemical and microbial properties.
The results of the comparative studies show that the differences in the physical and chemical properties of the soil (moisture contant, silt and clay, pH, mineral nutrient content, organic matter) greatly influence the microbiological processes and activity of the soils. Thus, we tried to evaluate our results in the knowledge of the most important soil properties.
Among soil physical parameters, the examined Hungarian soils had much (3–5 times) higher silt and clay content (48–61%) than the examined Japanese Andosols (9–20%). This is due to the longer soil formation period of Hungarian soils and to the parent material of Hungarian (sedimentation rock, loess) different from Japanese (volcanic ash). The Hungarian soils contain about half amount of moisture, than Japanese ones, which is due to large difference in annual precipitation (average 550–600 mm versus 1500 mm approximately for Hungary and Japan, respectively).
Regarding the pH value, the Hungarian soils are nearly neutral, with the soil in the Látókép experiment being slightly acidic (LE), and soils from Debrecen (DG, DF) and Görbeháza (GM) slightly alkaline. Among Japanese soil, the pH value was nearly neutral under grassland, while soils in the two other sampling places were acidic (NF, NO), again due to precipitation and parent materials. Organic matter content was high in Japanese soils, as the organic matter accumulates more in the Andosols (Wada Citation1986).
4.1. Effect of fertilization on soil of the experiments
According to our results – especially in the Hungarian fertilization experiments – the pH of the soil decrease by fertilization, but not significantly, so the fertilization can contribute to the acidification of the soils in some extent.
The Hungarian soils contained significantly less nitrate than the Japanese Andosols, while the addition of nitrogen fertilizer in all cases increased this parameter. The higher nitrate content of the Japanese soils is due to the larger organic matter content, which can supply nitrate through its mineralization and nitrification. The quantity of AL-P2O5 (ammonium-lactate soluble phosphorus) was higher in the Japanese soils, except in uncultivated forest soil, than in the Hungarian soils. The lowest AL-P2O5 was found in the control forest soil in Japan. As results of the long-term mineral fertilizer application under maize, the AL-P2O5 were significantly higher in fertilized plots than in control plots of the Hungarian experiments. Kátai (Citation2006) had previously reported that the fertilization as nutrient addition increased the nitrate content, the AL-soluble phosphorus and potassium content of soils in all experiments under various managements.
4.2. C cycle of experiments
Among the Hungarian soils, the Vertisol has the highest OC content; while the OC content of the Japanese soils was 2–3 times higher than Hungarian soils. The OC content in decreasing order in Japanese soils was: NF > NO > NG. In Hungary, significantly higher CO2-production was measured under maize culture than in all investigated Japanese soils. According to Kátai et al. (Citation2014), the amount of organic carbon (OC) and nitrogen (ON), as well as the quantity of MBC and MBN, were increased by fertilization in the long-term fertilizer experiment (LE). Geisseler and Scow (Citation2014) found that mineral fertilization increased soil organic carbon (OC) content and contributed to MBC growth. Evaluating the results of more than 60 long-term experiments, it was found that fertilization resulted in a 15% increase in MBC, compared to the unfertilized control. The effect of fertilizer was mainly observed in long-term experiments longer than 20 years. Hobbie (Citation2000) verified that the N addition would increase the N mineralization rate and thus promote CO2-production. Li et al. (Citation2012) also found that an elevated level of nitrogen fertilizer dosage exposure significantly increased CO2-productions on grassland. We confirmed this finding as the CO2-production increased due to mineral fertilization in Hungarian soils. As an exception, Compton et al. (Citation2004) established that N inputs can reduce the amount of soil microorganisms and their activities and thus restrict CO2-production in Andosols.
Fertilization significantly increased the microbiological index only in LE. According to Szili-Kovács, Kátai, and Takács (Citation2011), the microbial biomass is the living components of the soil and it constitutes less than 5% of the organic matter content in the soil. The specific respiratory activity (CO2/MBC), which expresses the respiration of microbial biomass per unit (Szili-Kovács, Kátai, and Takács Citation2011), in the Hungarian experiment ranged from 0.29–1.45, while in the Japanese experiments it varied between 0.09–0.37. Fertilization resulted in a significant increase in the respiratory index in the DF and DG experiments. According to Anderson (Citation2003), two variables (CO2/MBC and MBC/OC) express the energy efficiency of the microbial community. Higher specific respiratory activity means that more energy is needed to maintain the microbial biomass. This may also indicate the degradation or stress of the microbial communities (Brookes Citation1995). Those higher values in Látókép (LE) and Görbeháza (GE) in this study () indicate that these long-term experiments are located in the areas of intensive farming.
4.3. N cycle of experiments
The average values of net nitrification are 27 times, under the forest almost 30 times, and under the grass, almost 50 times higher in Japanese soils, compared to the Hungarians (). The rate of net nitrification in the Japanese soils decreased in the following order: forest (NF) grassland (NG) ≧ orchard (NO). According to Matsushima and Chang (Citation2007), the highest net-nitrification was observed when fertilizers were used for several years without grass. The smallest value was measured in the control with grass. A strong decrease in soil MBC was observed after N-addition (Ramirez, Craine, and Fierer Citation2012). Soil CO2-production and MBC reduction were closely correlated with the duration and amount of N added to the ecosystem.
Szili-Kovács et al. (Citation2007) showed that added organic matter (sucrose and sawdust) to the soil increased nitrogen immobilization in microbial biomass. N availability and inorganic nitrogen forms decreased in soil, at the same time, MBC and MBN increased significantly after the use of C addition. This result also proves that the cycle of C and N can be greatly influenced by human activity. According to Zhong, Yan, and Shangguan (Citation2015), in a fertilization long-term experiment where different amounts of N fertilizer were applied, CO2-productions also increased. It was found that microbial activity decreased above the application of 180 kg ha−1 year−1 of N-fertilizer. According to our experience in LE experiment, the microbial activity has not decreased in the treatments of 240 kg ha−1 year−1 N fertilizer.
4.4. Effect of vegetation on microbial processes
Comparing the amount of MBC, it was higher under forests, 4.7 times, while under grasses 3.7 times in Japanese soils, than in Hungarian ones (). In the values of the MBC, the following order was found: NF > NG > GM > NO > DM ~ DF ≧ DG.
The microbial activity of Japanese soils decreased according to land use in the following order: forest > grassland > apple orchards. Vegetation affected the soil properties in the two countries, and higher moisture content was observed under forest vegetation than in another plant cover, which confirms the favorable water retention function of woodland. Similar to Smith and Paul (Citation1990), the values of microbial biomass increase in the following order: arable < forest < grassland. The average microbial biomass can be 700, 850, 1090 kg C ha−1 for arable, forest and grassland, respectively. The values can change over a wide range and also overlapped. In contrast, in the Japanese soil, NF showed the highest MBC, followed by NG and NO, in this order. Kong et al. (Citation2013b) and Nagano et al. (Citation2013) showed the same order in this NE site, indicating consistent impact of land use on soil microbial properties.
Kong et al. (Citation2013b) found that land use has a significant effect on cumulative N2O-production and CO2-production. In the apple orchard soil, higher N2O and CO2-productions were found than for grassland or forest soil. Net nitrification also showed positive correlations with CO2 and N2O productions in these soils.
Ste-Marie and Paré (Citation1999) observed that net nitrification has been associated with soil type and type of trees in boreal forests. Their results indicated that nitrate production and consumption can be caused by the leaching of cations and can reduce the available nitrogen content, which can lead to soil acidification.
The urease and saccharase activities were higher in triculture maize than in the monoculture experiments (Kátai Citation2006). Kátai et al. (Citation2014) experienced that the CO2-production and the net-nitrification also increased, while the activity of saccharase and urease decreased in the fertilized plots. Xu, Inubushi, and Sakamoto (Citation2006) found that the MBC and qCO2 varied depending on tree species and temperatures in moderate climatic conditions. A high C/N ratio and slight soil acidification can contribute to decreasing MBC and increasing qCO2.
Based on our results, we can state that the CO2-production, saccharase activity and MBC content of the experimental fields were also different. In the Hungarian experiment fields, these parameters were greatly increased by long term NPK fertilization. This change was due to the direct effect of fertilization, as larger amounts of stem and root biomass were returned to the soil year after year in fertilized treatments, which were a suitable substrate for the enzyme.
These experimental results have proved that, under various climate conditions, fertilizer application and actual land use have large influences on the soil–plant–atmosphere systems, through the processes of the C and N cycles in the soil. This phenomenon may cause numerous unexpected consequences on the environment, with greenhouse gas productions, soil acidification and nitrate leaching, especially if farming is not properly done.
5. Conclusions
The investigations of the Hungarian and the Japanese soil samples from long-term fertilization experiments demonstrate that the moisture content and the organic matter content of soils have decisive influences on the mineral nutrient content and microbial processes in the soils. The plant available nutrient content of soils increased in the fertilized treatments of the investigated Hungarian and Japanese soils, with the higher doses causing the larger nutrient increase, also leading to greater acidification in some of the Hungarian fertilized plots. The fertilization significantly increased CO2-production and saccharase activity in all Hungarian experiments (except in the LE experiment). The average content of OC and MBC in Japanese soils was almost three times higher than in Hungarian soils. The saccharase activity measured in the soils of the Hungarian experiments proved to be almost 50% higher, while the CO2-production proved to be 30% higher.
In the soils of the Japanese experiment, a small change was observed as a result of fertilization. In the NG and NO experiments, in the fertilized plots decreased the saccharase activity, while the values of MBC significantly increased in the NF. Japanese Andosols have higher soil microbial activity through the measured microbial parameters. In addition to different land use, the microbiological activity of the Japanese soils decreased in the following order: forest > grassland > apple orchard. This is the order for Hungarian soils: maize > forest > grassland.
According to the mathematical-statistical evaluation, close positive correlations were found in all soils of the two countries fertilization long-term experiments between OC and CO2; OC and MBC; MBC and CO2; MBC and saccharase activity; MBN and saccharase activity. These results confirmed that climate factors and human activity have important impacts on the C and N cycles of the soil–plant–atmosphere: it influences the accumulation of organic matter, net nitrification, CO2-productions, and the acidification of the soils.
C and N cycles are more intensive in the Japanese soils compared to the Hungarian soils, which have different vegetation. By understanding the processes and the intensity of the soil-plant-atmosphere system in different ecosystems more precisely, in addition to employing various agro technical methods (land use, nutrient supply) under various climatic conditions, this system can be more effectively and sustainably protected against the unfavorable human activity.
Acknowledgments
Research work was carried out within the framework of the Hungarian-Japanese Intergovernmental Cooperation (2016-2017) “Comparative assessment of carbon-nitrogen cycle in the soil-plant systems of Hungarian and Japanese long-term experiments” The project was funded by the National, Research, Development and Innovation Fund, Hungary and JSPS-HAS.
We are also grateful to Professor Péter Pepó and Professor Mihály Sárvári for joining to the research work of the long term experiment they led.
Disclosure statement
No potential conflict of interest was reported by the authors.
Additional information
Funding
References
- Buzás, I., Ed. 1988. Soil and Agrochemical Test Methodology 2. Physico-chemical and Chemical Test Methods of Soils. Budapest: Agricultural Publishing House. 90–93. in Hungarian.
- Anderson, T. H. 2003. “Microbial Eco-Physiological Indicators to Asses Soil Quality.” Agriculture, Ecosystems & Environment 98: 285–293. doi:https://doi.org/10.1016/S0167-8809(03)00088-4.
- Brookes, P. C. 1995. “The Use of Microbial Parameters in Monitoring Soil Pollution by Heavy Metals.” Biology and Fertility of Soils 19: 269–279. doi:https://doi.org/10.1007/BF00336094.
- Cheng, W. 2020. “Soil Carbon and Nitrogen Dynamics by Land Use and Management Changes in East and Southeast Asian Countries (Soil C and N by LUMC).” Soil Science and Plant Nutrition 66: 34–36. doi:https://doi.org/10.1080/00380768.2020.1718923.
- Compton, J. P., L. S. Watrud, L. A. Porteous, and S. Degrood. 2004. “Response of Soil Microbial Biomass and Community Composition to Chronic Nitrogen Additions at Harvard Forest.” Forest Ecology and Management 196: 143–158. doi:https://doi.org/10.1016/j.foreco.2004.03.017.
- Frankenberger, W. T., and J. B. Johanson. 1983. “Method of Measuring Invertase Activity in Soils.” Plant and Soil 74: 301–311. doi:https://doi.org/10.1007/BF02181348.
- Geisseler, D., and K. M. Scow. 2014. “Long-term Effects of Mineral Fertilizers on Soil Microorganisms – A Review.” Soil Biology & Biochemistry 75: 54–62. doi:https://doi.org/10.1016/j.soilbio.2014.03.023.
- Hobbie, S. E. 2000. “Interactions between Lignin and Soil Nitrogen Availability during Leaf Litter Decomposition in a Hawaiian Montane Forest.” Ecosystems 3: 484–494. doi:https://doi.org/10.1007/s100210000042.
- Hopkins, D. W., and R. S. Shiel. 1996. “Size and Activity of Soil Microbial Communities in Long-term Experimental Grassland Plots Treated with Manure and Inorganic Fertilizers.” Biology and Fertility of Soils 22: 66–70. doi:https://doi.org/10.1007/BF00384434.
- Inubushi, K., K. Sakamoto, and T. Sawamoto. 2015. “Propeties of Microbial Biomass in Acid Soil and Their Turnover.” Soil Science and Plant Nutrition 51: 605–608. doi:https://doi.org/10.1111/j.1747-0765.2005.tb00073.x.
- Inubushi, K., M. Yashima, S. Hanazawa, A. Goto, K. Miyamoto, T. Tsuboi, and G. Asea. 2020. “Long-term Fertilizer Management in NERICA Cultivated Upland Affects on Soil Bio-chemical Properties.” Soil Science and Plant Nutrition 66: 247–253. doi:https://doi.org/10.1080/00380768.2019.1705738.
- Inubushi, K., and Y. Kong (Chapter 18). 2014. “Soil Microbial Biomass and C Storage of an Andosol.” In Soil Carbon, edited by H. A. E and K. Mc Sweeney, 173–178. Heidelberg: Springer.
- IPCC. 2014. “Summary for Policymakers.” In Climate Change 2014, Mitigation of Climate Change. Contribution of Working Group III to the Fifth Assessment Report. Intergovernmental Panel on Climate Change, 8. Cambridge, United Kingdom and New York, USA: Cambridge University Press.
- Kátai, J., O. Á Zsuposné, Z. Sándor, and M. Tállai. 2009. “Soil Microbiological Consequences of the Stress Effect of Soil Acidification in a Long Term Experiment.” In Cereal Research Communications, 403–406. Vol. 37, Budapest, Hungary: Academic Publisher. Supplement.
- Kátai, J., and O. Á. Zsuposné. 2015. “Changes in Soil Chemical Features and Microbiological Characteristics Connecting to Carbon- and Nitrogen Cycles in a Hungarian Long-term Experiment.” In Aspects of Applied Biology 128, Valuting Long-term Experiments for Agriculture and Ecology, edited by P. Simon, M. S. Barbara, A. S. Elizabeth, and W. Christine, 225–230. Warwick: Warwick Enterprise Park.
- Kátai, J., O. Á. Zsuposné, M. Tállai, and T. Alshaal. 2020. ““Would Fertilization History Render the Soil Microbial Communities and Their Activities More Resistant to Rainfall Fluctuations?”” Ecotoxicology and Environmental Safety 201: 15. doi:https://doi.org/10.1016/j.ecoenv.2020.110803.
- Kátai, J., O. Á. Zsuposné, Z. Sándor, and M. Tállai. 2014. “Comparison of Soil Parameters of the Carbon and Nitrogen Cycles in a Long-term Fertilization Field Experiment.” Agrochemistry and Soil Science 63: 129–138.
- Kátai, J. 1994. “The Effect of Different Ways of Nutrient Supply on the Soil Microflora and Activity.” In Soil, Plant and Environment Relationships, edited by J. Nagy, 210–219. Debrecen, Hungary: Printed by REXPO , October 1997.
- Kátai, J. 2006. “Changes in Soil Characteristics in a Mono- and Triculture Long-term Field Experiment.” Agrochemistry and Soil Science 55: 183–192.
- Katja, K., F. Louault, I. Bosio, L. Andanson, and O. Darsonville. 2020. “Risk Analysis of N Leaching from Grasslands under Different Management Practices.” In ISCRAES Book of Abstract Series 1; Published in 2020 by the CRAES Group, edited by M. I. Khalil and B. A. Osborne, 62. Ireland: University College Dublin and Prudence College Dublin.
- Kong, Y., H. Nagano, J. Kátai, I. Vágó, O. Á. Zsuposné, M. Yashima, and K. Inubushi. 2013a. “CO2, N2O and CH4 Production/consumption Potentials of Soils under Different Land-use Types in Central Japan and Eastern Hungary.” Soil Science and Plant Nutrition 59: 455–462. doi:https://doi.org/10.1080/00380768.2013.775005.
- Kong, Y., M. Watanabe, H. Nagano, K. Watanabe, M. Yashima, and K. Inubushi. 2013b. “Effect of Land-use Type and Nitrogen Addition on Nitrous Oxide and Carbon Dioxide Production Potentials in Japanese Andosol.” Soil Science and Plant Nutrition 59: 790–799. doi:https://doi.org/10.1080/00380768.2013.817938.
- Lal, R. 2020. “Managing Soils for Negative Feedback to Climate Change and Positive Impact on Food and Nutritional Security.” Soil Science and Plant Nutrition 66: 1–9. doi:https://doi.org/10.1080/00380768.2020.1718548.
- Li, K., Y. Gong, W. Song, G. He, Y. Hu, C. Tian, and X. Liu. 2012. “Responses of CH4, CO2 and N2O Fluxes to Increasing Nitrogen Deposition in Alpine Grassland of the Tianshan Mountains.” Chemosphere 88: 140–143. doi:https://doi.org/10.1016/j.chemosphere.2012.02.077.
- Luo, P., X. Han, Y. Wang, M. Han, H. Shi, N. Liu, and H. Bai. 2015. “Influence of Long-term Fertilization on Soil Microbial Biomass, Dehydrogenase Activity, and Bacterial and Fungal Community Structure in a Brown Soil of Northeast China.” Annals of Microbiology 65: 533–542. doi:https://doi.org/10.1007/s13213-014-0889-9.
- Matsushima, M., H. Nagano, and K. Inubushi. 2010. “Global Nitrogen Cycling and Its Availability from Soils.” In Nitrogen Assimilation in Plants, edited by O. T, and K. Sueyoshi, 19–32. Kerala, India: Research Signpost.
- Matsushima, M., and S. X. Chang. 2007. “Effects of Understory Removal, N Fertilization, and Litter Removal on Soil N Cycling in a 13-year-old White Spruce Plantation Infested with Canada Bluejoint Grass.” Plant and Soil 292: 243–258. doi:https://doi.org/10.1007/s11104-007-9220-x.
- Nagano, H., T. Kasahara, M. Takahashi, R. Yoshioka, Y. H. Kong, M. Yashima, M. Okazaki, et al. 2013. “Temporal and Spatial Variation in Physicochemical and Microbial Properties of Soils in Chiba University’s Environmental Horticulture and Forestry Farm – Comparative Research of the Results in 2002, 2003 and 2011.” HortResearch 67. 21–27. in Japanese with English summary.
- Pansu, M., and J. Gantheyrou. 2006. Handbook of Soil Analysis. Berlin Heilderberg: Springer-Verlag.
- Powlson, D. S., J. Xu, and P. C. Brookes. 2017. “Through the Eye of the Needle — The Story of the Soil Microbial Biomass.” In Microbial Biomass – A Paradigm Shift in Terrestrial Biogeochemistry, K. Tate edited by, 1–40. Singapore: World Scientific. https://doi.org/10.1142/9781786341310_0004c.
- Ramirez, K. S., J. M. Craine, and N. Fierer. 2012. “Consistent Effects of Nitrogen Amendments on Soil Microbial Communities and Processes across Biomes.” Global Change Biology 18: 1918–1927. doi:https://doi.org/10.1111/j.1365-2486.2012.02639.x.
- Sakata, R., S. Shimada, H. Arai, N. Yoshioka, R. Yoshioka, H. Aoki, N. Kimoto, A. Sakamoto, L. Melling, and K. Inubushi. 2015. “Effect of Soil Types and Nitrogen Fertilizer on Nitrous Oxide and Carbon Dioxide Productions in Oil Palm Plantations.” Soil Science and Plant Nutrition 61: 48–60. doi:https://doi.org/10.1080/00380768.2014.960355.
- Schinner, F., R. Öhlinger, E. Kandeler, and R. Margesin, ed. 1996. Berlin, Heidelberg, New York: Springer-Verlag. 435. ISBN-13: 978-3-642-64633-1.
- Shirato, Y., T. Hakamata, and I. Taniyama. 2004. “Modified Rothamsted Carbon Model for Andosols and Its Validation: Changing Humus Decomposition Rate Constant with Pyrophosphate-Extractable Al.” Soil Science and Plant Nutrition 50: 149–158. doi:https://doi.org/10.1080/00380768.2004.10408463.
- Sikora, L. J., and D. E. Stott. 1996. “Soil Organic Carbon and Nitrogen.” In Methods for Assessing Soil Quality, edited byJ. W. Doran and A. J. Jones, 157–168. SSSA Special Publication 49, SSSA, Madison, WI.
- Simon, T., and A. Tako. 2014. “Influence of Long-term Application of Organic and Inorganic Fertilizers on Soil Properties.” Plant, Soil and Environment 60: 314–319. doi:https://doi.org/10.17221/264/2014-PSE.
- Smith, J. L., and E. A. Paul. 1990. “The Significance of Soil Microbial Biomass Estimations.” In Soil Biochemistry, edited by B. J-m, and G. Stotzky, 357–396. Vol. 6. New York and Basel: Marcel Dekker.
- Ste-Marie, C., and D. Paré. 1999. “Soil, pH and N Availability Effects on Net Nitrification in the Forest Floors of a Range of Boreal Forest Stands.” Soil Biology & Biochemistry 31: 1579–1589. doi:https://doi.org/10.1016/S0038-0717(99)00086-3.
- Szili-Kovács, T., J. Kátai, and T. Takács. 2011. “Application of Microbiological Indicators for Soil Quality Assessment. Methods 1.” Agrochemistry and Soil Science 60. 273–286. in Hungarian.
- Szili-Kovács, T., K. Török, E. L. Tilston, and D. W. Hopkins. 2007. “Promoting Microbial Immobilization of Soil Nitrogen during Restoration of Abandoned Agricultural Fields by Organic Additions.” Biology and Fertility of Soils 43: 823–828. doi:https://doi.org/10.1007/s00374-007-0182-1.
- Vance, E. D., P. C. Brookes, and D. S. Jenkinson. 1987. “An Extraction Method for Measuring Soil Microbial Biomass-C.” Soil Biology & Biochemistry 19: 703–707. doi:https://doi.org/10.1016/0038-0717(87)90052-6.
- Wada, K. 1986. “Andosoils in Japan.” In Morphology and Classification, edited by M. Otowa, 3–19. Fukuoka, Japan: Kyushu University Press.
- Wong, M.-K., P. Selliah, T.-F. Ng, M. H. A. Hassan, E. Van Ranst, and K. Inubushi. 2020. “Impact of Agricultural Land Use on Physicochemical Properties of Soils Derived from Sedimentary Rocks in Malaysia.” Soil Science and Plant Nutrition 66: 214–224. doi:https://doi.org/10.1080/00380768.2019.1705180.
- WRB, Word Reference Base for Soil Resources. 2014. “IUSS Working Group. International Soil Classification System for Naming Soils and Creating Legends for Soil Maps.” In World Soil Resources Reports, No. 106. Rome: FAO.
- Xu, X., K. Inubushi, and K. Sakamoto. 2006. “Effect of Vegetation and Temperature on Microbial Biomass Carbon and Metabolic Quotients of Temperate Volcanic Forest Soils.” Geoderma 136: 310–319. doi:https://doi.org/10.1016/j.geoderma.2006.03.045.
- Zhong, Y., W. Yan, and Z. Shangguan. 2015. “Impact of Long-term N Additions upon Coupling between Soil Microbial Community Structure and Activity, and Nutrient-use Efficiencies.” Soil Biology & Biochemistry 91: 151–159. doi:https://doi.org/10.1016/j.soilbio.2015.08.030.