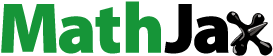
ABSTRACT
Countermeasures to reduce radiocesium uptake by crops have been implemented in farmlands affected by the accident at Tokyo Electric Power Company’s Fukushima Daiichi Nuclear Power Plant in 2011. A widely practiced countermeasure is the application of K. Long-term maintenance of soil K is crucial due to the long physical half-life of 137Cs (30 years). Plant-available K budgets can be used as an indication of sustainable maintenance. Our previous study found that large water outflow, especially percolation, associated with excessive irrigation caused major K outflow and a negative K budget in a paddy field in Fukushima in 2018. The present study evaluated whether decreasing the water outflow by reducing the irrigation volume from 6300 to 1900 mm reduced K outflow and improved the K budget in the field during the cropping period in 2019. K concentrations in soil solution were comparable in both years, and the percolation water volume decreased to 37% that of 2018, resulting in a 53% reduction in the K output from 392 to 184 kg ha–1. The input via irrigation also decreased (−35 kg ha–1), but the output via surface runoff showed a greater reduction (−58 kg ha–1) owing to a 70% decrease in the water volume compared with 2018. Consequently, the K budget was substantially improved (from −289 to −57 kg ha–1), highlighting the importance of controlling water outflow, particularly percolation, for soil K maintenance in paddy fields with high permeability.
KEYWORDS:
1. Introduction
The accident at Tokyo Electric Power Company’s Fukushima Daiichi Nuclear Power Plant in 2011 caused radionuclide contamination in eastern Japan. The radionuclides 131I, 134Cs, and 137Cs are particularly problematic for radiation exposure. 131I has a short physical half-life (8 days) and was present in the environment only immediately after the accident. In contrast, 134Cs and 137Cs have relatively long half-lives (2 and 30 years, respectively) and are characterized by their strong adsorption to clay minerals in soil (Cremers et al. Citation1988), leading to prolonged contamination in terrestrial ecosystems, for example, after the Chernobyl disaster (International Atomic Energy Agency Citation2006; Onda et al. Citation2020). Accordingly, extensive countermeasures against radiocesium have been implemented in eastern Japan.
Topsoil was removed from farmland in highly contaminated areas (>5000 Bq kg−1 in soil) around Fukushima to lower external exposure during farm work and the radiocesium concentration of agricultural products (Ministry of the Environment Citation2013). Kurokawa et al. (Citation2019) observed an 80% decrease in the concentration on average after decontamination in a town but with occasional fields of radiocesium contamination at levels of thousands of becquerels per kilogram. In this context, current guidelines (Fukushima Prefectural Government Citation2019) recommend maintaining the soil-exchangeable K content to reduce radiocesium uptake by crops. This countermeasure is based on the competition between K and Cs for uptake by plants. For rice, the staple grain in Japan, the target K content is >0.53 cmol K kg−1 at the beginning of cultivation in Fukushima. Owing to these countermeasures, radiocesium activity concentration in brown rice has not exceeded the safety standard limit of 100 Bq kg−1 since 2015 (Fukushima Prefectural Government Citation2021).
In part of Fukushima Prefecture, the countermeasure of increasing exchangeable K content was ended in 2019 after safety testing (Fukushima Prefectural Government Citation2019). However, the K contents could subsequently decrease to the level before the countermeasure was implemented if farmers do not pay attention to K application. Some experimental studies (Fukushima Prefectural Government Citation2019; Fujimura et al. Citation2020) have reported that the radiocesium activity concentration in rice could increase again as the exchangeable K content decreases. Therefore, developing an appropriate K maintenance plan to control long-term radiocesium uptake is important for paddy fields affected by the Fukushima Daiichi nuclear accident.
K is a macronutrient in crops, and its maintenance has been drawing increasing attention worldwide in relation to soil degradation and growing food demand as the global population increases. K is less retained in soils with a low content of clay minerals that selectively adsorb K, abundant competing cations, and high permeability (Askegaard, Eriksen, and Johnston Citation2004; Katoh et al. Citation2004; Mendes et al. Citation2016; Rosolem and Steiner Citation2017; Goulding et al. Citation2021). Total seasonal water input to paddy fields, which is typically 1300–1500 mm in Asia, is up to two to three times higher than that for other cereals and reaches more than 2000 mm in coarse‐textured (sandy or loamy) soils (Bouman et al. Citation2007). Thus, severe K leaching might occur in paddy fields with high permeability. In our previous study in 2018 in a paddy field with high permeability in Fukushima (Nishikiori et al. Citation2020), we observed such leaching (392 kg ha–1 crop–1), which resulted in a strongly negative K budget (−289 kg ha–1 crop–1). The field had a high irrigation volume (6300 mm) and the surface runoff (81 kg ha–1 crop–1) also increased K output. Thus, preventing excessive irrigation is expected to decrease the water volumes of percolation and surface runoff and decrease K outflow. In the present work, we evaluated the improvement in K budget when water outflow was reduced by controlling excessive irrigation in paddy fields. Although such tests have been conducted on columns (Mendes et al. Citation2016), to our knowledge, this is the first time they have been conducted on an entire field. The findings of a test conducted on this scale would be a meaningful for proposing countermeasures against radiocesium but also K maintenance in paddy fields in other regions.
2. Materials and methods
2.1. Site description
Detailed information on the experimental field has been described in our previous study (Nishikori et al. 2020). The field was 10 km northwest (37°30ʹ01”N and 140°58ʹ25”E) of the Fukushima Daiichi Nuclear Power Plant. The total deposition density of 134Cs and 137Cs was 2100 kBq m−2 (decay correction date: 2 July 2011) according to an airborne monitoring survey (Japan Atomic Energy Agency Citation2022). At the nearest meteorological weather station (AMeDAS Namie) in 2010–2019, the mean temperature was 13.1°C and precipitation was 1470 mm (Japan Meteorological Agency Citation2022).
The soil type was Gray Lowland soils (Gleyic Fluvisol). The field was decontaminated by the removal of plowed soil at a depth of approximately 5 cm and the addition of uncontaminated soil. The basic properties of plowed soil are presented in . The soil consisted of clay loam including gravel and had low total C, N, and cation-exchange capacity, but high exchangeable K.
Table 1. Basic properties of plowed soils.
Rice seedlings (Oryza sativa L., Koshihikari) were transplanted in May and harvested in October in both 2018 and 2019. Midsummer drainage was conducted in the middle of July. The fertilizer application rate of N-P-K (kg ha−1) was 82–75-149. The primary types of N, P, and K fertilizers were ammonium nitrogen, fused magnesium phosphate, and potassium chloride, respectively. Fertilizers were applied before transplanting. Harvested straw was returned to the field immediately after harvesting. The irrigation water source was the neighboring Ukedo River. Irrigation, transplantation, and fertilization were managed the same way by the farmer in both years, and accordingly, irrigation volume was adjusted by enlarging the field area from 400 m2 (5 × 80 m) in 2018 to 1400 m2 (14 × 100 m) in 2019. The fields (i.e., plots) were established in the same part of the paddy in both years. In 2018, the three longer sides were surrounded by plastic plates and the shorter side was surrounded by a bund, whereas in 2019, the three sides were surrounded by bunds and the remaining longer side was surrounded by plastic plates.
2.2. Hydrological observation, sampling, and K analysis
The observation and analysis methods were the same as those used in our previous work (Nishikiori et al. Citation2020), and the equipment model names are omitted here. Flow rates of irrigation and surface runoff were recorded at 10-min intervals using a Parshall flume and electromagnetic flowmeters. Flooding water depth was monitored using a water gauge, and the mean water requirement, measured as depth at night with no precipitation and no irrigation (n = 7 in 2018 and n = 9 in 2019), was used as the percolation rate. Water flow paths for infiltration consist of percolation (the down flow below the root zone) and seepage (the lateral subsurface flow through bunds). We assumed that the infiltration was solely caused by percolation in this field for the following reason. Because the field was surrounded mainly by plastic plates in 2018, seepage was negligible. Although three sides of the field were surrounded by bunds in 2019, the water requirement remained the same in both years, except in September 2019 when the values decreased (). This probably indicates a limited amount of seepage in 2019.
Table 2. Water budget (mm) in fields during the cropping period in 2018 and 2019.
The amount of precipitation was observed using a rain gauge. Crop evapotranspiration was quantified according to Food and Agriculture Organization guidelines (Allen et al. Citation1998), and the meteorological data required for the calculation were obtained from nearby meteorological weather stations (AMeDAS Namie and Onahama) (Japan Meteorological Agency Citation2022).
Irrigation water was collected from the canal every few days in polypropylene bottles. Ponding water near the outlet was collected every week and treated as surface runoff water. K input from precipitation was negligible in 2018, and samples were not collected. Two acrylic boxes were installed in the field at locations away from the inlet and outlet to obtain soil solutions from plowed soil. Samples were collected from depths of 4 and 11 cm in triplicate using sampling devices with porous cups every 2 weeks. Dissolved K concentration in water samples was measured using an ion chromatograph. Plant-available K in suspended solids in water samples was not analyzed because the amount in pathways, including irrigation and surface runoff, was estimated to be <1 kg ha−1 crop−1 (see the explanation in the previous study; Nishikiori et al. Citation2020).
The aboveground parts of the rice plant were collected from an area of 0.34 m2 in triplicate on 27 September 2019. Plants were separated into parts (brown rice, husk, and straw), dried for 48 h at 80°C in an oven, weighed, and ground using a mill for husk and straw. Then, 0.5 g of material was digested with HNO3, and the K concentration was determined by an atomic absorption spectrophotometer.
The amount of K via pathways was calculated by multiplying the K concentration by the water volume (or plant weight) and integrating it over each water sampling interval. The K budget during the observation period was determined by
where F is K in the applied fertilizer, I is K in the irrigation water, S is K in straw returned to the soil, R is K in harvested rice plants, SR is K in surface runoff, and Pc is K in percolated water. To quantify K leaching from plowed soil, the mean value for the soil solution from the lower part of plowed soil (11 cm in depth) was applied to the value via percolation. The budget of rice plant-available K in plowed soil was the focus of this study.
3. Results and discussion
3.1. Changes in water budget
The irrigation volume in 2019 was 30% that in 2018, and consequently the volumes of surface runoff and percolation in 2019 were decreased to 30% and 37% of those in 2018 (). The reason for the large decrease in percolation along with surface runoff was probably because the number of days that the daily inflow exceeded the daily percolation rate (24 mm) in 2019 declined to one-third that of the previous year (99 days in 2018 and 34 days in 2019). However, the surface runoff volume was still over 1000 mm, and it was possible to reduce the irrigation volume further.
3.2. K concentration
K concentrations in irrigation water were fairly constant (1 mg L–1) with one exceptionally high measurement during the cultivation period in 2018 and 2019 (). The K concentrations in surface runoff water were similar in both years and exceeded 3 mg L–1 at the beginning, declined until July, and then increased (). The K concentrations in soil solutions continued to decline over the cultivation period at a similar level in both years (); however, the decrease was more gradual in 2019, presumably due to reduced K leaching associated with lower water percolation ().
Figure 1. Temporal changes in K concentrations in (a) irrigation water, (b) surface runoff water, and (c) soil solution in the plowed soil during the cropping period in 2018 and 2019. The values and error bars in (c) are means and standard deviations, respectively.
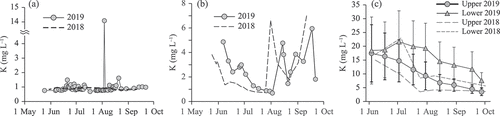
The re-elevation of the concentrations in surface runoff water could be due to diffusion from the soil solution or soil. The major K uptake of the rice variety (Oryza sativa L., Koshihikari) is completed approximately 70 days after transplanting (Ishikawa et al. Citation2021), which corresponded to the end of July at our test site. At this time, the K concentrations in the soil solution were several times higher than in the surface runoff water. From August in both years, the concentrations in surface runoff water increased to the same level as the soil solution, but it is unlikely that the diffusion between the continuously infiltrating surface water and soil solution achieved equilibrium in the highly permeable field. Consequently, there may be additional K sources, such as exudation from mature rice plants.
The aboveground biomass of rice plants was 13 t ha–1 (5.3, 1.2, and 6.7 t ha–1 for brown rice, husk, and straw, respectively), and the K concentration was 11 g kg–1 (2.5, 5.7, and 19 g kg–1 for brown rice, husk, and straw, respectively). The amount of K was 147 kg ha–1 (13, 6.8, and 127 kg ha–1 for brown rice, husk, and straw, respectively), which was roughly the same as 155 kg ha–1 in 2018.
3.3. Changes in K input, output, and budget
In 2019, daily inflow and outflow of K were clearly reduced owing to the large decrease in water volume by reducing irrigation (), despite the similar K concentrations in irrigation water, surface runoff water, and soil solution (). Although this resulted in a 13% decrease in the total K input (–43 kg ha–1), the decrease of the total output (–275 kg ha–1) was substantially greater, which resulted from 72% and 53% reductions in surface runoff and percolation, respectively (). Consequently, the K budget improved greatly from −289 kg ha–1 in 2018 to −57 kg ha–1 in 2019.
Table 3. K input, output, and budget (kg ha–1) during the cropping period in 2018 and 2019.
Figure 2. Daily K inflows and outflows and cumulative amounts during the cropping period in 2018 and 2019.
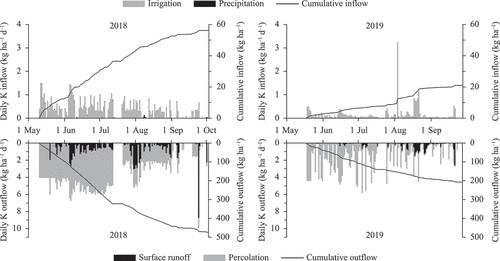
These results demonstrated that the large volume of water outflow associated with excess irrigation led to a substantial K outflow, especially via leaching, and a negative budget in this field, and that K outflow was mitigated by controlling the irrigation volume and reducing the water outflow. However, the leaching of 184 kg ha–1 exceeded the application rate of 149 kg ha–1 () and was still severe. Further reduction may be possible by reducing the irrigation volume further (see Section 3.1). Fertilizer reduction may also be another option. Leaching tends to increase with the fertilizer application rate (Rosolem and Steiner Citation2017), and in our study field, the recommended value for exchangeable K (>0.53 cmol K kg−1 at the beginning of the cultivation) was adequately met even in the harvest season ().
This finding is meaningful for K maintenance in paddy fields not only in Fukushima but also those elsewhere in the world. However, there are fields that require excess irrigation, including those with plot-to-plot irrigation. For the best management practice, it is necessary to determine the optimal irrigation volumes and application rates for the most commonly used highly permeable soil types and irrigation systems in future work.
Acknowledgments
We thank S. Fujimura, S. Wakabayashi, Y. Abe, and the staff of Technical Support Center Operations Unit 2 (National Agriculture and Food Research Organization) for advice and assistance with chemical analyses and sampling.
Disclosure statement
No potential conflict of interest was reported by the authors.
Additional information
Funding
References
- Allen, R., L. A. Pereira, D. Raes, and M. Smith. 1998. Crop Evapotranspiration: Guidelines for Computing Crop Water Requirements. FAO Irrigation and Drainage Paper 56. Rome: Food and Agriculture Organization.
- Askegaard, M., J. Eriksen, and A. E. Johnston. 2004. “Sustainable Management of Potassium.” In Managing Soil Quality: Challenges in Modern Agriculture, edited by P. Schjønning, S. Elmholt, S., Christensen, B. T., 85–102. Wallingford: CABI Publishing.
- Bouman, B. A., E. Humphreys, T. P. Tuong, and R. Barker. 2007. “Rice and Water.” Advances in Agronomy 92: 187–237. doi:10.1016/S0065-2113(04)92004-4.
- Carey, P. L., D. Curtin, and C. L. Scott. 2011. “An Improved Procedure for Routine Determination of reserve-K in Pastoral Soils.” Plant and Soil 341 (1–2): 461–472. doi:10.1007/s11104-010-0658-x.
- Cremers, A., A. Elsen, P. De Preter, and A. Maes. 1988. “Quantitative Analysis of Radiocaesium Retention in Soils.” Nature 335 (6187): 247–249. doi:10.1038/335247a0.
- Fujimura, S., J. Ishikawa, K. Niitsuma, S. Wakabayashi, and T. Shinano. 2020. “Risk Assessment for Radiocesium Concentration in Brown Rice Cultivated in Paddy Field (In Japanese).” Japanese Journal of Soil Science and Plant Nutrition 91 (4): 237–244. doi:10.20710/dojo.91.4_237.
- Fukushima Prefectural Government. 2019. “Guidelines for Decontamination and Technical Measures Related to Countermeasures against Radiocesium in Agricultural Products, Third Ed (In Japanese)”. Accessed 27 April 2022. https://www.pref.fukushima.lg.jp/sec/36021a/nogyo-nousin-gijyutu05.html
- Fukushima Prefectural Government. 2021. “Monitoring Results of Agricultural, Forestry and Fishery Products from Fukushima Prefecture”. Accessed 27 April 2022. https://www.pref.fukushima.lg.jp/site/portal-english/en01-01.html
- Goulding, K., T. S. Murrell, R. L. Mikkelsen, C. Rosolem, J. Johnston, H. Wang, and M. A. Alfaro. 2021. “Outputs: Potassium Losses from Agricultural Systems.” In Improving Potassium Recommendations for Agricultural Crops, edited by T. S. Murrell, Mikkelsen, R. L., Sulewski, G., Norton, R., Thompson, M. L., 47–73. Cham: Springer.
- International Atomic Energy Agency. 2006. Environmental Consequences of the Chernobyl Accident and Their Remediation: Twenty Years of Experience Report of the Chernobyl. Vienna: International Atomic Energy Agency.
- Ishikawa, J., S. Fujimura, M. Murai-Hatano, K. Baba, M. Furuya, A. Goto, and M. Kondo. 2021. “Changes in Cesium Distribution in field-grown Rice Plants Throughout the Cultivation Period.” Plant and Soil 469 (1): 475–487. doi:10.1007/s11104-021-05189-0.
- Japan Atomic Energy Agency. 2022. “Database for Radioactive Substance Monitoring Data”. Accessed 27 April 2022. https://emdb.jaea.go.jp/emdb/
- Japan Meteorological Agency. 2022. “Data and Documents (In Japanese)”. Accessed 27 April 2022. https://www.jma.go.jp/jma/menu/menureport.html
- Japanese Society of Soil Science and Plant Nutrition. 1997. Methods of Soil Environmental Analysis (In Japanese). Tokyo: Hakuyu-sha.
- Katoh, M., J. Murase, M. Hayashi, K. Matsuya, and M. Kimura. 2004. “Nutrient Leaching from the Plow Layer by Water Percolation and Accumulation in the Subsoil in an Irrigated Paddy Field.” Soil Science and Plant Nutrition 50 (5): 721–729. doi:10.1080/00380768.2004.10408528.
- Kurokawa, K., A. Nakao, H. Tsukada, Y. Mampuku, and J. Yanai. 2019. “Exchangeability of 137Cs and K in Soils of Agricultural Fields after Decontamination in the Eastern Coastal Area of Fukushima.” Soil Science and Plant Nutrition 65 (4): 401–408. doi:10.1080/00380768.2019.1622402.
- Mendes, W. D. C., J. Alves Júnior, P. C. Da Cunha, A. R. D. Silva, A. W. Evangelista, and D. Casaroli. 2016. “Potassium Leaching in Different Soils as a Function of Irrigation Depths.” Revista Brasileira de Engenharia Agrícola e Ambiental 20: 972–977. doi:10.1590/1807-1929/agriambi.v20n11p972-977.
- Ministry of the Environment. 2013. Decontamination Guidelines, Second Ed. Tokyo: Ministry of the Environment.
- Nishikiori, T., T. Kubota, S. Miyazu, N. Harada, N. Yoshikawa, H. Fujiwara, and T. Saito. 2020. “Input and Output Pathways Determining Potassium Budgets in Two Paddy Fields Subjected to Countermeasures against Radiocesium in Fukushima, Japan.” Plos one 15 (4): e0232139. doi:10.1371/journal.pone.0232139.
- Onda, Y., K. Taniguchi, K. Yoshimura, H. Kato, J. Takahashi, Y. Wakiyama, F. Coppin, and H. Smith. 2020. “Radionuclides from the Fukushima Daiichi Nuclear Power Plant in Terrestrial Systems.” Nature Reviews Earth & Environment 1 (12): 644–660. doi:10.1038/s43017-020-0099-x.
- Rosolem, C. A., and F. Steiner. 2017. “Effects of Soil Texture and Rates of K Input on Potassium Balance in Tropical Soil.” European Journal of Soil Science 68 (5): 658–666. doi:10.1111/ejss.12460.