ABSTRACT
Organic acid secretion from the roots enables plants to acquire phosphorus (P) which is poorly soluble in soil. We previously reported that when soybeans were cultivated in vermiculite in the presence of insoluble calcium phosphate, as a phosphorus source, sulfur (S) fertilization increased organic acid secretion from the roots and improved P acquisition in soybeans. In the present study, we confirmed that S fertilization increased secretion of organic acids such as citric acid when soybeans were cultivated in Andosols having a strong P fixation capacity. In contrast, concentration of citric acid in soybean roots did not increase by S fertilization. Therefore, the relationship between S nutrition and gene expression of citric acid exporters was investigated to understand the mechanisms of induction of citric acid secretion by S. Further, we verified whether the expression of citric acid transporter genes, GmMATE13 and GmMATE47, is involved in the induction of citric acid secretion from the roots by S fertilization. The expression level of GmMATE13 in roots was significantly increased by S fertilization compared to that without S fertilization. Therefore, our results suggest that S nutrition is involved in inducing GmMATE13 expression and contributes to the excretion of citric acid from the soybean roots.
1. Introduction
In general, organic acids are secreted from plant roots as a response to aluminum (Al) stress, phosphorus (P) deficiency, and iron deficiency (Wu et al. Citation2018). The macronutrient P easily binds to metal ions and is poorly soluble in soil; however, organic acids play an important role in P acquisition by plants. Various organic acids, including malic acid: CH2CH(OH)(COOH)2; oxalic acid: (COOH)2; malonic acid: CH2(COOH)2; and citric acid: CH2C(OH) CH2(COOH)3 solubilize inorganic P. Based on their efficiency in P solubilization, organic acids can be ordered as follows: tricarboxylic acid > dicarboxylic acid > monocarboxylic acid (Bolan et al. Citation1994). Among the organic acids secreted from plant roots, citric acid is often detected at very high levels and effectively solubilizes inorganic P as a tricarboxylic acid (Johnson and Loeppert Citation2006; Kpomblekou-A and Tabatabai Citation2003). The organic acids secreted from plant roots differ depending on the plant species. For example, soybean (Glycine max) is known to secrete malonic acid, malic acid, citric acid, succinic acid, and tartaric acid, among others (Stumpf and Burris Citation1981; Ohwaki and Hirata Citation1992).
Transporters of the multidrug and toxic compound extrusion (MATE) family in plants excrete citric acid as a response to aluminum stress (Magalhaes Citation2010). Liu et al. (Citation2016) selected 117 types of soybean MATE protein candidates from the soybean genome sequence. Wang et al. (Citation2019) added aluminum to the roots and examined the resulting changes in the expression of genes encoding seven soybean GmMATE proteins (GmMATE13, GmMATE47, GmMATE58, GmMATE74, GmMATE79, GmMATE84, and GmMATE87) that were classified in the same subgroup as known citric acid transporters in other plants, based on phylogenetic analysis. The expression of GmMATE13 and GmMATE47 showed a stronger response to aluminum stress, evidenced by their much higher expression compared to that of the other GmMATEs. In addition, GmMATE13 and GmMATE47 were localized in the cell membrane and highly expressed in the roots. Furthermore, in Arabidopsis thaliana plants overexpressing either GmMATE13 or GmMATE47, the amount of citric acid secreted from the roots was significantly increased in the presence of aluminum compared to that in the wild-type plants in both the presence and absence of aluminum. Therefore, GmMATE13 and GmMATE47 increase citric acid exudation from the roots and aluminum stress tolerance in soybean.
We previously reported that when soybeans were cultivated in the presence of poorly soluble calcium phosphate as a P source in vermiculite, sulfur (S) fertilization increased organic acid secretion from the roots and improved P acquisition in soybeans (Sugiura et al. Citation2021). In this study, we observed that S fertilization increased organic acid secretion, specifically citric acid secretion, without any variations in citric acid concentration in the soybean root tissues, when cultivated in Andosols having strong P fixation capacity. This result suggests the involvement of citric acid transporter expression in the phosphorous acquisition by soybean.
2. Materials and methods
2.1. Plant/soil tests and cultivation conditions
Soybean (G. max L. cv. Enrei) was used as the test organism and the unfertilized Andosol (20–30 cm from the surface layer) from the field of Tokyo University of Agriculture and Technology (35°68ʹN and 139°48ʹE) was used as the test soil. The soil was passed through a 2 cm sieve and dried at 80°C in a dryer for 2 d. A 1:5 ratio of soil to reverse osmosis (RO) water was used to determine soil pH (van Reeuwijk Citation2002). Before soybean cultivation, the following fertilizers were applied to the soil: 76.3 mg kg⁻1 of K (KCl or K₂SO₄), 38.4 mg kg⁻1 of Mg (MgCl₂ or MgSO₄), 100 mg kg⁻1 of N (CH₄N₂O), 0.06 mg kg⁻1 of Fe (FeNaEDTA), 0.15 mg kg⁻1 of Mn (MnCl₂, 4 H₂O), 0.53 mg kg⁻1 of Zn (ZnCl₂), 0.51 mg kg⁻1 of Cu (CuCl₂, 2H₂O), 0.11 mg kg⁻1 of Co (CoCl₂, 6 H₂O), 0.09 mg kg⁻1 of B (H₃BO₃), and 0.20 mg kg⁻1 of Mo (Na₂MoO₄, 2 H₂O) (Wang, Marschner, and Zhang Citation2012). Two fertilization treatments were designed for this experiment: control treatment without S (–S) and a test treatment with S application (+S). KCl and MgCl2 and K2SO₄ and MgSO₄ were applied as the K and Mg sources in the control and test fertilization treatments, respectively (81.9 mg kg⁻1 of S, which is the same amount applied in Wang, Marschner, and Zhang (Citation2012)).
The soybean seeds were sterilized with sodium hypochlorite and sown in a hole-less pot filled with 335 g of soil prepared to a maximum water holding capacity of 60%. Thinning was performed 2 weeks after sowing, leaving one plant per pot. The plants were cultivated for 6 weeks in a cultivation room maintained at 25°C with 16:8 h light (300 μmol− 1 m−2 s−1): dark period.
2.2. Measurement of available sulfur content in soil
To conduct chemical analysis, the soil was passed through a 2 mm sieve and dried at 80°C in a dryer for 2 d. The dried soil and reverse osmosis (RO) water were mixed at a 1:5 ratio, shaken for 2 h, and filtered through a filter paper (ADVANTEC, No. 6). Thereafter, 1 mL of 0.8 N nitric acid was added to 9 mL of the filtrate. The S concentration in the resulting solution was measured using inductively coupled plasma mass spectrometry (Agilent 7800; Agilent Technologies, Santa Clara, CA, USA) as described by Miyatake et al. (Citation2019) using 2 ppb Indium as an internal standard.
2.3. Measurement of available phosphorus content in soil
Available P in dried soils was extracted with 0.001 M sulfuric acid at pH 3.0 (Truog Citation1930). Sulfuric acid (100 mL of 0.002 N) was added to 0.5 g of dried soil; the suspension was shaken for 30 min and filtered through a filter paper (ADVANTEC, No. 6) to obtain an extract. The P content was measured using colorimetric analysis using the molybdenum sulfate method (Murphy and Reley Citation1962).
2.4. Phosphate absorption coefficient of soil
Diammonium phosphate (25 g) was dissolved in 1 L of RO water, and the pH was adjusted to 7.0 using a 1:1 solution of H2O:H₃PO₄. First, the phosphate concentration of this solution was measured using the vanadomolybdate method (Kitson and Mellon Citation1944) and adjusted to contain 2687 mg of P2O₅ in 200 mL of the solution (13,435 ppm P2O₅ solution) by diluting; this sample was used as the 2.5% (w/v) ammonium phosphate solution. Thereafter, ammonium phosphate solution (50 mL of 2.5% (w/v)) was added to 25 g of dried soil, and the mixture was shaken at 120 rpm in room temperature for 24 h. Subsequently, the mixture was filtered through a filter paper (ADVANTEC, No. 6) and the P content in the filtrate was measured using the vanadomolybdate method (Kitson and Mellon Citation1944).
2.5. Organic acid collection from root exudates and analysis
Soybean plants were removed from the soil 6 weeks after sowing, and their roots were gently washed with tap water and then rinsed with milli-Q water (Merk Millipore, Burlington, MA, USA). The roots were then soaked in 100 mL milli-Q water for 2 h to collect the root exudates, and the extracted solutions were freeze-dried. The freeze-dried extracts were suspended in 2 mL milli-Q water and filtered with a 0.22 µm sterile filter unit (Millex®-GV, Merk Millipore, Burlington, MA, USA). Organic acids were analyzed using a high-performance liquid chromatography system equipped with Shim-pack column SCR102 H and RSpack KC-811 (Shimadzu Co., Japan) at a column temperature of 40°C and the UV detector SPD-20A (Shimadzu) at 210 nm. Furthermore, 0.1% (v/v) of H3PO4 was used as the mobile phase in isocratic mode at 0.5 mL min−1. The analysis time was 50 min. Organic acid standards of dicarboxylic acid such as oxalic, malonic, malic, and tartaric acids and citric acid as tricarboxylic acids were used to determine the concentration of each organic acid in the extracts.
2.6. Measurement of organic acid content in the roots
After collecting organic acid extracts from the roots, the roots were cut into 2 cm pieces. The roots (100 mg) were added to a mortar and crushed using liquid nitrogen. MilliQ water (1 mL) was added to the crushed roots to prepare uniform samples. This solution was placed in a 0.45 µm filter tube (Ultrafree®-MC, Merk Millipore, Burlington, MA, USA) and centrifuged at 12,000 × g at 4°C for 5 min and the filtrate was used as the sample solution. The organic acid content was determined using high-performance liquid chromatography under the same conditions as used to measure organic acid secretion levels from the roots described above.
2.7. RNA extraction and quantitative PCR analysis
At 6 weeks after germination, roots were washed with tap water to remove soil and immediately frozen in liquid nitrogen and stored at −80°C until RNA extraction. RNA was extracted from the frozen root samples using the RNeasy Plant Mini Kit (Qiagen, Germany), followed by reverse transcription using the PrimeScript™ RT Reagent Kit with gDNA Eraser (Perfect Real Time) (Takara Bio, Shiga, Japan). Quantitative PCR was conducted using the KAPA SYBR FAST qPCR Master Mix (2X) Kit (KAPA Biosystems, MA, USA) and LightCycler 96 (Roche Diagnostics, Switzerland) following the manufacturer’s protocols. Serial dilutions of purified PCR products derived from the amplification of first-strand cDNA with specific primers were used as relative standards. The primers for GmMATE13 and GmMATE47 are shown in Wang et al. (Citation2019) and Supplementary Table S1. β-Tubulin was used as the control.
2.8. Total P content in plants
Shoots and roots were sampled separately 6 weeks after sowing and oven-dried at 80℃ for 48 h, after which they were powdered using a blender. The dried powder (100 mg) was digested with 2 mL HNO3 and 0.2 mL H2SO4 at 150℃ according to Kuboi (Citation1990). The digested samples were mixed with approximately 50 mL of 0.1 N HCl and subjected to colorimetric analysis via the MoO12S3 method (Murphy and Reley Citation1962).
3. Results
The available S in the Andosol used for soybean cultivation was 21.3 mg S kg−1, and the pH of the soil was 5.2. According to Shukla et al. (Citation2021), soil with available S levels between 15.0 and 22.5 mg S kg−1 is classified as latent deficient soil in India. In Okayama prefecture in Japan, S deficiency symptoms occur in paddy rice grown on cultivated land with an available S content of 19.2 mg S kg−1 (https://www.pref.okayama.jp/uploaded/life/715595_6461837_misc.pdf). Thus, the soil used in this study was considered as S deficient. The phosphate absorption coefficient was as high as 2757 mg P₂O₅ 100 g−1, conforming to the typical properties of Andosol. The available phosphoric acid was 2.31 mg P₂O₅ 100 g−1; the improvement target according to Japan’s Basic Guideline for Soil Fertility Improvement is 10–100 mg P₂O₅ 100 g−1 dry soil in Andosol. Based on this target, the soil used in this study had an extremely low level of available phosphoric acid. Thus, the soil was deficient in both S and P.
Both of concentrations () and amounts () of citric acid and malic acid secreted of from the roots were higher in S fertilization treatment than in the control treatment. Among organic acids in root tissues, tartaric acid and malic acid showed higher concentrations (), and malic acid showed higher amount () under S fertilization treatment than in the control treatment; however, the citric acid concentration and amount did not vary ().
Figure 1. Concentrations (A) and amounts (B) of organic acids exudated from roots. Plants were grown without sulfur application (–S) or with sulfur application (+S). The amounts of organic acids secreted were normalized to root fresh weight (A) or per plant (B). Each value is the mean of 3–5 biological replicates with standard errors. *, **, and *** indicates significant differences from the non-fertilized control by Student’s t-test at p < 0.05, p < 0.01, and p < 0.001, respectively.
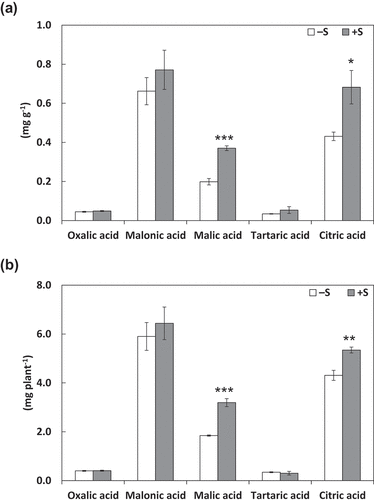
Figure 2. Organic acid concentrations in roots (A) and their total amounts in roots per plant (B). Plants were grown without sulfur application (–S) or with sulfur application (+S). Each value is the mean of 3–5 biological replicates with standard errors. * indicates significant differences from the non-fertilized control using Student’s t-test at p < 0.05.
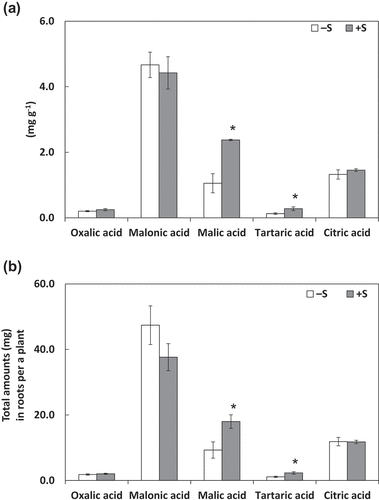
Citric acid as a tricarboxylic acid, is effective in phosphate solubilization in soils, thus it is important to clarify the mechanisms of induction of its secretion by S fertilization. We verified whether expression of the citric acid transporter genes, GmMATE13 and GmMATE47 is involved in the induction of citric acid secretion from the roots by S fertilization. The expression level of GmMATE13 in roots significantly increased by 1.83-fold under S fertilization treatment compared to that in the control treatment ().
Figure 3. Changes in expression of GmMAE13 and GmMATE47 genes in roots in response to sulfur fertilization. Plants were grown without sulfur application (–S) or with sulfur application (+S). Expression levels were measured using quantitative RT-PCR and normalized by those of β-tubulin. Each value is the mean of 4 biological replicates with standard errors. * indicates significant differences from the non-fertilized control by Student’s t-test at p < 0.05.
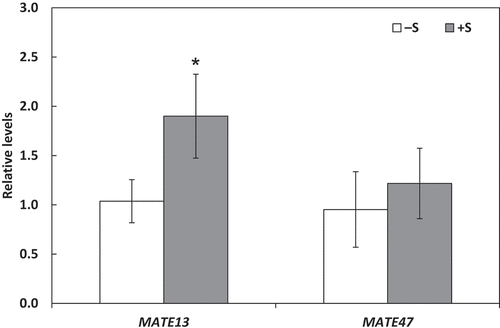
Dry weights and total P content in shoots and roots of 6-week-old soybean plants did not vary significantly between S fertilization treatment and control (Figure S1).
4. Discussion
S fertilization increased organic acid secretion from the roots of soybean grown in Andosol soil, with some organic acid types showing significant differences compared to those without S fertilization. Sugiura et al. (Citation2021) observed that the organic acid secretion from the roots increased when soybeans were cultivated in the presence of poorly soluble calcium phosphate as a P source in vermiculite. In this study, we used andosol, which shows a strong P fixation capacity and observed an increase in organic acid secretion from the soybean roots. The total P content in shoots in calcium phosphate supplemented vermiculite was slightly but significantly increased by S application (Sugiura et al. Citation2021); however, this increase was not observed in our study using Andosol soil (Figure S1). As discussed in Sugiura et al. (Citation2021), more than half of total P content in plants is supplied from the seeds at this age of soybean plants, which might have masked the smaller differences. Although Andosol, the test soil used in this study, is categorized as S deficient, some amounts of available sulfur are contained, which might additionally be responsible for the smaller effects of S fertilization in this soil compared to the vermiculite with almost no available sulfur. Irrespective of the smaller effects, organic acid secretion was increased by S application in this study with Andosol as well as with the vermiculite, indicating that physiological responses for the synthesis or secretion of organic acids were induced in soybean plants grown in Andosol.
Malic acid concentrations, both in the root exudates and within the roots were increased by S application. While the amount of citric acid secreted from the roots was significantly increased after S fertilization without significant difference in its content within root tissues. The contents in organic acids in root tissues are the balance of their synthesis and secretion, thus we cannot conclude that only secretion was induced for citric acid without induction of synthesis. As S nutrition is required for photosynthesis (Hawkesford et al. Citation2012), carbon fixation in leaves is considered to be activated by S application, followed by carbon transport into roots resulting in increase of organic acid in roots. Even though, it is likely that secretion of citric acid was more highly induced than its synthesis. While for malic acid, both the synthesis and secretion are considered to be highly induced by S application, resulting in an increase in both the secreted amount and content in root. Further studies are required to determine the mechanisms governing the variations between the secreted and internal levels of organic acids in response to S nutrition.
As citric acid is a tricarboxylic acid which can solubilize P in soils effectively, it is important to clarify the mechanisms of induction of its secretion by S fertilization. Therefore, the relationship between S nutrition and the gene expression of citric acid exporters was investigated. The expression level of a citric acid exporter gene, GmMATE13 was significantly increased following S fertilization, indicating that S nutrition induces GmMATE13 expression and consequently contributes to its excretion from the roots.
Wang et al. (Citation2019) investigated the relationship between Hydrogen sulfide (H₂S) and the expression of the citric acid transporter genes, GmMATE13 and GmMATE47 following aluminum treatment. H₂S has gained attention as a signal molecule involved in plant tolerance to various stresses (Singh et al. Citation2020). Aluminum treatment led to increased H₂S levels, induced citric acid secretion, and increased the expression levels of GmMATE13 and GmMATE47. Thus, H₂S may be involved in inducing GmMATE13 and GmMATE47 expression and promoting citric acid secretion. Our results suggest that GmMATE13 expression is induced in soybean roots following S application in the form of sulfate. In plant S assimilation pathway, sulfate absorbed by plants are reduced to sulfide, followed by incorporation into cysteine (Cys). Subsequently, H2S is synthesized from Cys in the chloroplasts using cysteine desulfhydrase and β-cyanoalanine synthase. Thus, sulfate is the donor for H2S production in plants (Khan et al. Citation2022). Sulfate fertilization is considered to increase H₂S in the plant body, leading to induced expression of GmMATE13. Further investigations are required for the commonality of signaling such as H₂S in regulating GmMATE expression under aluminum stress and S fertilization, and the location of the GmMATEs secreting organic acids from roots into the rhizosphere.
Disclosure statement
The authors declare that they have no known competing financial interests or personal relationships that could have appeared to influence the work reported in this paper.
Additional information
Funding
References
- Bolan, N. S., R. Naidu, S. Mahimairaja, and S. Baskaran. 1994. “Influence of Low-Molecular-Weight Organic-Acids on the Solubilization of Phosphates.” Biology and Fertility of Soils 18 (4): 311–319. doi:10.1007/BF00570634.
- Hawkesford, M., W. Horst, T. Kichey, H. Lambers, J. Schjoerring, I. S. Moller, and P. White. 2012. “Functions of Macronutrients.” In Marschner’s Mineral Nutrition of Higher Plants, edited by P. Marschner, 135–189. 3rd ed. Netherlands: Academic Press Amsterdam.
- Johnson, S. E., and R. H. Loeppert. 2006. “Role of Organic Acids in Phosphate Mobilization from Iron Oxide.” Soil Science Society of America Journal 70 (1): 222–234. doi:10.2136/sssaj2005.0012.
- Khan, M. S. S., F. Islam, Y. Ye, M. Ashline, D. Wang, B. Zhao, Z. Q. Fu, and J. Chen. 2022. “The Interplay between Hydrogen Sulfide and Phytohormone Signaling Pathways under Challenging Environments.” International Journal of Molecular Sciences 23 (8): 4272. doi:10.3390/ijms23084272.
- Kitson, R., and M. G. Mellon. 1944. “Colorimetric Determination of Phosphorus as Molybdivanadophosporic Acid.” Industrial and Engineering Chemistry, Analytical Edition 16 (6): 379–383. doi:10.1021/i560130a017.
- Kpomblekou-A, K., and M. A. Tabatabai. 2003. “Effect of Low-Molecular Weight Organic Acids on Phosphorus Release and Phytoavailabilty of Phosphorus in Phosphate Rocks Added to Soils.” Agriculture, Ecosystems & Environment 100 (2–3): 275–284. doi:10.1016/S0167-8809(03)00185-3.
- Kuboi, T. 1990. “Ashing: 4. Digestion by Nitric Acid - Sulfuric Acid.” Experiment Methods for Plant Nutrition, edited by Japanese Society of Soil Science and Plant Nutrition 126. Tokyo: Hakuyusha.
- Liu, J., Y. Li, W. Wang, and J. Gai. 2016. “Genome-wide Analysis of MATE Transporters and Expression Patterns of a Subgroup of MATE Genes in Response to Aluminum Toxicity in Soybean.” BMC Genomics 17 (1): 223. doi:10.1186/s12864-016-2559-8.
- Magalhaes, J. V. 2010. “How a Microbial Drug Transporter Became Essential for Crop Cultivation on Acid Soils: Aluminium Tolerance Conferred by the Multidrug and Toxic Compound Extrusion (MATE) Family.” Annals of Botany 106 (1): 199–203. doi:10.1093/aob/mcq115.
- Miyatake, M., T. Ohyama, T. Yokoyama, S. Sugihara, T. Motobayashi, T. Kamiya, T. Fujiwara, K. Yuan, S. D. Bellingrath-Kimura, and N. Ohkama-Ohtsu. 2019. “Effects of Deep Placement of Controlled-Release Nitrogen Fertilizer on Soybean Growth and Yield under Sulfur Deficiency.” Soil Science and Plant Nutrition 65 (3): 259–266. doi:10.1080/00380768.2019.1615827.
- Murphy, J., and J. Reley. 1962. “A Modified Single Solution Method for the Determination of Phosphate in Natural Waters.” Analytica Chimica Acta 27: 31–36. doi:10.1016/S0003-2670(00)88444-5.
- Ohwaki, Y., and H. Hirata. 1992. “Differences in Carboxylic Acid Exudation among P-Starved Leguminous Crops in Relation to Carboxylic Acid Contents in Plant Tissues and Phospholipid Level in Roots.” Soil Science and Plant Nutrition 38 (2): 235–243. doi:10.1080/00380768.1992.10416486.
- Shukla, A. K., S. K. Behera, C. Prakash, A. Tripathi, A. K. Patra, B. S. Dwivedi, V. Trivedi, et al. 2021. “Deficiency of Phyto-Available Sulphur, Zinc, Boron, Iron, Copper and Manganese in Soils of India.” Scientific Reports 11 (1): 19760. doi:10.1038/s41598-021-99040-2.
- Singh, S., V. Kumar, D. Kapoor, S. Kumar, D. S. Dhanjal, S. Datta, J. Samuel, et al. 2020. “Revealing on Hydrogen Sulfide and Nitric Oxide Signals Co-Ordination for Plant Growth under Stress Conditions.” Physiologia Plantarum 168 (2): 301–317. doi:10.1111/ppl.13002.
- Stumpf, D. K., and R. H. Burris. 1981. “Organic Acid Contents of Soybean: Age and Source of Nitrogen.” Plant Physiology 68 (5): 989–991. doi:10.1104/pp.68.5.989.
- Sugiura, H., S. Sugihara, T. Kamiya, M. D. A. Ramirez, M. Miyatake, T. Fujiwara, O. Takuji, et al. 2021. “Sulfur Application Enhances Secretion of Organic Acids by Soybean Roots and Solubilization of Phosphorus in Rhizosphere.” Soil Science and Plant Nutrition 67 (4): 400–407. doi:10.1080/00380768.2021.1919011.
- Truog, E. 1930. “The Determination of Readily Available Phosphorus in Soils.” Journal of the America Society of Agronomy 22: 874–882. doi:10.2134/agronj1930.00021962002200100008x.
- van Reeuwijk, L. 2002. Procedures for Soil Analysis. Wageningen: International soil and reference information centre. https://www.isric.org/sites/default/files/ISRIC_TechPap09.pdf.
- Wang, Y., P. Marschner, and F. Zhang. 2012. “Phosphorus Pools and Other Soil Properties in the Rhizosphere of Wheat and Legumes Growing in Three Soils in Monoculture or as a Mixture of Wheat and Legume.” Plant and Soil 354 (1–2): 283–298. doi:10.1007/s11104-011-1065-7.
- Wang, H., F. Ji, Y. Zhang, J. Hou, W. Liu, J. Huang, and W. Liang. 2019. “Interactions between Hydrogen Sulphide and Nitric Oxide Regulate Two Soybean Citrate Transporters during the Alleviation of Aluminium Toxicity.” Plant, Cell & Environment 42 (8): 2340–2356. doi:10.1111/pce.13555.
- Wu, L. J., Y. Kobayashi, J. Wasaki, and H. Koyama. 2018. “Organic Acid Excretion from Roots: A Plant Mechanism for Enhancing Phosphorus Acquisition, Enhancing Aluminum Tolerance, and Recruiting Beneficial Rhizobacteria.” Soil Science and Plant Nutrition 64 (6): 697–704. doi:10.1080/00380768.2018.1537093.