ABSTRACT
In organic paddy fields, one of the toughest weeds to control is Monochoria vaginalis (Burm. f.) Kunth. The application of fresh rice (Oryza sativa L.) bran to soil suppresses the germination of this weed. In stark contrast, the scarcely decomposable component of this material stimulates the germination. This review focuses on the elucidation of these conflicting traits. One of the chemical factors for the suppression is toxic substances such as organic acids. In addition, rice bran application enhances the piling of small soil particles by tubificids on the soil surface. This layer acts as a physical factor that slows germination, presumably because its fine grains prevent light from reaching the seed. The newly accumulated soil with small particle was sampled for the analysis of the settled soil volume in water (SSVW). This value corresponds to the mud volume in water per dry weight. The electrical conductivity (EC) of the soil solution and the SSVW of the soil surface are useful indicators to evaluate the chemical and physical factors that control germination, respectively. EC and SSVW have been traced in paddy fields where rice bran was applied. The chemical and physical factors were active during the earlier and latter halves of the month after transplanting, respectively. These factors worked in a complementary manner. Paddy soil is typically equipped with the ability to reduce oxygen (O2). This trait enhances the number of M. vaginalis plants because hypoxic conditions are preferable for germination. Anaerobic soil conditions are not required for the expression of O2-reducing power. A long-term application of rice bran might intensify this power because a scarcely decomposable component of rice bran contains a significant amount of O2-reducing substances. The presence of conflicting factors that suppress and enhance germination might destabilize the efficacy of the rice bran.
1. Introduction
Since the late 1990s, the global market for organic food and area under organic production have expanded (Reganold and Wachter Citation2016). The Ministry of Agriculture, Forestry and Fisheries of Japan launched the ‘Measures for achievement of Decarbonization and Resilience with Innovation (MeaDRI)’ in May 2021. In this strategy, one of the targets is to increase the area of organic farming to 1 Mha (equivalent to 25% of farmland), by 2050. If rice (Oryza sativa L.) farmers convert their farming systems from conventional to organic, new or advanced procedures are required to maintain crop production. Notably, they are forced to employ a non-herbicide management system, which can be a laborious operation (Bàrberi Citation2002).
In Japan, various means such as manual labor, machines, and ducks have been used for weed control in organic paddy fields. The application of rice bran is one of the most accepted methods among these because it is relatively labor-saving and less expensive than others. The rice bran is simply spread on the soil surface at transplanting or very soon afterward. However, its effectiveness varies greatly depending on year and location. Although a certain number of Japanese farmers practice this way, reports on this type of operation are limited. Nakai and Toritsuka (Citation2009), Miura et al. (Citation2015), and Uno et al. (Citation2021) conducted field experiments with the application of 1.5, 1.0 and 1.2 t ha−1 of rice bran, respectively. In these experiments, the application was completed at transplanting and/or before 10 days after transplantation (DAT). All of their trials reduced the number of weeds significantly.
Huge amounts of less crystalline or amorphous ferric oxides are present in soil. These substances are insoluble and extractable with chelating agents (Nozoe, Yoshida, and Yasuda Citation1994). Iron(III)-reducing bacteria use these ferric iron as the terminal electron acceptor for the oxidation of organic compounds to other oxidized metabolites, and they conserve energy for growth (Lu et al. Citation2002). Accordingly, the application of rice bran to soil increases soluble Fe2+ as well as organic acids (). In the conventional rice production, Fe2+ and organic acids also sometimes retard the growth of rice (Tanaka, Ono, and Hayasaka Citation1990) as well as Echinochloa weeds (Nozoe et al. Citation2010). Generally, the oxidation-reduction status (Eh) of the soil surface tends to be higher than that of whole topsoil (Hasebe, Sekiya, and Iimura Citation1985; Mikkelsen and Finfrock Citation1957). The Eh of the flooded soil at 0–1 cm depth (−50 mV to – 150 mV) is higher than that beneath (< – 150 mV) (Schmidt, Eickhorst, and Tippkötter Citation2011). Based on these reports, the condition of the soil surface is mildly anaerobic throughout flooding, but deeper soil becomes strictly anaerobic. Takai and Kamura (Citation1966) found that both Fe2+ and organic acids are produced under facultative anaerobic conditions. In contrast, the disintegration of organic acids requires strictly anaerobic microbes, including methane-producing bacteria (Shan et al. Citation2008) (). For instance, acetic (Nozoe and Yasuda Citation1995) and propionic (Nozoe Citation1997) acids decompose under methanogenesis. With decrease in Eh, sulfate (SO42–)-reducing bacteria boosts sulfur (S2–) content with accepting an electron from organic compounds (He et al. Citation2010). Consequently, the concentration of Fe2+ in soil solution decreases under strictly anaerobic conditions because it turns to precipitates of iron sulfide (FeS) (Li and Horikawa Citation1998). These reports suggest that the amount of the toxic substances at the soil surface might be greater than those in the soil below.
Figure 1. Changes in substances in flooded soil with the application of organic material during the proceeding of soil reduction.
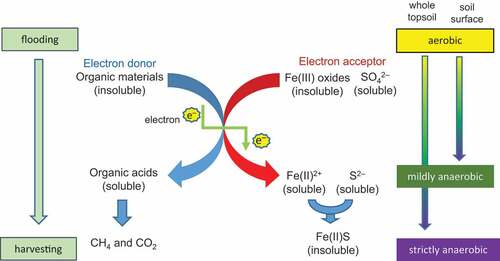
Although herbicides, such as butachlor, easily kill the heart-shaped false pickerelweed (Monochoria vaginalis) (Burm. f.) Kunth (De Datta and Bernasor Citation1973), its growth in organic paddy field is a very serious problem (). Farmers are practically aware that the numbers of this weed change depending on the field. Experienced agronomists have noted that its germination is stimulated specifically under paddy conditions. In practice, more than 90% of M. vaginalis seedlings emerge from puddled paddy soil within 2 mm depth (Koarai and Shibayama Citation2001). Presumably, this plant overwhelms other weeds because of this uniqueness. The pole surface of the paddy soil, where its seed can germinate, has a unique rhizosphere. This soil part is located at the border of aerobic flooded water and anaerobic paddy soil. M. vaginalis may be the weed that is best adapted to this circumstance.
Figure 2. Monochoria vaginalis on field (left) and its overview (right).
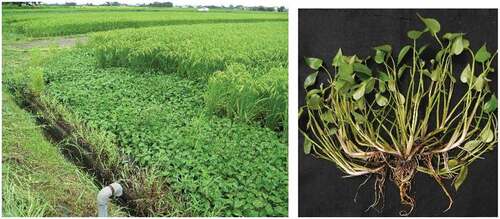
If rice bran is applied to paddy soil, the rhizosphere consists of opposite momentums that promote and suppress the germination of M. vaginalis. The former originates from the soil and the latter from rice bran. This promotive power might destabilize the efficacy of rice bran. This review focuses on the elucidation of these mechanisms.
2. Effects of rice bran on germination
2.1. Suppression by fresh rice bran
Nozoe et al. (Citation2018) conducted an experiment regarding the germination of M. vaginalis in soil with rice bran. Soil sample without and with 0.3%, 0.6%, and 0.9% rice bran was flooded with distilled water and incubated at 30°C for 3 days. On the assumption that the bulk density of paddy soil is 1.00 Mg m−3 (g cm−3) (Terasawa Citation1975), the weight of soil 1 cm in depth was estimated to be 100 t ha−1 (100 m × 100 m × 1 cm = 108 cm3 ≅ 108 g = 100 t). These amounts in the experiment correspond to the application of approximately 0.3, 0.6 and 0.9 ton per 1 ha × 1 cm field. After the soil solution was recovered, seed of M. vaginalis was incubated in the solution at 30°C for 3 days, followed by analysis of germination. The rate decreased with increasing rice bran content indicating that the addition of rice bran suppressed the germination ().
Figure 3. Differences in the germination of Monochoria vaginalis in soil solution with and without the addition of fresh rice bran.
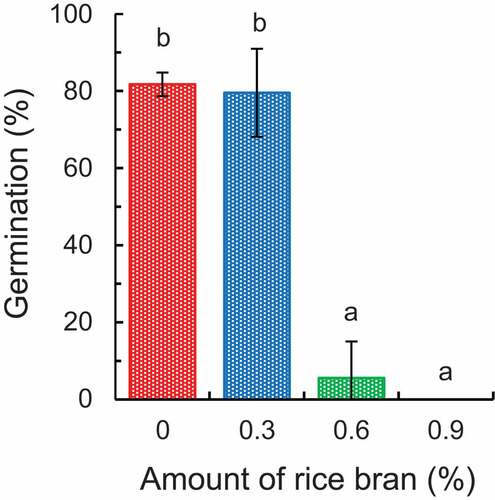
2.2. Promotion by scarcely decomposable component
Miura et al. (Citation2015) demonstrated that a combination of mechanical weeding and the application of 1 t ha−1 of rice bran into soil significantly reduces the number of M. vaginalis plants. As indicated above, the weight of soil 1 cm in depth is estimated to be approximately 100 t ha−1. Therefore, the weight of topsoil 10 cm in depth is estimated to be 1,000 t ha−1. When 1 t of rice bran is applied to 1 ha and incorporated with 10 cm of topsoil after harvesting, the ratio of rice bran corresponds to 0.1%. Therefore, if the application of rice bran continues for 10 years, the total amount of rice bran is 1%. Nozoe et al. (Citation2021a) conducted a laboratory experiment to prepare soil samples with scarcely decomposable organic component. After rice bran was mixed with air-dried paddy soil (rice bran/soil [w/w] was 0, 0.4%, 0.8% and 1.2%), the mixture was flooded with distilled water and incubated at 30°C for 3 days. Most of the easily decomposable components were speculated to be removed during incubation because of anaerobic decomposition. The mixture was air-dried and then distilled water was added, followed by shaking and filtration. This air-drying could accelerate the disintegration of the remained components because this procedure stimulated the solubilization of organic matter and the microbiological activity (Bartlett and James Citation1980). After incubation of the seed in the filtrate at 30°C for 4 days, the germination was analyzed. There was a positive and significant correlation between the amount of rice bran and germination, indicating that the scarcely decomposable organic components enhanced germination ().
Figure 4. Effects of the scarcely decomposable components of rice bran on the germination of Monochoria vaginalis.
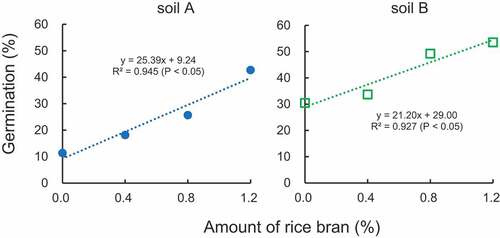
The easily and scarcely decomposable components of rice bran had conflicting powers. When the flooded soil with fresh rice bran was incubated, the toxic substances from the easily decomposable component suppressed the germination (). However, further waterlogging and subsequent drying remove this component due to the microbial disintegration, and these procedures leave the scarcely decomposable unit. The incubation of seed in the soil solution with this residue enhanced the germination (). This phenomenon could be imputed to the stimulative power of the scarcely decomposable component. As will be discussed below, each soil is also equipped with this power. Therefore, the germination rate fluctuates characteristically according to soils. The long-term application of rice bran may make weed control even more difficult and complex because of the stimulative power of scarcely decomposable component.
3. Factors affecting the germination
3.1. Difference in the effectiveness of rice bran among soils
Under laboratory conditions, Nozoe et al. (Citation2021a) mixed eight soils with 0% (control), 0.15%, 0.3%, and 0.45% rice bran, then flooded them with distilled water. After incubation at 30°C for 7 days, the soil solution was recovered. The seed of M. vaginalis was incubated in the solution at 30°C for 2 days. Although the germination percentages in all solutions decreased linearly with increasing amounts of rice bran, its efficacy notably fluctuated according to the soil (). This phenomenon supported the field observation that the efficacy of this material is not stable over paddy fields, even when the same amount is applied. Without the addition of rice bran (0%), the germination percentage in soil A was larger than that in soil E. In the presence of 0.45% rice bran, however, the relationship was reversed. When the rice bran is applied, therefore, 1) the gemination in the absence of bran (y-intercept) and 2) the efficacy of this (slope) are the important factors in determining the amount of rice bran for application.
Figure 5. Effects of the addition of fresh rice bran on the decrease in germination of Monochoria vaginalis.
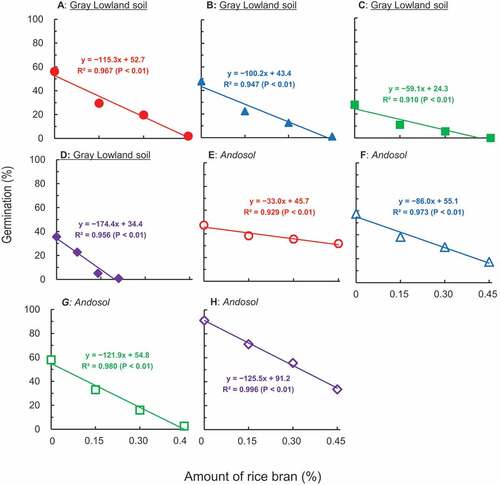
In this experiment, the germination rate decreased linearly as the amount of rice bran increased (), but the straightness was not observed in . In both experiments, soil solution was obtained after the incubation of flooded soil with rice bran. Seeds were then sank to the solution, followed by incubation. During the latter incubation, the Fe2+ content in the test tube decreased. This decline was due to the precipitation of Fe(III) oxides by the oxygen (O2) in the air. This oxidation of solution is presumably responsible for the inconsistency with the linearity. To obtain linear correlation, the incubation period of test tube was reduced from 3 days () to 2 days (). In addition, in , seeds that germinated quickly was used. While most of the seeds germinated within 24 hours in , those in took 3 days.
If a copious volume of this material were applied to a field, this weed would be terminated. Nevertheless, an excessive quantity might have negative effects such as retardation of rice growth. The policy of weeding depends on the farmer. If rice bran reduced this plant not completely but reasonably, farmers could benefit economically. Obtaining field-specific information about these two related factors will be useful for farmers.
3.2. Organic acids
Tanaka, Tazawa, and Uchino (Citation2020) determined several organic acids in paddy soil with rice bran. Based on this information, Tazawa et al. (Citation2021) evaluated the toxicities of some organic acids to the germination of M. vaginalis. For instance, although neither 0.5 mM iso-butyric acid nor 0.05 mM 3-phenylpropionic acid individually affect the germination of this weed, their mixture suppressed germination. These findings imply that combinations of organic acids are the basis of M. vaginalis suppression by rice bran application. While Fe2+ suppressed the germination of most paddy weeds, M. vaginalis show relatively strong tolerance to Fe2+ toxicity among these plants (Nozoe et al. Citation2009). Tazawa et al. (Citation2021) indicate the necessity of investigating the synergic interaction between Fe2+ and organic acids.
3.3. Oxygen
Momonoki (Citation1992), and Kataoka and Kim (Citation1978) analyzed the germination of M. vaginalis in a sealed tube whose inner air was replaced with a hypoxic gas. They confirmed that one of the most unique characteristics of M. vaginalis is its requirement of very little O2 for germination. Nozoe et al. (Citation2018) conducted a laboratory experiment to investigate the relationship between O2 and germination using five soil samples. A filtrate was obtained immediately after mixing air-dried soil and distilled water. Subsequently, the seed was incubated at 30°C for 4 days in the filtrate. In addition, the germination was analyzed in sodium ascorbate solution that reduced the O2 content for 4 days. Low dissolved oxygen (DO) in both the filtrate and the ascorbate solution enhanced germination significantly, indicating that low O2 content enhanced germination (). The Eh of those solutions remained high (> +300 mV) throughout the incubation. It is generally accepted that O2 content in paddy soil decreases with a decrease in Eh (Tanji et al. Citation2003), and that the reduction of O2 is due to the respiration of microorganisms (Takai and Kamura Citation1966). Based on our findings, however, anaerobic status is not required for the enhancement of germination.
Figure 6. Relationship between germination of Monochoria vaginalis and integrated DO.
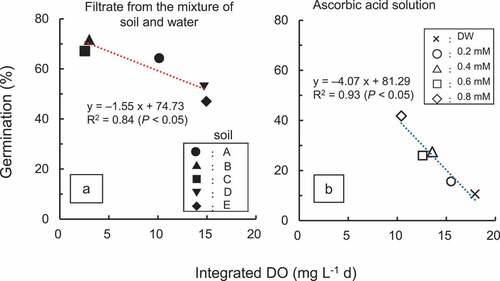
When rice bran is applied to the soil surface, its easily decomposable components disintegrate to intermediate products such as organic acids, and these are further broken down to methane (CH4) and carbon dioxide (CO2) (). In contrast, a scarcely decomposable part, which contains several oxidation inhibitors, remains in the soil. This component is incorporated into the whole topsoil after rice harvesting. For instance, oryzanols, tocopherols, and tocotrienols have strong antioxidant activities (Lloyd, Siebenmorgen, and Beers Citation2000). Ferulate esters are the main components of oryzanols (Xu and Godber Citation1999), and these have reducing powers (Cheng and Li Citation2004). According to Harukaze, Murata, and Homma (Citation1999), the average ferulate content in rice bran of 21 Japanese cultivars is 1.55 g kg−1, and there are differences in content among varieties ranging from 0.22 to 2.25 g kg−1 (ferulic acid basis). The total content of bound phenolics, including ferulics, is 12.2 g kg−1. Zengin et al. (Citation2014) showed that most of these phenolics have O2-reducing activity. These components would be assimilated with the soil over a long period of time. Eventually, those could become one of the factors that enhance the germination.
3.4. Soil pH
Soil pH is one of the most important factors affecting organic acids and Fe2+ toxicity. The pH of soil solution affects the dissociation of the organic acids significantly because these substances are weak acids (Yoshida Citation1981). While a large fraction of organic acids is present in undissociated forms such as acetic acid (CH3COOH) at high pH levels, dissociated forms of acid such as CH3COO – prevail at low pH levels. The noxious symptoms in rice caused by organic acids are aggravated with decreasing pH because the toxicity of dissociated forms is greater than that of undissociated ones (Tanaka and Navasero Citation1967). Additionally, Fe2+ toxicity is intensified under acidic conditions due to the high solubility of Fe2+ at low pH (Nozoe et al. Citation2008). These facts suggest that low pH is also preferable for the control of M. vaginalis by rice bran. Nevertheless, low pH is not always beneficial for rice cultivation. First, it stimulates the germination of M. vaginalis if the pH is between 4 and 7 (Nozoe et al. Citation2018; Tazawa et al. Citation2021). Second, the optimum pH for rice growth is around 5 to 6 (Alam Citation1981; Fageria and Baligar Citation1999), indicating that pH < 5 could retard rice growth. Finally, low pH could accelerate aluminum (Tanaka and Navasero Citation1967) as well as Fe2+ toxicity. Since the pH of most Japanese soil is acidic (Nishida, Sekiya, and Yoshida Citation2013), there is a risk that low pH could unsteady the efficacy of rice bran. The application rate of this material should be determined based on these factors. Kawaguchi et al. (Citation1997) reported that pH barely affected the germination of M. vaginalis in the range from 7 to 9. However, the growth tendency of this weed under alkaline conditions is still unclear.
3.5. Other factors
Rice seeds, hull shoots, and straw contain allelochemicals that enhance the germination of M. vaginalis (Takeuchi, Kawaguchi, and Yoneyama Citation2001). Xuan et al. (Citation2003) suggested that allelopathic materials from rice bran promote the germination of M. vaginalis. Although light exposure is required for the germination of M. vaginalis, the allelochemicals from rice seed compensate for the lack of light (Kawaguchi et al. Citation1997). This substance is water soluble, but has not yet been identified. Yokota et al. (Citation2014) reported that rice hull cooperates with microorganisms to stimulate the germination of M. vaginalis. They speculated that bacteria digest the surface of the seed coat, leading to the enhancement of germination. However, this speculation has not been proven to date.
Uno et al. (Citation2021) reported that the application of rice bran stimulates the turbidity of flooded water. Although numerous factors might be related to turbidity, its mechanism has not been fully investigated. The lack of clarity could be caused by the accumulation of fine-grained and smooth-textured particles on the soil surface. Certain animals as well as water movement may agitate a certain part of the soil surface constantly. Consequently, the lightest particles could keep floating in flooded water. The increase in turbidity could retard germination because it prevents light from reaching the seed.
4. Indicators for the germination suppressor
4.1. EC of soil solution
The addition of rice bran boosts the EC of soil solution (Nozoe et al. Citation2012). This rise results from the increment of various ionic components derived from the disintegration of rice bran. In this context, organic acids and Fe2+ are the influential EC enhancers. EC is a useful indicator to evaluate the efficacy of rice bran because there is a negative and significant correlation between the germination of M. vaginalis and EC. The EC of soil solution is also useful for evaluating the emergence of M. vaginalis seedlings in the presence of poultry manure (Watanabe, Hoshino, and Adachi Citation2017). Utami et al. (Citation2020) analyzed the effects of application of winter grasses to paddy fields on summer weed germination. They confirmed a significant and negative correlation between the EC and the amount of Alopecurus aequalis (foxtail) and that of Astragalus sinicus (milk vetch).
In a soil solution obtained from Gray Lowland soils, none of the M. vaginalis seeds germinated when EC was over 130 mS m−1 (Nozoe et al. Citation2021a). In contrast, seeds in some Andosol solutions germinated even when the EC was above this critical level. These findings indicate that solution from Andosols might have a different mechanism of suppression compared with that of the Gray Lowland soils. Takijima (Citation1964) reported that humus absorbs organic acids and speculated that the high content of humus in paddy soil reduces the toxicity of organic acids to rice. The high tolerance of germination of M. vaginalis in Andosol solutions might be associated with the high content of humus in Andosols.
4.2. Settled soil volume in water
In paddy soil, tubificids ingest mud with their head down and tail up (Kikuchi and Kurihara Citation1977). After absorption and digestion of the nutrients in the mud, the earthworm ejects the soil particles onto the surface layer. During this procedure, soils with fine particles are selectively accumulated on the topsoil surface because large particles cannot pass through its gut. The texture of the newly produced layer is smoother than that of whole topsoil. Presumably, other factors than particle size are also attributable to this difference in texture. The accumulation of a fine-grained and smooth-textured layer compels the seeds to be placed deeper, making it difficult for them to receive light. Although insufficient light exposure is one of the factors that slow the germination of M. vaginalis, its mechanisms have not been fully elucidated.
The sediment volume (SV), which corresponds to the soil volume in water per dry matter weight, is sometimes used for the evaluation of soil microstructure in the paddy-upland rotation soils (Takahashi et al. Citation1999). The soil with high SV can be pulverized easily into small clods, and it improves workability. As one of the physical factors, Nozoe et al. (Citation2016) analyzed the settled soil volume in water (SSVW) of the newly produced layer over the topsoil. The SSVW was measured using the modified method of the SV. Soil was sampled from the superficial layer of the topsoil (<10 mm), which was of a finer texture than the rest of the topsoil. A volume of moist soil corresponding to 10 g of oven-dried soil was placed in a measuring cylinder, which was filled with 50 mL distilled water. The mixture was shaken thoroughly and allowed to stand for 18–24 h. The soil volume in the cylinder, divided by the soil weight on an oven-dried basis, was expressed as SSVW. There was a negative correlation between SSVW and the number of individuals of M. vaginalis. This finding suggests that SSVW is useful as a physical indicator for the growth suppression of M. vaginalis. The application of rice bran raised the SSVW and reduced the number of M. vaginalis plants dramatically.
4.3. Changes in chemical and physical factors in organic farming fields
Nozoe et al. (Citation2021b) summarized the relationship between the change in EC and that in SSVW based on a field trial. They spread 1 t ha−1 of rice bran on fields, followed by the analysis of both the EC of the soil solution and the SSVW of the soil surface. The application of rice bran reduced the number of M. vaginalis plants. Although this operation enhanced both EC and SSVW during the 29-day period after transplanting, the tendency of their changes was contrasting (). The difference in EC with and without the application of this material was maximal at 2 DAT, followed by a decrease until 19 DAT. These findings suggest that the chemical factor was effective during the first half of month after transplanting. In contrast, the difference in SSVW increased from 8 to 29 DAT, indicating that the physical factor was effective during the latter half of the month after transplanting. Thus, these factors worked for the suppression of M. vaginalis in a complementary manner.
Figure 7. Changes in EC and SSVW with and without the application of rice bran.
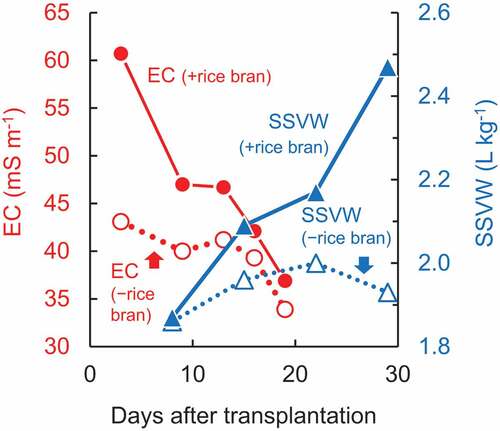
5. Conclusion
Rice bran has conflicting effects on the growth of M. vaginalis: the easily and scarcely decomposable components suppresses and enhances the germination, respectively. When fresh rice bran is applied to the field, the increase in toxic substances such as organic acids suppresses the germination of M. vaginalis. Anaerobic status is necessary to increase these toxins. Accumulation of these substances is a chemical factor that suppresses germination. In addition, rice bran application stimulates the piling of small particles on the soil surface. This layer acts as a physical factor to retard germination because its fine grains prevent light from reaching the seed. The EC of soil solution and the SSVW of the soil surface are useful indicators to evaluate the chemical and physical factors that control germination, respectively. Based on the changes in these indicators in the field, the chemical and physical factors were effective during the former and latter halves of month after transplanting, respectively. Basically, these factors worked in a complementary manner. Paddy soil is typically equipped with the ability to reduce O2. This trait enhances the number of M. vaginalis because hypoxic conditions are preferable for germination. Presumably, the combination of this peculiarity of M. vaginalis and the O2-reducing power of soil accounts for the dominance of this weed in organic fields. Anaerobic conditions are not required for the expression of O2-reducing power. A long-term application of rice bran might intensify this power because the scarcely decomposable component of rice bran contains a significant amount of O2-reducing substances.
In general, the most serious drawback to using rice bran is that the efficacy of this material varies depending on the year of cultivation and the field. The presence of contradictory powers that suppress or enhance germination make the efficacy of rice bran unstable. Although the results presented here would contribute the improvement of organic rice production, the reasons that M. vaginalis could survive in paddy fields, especially under organic farming systems, remain unclear. For instance, the role of microorganisms in germination is still unknown. Cross-disciplinary research related to soil and weed sciences and agronomy is needed to understand the complexity of the problem.
Disclosure statement
No potential conflict of interest was reported by the author(s).
References
- Alam, S. M. 1981. “Effects of Solution pH on the Growth and Chemical Composition of Rice Plants.” Journal of Plant Nutrition 4 (3): 247–260. doi:10.1080/01904168109362916.
- Bàrberi, P. 2002. “Weed Management in Organic Agriculture: Are We Addressing the Right Issues?” Weed Research 42 (3): 177–193. doi:10.1046/j.1365-3180.2002.00277.x.
- Bartlett, R., and B. James. 1980. “Studying Dried, Stored Soil Samples—some Pitfalls.” Soil Science Society of America Journal 44 (4): 721–724. doi:10.2136/sssaj1980.03615995004400040011x.
- Cheng, Z., and Y. Li. 2004. “Reducing Power: The Measure of Antioxidant Activities of Reductant Compounds?” Redox Report 9 (4): 213–217. doi:10.1179/135100004225005994.
- De Datta, S. K., and P. C. Bernasor. 1973. “Chemical Weed Control in Broadcast-seeded Flooded Tropical Rice.” Weed Research 13 (4): 351–354. doi:10.1111/j.1365-3180.1973.tb01285.x.
- Fageria, N. K., and V. C. Baligar. 1999. “Growth and Nutrient Concentrations of Common Bean, Lowland Rice, Corn, Soybean, and Wheat at Different Soil pH on an Inceptisol.” Journal of Plant Nutrition 22 (9): 1495–1507. doi:10.1080/01904169909365730.
- Harukaze, A., M. Murata, and S. Homma. 1999. “Analyses of Free and Bound Phenolics in Rice.” Food Science and Technology Research 5 (1): 74–79. doi:10.3136/fstr.5.74.
- Hasebe, A., S. Sekiya, and K. Iimura. 1985. “Direct Determination of the Differentiation Process of the Oxidized and Reduced Soil Layers in Paddy Fields.” Japanese Agricultural Research Quarterly: JARQ 19 (3): 172–177. Accecced 11 February 2022.https://www.jircas.go.jp/ja/publication/jarq/19/3/172.
- He, J. Z., X. Z. Liu, Y. Zheng, J. P. Shen, and L. M. Zhang. 2010. “Dynamics of Sulfate Reduction and Sulfate-reducing Prokaryotes in Anaerobic Paddy Soil Amended with Rice Straw.” Biology and Fertility of Soils 46 (3): 283–291. doi:10.1007/s00374-009-0426-3.
- Kataoka, T., and S. Y. Kim. 1978. “Oxygen Requirement for Seed Germination of Several Weeds.” Weed Research, Japan 23 (1): 9–12. doi:10.3719/weed.23.9. in Japanese with English summary.
- Kawaguchi, S., K. Yoneyama, T. Yokota, Y. Takeuchi, M. Ogasawara, and M. Konnai. 1997. “Effects of Aqueous Extract of Rice Plants (Oryza Sativa L.) On Seed Germination and Radicle Elongation of Monochoria Vaginalis Var. Plantaginea.” Plant Growth Regulation 23 (3): 183–189. doi:10.1023/A:1005986126035.
- Kikuchi, E., and Y. Kurihara. 1977. “In Vitro Studies on the Effects of Tubificids on the Biological, Chemical and Physical Characteristics of Submerged Ricefield Soil and Overlying Water.” Oikos 29 (2): 348–356. doi:10.2307/3543626.
- Koarai, A., and H. Shibayama. 2001. “Effects of Puddling Time on Seasonal Changes in Number and Depth of Emergence of Annual Weeds in Paddy Fields.” Journal of Weed Science and Technology (Japan) 46 (1): 5–12. doi:10.3719/weed.46.5. in Japanese with English summary.
- Li, Z., and Y. Horikawa. 1998. “Stability Behavior of Soil Colloidal Suspensions in Relation to Sequential Reduction of Soils.” Soil Science and Plant Nutrition 44 (2): 227–235. doi:10.1080/00380768.1998.10414443.
- Lloyd, B. J., T. J. Siebenmorgen, and K. W. Beers. 2000. “Effects of Commercial Processing on Antioxidants in Rice Bran.” Cereal Chemistry 77 (5): 551–555. doi:10.1094/CCHEM.2000.77.5.551.
- Lu, W. J., H. T. Wang, C. Y. Huang, and W. Reichardt. 2002. “Communities of Iron (Iii)-reducing Bacteria in Irrigated Tropical Rice Fields.” Microbes and Environments 17 (4): 170–178. doi:10.1264/jsme2.17.170.
- Mikkelsen, D. S., and D. C. Finfrock. 1957. “Availability of Ammoniacal Nitrogen to Lowland Rice as Influenced by Fertilizer Placement 1.” Agronomy Journal 49 (6): 296–300. doi:10.2134/agronj1957.00021962004900060005x.
- Miura, S., A. Uchino, T. Nozoe, J. Tazawa, T. Yoshida, T. Mizukami, B. H. Jeong, et al. 2015. “Weed Suppression and Rice Production by Mechanical Weeding and Rice Bran Application Work in Organic Rice Cultivation System.” Bullerin of the NARO Agricultural Research Cener 24: 55–69. 10.24514/00001591.in Japanese with English summary.
- Momonoki, Y. 1992. “Effect of Ethylene and Carbon Dioxide on Seed Germination of Monochoria Vaginalis Var. Plantaginea.” Weed Research, Japan 37 (2): 121–128. doi:10.3719/weed.37.121.
- Nakai, J., and S. Toritsuka. 2009. “Inhibitive Effect of Rice Bran Treatment of Soil Surface on Paddy-field Weeds.” Journal of Weed Science and Technology 54 (4): 233–238. in Japanese with English summary. doi:10.3719/weed.54.233.
- Nishida, M., H. Sekiya, and K. Yoshida. 2013. “Status of Paddy Soils as Affected by Paddy Rice and Upland Soybean Rotation in Northeast Japan, with Special Reference to Nitrogen Fertility.” Soil Science and Plant Nutrition 59 (2): 208–217. doi:10.1080/00380768.2012.762588.
- Nozoe, T. 1997. “Effects of Methanogenesis and Sulfate-reduction on Acetogenetic Oxidation of Propionate and Further Decomposition of Acetate in Paddy Soil.” Soil Science and Plant Nutrition 43 (1): 1–10. doi:10.1080/00380768.1997.10414709.
- Nozoe, T., R. Agbisit, Y. Fukuta, R. Rodriguez, and S. Yanagihara. 2008. “Characteristics of Iron Tolerant Rice Lines Developed at IRRI under Field Conditions.” Japan Agricultural Research Quarterly: JARQ 42 (3): 187–192. doi:10.6090/jarq.42.187.
- Nozoe, T., D. Aoki, H. Matsuoka, K. Matsushima, S. Miura, A. Uchino, and X.-C. Wan. 2016. “Relationship between Physical Property of Soil and Growth of Monochoria Vaginalis under Paddy Condition of Organic Farming—analysis Using Settled Soil Volume in Water of Superficial Layer.” Plant Production Science 19 (2): 238–245. doi:10.1080/1343943X.2015.1128105.
- Nozoe, T., S. Miura, J. Tazawa, A. Uchino, and Y. Usui. 2021a. “Factors Destabilizing the Control of Monochoria Vaginalis by Rice Bran: Its Conflicting Powers Influence Both Suppression and Promotion of Germination in Paddy Soil.” Plant Production Science 24 (1): 83–93. doi:10.1080/1343943X.2020.1794916.
- Nozoe, T., T. Shinano, M. Tachibana, and A. Uchino. 2010. “Tolerance of Rice (Oryza Sativa L.) And Echinochloa Weeds to Growth Suppression by Rice Straw Added to Paddy Soil in Relation to Iron Toxicity.” Plant Production Science 13 (3): 314–318. doi:10.1626/pps.13.314.
- Nozoe, T., M. Tachibana, A. Uchino, and N. Yokogami. 2009. “Effects of Ferrous Iron (Fe) on the Germination and Root Elongation of Paddy Rice and Weeds.” Weed Biology and Management 9 (1): 20–26. doi:10.1111/j.1445-6664.2008.00314.x.
- Nozoe, T., J. Tazawa, A. Uchino, and S. Miura. 2018. “Promotive Effect of Soil Solution on Germination of Monochoria Vaginalis under Paddy Conditions.” Soil Science and Plant Nutrition 64 (3): 396–405. doi:10.1080/00380768.2018.1433957.
- Nozoe, T., J. Tazawa, A. Uchino, and S. Miura. 2021b. “Changes in Physical and Chemical Properties of Soil with the Application of Rice Bran in Relation to the Growth Suppression of Monochoria Vaginalis.” Abstracts of the 251st Meeting of the Crop Science Society of Japan (CSSJ) Kyoto, Japan, p. 129. doi:10.14829/jcsproc.251.0_129.
- Nozoe, T., A. Uchino, S. Okawa, S. Yoshida, Y. Kanda, and Y. Nakayama. 2012. “Suppressive Effect of Rice Bran Incorporation in Paddy Soil on Germination of Monochoria Vaginalis and Its Relationship with Electric Conductivity.” Soil Science and Plant Nutrition 58 (2): 200–205. doi:10.1080/00380768.2012.670864.
- Nozoe, T., and M. Yasuda. 1995. “Mechanisms of Decomposition of Acetic Acid by Methane-producing Bacteria in Submerged Paddy Soil.” Soil Science and Plant Nutrition 41 (1): 151–155. doi:10.1080/00380768.1995.10419569.
- Nozoe, T., K. Yoshida, and M. Yasuda. 1994. “Effect of EDTA on Reduction Process in Submerged Paddy Soil with Rice Straw.” Soil Science and Plant Nutrition 40 (1): 155–164. doi:10.1080/00380768.1994.10414288.
- Reganold, J. P., and J. M. Wachter. 2016. “Organic Agriculture in the Twenty-first Century.” Nature Plants 2 (2): 1–8. doi:10.1038/nplants.2015.221.
- Schmidt, H., T. Eickhorst, and R. Tippkötter. 2011. “Monitoring of Root Growth and Redox Conditions in Paddy Soil Rhizotrons by Redox Electrodes and Image Analysis.” Plant and Soil 341 (1): 221–232. doi:10.1007/s11104-010-0637-2.
- Shan, Y., Z. Cai, Y. Han, S. E. Johnson, and R. J. Buresh. 2008. “Organic Acid Accumulation under Flooded Soil Conditions in Relation to the Incorporation of Wheat and Rice Straws with Different C: N Ratios.” Soil Science and Plant Nutrition 54 (1): 46–56. doi:10.1111/j.1747-0765.2007.00218.x.
- Takahashi, T., C. Y. Park, H. Nakajima, H. Sekiya, and K. Toriyama. 1999. “Ferric Iron Transformation in Soils with Rotation of Irrigated Rice-upland Crops and Effect on Soil Tillage Properties.” Soil Science and Plant Nutrition 45 (1): 163–173. doi:10.1080/00380768.1999.10409332.
- Takai, Y., and T. Kamura. 1966. “The Mechanism of Reduction in Waterlogged Paddy Soil.” Folia Microbiologica 11 (4): 304–313. doi:10.1007/BF02878902.
- Takeuchi, Y., S. Kawaguchi, and K. Yoneyama. 2001. “Inhibitory and Promotive Allelopathy in Rice (Oryza Sativa L.).” Weed Biology and Management 1 (3): 147–156. doi:10.1046/j.1445-6664.2001.00031.x.
- Takijima, Y. 1964. “Studies on Organic Acids in Paddy Field Soils with Reference to Their Inhibitory Effects on the Growth of Rice Plants: Part 1. Growth Inhibiting Action of Organic Acids and Absorption and Decomposition of Them by Soils.” Soil Science and Plant Nutrition 10 (5): 14–21. doi:10.1080/00380768.1964.10431135.
- Tanaka, A., and S. A. Navasero. 1967. “Carbon Dioxide and Organic Acids in Relation to the Growth of Rice.” Soil Science and Plant Nutrition 13 (1): 25–30. doi:10.1080/00380768.1967.10431969.
- Tanaka, F., S. L. Ono, and T. Hayasaka. 1990. “Identification and Evaluation of Toxicity of Rice Root Elongation Inhibitors in Flooded Soils with Added Wheat Straw.” Soil Science and Plant Nutrition 36 (1): 97–103. doi:10.1080/00380768.1990.10415714.
- Tanaka, F., J. Tazawa, and A. Uchino. 2020. “’Komenuka No Tansuidojo Bunkaibutsu Tyu No Kihatsusei Seibun No Bunseki’ [Analysis of Volatile Substances in Flooded Soil with Rice Bran] Annual meetings Japanese Society of Soil Science and Plant Nutrition Okayama, Japan.” 66: 85. in Japanese. doi:10.20710/dohikouen.66.0_85_1.
- Tanji, K. K., S. Gao, S. C. Scardaci, and A. T. Chow. 2003. “Characterizing Redox Status of Paddy Soils with Incorporated Rice Straw.” Geoderma 114 (3–4): 333–353. doi:10.1016/S0016-7061(03)00048-X.
- Tazawa, J., D. Aoki, H. Hayakawa, K. I. Matsushima, T. Nozoe, A. Uchino, and S. Miura. 2021. “Suppressive Activity of Volatile Fatty Acids and Aromatic Carboxylic Acids on the Germination of Monochoria Vaginalis.” Plant Production Science 24 (4): 505–511. doi:10.1080/1343943X.2021.1896953.
- Terasawa, S. 1975. “Physical Properties of Paddy Soil in Japan.” Japan Agricultural Research Quarterly: JARQ 9 (1): 18–23. Accessed 11 February 2022. https://www.jircas.go.jp/ja/publication/jarq/9/1/18.
- Uno, T., R. Tajima, K. Suzuki, M. Nishida, T. Ito, and M. Saito. 2021. “Rice Yields and the Effect of Weed Management in an Organic Production System with Winter Flooding.” Plant Production Science 24 (4): 405–417. doi:10.1080/1343943X.2020.1865823.
- Utami, A. I., P. O. Bimantara, R. Umemoto, R. K. Sabri, V. Kautsar, K. Tawaraya, E. Hanudin, and W. Cheng. 2020. “Incorporation of Winter Grasses Suppresses Summer Weed Germination and Affects Inorganic Nitrogen in Flooded Paddy Soil.” Soil Science and Plant Nutrition 66 (2): 389–397. doi:10.1080/00380768.2020.1725914.
- Watanabe, H., K. Hoshino, and Y. Adachi. 2017. “Effects of Poultry Manure on Soil Solution Electrical Conductivity and Early Growth of Monochoria Vaginalis.” Plant Production Science 20 (1): 67–71. doi:10.1080/1343943X.2016.1246064.
- Xu, Z., and J. S. Godber. 1999. “Purification and Identification of Components of γ-oryzanol in Rice Bran Oil.” Journal of Agricultural and Food Chemistry 47 (7): 2724–2728. doi:10.1021/jf981175j.
- Xuan, T. D., E. Tsuzuki, H. Terao, M. Matsuo, T. D. Khanh, S. Murayama, and N. H. Hong. 2003. “Alfalfa, Rice By‐products and Their Incorporation for Weed Control in Rice.” Weed Biology and Management 3 (2): 137–144. doi:10.1046/j.1445-6664.2003.00095.x.
- Yokota, T., H. Handa, Y. Yamada, K. Yoneyama, and Y. Takeuchi. 2014. “Mechanism of the Rice Hull‐induced Germination of Monochoria Vaginalis Seeds in Darkness.” Weed Biology and Management 14 (2): 138–144. doi:10.1111/wbm.12042.
- Yoshida, S. 1981. “Organic Acid .“ Fundamentals of Rice Crop Science, 171–174. Manila: International Rice Research Institute.
- Zengin, G., A. Uysal, E. Gunes, and A. Aktumsek. 2014. “Survey of Phytochemical Composition and Biological Effects of Three Extracts from a Wild Plant (Cotoneaster Nummularia Fisch. Et Mey.): A Potential Source for Functional Food Ingredients and Drug Formulations.” PloS One 9 (11): e113527. doi:10.1371/journal.pone.0113527.