ABSTRACT
Japanese wasabi is traditionally cultivated in terraced fields with running stream water conditions which typically produce a high-quality of yield. Previous studies have explored some of the effects of water nutrient status under such conditions; however, the relationship between water nutrient status in these cultivation conditions and the growth and quality of wasabi is still completely unknown. We evaluated the effects of nutrient strength, inorganic nitrogen sources, and pH of the medium on the growth of wasabi plants. Then, we analyzed the photosynthetic capacity and the ionomes of two major Japanese wasabi cultivars (‘Onimidori,’ an early-growing green-stem type; and ‘Mazuma No.1,’ a late-growing red-stem type) grown under a range of pH conditions. These experiments were conducted using a hydroponic system. Wasabi plants showed the best shoot and root growth in 1/10- and 1/2-strength Hoagland’s solution with a 50:50 ratio of NH4-N:NO3-N. On the basis of the accumulation patterns of NO3−, amino acids, and chlorophylls, the 1/10-strength nutrient solution provided optimal conditions for wasabi growth. For both wasabi cultivars, the best shoot and root growth was in medium at pH 6.0. The photosynthetic capacity of ‘Onimidori’ was greater than that of ‘Mazuma No.1,’ suggesting that this may contribute to the faster growth of ‘Onimidori.’ Ionome analyses revealed tissue-specific mineral accumulation patterns and their differences among different pH conditions and between the two cultivars. Ionome-based multiple-regression analysis revealed a relationship between element concentration profiles in the wasabi plants, especially those in root, and the shoot growth. Wasabi plants are ammonium-sensitive and nitrate-preferring plants, in addition to relatively low nutrient requirements for optimal growth. The results of this study describe the basic nutritional characteristics of wasabi plants. These findings represent an important step toward optimizing fertilization to control wasabi quality and growth in the traditional flooded cultivation system.
KEY WORDS:
1. Introduction
Wasabi (Eutrema japonicum (Miq.) Matsum.) is a perennial plant native to Japan, where it has been cultivated for more than a thousand years. Until around 1990, wasabi cultivation and research had only been conducted in Japan. Since then, the demand for wasabi has increased with the spread of Japanese cuisine to modern Western food (Chadwick, Lumpkin, and Elberson Citation1993). Currently, wasabi plants are grown in upland or flooded field of many other countries around the world such as New Zealand, Taiwan, South Korea, Israel, Brazil, Thailand, Columbia, Canada, U.S.A., Great Britain, and China (Gałczyńska et al. Citation2017).
Japanese wasabi, known as ‘Sawa wasabi’ or ‘Mizu wasabi,’ is traditionally cultivated in a flooded system using terraced fields and running stream water. This method of cultivation yields primarily rhizomes, which are the enlarged stems of the plant. Sawa wasabi is highly valued and commands premium prices in the market (Chadwick, Lumpkin, and Elberson Citation1993). Upland cultivation of wasabi produces leaves and petioles as the main agricultural products. Rhizomes start to form as the wasabi plant ages. The growth rate of wasabi plants in the flooded system is very slow. It takes 18 months for wasabi plants to form the distinctive thickened rhizome (Sultana and Savage Citation2008). To increase the growth rate of wasabi plants, it is necessary to clarify the cultivation conditions that are suitable for their growth. Several studies have explored the effects of environmental factors (water temperature, O2 saturation and quantity, air temperature) on wasabi growth and quality (Chadwick, Lumpkin, and Elberson Citation1993; Sultana and Savage Citation2008; Tanaka et al. Citation2009). An adequate supply of nutrients is also important for the efficient production of large, uniform rhizomes (Chadwick, Lumpkin, and Elberson Citation1993). Generally, the concentrations of essential minerals in the culture medium are important factors affecting the plant growth rate. In particular, the amount and form of nitrogen (N) greatly influence plant growth and quality. The preference for N in the form of ammonium (NH4-N), nitrate (NO3-N), or a combination of these two inorganic forms, differs among plant species (Britto and Kronzucker Citation2002; Raven, Wollenweber, and Handley Citation1992). The preferred N source is often NH4-N, because it is an intermediate in many metabolic reactions (Bloom, Sukrapanna, and Warner Citation1992). However, plants can show symptoms of NH4+ toxicity if only NH4-N nutrition is supplied (Findenegg Citation1987), suggesting that NO3-N is also required for optimal growth (Rideout et al. Citation1994). The optimal combination of inorganic N sources for wasabi plants is still unknown.
Wasabi plants in this flooded system are cultivated with only stream water. One study found that the pH of irrigation water in the flooded wasabi fields was in the range of 6.0 to 7.5 (Adachi Citation1987), and that the nutrient contents in irrigation water were higher in superior wasabi fields than in inferior wasabi fields: for N, 3.0 times higher; for P205, 2.9 times higher; and for K2O, 1.6 times higher (Adachi Citation1987). In addition, there was more NO3-N than NH4-N in the irrigation water (Adachi Citation1987). Thus, flooded cultivation is sometimes managed by utilizing coated fertilizers and foliar sprays (Adachi Citation1987). These previous reports suggest that the growth and quality of wasabi is closely related to the N source status of the growing environment, but this relationship has not yet been investigated. Besides that study, few other studies have focused on the relationship between the nutrient status under flooded cultivation conditions and the growth and quality (pungency, odor, etc.) of wasabi plants.
The ionome is defined as the elemental composition of a subcellular structure, cell, tissue organ or organism (Salt, Baxter, and Lahner Citation2008). In plants, especially the shoot ionome, it is controlled by various physiological processes that originate in the rhizosphere and extend to evapotranspiration and phloem recycling. Consequently, the shoot ionome is likely to be highly responsive to the plant’s physiological state, with distinct ionomic signatures reflecting different physiological conditions (Baxter et al. Citation2008). Changes in the rhizosphere environment can also impact the plant ionome (Watanabe et al. Citation2015). Watanabe et al. (Citation2022) observed alterations in the ionomic profiles of certain crops cultivated in a field subjected to long-term fertilizer treatments [complete fertilization, fertilization without N, without phosphorus (P), without potassium (K), and no fertilization] in response to N, P, and K deficiencies. However, the ionomic profiles and their dynamic responses to different nutrient conditions in wasabi plants are still unknown.
In this study, first, we evaluated the effects of nutrient strength on the growth of wasabi plants by growing them in a hydroponic system with different NO3-N/NH4-N ratios in the culture solution. Second, we evaluated the effects of rhizosphere pH conditions on the growth and ionomic profiles of two major Japanese wasabi cultivars; an early-growing green-stem type and a late-growing red-stem type. Finally, we evaluated the relationship between tissue mineral concentration and shoot growth based on regression models to reveal the optimal nutrient status in wasabi plants.
2. Materials and Methods
2.1. Plant materials and hydroponic cultivation system
Wasabi plants were grown in a hydroponic system under ambient light in an unheated greenhouse (120 m2) at Shizuoka University (Shizuoka, Japan) from December to March of 2018 and 2019. One-year-old wasabi mericlone seedlings of two leading Japanese wasabi cultivars, ‘Onimidori’ (early-growing green-stem type) and ‘Mazuma No.1’ (late-growing red-stem type) (Miyoshi Agritech & Co., Ltd.), were transplanted into Wagner pots (1 a/5000; one individual per pot) containing 3.0 L of 1/100-strength modified Hoagland’s solution (Table S1) adjusted to pH 6.0 or 7.0, and continuously aerated. The temperatures of air in the greenhouse and solutions in the pots were recorded hourly with an environment data logger (Ondotori TR7 series or TR5i series, T&D Corp. Matsumoto, Japan) and are shown in Figure S1.
The first hydroponic experiment (2018–2019) tested the effects of inorganic N sources and ionic strength of the medium on plant growth. After 1 month in 1/100-strength Hoagland’s solution at pH 6.0, ‘Onimidori’ plants were transferred to treatment solutions with a range of ionic strengths (1/100-, 1/10-, and 1/2-strength Hoagland’s solution) and different ratios of inorganic N: i.e., NH4-N:NO3-N, 100:0, 90:10, 75:25, 50:50, 25:75, 10:90, and 0:100. This experiment was conducted with three biological replicates. The treatment solutions were renewed every week and continuously aerated. After 11 weeks, wasabi plants were harvested, washed with deionized water at least three times, then wiped dry, and separated into all of leaves, petioles, rhizomes, and roots. The samples were weighed fresh, and then freeze-dried. The freeze-dried samples were ground into a fine powder to measure anion and mineral contents. The samples were stored in a desiccator at room temperature until analysis.
A second hydroponic experiment (2019–2020) investigated the effect of growth medium pH and plant genotype on growth and ionomic profiles. After 1 month in 1/100-strength Hoagland’s solution at pH 7.0, ‘Onimidori’ and ‘Mazuma No.1’ plants were transferred to 1/10-strength Hoagland’s solutions (NH4-N:NO3-N, 50:50) adjusted to pH 5.0, 6.0, 7.0, 8.0, and 9.0. This experiment was conducted with five biological replicates. The treatment solutions were renewed every week and continuously aerated. On sunny days in late March, we measured the photosynthetic rate of five plants per cultivar in the pH 6.0 treatment. After 9 weeks, wasabi plants were harvested and sampled as described above.
2.2. Measurement of chlorophyll content
Chlorophyll a and b were extracted from 5.0 mg freeze-dried powdered leaf samples using 5.0 mL N,N’-dimethylformamide. After incubation for 24 h at 4°C in the dark to allow complete extraction, the samples were centrifuged at 2,000 × g for 30 min. The absorbance of the supernatant was measured at 663.8 and 646.8 nm using a spectrophotometer (UV-1800, Shimadzu). The chlorophyll a and b contents were calculated using the equations of Porra et al. (Citation1989).
2.3. Measurement of photosynthetic rate
The photosynthetic rate (μmol CO2 m−2s−1) of fully expanded leaves was measured using a portable photosynthesis system (Li-6400 XT, Li-Cor, Lincoln, NE, U.S.A.). To analyze the light response curve, we used the ‘Light Curve’ program in Li-6400XT, with the photosynthetically active radiation (PAR) set to 14 levels (0, 100, 200, 300, 400, 500, 600, 800, 1000, 1200, 1400, 1600, 1800, 2000) in decreasing order. The minimum time allowed to read the stabilized rate at each PAR level was 60 s, and the maximum time for saving each reading was 120 s. The other conditions in the leaf chamber were set as follows: flow rate, 500 μmol s−1; CO2 concentration, 350 ppm; temperature, 20°C. The measurements were performed from 9:00 to 12:00.
2.4. Anion analysis
Freeze-dried fine powder (10 mg) of each tissue was added to 10 mg polyvinylpolypyrrolidone and 5 mL ultra-pure water and extracted by shaking (130 strokes per min) for 60 min at room temperature. After centrifugation (2000 × g, 15 min, 4°C), the supernatant was passed through a 0.45-μm cellulose acetate filter (Advantec, Tokyo, Japan). The resulting solution was stored at −30°C until analysis by ion chromatography (IC). The IC system (ICS-2000, Dionex, Sunnyvale, CA, U.S.A.) was equipped with a suppressor (ASRS3000, Dionex), a DS6 heated conductivity cell (Dionex), and an autosampler (AS50, Dionex). The other conditions were as follows: injection volume, 25 μL; guard column, 4 × 50 mm Ion Pac AG17-C (Dionex); analytical column, 4 × 250 mm Ion Pac AS17-C; column oven temperature, 30°C. The mobile phase consisted of eluent A (ultra-pure water) and eluent B (EGC III KOH eluent generator cartridge, Dionex), supplied at a flow rate of 1.0 mL min−1. The gradient was as follows: initial concentration of 5 mM KOH, hold for 1.5 min; then 3.5 min linear gradient from 5 mM to 10 mM KOH, hold for 3 min at 10 mM KOH, 2 min linear gradient from 10 mM to 20 mM KOH, 2 min linear gradient from 20 mM to 40 mM, and final concentration of 40 mM KOH for 10 min (total, 22 min per sample). Anion Mixture Standard Solution 1 (Wako), including nitrate ions, was used as the standard for quantification.
2.5. Free amino acid analysis
The free amino acid content was measured according to the methods described by Goto et al. (Citation1993) and Yamashita et al. (Citation2020a, Citation2020b) The aqueous extract was analyzed by high-performance liquid chromatography (HPLC). Homoserine, as an internal standard, was added to the solution, and o-phthalaldehyde derivatives were analyzed by HPLC (Shimadzu, Japan, Tokyo). The HPLC system and analytical conditions were as described by Yamashita et al. (Citation2020a). Eight amino acids, aspartate (Asp), asparagine (Asn), glutamate (Glu), glutamine (Gln), serine (Ser), arginine (Arg), alanine (Ala), and γ-aminobutyric acid (GABA), were used as standards for quantification.
2.6. Mineral analysis
Mineral analysis was performed as described by Yamashita et al. (Citation2020a). Freeze-dried fine powder (50 mg) of each tissue was digested in 2.0 mL 60% (w/v) HNO3 at 110°C in DigiTUBE® tubes (SCP Science, Quebec, Canada) for approximately 2 h. Once the solution had cooled, 2.0 mL 60% HClO (w/v) was added, and the mixture was heated at 110°C for approximately 2 h. After complete digestion, the solutions were cooled and made up to a volume of 10 mL with ultra-pure water. The concentrations of the following 12 elements were measured based on selected specific wavelengths by inductively coupled plasma optical emission spectrometry (ICP-OES) using an iCAP 7400 instrument (Thermo Fisher Scientific, Waltham, MA, U.S.A.). The specific wavelengths were as follows: P, 213.618 nm; K, 766.490 nm; sulfur (S), 180.731 nm; silicon (Si), 251.611 nm; calcium (Ca), 315.887 nm; magnesium (Mg), 285.213 nm; iron (Fe), 259.940 nm; manganese (Mn), 257.610; nm, zinc (Zn), 206.200 nm; copper (Cu), 324.754 nm; boron (B), 249.773 nm; sodium (Na), 589.592 nm. The measured Si concentration is limited to that obtained by wet digestion, not the total.
2.7. Statistical analyses
Significant differences in each trait among the different ionic strength, inorganic N sources, or pH treatments and between the two cultivars were determined by two-way analysis of variance (ANOVA).
The individual values for each treatment were used for multivariate analysis of the ionome data (12 elements) with normalization by z-score values for each mineral. The hierarchical cluster analysis was based on Ward’s method using Euclidean distance and was conducted using ‘hclust’ function in R. Heatmaps were visualized using ‘heatmap.2’ in the R package ‘gplots’ ver. 3.1.3. The PCA was performed using ‘prcomp,’ and then PC scores and biplots were visualized using ‘ggplot2’ ver. 3.3.2, ‘cowplot’ ver. 1.1.0, ‘factoextra’ ver. 1.0.7, and ‘FactoMineR’ ver. 2.3, all of which are in the R package. Significant differences in ionome profiles of wasabi among the different pH treatments and between the cultivars were determined using permutational multivariate analysis of variance (PERMANOVA), which was performed using the ‘adonis’ function in the R package ‘vegan’ ver. 2.5.6.
Multiple-regression analysis (MRA) was performed using the ‘lm’ function in R. The scaled values of shoot dry weight were used as the response variables. The scaled values of 12 mineral concentrations in leaves, petioles, rhizomes, and roots were used as the explainable variables. Data were from the first hydroponic experiment in this study. The effect of the interaction among the explainable variables was not considered in the modeling. The relationship between each mineral concentrations and the shoot dry weight were constructed by the quadratic polynomial regression.
3. Results and discussion
3.1. Effects of different nutrient strengths and inorganic nitrogen sources on wasabi plant growth
We evaluated the effects of nutrient strength and the ratio of inorganic N sources in the medium on the growth of young wasabi plants in a hydroponic system. The growth of whole plants and all parts was significantly affected by the nutrient strength and inorganic N sources (; two-way ANOVA, P < 0.001). Wasabi plant growth of all parts, shoot and root, was suppressed under a high ratio of NH4-N, regardless of the nutrient strength (). When wasabi plants were exclusively supplied with NH4+, their shoot and root growth were significantly inhibited. However, under hydroponic conditions with the addition of 10% NO3−, their growth was restored (). These findings suggest that moderate amounts of NH4+ contribute to the growth of wasabi plants by improving the efficiency of N assimilation, even though high concentrations of NH4+ (>0.68 mM) have been found to be toxic. Previous studies have shown that many plant species show higher growth rates and yields when supplied with a combination of NH4-N and NO3-N, and their responses are species-dependent (Cao and Tibbitts Citation1993; Gill and Reisenauer Citation1993; Hagin, Olsen, and Shaviv Citation1990; Kim, Mills, and Wetzstein Citation2002; Zhang et al. Citation2007). For most plant species, a combination of NO3− and NH4+ supply has been found to be more beneficial for growth compared to supplying only NO3− or NH4+ separately (Cao and Tibbitts Citation1993; Zhang et al. Citation2007). Adding NO3− to NH4+-dominated media has also been shown to promote plant growth and alleviate the stress caused by ammonia accumulation (Zhang et al. Citation2007). The application of NO3− resulted in an increase in its concentration in various tissues (). The concentrations of chlorophyll and amino acids, which are important metabolites involved in N assimilation, were observed to increase with higher nutrient strength and were higher in treatments with a higher NO3− ratio (, Table S2). These findings indicate that wasabi exhibits a well-functioning N assimilation metabolism even in an environment that favors NO3− over NH4+, leading to superior growth. In the present study, wasabi plants showed the best shoot and root growth when supplied with a 50:50 ratio of NH4-N:NO3-N, regardless of the nutrient strength. These responses are similar to those observed in cabbage (Brassica oleracea L.) (Zhang et al. Citation2007). These findings indicate that wasabi is an ammonium-sensitive and nitrate-preferring plant. In this experiment, the pH of the hydroponic solution was not perfectly controlled. Depending on the growth level of the wasabi plants, the initial pH tended to increase by approximately 1 to 1.5 following the renewal of the hydroponic solution, which occurred 1 week after the renewal. Due to the large-scale nature of the hydroponic experiments, it was challenging to maintain the pH of the hydroponic solution with a stable buffer. However, for a more detailed understanding of nutrient response, further investigation of the pH status will be necessary in future studies.
Figure 1. Effects of nutrient strength and inorganic nitrogen (N) sources on growth of young wasabi plants.
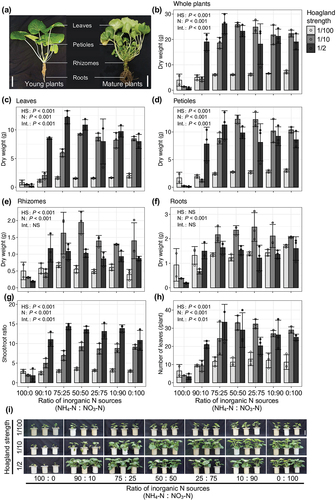
Figure 2. Effects of nutrient strength and inorganic N sources on chlorophyll and nitrate concentrations in young wasabi plants.
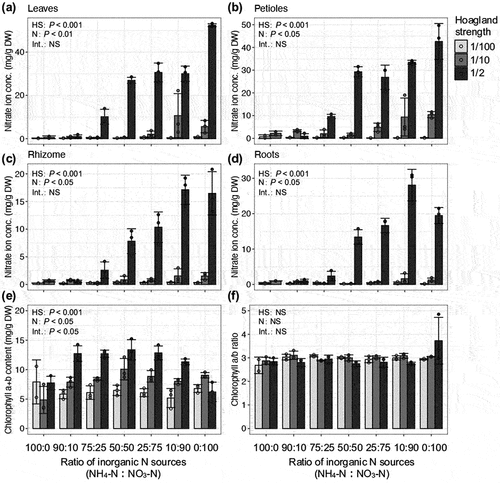
Plants growing in 1/10-strength nutrient medium required at least 25% NO3-N for satisfactory growth (), while those growing in 1/2-strength nutrient conditions required at least 10% NO3-N (). The best shoot growth under 1/2-strength nutrient conditions was in the 75:25 NH4-N:NO3-N treatment (). The best root and rhizome growth under 1/10-strength nutrient conditions was in the 75:25 and 50:50 NH4-N:NO3-N treatments (). The shoot-to-root ratio was strongly affected by the nutrient strength of the medium (). These results indicated that wasabi plants grew well enough under 1/10-strength Hoagland solution. In previous studies, Arabidopsis and onion were found to grow best in 1/2-strength Hoagland solution (Kane et al. Citation2006; van Delden, Nazarideljou, and Marcelis Citation2020). These results suggest that wasabi plants have relatively low nutrient requirements for optimal growth. This was supported by the accumulation patterns of minerals, NO3−, amino acids, and chlorophyll in plants grown in 1/10- and 1/2-strength Hoagland solution (, Table S2, S3).
3.2. Effects of rhizosphere pH on growth of two wasabi cultivars
We evaluated the effects of rhizosphere pH on the growth of two main types of wasabi cultivars, ‘Onimidori’ (an early-growing green-stem type) and ‘Mazuma No.1’ (a late-growing red-stem type). Both cultivars showed the best shoot and root growth at pH 6.0 () and also showed the highest chlorophyll concentrations at pH 6.0, whereas its concentrations decreased as pH increased from 6.0 to 9.0 (). Kurita et al. (Citation1953) reported that the soil pH of flooded wasabi fields was in the range of 6.0 to 7.0, while Adachi (Citation1987) reported a pH range of 6.0 to 7.5 for the irrigation water used in wasabi cultivation. The results of our hydroponic experiments confirm that the pH conditions of flooded wasabi fields are appropriate for wasabi plant growth.
Figure 3. Effects of rhizosphere pH on growth of two wasabi cultivars, ‘Onimidori’ and ‘Mazuma No.1’.
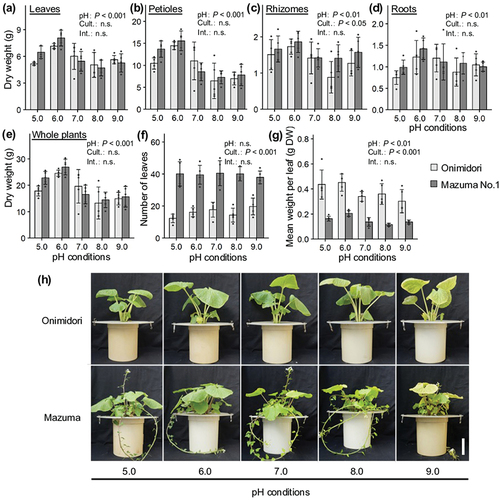
Figure 4. Effects of rhizosphere pH on chlorophyll concentrations and photosynthetic capacity of two wasabi cultivars, ‘Onimidori’ and ‘Mazuma No.1’.
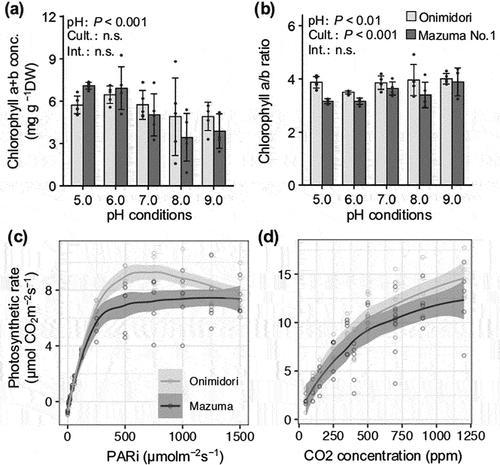
The number of leaves per plant was higher in ‘Mazuma No.1’ than in ‘Onimidori’ (), but the weight per leaf was higher in ‘Onimidori’ than in ‘Mazuma No.1’ (). These data reflect the characteristics of early- and late-growing wasabi cultivars. Analyses of photosynthetic characteristics revealed that the light and CO2 response curves of photosynthesis were higher in ‘Onimidori’ than in ‘Mazuma No.1’ (, d). These results suggest that a higher photosynthetic capacity may contribute to the faster growth of some of the main types of wasabi cultivars.
3.3. Effects of rhizosphere pH on ionome profiles of two wasabi cultivars
To characterize the mineral accumulation patterns in wasabi plants, we analyzed the ionome profiles (12 elements) of each tissue by ICP-OES. First, we compared the tissue-specific ionome profiles of materials in the pH 6.0 treatment, in which the wasabi plants showed optimal growth. The mineral accumulation patterns were similar among tissues in the two wasabi cultivars (). Many elements accumulated in the leaves or roots, and not in the rhizomes or petioles (). The contents of K, Ca, Mg, and B were higher in leaves than in roots, and the contents of P and most of the minor elements were higher in roots than in leaves (). The S contents were higher in leaves and roots than in rhizomes and petioles, showing a different accumulation pattern from that of the other major elements. The S content in leaves was higher in ‘Onimidori’ than in ‘Mazuma No.1,’ but the S content in rhizomes was higher in ‘Mazuma No.1’ than in ‘Onimidori’ (Table S3). These may be due to cultivar characteristics, because ‘Onimidori’ is an early-growing rhizome type that produces fewer leaves than ‘Mazuma No.1’ (, g). Members of the order Brassicales are characterized by relatively high contents of Ca (20.8 ± 7.7 mg g−1 DW) and S (11.3 ± 4.1 mg g−1 DW) in the leaves (Neugebauer et al. Citation2018). Compared with other members of the order Brassicales, wasabi showed lower concentrations of Ca in the leaves (‘Onimidori,’ 9.7 ± 1.4 mg g−1 DW; ‘Mazuma No.1,’ 8.5 ± 1.1 mg g−1 DW) and similar concentrations of S in the leaves (‘Onimidori,’ 8.0 ± 1.0 mg g−1 DW; ‘Mazuma No.1,’ 7.2 ± 2.1 mg g−1 DW) (, Table S3). The ability of species in the Brassicales to accumulate S has been attributed to their unique ability to synthesize glucosinolates (Ernst Citation1998). Like other members of the Brassicales, wasabi accumulates S to high levels (, Table S3). Further research is required to investigate the effects of these nutritional factors and genotype on the contents of glucosinolates and isothiocyanate in wasabi. This study is the first to report the ionome profiles of a member of the genus Eutrema. This information will be useful for understanding the diversity of ionome profiles within the Brassicales.
Figure 5. Mineral accumulation patterns in each tissue of young wasabi plants under optimal pH conditions (pH 6.0).
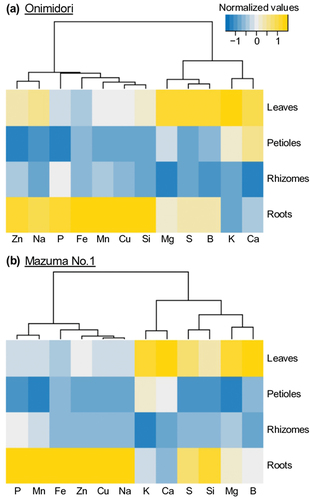
We performed PCA to determine the effects of different pH conditions and genotype on ionome profiles. The PCA indicated that ionome profiles in all tissues were affected by both the pH of the medium and the plant genotype (; PerMANOVA, P < 0.01 or P < 0.05). The largest differences in ionome profiles were in the rhizomes (), the main edible part. For the rhizome, the first PC score (PC1) separated samples from different pH treatments and the second PC score (PC2) separated samples based on genotype (). The S, K, and Na contents were higher in ‘Onimidori’ than in ‘Mazuma No. 1,’ and the contents of other minerals were affected by the pH of the medium (, Table S3). A previous study indicated that the allyl isothiocyanate content in rhizomes was higher in ‘Mazuma No.1’ (a slow-growing type) than in two early-growing types (Arakawa et al. Citation2001). Further research is required to clarify the relationship between these mineral profiles, including the high S contents in the early-growing cultivars and quality-related metabolites in wasabi.
Figure 6. PCA of ionome profiles of wasabi cultivars ‘Onimidori’ and ‘Mazuma No.1’ cultivated under different pH conditions.
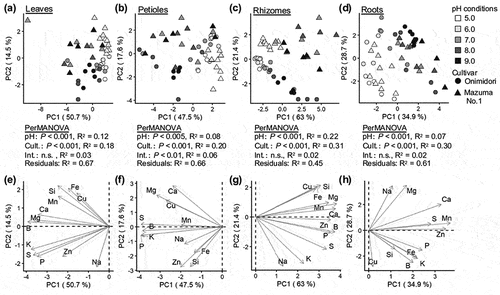
3.4. Relationship between tissue mineral concentration and shoot growth
To determine the optimal nutrient status for wasabi plants, we conducted regression analysis to assess the relationship between tissue mineral concentrations and shoot growth. In this study, we utilized data from the first hydroponic experiments using the ‘Onimidori’ cultivar. Multiple-regression analysis (MRA) was performed to reveal the connection between mineral profiles in each tissue and shoot growth. The model based on root minerals showed the highest coefficient of determination (R2 = 0.919) among all tissues; followed by models based on petiole (R2 = 0.888), rhizome (R2 = 0.785), and leaf (R2 = 0.651) minerals (). This finding suggests that the mineral concentration profiles in roots and petioles provide a well reflection of shoot growth in wasabi plants. Some minerals, particularly S, showed high values of standardized partial regression coefficient in leaf, petiole, and root models (). Furthermore, we visualized the relationship between individual mineral concentrations and shoot growth using quadratic polynomial regression ( and S2–4). In the case of root P, Fe, B, Cu, and Mn concentrations, a decrease in shoot growth was observed at higher concentrations (). In leaves, petioles, and root, shoot growth was higher as S concentrations increased ( and S2, 3). However, the relationship between shoot growth and the concentrations of other elements was not consistent (, S2–4). These results suggest that evaluating the nutritional status of wasabi plants based on a single mineral concentration is challenging, as each element interacts with others, and it is crucial to consider the overall mineral profiles. Miyamoto-Maeta et al., (Citation2021) reported that the ionomic profiles in rice leaves could predict growth stages, and our results indicate that ionomic profiles may also serve as an estimate of growth conditions in wasabi plants. However, it is important to note that we have not observed any toxic symptoms associated with high concentrations of most minerals, suggesting that there is room for further optimization of the nutritional conditions to achieve superior growth in wasabi plants.
Figure 7. Relationship between tissue mineral concentrations and shoot dry weight in wasabi plants under hydroponics.
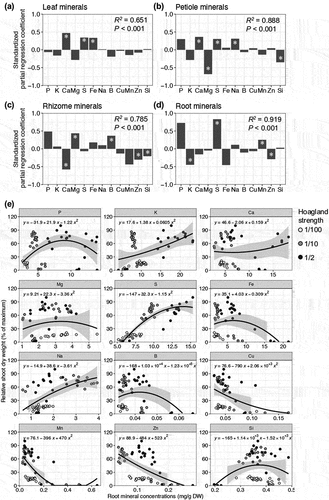
4. Conclusion
This study is the first to reveal how various medium conditions (inorganic N source, nutrient strength, and pH) affect the growth of wasabi plants. Our results show that wasabi is an ammonium-sensitive and nitrate-preferring plant, like most other species in the Brassicales. We also detected differences in mineral accumulation patterns and photosynthetic abilities between two main Japanese wasabi cultivars, one early-growing green-stem type (‘Onimidori’) and the other a late-growing red-stem type (‘Mazuma No.1’). These differences may explain the differences in growth rate among the main types of wasabi cultivars. Ionome-based multiple-regression analysis revealed a relationship between element concentration profiles in the wasabi plants, especially those in root, and the shoot growth. The results of the present study will be useful for optimizing fertilizer management in the traditional flooded system to improve wasabi quality.
Supplemental Material
Download Zip (780.5 KB)Acknowledgments
We thank Jennifer Smith, PhD, from Edanz (https://jp.edanz.com/ac) for editing a draft of this manuscript.
Disclosure statement
No potential conflict of interest was reported by the author(s).
Supplementary material
Supplemental data for this article can be accessed online at https://doi.org/10.1080/00380768.2023.2276716
Additional information
Funding
References
- Adachi, S. 1987. Wasabi Cultivation. Wasabi Cultivation. Shujunsha.
- Arakawa, H., T. Ina, H. Matuura, S. Oba, M. Taneishi, and T. Nakane. 2001. “Content and Distribution of Pungent Components in Several Cultivars and Lines of Wasabi (Wasabia Japonica Matsum.).” Bulletin of Shizuoka Agricultural Experiment Station (46):35–43.
- Baxter, I. R., O. Vitek, B. Lahner, B. Muthukumar, M. Borghi, J. Morrissey, M. L. Guerinot, and D. E. Salt. 2008. “The Leaf Ionome as a Multivariable System to Detect a Plant’s Physiological Status.” Proceedings of the National Academy of Sciences 105 (33): 12081–12086. https://doi.org/10.1073/pnas.0804175105.
- Bloom, A. J., S. S. Sukrapanna, and R. L. Warner. 1992. “Root Respiration Associated with Ammonium and Nitrate Absorption and Assimilation by Barley.” Plant Physiology 99 (4): 1294–1301. https://doi.org/10.1104/pp.99.4.1294.
- Britto, D. T., and H. J. Kronzucker. 2002. “NH4+ Toxicity in Higher Plants: A Critical Review.” Journal of Plant Physiology 159 (6): 567–584. https://doi.org/10.1078/0176-1617-0774.
- Cao, W., and T. W. Tibbitts. 1993. “Study of Various NH 4 + /NO 3 − Mixtures for Enhancing Growth of Potatoes.” Journal of Plant Nutrition 16 (9): 1691–1704. https://doi.org/10.1080/01904169309364643.
- Chadwick, C. I., T. A. Lumpkin, and L. R. Elberson. 1993. “The Botany, Uses and Production ofWasabia Japonica (Miq.) (Cruciferae) Matsum.” Economic Botany 47 (2): 113–135. https://doi.org/10.1007/BF02862015.
- Ernst, W. H. 1998. “Sulfur Metabolism in Higher Plants: Potential for Phytoremediation.” Biodegradation 9 (3/4): 311–318. https://doi.org/10.1023/a:1008250827209.
- Findenegg, G. R. 1987. “A Comparative Study of Ammonium Toxicity at Different Constatn pH of the Nutrient Solution.” Plant and Soil 103 (2): 239–243. https://doi.org/10.1007/BF02370395.
- Gałczyńska, A., P. Trzcinska, M. Gumienna, J. Nowak, and R. Hołubowicz. 2017. “Production of Japanese Horseradish (Wasabia Japonica (Miq.) Matsumara) in Poland. Chemical Contents of Roots.” Notulae Botanicae Horti Agrobotanici Cluj-Napoca 45 (2): 466–472. (November, 2022). https://www.notulaebotanicae.ro/index.php/nbha/article/view/10887.
- Gill, M. A., and H. M. Reisenauer. 1993. “Nature and Characterization of Ammonium Effects on Wheat and Tomato.” Agronomy Journal 85 (4): 874–879. https://doi.org/10.2134/agronj1993.00021962008500040018x.
- Goto, T., H. Horie, and T. Mukai. 1993. “Analysis of Major Amino Acids in Green Tea by High-Performance Liquid Chromatography Coupled with OPA Precolumn Derivatization.” Tea Research Journal 1993:29–33. https://doi.org/10.5979/cha.1993.29.
- Hagin, J., S. R. Olsen, and A. Shaviv. 1990. “Review of Interaction of Ammonium ‐ Nitrate and Potassium Nutrition of Crops.” Journal of Plant Nutrition 13 (10): 1211–1226. https://doi.org/10.1080/01904169009364147.
- Kane, C. D., R. L. Jasoni, E. P. Peffley, L. D. Thompson, C. J. Green, P. Pare, and D. Tissue. 2006. “Nutrient Solution and Solution pH influences on Onion Growth and Mineral Content.” Journal of Plant Nutrition 29 (2): 375–390. https://doi.org/10.1080/01904160500477028.
- Kim, T., H. A. Mills, and H. Y. Wetzstein. 2002. “STUDIES ON EFFECTS OF NITROGEN FORM ON GROWTH, DEVELOPMENT, AND NUTRIENT UPTAKE IN PECAN.” Journal of Plant Nutrition 25 (3): 497–508. https://doi.org/10.1081/PLN-120003378.
- Kurita, K., M. Suzuki, and A. Ando. 1953. “Relation Between the Growth of the Horse Radish (Eutrema Wasabi) and Properties of Its Bed Soils.” Journal of the Japanese Forestry Society 35:14–16. https://doi.org/10.11519/jjfs1953.35.1_14.
- Miyamoto-Maeta, M., T. Kamiya, T. Fujiwara, D. Hirotomi, and H. Iwata. 2021. “Time‐Course Changes in the Ionomic Profiles of Rice Leaves and Their Application in Growth Stage Prediction.” Crop Science 61 (6): 4239–4254. https://doi.org/10.1002/csc2.20593.
- Neugebauer, K., M. R. Broadley, H. A. El-Serehy, T. S. George, J. W. McNicol, M. F. Moraes, and P. J. White. 2018. “Variation in the Angiosperm Ionome.” Physiologia plantarum 163 (3): 306–322. https://doi.org/10.1111/ppl.12700.
- Porra, R. J., W. A. Thompson, and P. E. Kriedemann. 1989. “Determination of Accurate Extinction Coefficients and Simultaneous Equations for Assaying Chlorophylls a and B Extracted with Four Different Solvents: Verification of the Concentration of Chlorophyll Standards by Atomic Absorption Spectroscopy.” Biochimica et biophysica acta 975 (3): 384–394. https://doi.org/10.1016/S0005-2728(89)80347-0.
- Raven, J. A., B. Wollenweber, and L. L. Handley. 1992. “A Comparison of Ammonium and Nitrate as Nitrogen Sources for Photolithotrophs.” The New Phytologist 121 (1): 19–32. https://doi.org/10.1111/j.1469-8137.1992.tb01088.x.
- Rideout, J. W., S. Chaillou, C. D. Raper Jr, and J.-F. Morot-Gaudry. 1994. “Ammonium and Nitrate Uptake by Soybean During Recovery from Nitrogen Deprivation.” Journal of Experimental Botany 45 (1): 23–33. https://doi.org/10.1093/jxb/45.1.23.
- Salt, D. E., I. Baxter, and B. Lahner. 2008. “Ionomics and the Study of the Plant Ionome.” Annual Review of Plant Biology 59 (1): 709–733. https://doi.org/10.1146/annurev.arplant.59.032607.092942.
- Sultana, T., and G. P. Savage. 2008. “Wasabi - Japanese Horseradish.” Bangladesh Journal of Scientific & Industrial Research 43 (4): 433–448. https://doi.org/10.3329/bjsir.v43i4.2234.
- Tanaka, I., Y. Ito, M. Shinozuka, and T. Shimazu. 2009. “Indoor Cultivation of Japanese Horseradish (Wasabia Japonica Matsum.) Using Artificial Light -Effects of Air and Solution Temperatures on Plant Growth-.” Journal of Society of High Technology in Agriculture 21:175–178. https://doi.org/10.2525/shita.21.175.
- van Delden, S. H., M. J. Nazarideljou, and L. F. M. Marcelis. 2020. “Nutrient Solutions for Arabidopsis Thaliana: A Study on Nutrient Solution Composition in Hydroponics Systems.” Plant Methods 16 (1): 72. https://doi.org/10.1186/s13007-020-00606-4.
- Watanabe, T., R. Okada, and M. Urayama. 2022. “Differences in Ionomic Responses to Nutrient Deficiencies Among Plant Species Under Field Conditions.” Journal of Plant Nutrition 45 (10): 1493–1503. https://doi.org/10.1080/01904167.2021.2020837.
- Watanabe, T., M. Urayama, T. Shinano, R. Okada, and M. Osaki. 2015. “Application of Ionomics to Plant and Soil in Fields Under Long-Term Fertilizer Trials.” Springerplus 4 (1): 781. https://doi.org/10.1186/s40064-015-1562-x.
- Yamashita, H., Y. Fukuda, S. Yonezawa, A. Morita, and T. Ikka. 2020a. “Tissue ionome response to rhizosphere pH and aluminum in tea plants (Camellia sinensis L.), a species adapted to acidic soils.” Plant-Environment Interactions 1 (2): 152–164. https://onlinelibrary.wiley.com/doi/10.1002/pei3.10028.
- Yamashita, H., T. Uchida, Y. Tanaka, H. Katai, A. J. Nagano, A. Morita, and T. Ikka. 2020b. “Genomic Predictions and Genome-Wide Association Studies Based on RAD-Seq of Quality-Related Metabolites for the Genomics-Assisted Breeding of Tea Plants.” Scientific Reports 10 (1): 17480. https://doi.org/10.1038/s41598-020-74623-7.
- Zhang, F.-C., S.-Z. Kang, F.-S. Li, and J.-H. Zhang. 2007. “Growth and Major Nutrient Concentrations in Brassica Campestris Supplied with Different NH 4 + /NO 3 ? Ratios.” Journal of Integrative Plant Biology 49 (4): 455–462. https://onlinelibrary.wiley.com/doi/10.1111/j.1744-7909.2007.00373.x.