ABSTRACT
Agriculture is a significant anthropogenic source of methane (CH4) and nitrous oxide (N2O). This review provides an overview of the emissions of the two greenhouse gases (GHGs) from agricultural fields and mitigation options based on previously published field studies mainly conducted in Japan. Rice paddy fields in Japan emitted 11,982 kt CO2eq of CH4, accounting for 43.6% of the total CH4 anthropogenic emissions in 2021. Global meta-analysis shows that mid-season drainage (MD) is more effective in reducing CH4 emissions from rice paddy fields than continuous flooding. In the regions where MD is already practiced, prolonged MD (a week longer than usual MD) has been proposed to further reduce CH4 emissions compared to conventional MD. A field survey conducted at nine sites across Japan showed that prolonging MD reduced CH4 emissions and global warming potential (CH4 + N2O) by 30% and 28%, respectively. Moreover, in Japan, the autumn incorporation of rice straw was shown to reduce annual CH4 emissions by 54% on average compared with spring incorporation. Subsurface drainage and the application of iron-containing materials have been shown to reduce CH4 emissions from paddy fields, although further field studies testing various conditions are needed. In addition to CH4 emissions from rice paddy fields, agricultural soil in Japan in 2021 emitted 5962 kt CO2eq of N2O, accounting for 28.9% of the total anthropogenic N2O emissions. Meta-analyses indicated that nitrification inhibitors and polymer-coated fertilizers were effective in reducing N2O emissions after the application of synthetic N fertilizers. Moreover, biochar application was shown to be effective in sequestering soil carbon and has also been investigated as a mitigation option to reduce CH4 and N2O emissions; however, the results were inconclusive. The Ministry of Agriculture, Forestry, and Fisheries is now promoting prolonged MD and autumn incorporation of rice straw through a direct payment program to reduce CH4 emissions from paddy fields in Japan. In addition, prolonged MD and biochar application have been approved as J-Credit methodologies to reduce CH4 emissions from paddy fields and sequester soil carbon, respectively. Further studies are warranted to develop practical mitigation strategies to reduce GHG emissions while maintaining productivity.
KEY WORDS:
1. Introduction
Agriculture is a significant source of two important greenhouse gases (GHGs): methane (CH4) and nitrous oxide (N2O). Specifically, agriculture account for 39% of CH4 and 78% of N2O emissions, equivalent to 12% (6.2 ± 3.4 GtCO2 equivalent yr−1) of global anthropogenic GHG emissions (Jia et al., Citation2019). CH4 is a short-lived GHG with a lifetime of 11.8 years, and a global warming potential (GWP) of over 100 years of CH4 is 27 times that of carbon dioxide (CO2) (Forster et al. Citation2021). The atmospheric concentrations of CH4 have increased from 729 ppb in 1750 to 1866 ppb in 2019 (Forster et al. Citation2021). The Global Methane Pledge signed by over 100 countries at the COP26 climate summit in 2021 agreed to reduce anthropogenic CH4 emissions by 30% in 2030, stating that this is the most effective strategy to achieve the Paris Agreement’s 1.5°C temperature goal due to the short lifetime of CH4 in the atmosphere (Global Methane Pledge, 2023). Paddy rice fields are a significant source of CH4, accounting for 9% of the global anthropogenic CH4 emissions (Canadell et al. Citation2021). In contrast to CH4, N2O is a long-lived GHG with a lifetime of 109 years; it has a GWP of 273 times that of CO2 and also contributes to the depletion of the ozone layer in the stratosphere (Forster et al. Citation2021). The concentrations of N2O have increased from 270 ppb in 1750 to 332 ppb in 2019 (Forster et al. Citation2021). Agriculture is the largest source of anthropogenic N2O, accounting for 51% of the global anthropogenic N2O emissions (Canadell et al. Citation2021). This review provides an overview of CH4 and N2O emissions from agricultural fields and potential mitigation options based on field studies mainly conducted in Japan.
2. The GHG inventory of Japan
Agriculture is a significant source of anthropogenic GHGs globally. However, according to the National Greenhouse Gas Inventory Report (NIR) of Japan, the agricultural sector accounted for only 2.7% (excluding fuel combustion in agriculture, forestry, and fisheries) and 4.1% (including fuel combustion in agriculture, forestry, and fisheries) of the total GHG emissions in 2021 (GIO and MOE, 2023; ) because CO2 emissions from industries and transport represent the majority of GHG sources in Japan. In addition to the GHG emissions reported in the NIR, Japan imported 62% of food and feed in 2021 on a calorie basis (Ministry of Agriculture, Forestry, and Fisheries (MAFF), 2023a); however, the GHG emissions emitted during their production in the countries of origin were not included in the NIR. While being a relatively minor source of total GHG including CO2, agriculture is the largest source of CH4 and N2O in Japan. The agricultural sector accounted for 81.1% of the total CH4 emissions in 2021 (GIO and MOE, 2023; ), and the sources of CH4 are enteric fermentation in ruminant animals, manure management, rice cultivation, and field burning of agricultural residues. The agricultural sector accounted for 49.1% of the total N2O emissions in 2021 (GIO and MOE, 2023; ), and the sources of N2O include agricultural soil and manure management. In 2021, rice paddy fields emitted 11,982 kt CO2eq of CH4, accounting for 43.6% of the total CH4 emissions, and agricultural soil emitted 5,962 kt CO2eq of N2O, accounting for 28.9% of the total N2O emissions in Japan.
Figure 1. Anthropogenic greenhouse gas emissions (kt CO2eq) from the agricultural sector in Japan in 2021.
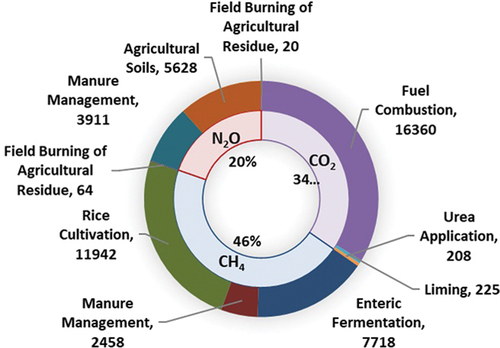
Figure 2. Anthropogenic CH4 (a) and N2O (b) emissions in Japan in 2021.
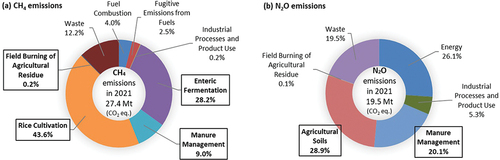
The methods used to compile GHG inventories for the NIR must follow the Intergovernmental Panel on Climate Change Guidelines for National Greenhouse Gas Inventories (IPCC GLs). In the guidelines, Tier 1 is the basic method to estimate GHG emissions at the national scale using default emission factors (EFs) and a common set of activity data. Countries can also adopt Tier 2 (country-specific EFs) or Tier 3 (model-based approach) methods to improve accuracy and reduce the overall uncertainty when adequate data are available. In addition, continuous efforts are needed to update the national inventory system to maintain a record of unbiased estimates with the highest possible accuracy for the creation of government policies aimed at reducing GHG emissions (Ogle et al., Citation2020).
The 2019 IPCC GLs (Ogle et al. Citation2019) provided a default method for calculating CH4 emissions from paddy rice fields based on global statistical analysis (Wang et al. Citation2018). In these guidelines, the default EFs to estimate direct N2O emissions from synthetic and organic nitrogen (N) fertilizer applications, return of crop residues to soils, and decomposition of soil organic matter of mineral soils (EF1) were revised as an aggregated EF of 1% and disaggregated EFs of 1.6% for synthetic and mixed fertilizer inputs (synthetic and organic) in wet climates, 0.6% for other N inputs (i.e., organic amendments, animal manures, N in crop residues, and mineralized N from soil organic matter decomposition) in wet climates, and 0.5% for all N inputs in dry climates (Hergoualc’h et al. Citation2021; Hergoualc’h et al. Citation2019).
In the NIR of Japan, CH4 emissions from rice paddy fields were estimated using EFs calculated using the Tier 3 method, i.e., through the DeNitrification-DeComposition-Rice model (DNDC-Rice model; Fumoto et al. Citation2010; Katayanagi et al. Citation2017). In addition, the report also accounted for a CH4 reduction rate of 30% associated with prolonged mid-season drainage (MD) (Itoh et al. Citation2011), which is described in section 3.2.2.
In the NIR of Japan (GIO and MOE Citation2023), N2O emissions from synthetic N fertilizers were estimated using the Tier 2 method, i.e., country-specific EFs for synthetic N fertilizers (0.31% for rice paddy, 2.9% for tea, and 0.62% for other crops) were used based on mean N2O EFs for synthetic N fertilizers in Japanese agricultural fields (Akiyama, Yan, and Yagi Citation2006). In addition, the NIR included a 38% reduction rate in N2O for nitrification inhibitors (Akiyama, Yan, and Yagi Citation2010).
The EF for other crops (0.62%) were lower than the IPCC default value of 1.6% for wet climate, although Japan is included in wet climate. This would be mainly because N2O emissions from Andosols are generally lower than those from non-Andosols (Akiyama, Yan, and Yagi Citation2006). Japan is part of the Ring of Fire and, consequently, volcanic tephra significantly affects its soils. Fluvisols occupy 47%, followed by Andosols (29%) of cultivated lands, whereas the former are mainly used as paddy rice fields while the latter are used as upland fields; consequently, andosols occupy 47% of upland fields (NARO, Citation2023).
The total amount of N in organic amendments (444 ktN) used in Japan in 2021 was larger than that of synthetic N fertilizers (375 ktN) (GIO and MOE Citation2023). However, the current NIR (GIO and MOE Citation2023) assumed that the EF for organic amendments was the same as that for synthetic N fertilizers because country-specific EFs for organic amendments were not available. Therefore, a field survey across Japan and a systematic review were conducted to estimate the country-specific N2O EFs for organic amendments, and the results showed that the mean EFs for poultry manure compost, swine manure compost, cattle manure (compost and slurry), and organic fertilizers derived from non-animal manure were 0.83%, 0.70%, 0.39%, and 1.16%, respectively. The mean EF for all organic amendments was 0.84% (Akiyama et al. Citation2023).
3. CH4 emissions from paddy rice fields and mitigation options
Rice is an important staple food in Japan as well as in other Asian countries. In 2021, the rice cultivation area in Japan was 1549 kha, accounting for 36% of the total cultivated area (3922 kha) (GIO and MOE Citation2023). Rice paddy cultivation is a significant source of CH4 (Canadell et al. Citation2021), while N2O emissions from paddy fields are generally lower than those from upland fields (Akiyama, Yan, and Yagi Citation2005). Due to CH4 emissions, the GWP of rice cultivation is the highest among cereals; for example, it is 467% and 169% higher than that of wheat and maize, respectively (Linquist et al. Citation2012). Because rice paddy fields are the largest source of CH4 emissions in Japan (), they are the primary target for reducing GHG emissions derived from the agricultural sector.
3.1. CH4 production and oxidation in soil and transportation to the atmosphere
In paddy rice fields, CH4 is generated by CH4-producing archaea (methanogens) during the anaerobic decomposition of organic matter (such as crop residue) and rhizodeposition (Conrad Citation2020a, Citation2020b; Kimura, Murase, and Lu Citation2004). Methanogenesis is the final step in the anaerobic degradation of organic matter, and occurs only after inorganic electron acceptors, such as nitrate, ferric iron, or sulfate, have been depleted (Conrad Citation2020a, Citation2020b). Part of the produced CH4 is oxidized by CH4-oxidizing bacteria (methanotrophs) (Conrad Citation2020b; Hayashi et al. Citation2015). The accumulated CH4 in paddy soils is transported to the atmosphere mainly through the aerenchyma tissues of rice plants and the ebullition of gas bubbles, while the contribution of diffusion is considered marginal (Kajiura and Tokida Citation2021). More than 90% of CH4 is emitted through rice plants in paddy fields (Schutz, Seiler, and Conrad Citation1989). However, a recent field study showed that ebullition contributed to only 4% of the total CH4 emissions at the panicle formation stage, whereas it accounted for 32% and 60% of the total emissions at the booting and heading stages, respectively (Kajiura and Tokida Citation2021).
3.2. Mitigation options for CH4 emissions from rice paddy fields
Water and straw management are basic and low-cost strategies to mitigate CH4 emissions derived from paddy rice fields. Wang et al. (Citation2023) estimated that improved water and straw management could reduce global CH4 emissions from rice paddy fields (27 Tg CH4 year−1) by one-third (8.8 Tg CH4 year−1).
3.2.1. Water management: MD and multiple drainages
Water management largely influences CH4 emissions from paddy rice fields because CH4 production requires anaerobic conditions. MD, which is the traditional practice of draining water for about a week during the growing season to enhance grain yield, is common in Japan, China, and other Asian countries. Intermittent irrigation is also a traditional practice in which drainage and irrigation are repeated within a cycle of a few days during the growing season and is commonly practiced in Japan, China, India, and other Asian countries. According to a worldwide statistical analysis by Wang et al. (Citation2018), CH4 emissions from single MD and multiple drainages, such as intermittent irrigation and alternate wetting and drying (AWD), were lower by 29% and 46%, respectively, compared to those of continuous flooding. Intermittent irrigation consisting, for example, of 3-day flooding and 4-day drainage cycles after MD is widely practiced in Japan, while AWD is uncommon.
3.2.2. Water management: prolonged MD
According to a worldwide statistical analysis by Wang et al. (Citation2018), CH4 emissions from MD were lower by 29% compared to those from continuous flooding. In Japan, all paddy fields are irrigated, and MD is already practiced in about 90% of them, except for those in the Hokkaido region. Thus, introducing MD or intermittent irrigation is the applicable mitigation measure only in Hokkaido. In this region, continuous flooding is practiced in 52% of paddy fields (GIO and MOE Citation2023) to prevent potential reductions in rice grain yield due to cold weather. However, a recent study suggested that, even in this cold region, intermittent irrigation reduced CH4 emissions by 21–91% compared to continuous flooding, without any increase in N2O emissions or decrease in rice grain yields (Nishimura et al. Citation2020).
To further reduce CH4 emissions in the regions where MD is already adopted, prolonged MD (1 week longer than the local MD practice) was proposed instead. Based on the results of a field survey conducted in nine sites across Japan for 2 years, prolonging MD reduced CH4 emissions and GWPs (CH4 + N2O) by 30% and 28%, respectively (Itoh et al. Citation2011). Since 2015, the MAFF has been promoting prolonged MD as part of a direct payment program ‘the Direct Payments for Environmentally Friendly Agriculture’ to reduce GHG emissions (MAFF, Citation2023b). As of 2021, prolonged MD is practiced in 15,000 ha as part of the above-mentioned direct payment program (MAFF, Citation2023b). However, according to a survey by MAFF, 25.9% of rice farmers had already been adopting this strategy (MAFF, Citation2022), suggesting that the overall area subjected to prolonged MD would be much larger than 15,000 ha. In addition, prolonged MD was approved as a J-Credit methodology in 2022 (METI Citation2023). J-Credit is a carbon credit scheme certified by the Japanese government.
3.2.3. Water management: improvement of drainage by installing subsurface drainage
Katayanagi et al. (Citation2017) reported that field drainage is an important factor along with organic C application rate and water management regime to estimate CH4 emissions from paddy fields using the DNDC-Rice model. Accordingly, these factors have been used to run the model, whose results have been included in the NIR of Japan (GIO and MOE Citation2023)).
Currently, subsurface drainage systems (tile drainage systems) are installed in more than 60% of the paddy fields in Japan to improve farming efficiency and increase rice productivity (Shiratori et al. Citation2007). In a Gray lowland soil at Niigata, it was shown that CH4 emissions from a paddy field with subsurface drainage were 70% lower than those from an adjacent paddy field with no drainage system, although with large interannual variations (Shiratori et al. Citation2007). Although the improvement of drainage has a great potential to reduce CH4 emissions and increase productivity, it has not been widely tested so far, and further studies are needed for a wide variety of soil types and climates.
3.2.4. Organic matter management
According to a worldwide statistical analysis by Wang et al. (Citation2018), at a typical rate of 6 t ha−1, the application of rice straw off-season, known as autumn incorporation of rice straw in temperate regions such as Japan (rice straw from a previous season is incorporated into the soil long before cultivation so that it decomposes under aerobic conditions), reduced CH4 emissions by 50% compared to the application of rice straw on-season, known as spring incorporation in temperate regions (rice straw application just before cultivation).
Similarly, a statistical analysis by Kajiura et al. (Citation2018) showed that the autumn incorporation of rice straw reduced CH4 emission by 54% on average compared with spring incorporation. Takakai et al. (Citation2021) also reported that autumn incorporation reduced CH4 emissions and GWP by 21–37% compared to spring incorporation in the Tohoku region. The autumn incorporation of rice straw has been approved as a component of ‘the Direct Payments for Environmentally Friendly Agriculture’ in 2020 and, as of 2021, it is practiced over an area of 884 ha (MAFF, Citation2023b). However, this area is estimated to be larger because a MAFF survey revealed that 59.5% of rice farmers had already been practicing autumn incorporation in Japan (MAFF, 2022).
Especially in cold regions, such as Hokkaido, Tohoku, and Hokuriku, the slow rate of rice straw decomposition during winter leads to an increase in CH4 emissions. Therefore, accelerating straw decomposition during winter could mitigate CH4 emissions. To explore this strategy, Takakai et al. (Citation2021) tested the application of lime nitrogen in a field in the Tohoku region, but the effects on straw decomposition and CH4 emissions were not observed. Additionally, in the same region, Nakajima et al. (Nakajima et al. Citation2017) tested shallow autumn tillage to incorporate straw and accelerate decomposition, but CH4 emissions were not reduced, probably due to a small difference in soil water content between shallow autumn tillage treatment and conventional autumn tillage treatment. The study concluded that, in winter, moderate soil water content is more important for rice straw decomposition than tillage depth.
3.2.5. Other mitigation options to reduce CH4 emissions
Studies have shown that the application of iron-containing materials, such as steel slag, was effective in reducing CH4 emissions in pot experiments (Furukawa and Inubushi Citation2004; Singla and Inubushi Citation2015). Field experiments investigating the effect of iron-containing materials on CH4 emissions have been conducted in China and Indonesia (Susilawati et al. Citation2015; Zhang et al. Citation2023), and further field studies are needed under various conditions.
It is known that CH4 emissions differ depending on rice cultivars (Gupta et al. Citation2021; Hayashi et al. Citation2015). Identifying or breeding low-CH4 rice varieties can be an effective mitigation strategy in the future (Chirinda et al. Citation2018; Hayashi et al. Citation2015; Jiménez and Pedersen Citation2023; Wang et al. Citation2023).
4. N2O emissions from agricultural fields and mitigation options
4.1. N2O production in agricultural soils
In agricultural soils, N2O is emitted as a result to soil management practices, such as the application of synthetic N fertilizers and organic N, crop residue N inputs, and the enhanced mineralization of N of soil organic matter caused by continuous land cultivation or land use change. The application of synthetic or organic N stimulates N2O production predominantly via the microbial processes of nitrification and denitrification (Hayatsu, Katsuyama, and Tago Citation2021; Hayatsu, Tago, and Saito Citation2008). The nonbiochemical process of chemodenitrification also produces N2O; however, its contribution would be minor in cropland (Hayashi et al. Citation2015). The N application rate and climate type (wet or dry, affecting soil moisture) are major factors influencing N2O emissions, followed by land cover, soil texture, C content, and pH (Hergoualc’h et al. Citation2021; Hergoualc’h et al, Citation2019). The water-filled pore space (WFPS) of soil is a key factor controlling N2O fluxes and N2O production, i.e., the relative contributions of nitrification and denitrification (Davidson Citation1991). Congreves et al. (Citation2019) showed that at WFPS values < 53%, nitrification was the dominant N2O production process; however, it decreased, and denitrification increased as WFPS rose from 53% to 78%, becoming the dominant N2O production process at WFPS values > 78%.
4.2. Mitigation options for N2O emissions from agricultural fields
4.2.1. Optimizing the N application rate
N2O emissions generally increase with the increase of N fertilizer application rates (Gerber et al. Citation2016; Shcherbak, Millar, and Robertson Citation2014; Van Groenigen et al. Citation2010). Thus, optimizing N use efficiency is a basic strategy for mitigating N2O emissions, although this does not always result in lower N2O emissions due to the complexity of factors affecting them (Venterea et al. Citation2012). Yield-scaled N2O emissions (N2O emissions per aboveground N uptake or crop yield) are useful to assess the strategy quantitatively. A meta-analysis showed that, for non-leguminous arable crops, optimizing the use efficiency of N fertilizers led to the lowest yield-scaled N2O emissions (N2O emissions per aboveground N uptake) and was thus preferable to minimizing N application rates (Van Groenigen et al. Citation2010). Specifically, the yield-scaled N2O emissions were the lowest (8.4 g N2O-N kg−1 N uptake) at N rates ranging from 180 to 190 kg N ha−1 and increased sharply above this level. Another meta-analysis also reported that fertilizer optimization was effective in reducing N2O emissions (mean: −31%) (Grados et al. Citation2022). This strategy is not only effective to mitigate N2O emissions but also to reduce other types of environmental pollution, e.g., water pollution and ammonia volatilization.
4.2.2. Enhanced-efficiency fertilizers
Enhanced-efficiency fertilizers (i.e., nitrification and urease inhibitors and polymer-coated fertilizers) have been used to increase the N use efficiency of crops. Nitrification inhibitors delay ammonia oxidation by inhibiting the activities of nitrifiers, and urease inhibitors delay urea hydrolysis. Polymer-coated fertilizers (slow or controlled-release fertilizers) release N at a slower rate compared to conventional fertilizers. Nitrification inhibitors and polymer-coated fertilizers have been shown to reduce N2O emissions in the Andosol and Fluvisol fields (Akiyama and Tsuruta Citation2002; Akiyama et al. Citation2013, Citation2015; Akiyama, Tsuruta, and Watanabe Citation2000). The effect of enhanced-efficiency fertilizers on N2O emissions has been reported worldwide; however, the observed effectiveness differed among studies probably due to environmental factors and different agricultural practices adopted in different regions. Therefore, statistical models and meta-analyses are useful tools to estimate the overall effectiveness of mitigation options. A meta-analysis by Akiyama et al. (Citation2010) reported that the mean N2O reduction rates of nitrification inhibitors and polymer-coated fertilizers were −38% and −35%, respectively, compared with those of conventional fertilizers, while urease inhibitors did not reduce N2O emissions. Moreover, nitrification inhibitors and polymer-coated fertilizers were effective in reducing nitric oxide emissions (−46% and −40%, respectively). Other meta-analyses have also reported significant N2O reductions by nitrification inhibitors (Chen et al. Citation2023; Grados et al. Citation2022; Ruser and Schulz Citation2015; Tufail et al. Citation2023) and polymer-coated fertilizers (Grados et al. Citation2022; Pan et al. Citation2023). Another meta-analysis suggested that dicyandiamide (DCD), a nitrification inhibitor, resulted in lower emissions of both CH4 (−18%) and N2O (−29%) from paddy rice fields (Linquist et al. Citation2012). Some studies suggested that nitrification inhibitors would increase NH3 volatilization (Adu-Poku et al. Citation2022; Kim, Saggar, and Roudier Citation2012); however, such an increase would be minimal in Japan, where NH3 volatilization is generally low because of the relatively low pH and high cation exchange capacity of volcanic ash soils (Hayashi and Yan Citation2010).
The global mean N2O emissions from ammonium-based fertilizers are higher than that from nitrate-based fertilizers (Bouwman, Boumans, and Batjes Citation2002). In well-drained soils where nitrification is the dominant N2O production process, the application of nitrate-based fertilizers may be more effective in reducing N2O emissions than the application of commonly used ammonium-based fertilizers or urea. This is because, while NO3-N produces N2O only via denitrification, ammonium-based fertilizers produce it via both nitrification and denitrification. To avoid N loss by leaching after the application of nitrate-based fertilizers, polymer-coated calcium nitrate would be a better option for the reduction of N2O emissions in well-drained fields (Akiyama and Tsuruta Citation2002; Hasukawa et al. Citation2021; Nishimura et al. Citation2021, Citation2022). It should be noted that nitrate-based fertilizers may increase N2O emissions in poorly drained fields where denitrification is the dominant N2O production process.
4.2.3. Mitigation of N2O emissions from acidic tea soils
In 2021, the tea cultivation area in Japan was 38,000 ha, accounting for only 1% of the total cultivated area (3,922,000 ha). However, tea cultivation was responsible for 14% of the total N2O emissions from synthetic N fertilizers and organic N amendments that year (calculated based on the NIR data; GIO and MOE Citation2023), due to their high average application rates, i.e., 447.6 kgN ha−1 and 162.4 kgN ha−1, respectively, and high N2O EF (2.9%) (GIO and MOE Citation2023). Thus, tea fields should be a primary target for the mitigation of N2O emissions from Japanese agricultural soil because of the unique agricultural practices, such as the high application rate of synthetic and organic N amendments in the acidic soils and the large amount of tea pruning litter input. Hirono and Nonaka (Citation2014) reported that, in a tea field, N2O emissions from lime nitrogen treatment were 50% lower than those of conventional fertilizer treatment, suggesting that the increase in soil pH caused by lime nitrogen affected N2O emissions. Although DCD was also produced during lime nitrogen decomposition, it would not be the main cause of the N2O reduction because DCD treatment was not effective to reduce N2O emissions. Similarly, Yamamoto et al. (Citation2014) reported that N2O emissions from lime nitrogen treatment were lower by 36% than those of conventional fertilizer treatment in a tea field. It should be noted that tea plants prefer acidic soils, with optimal pH values ranging from 4.0 to 5.0 (Kosuge Citation1987); therefore, the lime application rate should be limited to avoid exceeding the optimal pH of tea soil. Because tea fields are a hotspot of N2O emissions among Japanese agricultural fields, further studies on effective mitigation options for their acidic soils are needed.
4.2.4. Other mitigation options to reduce N2O emissions
A second-order meta-analysis by Grados et al. (Citation2022) reported that drip irrigation significantly reduced N2O emissions (mean: −26.5%) in addition to the optimization of fertilizer rates and application of biochar, controlled-release fertilizers, and nitrification inhibitors. In contrast, frequent fertilizer applications, crop residue removal, lime amendments, organic fertilizer applications, diversified crop rotation, deep fertilization, and no-tillage or reduced tillage were ineffective in reducing N2O emissions, with values increasing by 36.7% for cover crops. The effect of drip irrigation on GHG emissions has yet to be reported in Japan.
Microbial methods to reduce N2O emissions may be a potential option in the future; for example, N2O emissions during nodule decomposition from soybean fields can be reduced by the inoculation of nosZ++ strains of Bradyrhizobium diazoefficiens, a mutant with increased N2O reductase (N2OR) activity (Itakura et al. Citation2013) or by inoculation of a mixed culture of indigenous nosZ+ strains (with N2OR) of the same species; Akiyama et al., (Citation2016). Increasing the number of fungivorous mites through the application of coconut husks has been shown to reduce N2O emissions after organic fertilizer application when fungal denitrification would be the dominant N2O production process (Shen et al. Citation2021).
5. Effect of biochar on GHG emissions
Biochar application is an effective method to sequester soil carbon (C) (Spokas Citation2010) and has been approved in Japanese agricultural fields as a J-Credit methodology for this purpose in 2020 (METI, MOE and MAFF Citation2023). In addition to C sequestration, biochar application in tropical regions was shown to increase crop yield due to the low pH and low fertility of tropical soils and low fertilizer input; however, this effect has yet to be observed in temperate climates, according to a meta-analysis (Jeffery et al. Citation2017). Although the effect of biochar application on GHG emissions has been intensively investigated, the results obtained are not conclusive. Kaur et al. (Citation2023) evaluated various meta-analyses of the effects of biochar on N2O emissions. Among them, four showed that biochar application had insignificant effects on N2O emissions, while 14 reported average N2O reductions of −38.8% (ranging from −10.5% to −54.8%). A second-order meta-analysis by Grados et al. (Citation2022) reported that biochar application significantly reduced N2O emissions (−26.6%). However, Joseph et al. (Citation2021) pointed out that early meta-analyses including laboratory experiments with a very high biochar application rate (>100 Mg ha−1) showed high mitigation of N2O emissions, with reductions as high as 50%. In contrast, recent meta-analyses based on field studies testing realistic biochar application rates reported a considerably lower N2O reduction of about 10%. In addition, Jeffery et al. (Citation2016) reported that biochar reduced CH4 emissions from paddy rice fields, particularly from acidic soils, but decreased the CH4 sink potential of upland soils. Another meta-analysis by Wang et al. (Citation2023) suggested that biochar application reduced CH4 and N2O emissions from paddy rice fields although insignificant. In contrast, another meta-analysis by Jia et al. (Citation2023) found that biochar applications had an insignificant effect on CH4 fluxes in both paddy and upland fields.
While the results of the above-mentioned global meta-analyses on GHG reduction by biochar application are contradictory, the results of field studies conducted in Japan suggest that biochar application does not reduce GHG emissions irrespective of biochar types, application rates, or soil types. For example, Watanabe et al. (Citation2014) revealed that bamboo biochar application (20 Mg ha−1) did not affect CO2, N2O, and CH4 emissions or the yields of various vegetables and sweet potato in a Cambisol field. Similarly, the application of rice husk biochar (10–40 Mg ha−1) had no effect on CH4 and N2O emissions from a rice paddy field (Koyama et al. Citation2015), and that of wood residue-derived biochar (10–40 Mg ha−1) had no effect on CO2, N2O, and CH4 emissions or the yield and quality of various crops in an Andosol field (Koga, Shimoda, and Iwata Citation2017). The application of orchard pruning residues biochar (2–10 Mg ha−1) did not affect N2O emissions in an Andosol pear field (Oo et al. Citation2018), and that of four different biochar types (rice husk, bamboo, hardwood, and wood briquet of mixed hardwood and softwood) at 25 Mg ha−1 also did not affect N2O and CH4 emissions or the yield of spinach and Brassica spp. in an Andosol field (Yamamoto et al. Citation2019). Similarly, the application of palm shell biochar (40 Mg ha−1) did not affect N2O, CH4, and CO2 emissions in a Cambisol field (Basalirwa et al. Citation2020).
6. Conclusions
In recent years, some of the GHG mitigation techniques in the agricultural sector in Japan have been transitioning from the research stage to the implementation stage. MAFF has been promoting prolonged MD and the autumn incorporation of rice straw as parts of the direct payment program since 2015 and 2020, respectively. In addition, prolonged MD is expected to be more widely applied since its approval as a J-Credit methodology in 2022. The same approval has also been granted to biochar application for the sequestration of soil C in 2020. Mitigation options such as subsurface drainage and iron-containing material have been reported to reduce CH4 emissions from rice paddy fields, although further studies are needed to test their effects under various conditions. Numerous meta-analyses have reported the effectiveness of nitrification inhibitors and polymer-coated fertilizers in reducing N2O emissions from agricultural soils. Biochar application has demonstrated effectiveness in sequestering soil C and is intensively being investigated as a mitigation option to reduce CH4 and N2O emissions; however, the results are inconclusive. Further studies are needed to develop practical mitigation options that can be applied by local farmers to reduce GHG emissions from the agricultural sector while maintaining productivity.
Disclosure statement
No potential conflict of interest was reported by the author.
Additional information
Funding
References
- Adu-Poku, D., N. O. B. Ackerson, R. N. O. A. Devine, and A. G. Addo. 2022. “Climate Mitigation Efficiency of Nitrification and Urease Inhibitors: Impact on N2O Emission–A Review.” Scientific African 16:e01170. https://doi.org/10.1016/j.sciaf.2022.e01170.
- Akiyama, H., Y. T. Hoshino, M. Itakura, Y. Shimomura, Y. Wang, A. Yamamoto, K. Tago, Y. Nakajima, K. Minamisawa, and M. Hayatsu. 2016. “Mitigation of Soil N2O Emission by Inoculation with a Mixed Culture of Indigenous Bradyrhizobium Diazoefficiens.” Scientific Reports 6:32869. https://doi.org/10.1038/srep32869.
- Akiyama, H., S. Morimoto, M. Hayatsu, A. Hayakawa, S. Sudo, and K. Yagi. 2013. “Nitrification, Ammonia-Oxidizing Communities, and N2O and CH4 Fluxes in an Imperfectly Drained Agricultural Field Fertilized with Coated Urea with and without Dicyandiamide.” Biology and Fertility of Soils 49 (2): 213–223. https://doi.org/10.1007/s00374-012-0713-2.
- Akiyama, H., T. Sano, K. Nishina, S. Sudo, N. Oura, M. Fujimori, I. Uezono, et al. 2023. “N2O Emission Factors for Organic Amendments in Japan from Measurement Campaign and Systematic Review.” Science of the Total Environment 864:161088. https://doi.org/10.1016/j.scitotenv.2022.161088.
- Akiyama, H., and H. Tsuruta. 2002. “Effect of Chemical Fertilizer Form on N2O, NO and NO2 Fluxes from Andisol Field.” Nutrient Cycling in Agroecosystems 63 (2/3): 219–230. https://doi.org/10.1023/A:1021102925159.
- Akiyama, H., H. Tsuruta, and T. Watanabe. 2000. “N2O and NO Emissions from Soils After the Application of Different Chemical Fertilizers.” Chemosphere – Global Change Science 2 (3–4): 313–320. https://doi.org/10.1016/S1465-9972(00)00010-6.
- Akiyama, H., Y. Uchida, K. Tago, Y. T. Hoshino, Y. Shimomura, Y. Wang, and M. Hayatsu. 2015. “Effect of Dicyandiamide and Polymer Coated Urea Applications on N2O, NO and CH4 Fluxes from Andosol and Fluvisol Fields.” Soil Science and Plant Nutrition 61 (3): 541–551. https://doi.org/10.1080/00380768.2015.1028103.
- Akiyama, H., K. Yagi, and X. Yan. 2005. “Direct N2O Emissions from Rice Paddy Fields: Summary of Available Data.” Global Biogeochemical Cycles 19:1. https://doi.org/10.1029/2004GB002378.
- Akiyama, H., X. Y. Yan, and K. Yagi. 2006. “Estimations of Emission Factors for Fertilizer-Induced Direct N2O Emissions from Agricultural Soils in Japan: Summary of Available Data.” Soil Science and Plant Nutrition 52 (6): 774–787. https://doi.org/10.1111/j.1747-0765.2006.00097.x.
- Akiyama, H., X. Y. Yan, and K. Yagi. 2010. “Evaluation of Effectiveness of Enhanced-Efficiency Fertilizers as Mitigation Options for N2O and NO Emissions from Agricultural Soils: Meta-Analysis.” Global Change Biology 16 (6): 1837–1846. https://doi.org/10.1111/j.1365-2486.2009.02031.x.
- Basalirwa, D., S. Sudo, C. Wacal, A. Z. Oo, D. Sasagawa, S. Yamamoto, T. Masunaga, and E. Nishihara. 2020. “Impact of Fresh and Aged Palm Shell Biochar on N2O Emissions, Soil Properties, Nutrient Content and Yield of Komatsuna (Brassica Rapa Var. Perviridis) Under Sandy Soil Conditions.” Soil Science and Plant Nutrition 66 (2): 328–343. https://doi.org/10.1080/00380768.2019.1705737.
- Bouwman, A. F., L. J. M. Boumans, and N. H. Batjes. 2002. “Emissions of N2O and NO from Fertilized Fields: Summary of Available Measurement Data.” Global Biogeochemical Cycles 16 (4): 6–1/2001. https://doi.org/10.1029/2001GB001811.
- Canadell, J. G., P. M. S. Monteiro, M. H. Costa, L. Cotrim da Cunha, P.M. Cox, A.V. Eliseev, S. Henson. 2021 “The Earth’s Energy Budget, Climate Feedbacks, and Climate Sensitivity.” In Climate Change 2021: the Physical Science Basis. Contribution of Working Group I to the Sixth Assessment Report of the Intergovernmental Panel on Climate Change, edited by V. Masson-Delmotte,P. Zhai, A. Pirani, S.L. Connors, C. Péan, S. Berger, N. Caud, et al., 673–816. Cambridge: United Kingdom and Cambridge: Cambridge University Press. https://doi.org/10.1017/9781009157896.007.
- Chen, M., A. Schievano, S. Bosco, A. Montero-Castaño, G. Tamburini, M. Pérez-Soba, and D. Makowski. 2023. “Evidence Map of the Benefits of Enhanced-Efficiency Fertilisers for the Environment, Nutrient Use Efficiency, Soil Fertility, and Crop Production.” Environmental Research Letters 18 (4). https://doi.org/10.1088/1748-9326/acb833.
- Chirinda, N., L. Arenas, M. Katto, S. Loaiza, F. Correa, M. Ishitani, A. Loboguerrero, et al. 2018. “Sustainable and Low Greenhouse Gas Emitting Rice Production in Latin America and the Caribbean: A Review on the Transition from Ideality to Reality.” Sustainability 10:3. https://doi.org/10.3390/su10030671.
- Congreves, K. A., T. Phan, and R. E. Farrell. 2019. “A New Look at an Old Concept: Using 15N2O Isotopomers to Understand the Relationship Between Soil Moisture and N2O Production Pathways.” SOIL 5 (2): 265–274. https://doi.org/10.5194/soil-5-265-2019.
- Conrad, R. 2020a. “Importance of Hydrogenotrophic, Aceticlastic and Methylotrophic Methanogenesis for Methane Production in Terrestrial, Aquatic and Other Anoxic Environments: A Mini Review.” Pedosphere 30 (1): 25–39. https://doi.org/10.1016/S1002-0160(18)60052-9.
- Conrad, R. 2020b. “Methane Production in Soil Environments—Anaerobic Biogeochemistry and Microbial Life Between Flooding and Desiccation.” Microorganisms 8 (6): 881. https://doi.org/10.3390/microorganisms8060881.
- Davidson, E. A. 1991. “Fluxes of Nitrous Oxide and Nitric Oxide from Terrestrial Ecosystems.” In Microbial Production and Consumption of Greenhouse Gases: Methane, Nitrogen Oxides and Halomethanes. Società, edited by J. E. Rogers and W. B. Am Whitman, 219–235. Washington, DC: Microbiol.
- Forster, P., T. Storelvmo, K. Armour, W. Collins, J.-L. Dufresne, D. Frame, D. J. Lunt, et al. 2021. “The Earth’s Energy Budget, Climate Feedbacks, and Climate Sensitivity.”In Climate Change 2021: The Physical Science Basis. Contribution of Working Group I to the Sixth Assessment Report of the Intergovernmental Panel on Climate Change, edited by, V. Masson-Delmotte, P. Zhai, A. Pirani, S. L. Connors, C. Péan, S. Berger, N. Caud, et al., 923–1054. Cambridge, United Kingdom and New York, NY, USA: Cambridge University Press. https://doi.org/10.1017/9781009157896.009.
- Fumoto, T., T. Yanagihara, T. Saito, and K. Yagi. 2010. “Assessment of the Methane Mitigation Potentials of Alternative Water Regimes in Rice Fields Using a Process-Based Biogeochemistry Model.” Global Change Biology 16 (6): 1847–1859. https://doi.org/10.1111/j.1365-2486.2009.02050.x.
- Furukawa, Y., and K. Inubushi. 2004. “Evaluation of Slag Application to Decrease Methane Emission from Paddy Soil and Fate of Iron.” Soil Science and Plant Nutrition 50 (7): 1029–1036. https://doi.org/10.1080/00380768.2004.10408570.
- Gerber, J. S., K. M. Carlson, D. Makowski, N. D. Mueller, G. de Cortazar-Atauri, H. Iñaki, H. Petr, et al. 2016. “Spatially Explicit Estimates of N2O Emissions from Croplands Suggest Climate Mitigation Opportunities from Improved Fertilizer Management.” Global Change Biology 22 (10): 3383–3394. https://doi.org/10.1111/gcb.13341.
- GIO and MOE (Greenhouse Gas Inventory Office of Japan and Ministry of the Environment). 2023. Center for Global Environmental Research, Earth System Division, National Institute for Environmental Studies, Japan. https://www.nies.go.jp/gio/en/archive/nir/jqjm1000001v3csj-att/NIR-JPN-2023-v3.0_gioweb.pdf.
- Grados, D., K. Butterbach-Bahl, J. Chen, K. Jan van Groenigen, J. E. Olesen, J. Willem van Groenigen, and D. Abalos. 2022. “Synthesizing the Evidence of Nitrous Oxide Mitigation Practices in Agroecosystems.” Environmental Research Letters 17 (11). https://doi.org/10.1088/1748-9326/ac9b50.
- Gupta, K., R. Kumar, K. K. Baruah, S. Hazarika, S. Karmakar, and N. Bordoloi. 2021. “Greenhouse Gas Emission from Rice Fields: A Review from Indian Context.” Environmental Science and Pollution Research International 28 (24): 30551–30572. https://doi.org/10.1007/s11356-021-13935-1.
- Hasukawa, H., Y. Inoda, T. Takayama, K. Takehisa, S. Sudo, H. Akiyama, and J. Yanai. 2021. “Effects of Controlled Release N Fertilizers and Reduced Application Rate on Nitrous Oxide Emissions from Soybean Fields Converted from Rice Paddies.” Soil Science and Plant Nutrition 67 (6): 716–726. https://doi.org/10.1080/00380768.2021.2011614.
- Hayashi, K., T. Tokida, M. Kajiura, Y. Yanai, and M. Yano. 2015. “Cropland Soil-Plant Systems Control Production and Consumption of Methane and Nitrous Oxide and Their Emissions to the Atmosphere.” Soil Science and Plant Nutrition 61 (1): 2–33. https://doi.org/10.1080/00380768.2014.994469.
- Hayashi, K., and X. Yan. 2010. “Airborne Nitrogen Load in Japanese and Chinese Agroecosystems.” Soil Science and Plant Nutrition 56 (1): 2–18. https://doi.org/10.1111/j.1747-0765.2009.00423.x.
- Hayatsu, M., C. Katsuyama, and K. Tago. 2021. “Overview of Recent Researches on Nitrifying Microorganisms in Soil.” Soil Science and Plant Nutrition 67 (6): 619–632. https://doi.org/10.1080/00380768.2021.1981119.
- Hayatsu, M., K. Tago, and M. Saito. 2008. “Various Players in the Nitrogen Cycle: Diversity and Functions of the Microorganisms Involved in Nitrification and Denitrification.” Soil Science and Plant Nutrition 54 (1): 33–45. https://doi.org/10.1111/j.1747-0765.2007.00195.x.
- Hergoualc’h, K., H. Akiyama, M. Bernoux, N. Chirinda, A. del Prado, Å. Kasimir, J. D. MacDonald, S. M. Ogle, K. Regina, T. J. van der Weerden. 2019. “N2O emissions from managed soils, and CO2 emissions from lime and urea application.” Agriculture, Forestry and Other Land Use, 2019 Refinement to the 2006 IPCC Guidelines for National Greenhouse Gas Inventories, Vol. 4. Cambridge University Press. https://www.ipcc-nggip.iges.or.jp/public/2019rf/pdf/4_Volume4/19R_V4_Ch11_Soils_N2O_CO2.pdf.
- Hergoualc’h, K., N. Mueller, M. Bernoux, Ä. Kasimir, T. J. van der Weerden, and S.M. Ogle. 2021. “Improved Accuracy and Reduced Uncertainty in Greenhouse Gas Inventories by Refining the IPCC Emission Factor for Direct N2O Emissions from Nitrogen Inputs to Managed Soils.” Global Change Biology 27 (24): 6536–6550. https://doi.org/10.1111/gcb.15884.
- Hirono, Y., and K. Nonaka. 2014. “Effects of Application of Lime Nitrogen and Dicyandiamide on Nitrous Oxide Emissions from Green Tea Fields.” Soil Science and Plant Nutrition 60 (2): 276–285. https://doi.org/10.1080/00380768.2014.890015.
- Itakura, M., Y. Uchida, H. Akiyama, Y. T. Hoshino, Y. Shimomura, S. Morimoto, K. Tago, et al. 2013. “Mitigation of Nitrous Oxide Emissions from Soils by Bradyrhizobium japonicum Inoculation.” Nature Climate Change 3 (3): 208–212. https://doi.org/10.1038/nclimate1734.
- Itoh, M., S. Sudo, S. Mori, H. Saito, T. Yoshida, Y. Shiratori, S. Suga, et al. 2011. “Mitigation of Methane Emissions from Paddy Fields by Prolonging Midseason Drainage.” Agriculture, Ecosystems and Environment 141 (3–4): 359–372. https://doi.org/10.1016/j.agee.2011.03.019.
- Jeffery, S., D. Abalos, M. Prodana, A. C. Bastos, J. W. van Groenigen, B. A. Hungate, and F. Verheijen. 2017. “Biochar Boosts Tropical but Not Temperate Crop Yields.” Environmental Research Letters 12 (5). https://doi.org/10.1088/1748-9326/aa67bd.
- Jeffery, S., F. G. A. Verheijen, C. Kammann, and D. Abalos. 2016. “Biochar Effects on Methane Emissions from Soils: A Meta-Analysis.” Soil Biology and Biochemistry 101:251–258. https://doi.org/10.1016/j.soilbio.2016.07.021.
- Jia, G., E. Shevliakova, P. Artaxo, N. De Noblet-Ducoudré, R. Houghton, J. House, K. Kitajima, et al. “Land–Climate Interactions.” In Climate Change and Land: An IPCC Special Report on Climate Change, Desertification, Land Degradation, Sustainable Land Management, Food Security, and Greenhouse Gas Fluxes in Terrestrial Ecosystems, edited by P. R. Shukla, J. Skea, E. Calvo Buendia, V. Masson-Delmotte, H.-O. Pörtner, D.C. Roberts, P. Zhai, et al. Cambridge University Press. https://doi.org/10.1017/9781009157988.004.
- Jia, X., W. Yan, H. Ma, and Z. Shangguan. 2023. “Antagonistic and Synergistic Interactions Dominate GHGs Fluxes, Soil Properties and Yield Responses to Biochar and N Addition.” Frontiers in Environmental Science 11. https://doi.org/10.3389/fenvs.2023.1123897.
- Jiménez, J. C., and O. Pedersen. 2023. “Mitigation of Greenhouse Gas Emissions from Rice via Manipulation of Key Root Traits.” Rice 16 (1): 24. https://doi.org/10.1186/s12284-023-00638-z.
- Joseph, S., A. L. Cowie, L. Van Zwieten, N. Bolan, A. Budai, W. Buss, M. L. Cayuela, et al. 2021. “How Biochar Works, and When It Doesn’t: A Review of Mechanisms Controlling Soil and Plant Responses to Biochar.” GCB Bioenergy 13 (11): 1731–1764. https://doi.org/10.1111/gcbb.12885.
- Kajiura, M., K. Minamikawa, T. Tokida, Y. Shirato, and R. Wagai. 2018. “Methane and Nitrous Oxide Emissions from Paddy Fields in Japan: An Assessment of Controlling Factor Using an Intensive Regional Data Set.” Agriculture, Ecosystems and Environment 252:51–60. https://doi.org/10.1016/j.agee.2017.09.035.
- Kajiura, M., and T. Tokida. 2021. “Quantifying Bubbling Emission (Ebullition) of Methane from a Rice Paddy Using High-Time-Resolution Concentration Data Obtained During a Closed-Chamber Measurement.” Journal of Agricultural Meteorology 77 (4): 245–252. https://doi.org/10.2480/agrmet.D-21-00022.
- Katayanagi, N., T. Fumoto, M. Hayano, Y. Shirato, Y. Takata, A. Leon, and K. Yagi. 2017. “Estimation of Total CH4 Emission from Japanese Rice Paddies Using a New Estimation Method Based on the DNDC-Rice Simulation Model.” Science of the Total Environment 601–602:346–355. https://doi.org/10.1016/j.scitotenv.2017.05.090.
- Kaur, N., C. Kieffer, W. Ren, and D. Hui. 2023. “How Much is Soil Nitrous Oxide Emission Reduced with Biochar Application? An Evaluation of Meta-Analyses.” GCB Bioenergy 15 (1): 24–37. https://doi.org/10.1111/gcbb.13003.
- Kim, D., S. Saggar, and P. Roudier. 2012. “The Effect of Nitrification Inhibitors on Soil Ammonia Emissions in Nitrogen Managed Soils: A Meta-Analysis.” Nutrient Cycling in Agroecosystems 93 (1): 51–64. https://doi.org/10.1007/s10705-012-9498-9.
- Kimura, M., J. Murase, and Y. Lu. 2004. “Carbon Cycling in Rice Field Ecosystems in the Context of Input, Decomposition and Translocation of Organic Materials and the Fates of Their End Products (CO2 and CH4).” Soil Biology and Biochemistry 36 (9): 1399–1416. https://doi.org/10.1016/j.soilbio.2004.03.006.
- Koga, N., S. Shimoda, and Y. Iwata. 2017. “Biochar Impacts on Crop Productivity and Greenhouse Gas Emissions from an Andosol.” Journal of Environmental Quality 46 (1): 27–35. https://doi.org/10.2134/jeq2016.04.0156.
- Kosuge, N. 1987. “Optimum pH of Tea Soil.” Chagyo Kenkyu Hokoku 66:98–101. in Japanese. https://doi.org/10.5979/cha.1987.66_98.
- Koyama, S., F. Inazaki, K. Minamikawa, M. Kato, and H. Hayashi. 2015. “Increase in Soil Carbon Sequestration Using Rice Husk Charcoal without Stimulating CH4 and N2O Emissions in an Andosol Paddy Field in Japan.” Soil Science and Plant Nutrition 61 (5): 873–884. https://doi.org/10.1080/00380768.2015.1065511.
- Linquist, B. A., M. A. Adviento-Borbe, C. M. Pittelkow, C. van Kessel, and K. J. van Groenigen. 2012. “Fertilizer Management Practices and Greenhouse Gas Emissions from Rice Systems: A Quantitative Review and Analysis.” Field Crops Research 135:10–21. https://doi.org/10.1016/j.fcr.2012.06.007.
- Linquist, B., K. J. van Groenigen, M. A. Adviento-Borbe, C. Pittelkow, and C. van Kessel. 2012. “An Agronomic Assessment of Greenhouse Gas Emissions from Major Cereal Crops.” Global Change Biology 18 (1): 194–209. https://doi.org/10.1111/j.1365-2486.2011.02502.x.
- MAFF (Ministry of Agriculture Forestry and Fisheries). 2022. “Awareness and Intention Survey on Food, Agriculture, Forestry and Fisheries, and Rural Areas 2021” ( in Japanese). https://www.maff.go.jp/j/finding/mind/attach/pdf/index-74.pdf.
- MAFF (Ministry of Agriculture Forestry and Fisheries). 2023a. “ The 96th Statistical Yearbook of Ministry of Agriculture. Forestry and Fisheries.” https://www.maff.go.jp/e/data/stat/96th/index.html#1.
- MAFF (Ministry of Agriculture Forestry and Fisheries). 2023b. “The Direct Payments for Environmentally Friendly Agriculture.” ( in Japanese). https://www.maff.go.jp/j/seisan/kankyo/kakyou_chokubarai/mainp.html.
- METI (Ministry of Economy Trade and Industry), MOE (Ministry of Environment and MAFF (Ministry of Agriculture, Forestry and Fisheries). 2023. “J-Credit Scheme.” https://japancredit.go.jp/.
- Nakajima, M., W. Cheng, S. Hanayama, and M. Okada. 2017. “Shallow Autumn Tillage Does Not Reduce CH4 Emission from an Andisol Paddy Field in Morioka, a Cold Region in Japan.” Journal of Agricultural Meteorology 73 (3): 92–99. https://doi.org/10.2480/agrmet.D-16-00009.
- NARO (National Agriculture and Food Research Organization). 2023. Japan Soil Inventory. soil-inventory.dc.affrc.go.jp.
- Nishimura, S., K. Kimiwada, A. Yagioka, S. Hayashi, and N. Oka. 2020. “Effect of Intermittent Drainage in Reduction of Methane Emission from Paddy Soils in Hokkaido, Northern Japan.” Soil Science and Plant Nutrition 66 (2): 360–368. https://doi.org/10.1080/00380768.2019.1706191.
- Nishimura, S., T. Sugito, A. Nagatake, and N. Oka. 2021. “Nitrous Oxide Emission Reduced by Coated Nitrate Fertilizer in a Cool-Temperate Region.” Nutrient Cycling in Agroecosystems 119 (2): 275–289. https://doi.org/10.1007/s10705-020-10116-3.
- Nishimura, S., M. Yoshimura, T. Yamane, and N. Oka. 2022. “Effects of Coated Slow-Release Fertilizers on Nitrous Oxide Emission from Winter Wheat Field in a Cool-Temperate Region in Japan.” Soil Science and Plant Nutrition 68 (2): 305–316. https://doi.org/10.1080/00380768.2022.2038521.
- Ogle, S. M., K. Butterbach-Bahl, L. Cardenas, U. Skiba, and C. Scheer. 2020. “From Research to Policy: Optimizing the Design of a National Monitoring System to Mitigate Soil Nitrous Oxide Emissions.” Current Opinion in Environmental Sustainability 47:28–36. https://doi.org/10.1016/j.cosust.2020.06.003.
- Ogle, S. M., S. J. Wakelin, L. Buendia, B. McConkey, J. Baldock, H. Akiyama, A. W. M. Kishimoto, et al. 2019. “Cropland.” Agriculture, Forestry and Other Land Use, 2019 Refinement to the 2006 IPCC Guidelines for National Greenhouse Gas Inventories, Vol. 4. Cambridge University Press. https://www.ipcc-nggip.iges.or.jp/public/2019rf/pdf/4_Volume4/19R_V4_Ch05_Cropland.pdf.
- Oo, A. Z., T. Gonai, S. Sudo, K. T. Win, and A. Shibata. 2018. “Surface Application of Fertilizers and Residue Biochar on N2O Emission from Japanese Pear Orchard Soil.” Soil and Environment 64 (12): 597–604. https://doi.org/10.17221/114/2018-PSE.
- Pan, Z., D. Fan, R. Jiang, N. Abbasi, D. Song, G. Zou, D. Wei, P. He, and W. He. 2023. “Improving Potato Productivity and Mitigating Nitrogen Losses Using Enhanced-Efficiency Fertilizers: A Global Meta-Analysis.” Agriculture, Ecosystems and Environment 348:108416. https://doi.org/10.1016/j.agee.2023.108416.
- Ruser, R., and R. Schulz. 2015. “The Effect of Nitrification Inhibitors on the Nitrous Oxide (N2O) Release from Agricultural Soils-A Review.” Journal of Plant Nutrition and Soil Science 178 (2): 171–188. https://doi.org/10.1002/jpln.201400251.
- Schutz, H., W. Seiler, and R. Conrad. 1989. “Processes Involved in Formation and Emission of Methane in Rice Paddies.” Biogeochemistry 7 (1): 33–53. https://doi.org/10.1007/BF00000896.
- Shcherbak, I., N. Millar, and G. P. Robertson. 2014. “Global Metaanalysis of the Nonlinear Response of Soil Nitrous Oxide (N2O) Emissions to Fertilizer Nitrogen.” Proceedings of the National Academy of Sciences of the United States of America 111 (25): 9199–9204. https://doi.org/10.1073/pnas.1322434111.
- Shen, H., Y. Shiratori, S. Ohta, Y. Masuda, K. Isobe, and K. Senoo. 2021. “Mitigating N2O Emissions from Agricultural Soils with Fungivorous Mites.” The ISME Journal 15 (8): 2427–2439. https://doi.org/10.1038/s41396-021-00948-4.
- Shiratori, Y., H. Watanabe, Y. Furukawa, H. Tsuruta, and K. Inubushi. 2007. “Effectiveness of a Subsurface Drainage System in Poorly Drained Paddy Fields on Reduction of Methane Emissions.” Soil Science and Plant Nutrition 53 (4): 387–400. https://doi.org/10.1111/j.1747-0765.2007.00171.x.
- Singla, A., and K. Inubushi. 2015. “Effect of Slag-Type Fertilizers on N2O Flux from Komatsuna Vegetated Soil and CH4 Flux from Paddy Vegetated Soil.” Paddy and Water Environment 13 (1): 43–50. https://doi.org/10.1007/s10333-013-0405-z.
- Spokas, K. A. 2010. “Review of the Stability of Biochar in Soils: Predictability of O: C Molar Ratios.” Carbon Management 1 (2): 289–303. https://doi.org/10.4155/cmt.10.32.
- Susilawati, H. L., P. Setyanto, A. K. Makarim, M. Ariani, K. Ito, and K. Inubushi. 2015. “Effects of Steel Slag Applications on CH4, N2O and the Yields of Indonesian Rice Fields: A Case Study During Two Consecutive Rice-Growing Seasons at Two Sites.” Soil Science and Plant Nutrition 61 (4): 704–718. https://doi.org/10.1080/00380768.2015.1041861.
- Takakai, F., M. Goto, H. Watanabe, K. Hatakeyama, K. Yasuda, T. Sato, and Y. Kaneta. 12 2021. “Effects of the Autumn Incorporation of Rice Straw and Application of Lime Nitrogen on Methane and Nitrous Oxide Emissions and Rice Growth of a High–Yielding Paddy Field in a Cool–Temperate Region in Japan.” Agriculture 11 (12): 1298. https://doi.org/10.3390/agriculture11121298.
- Tufail, M. A., M. Irfan, W. Umar, A. Wakeel, and R. A. Schmitz. 2023. “Mediation of Gaseous Emissions and Improving Plant Productivity by DCD and DMPP Nitrification Inhibitors: Meta–Analysis of Last Three Decades.” Environmental Science and Pollution Research International 30 (23): 64719–64735. https://doi.org/10.1007/s11356–023–26318–5.
- Van Groenigen, J. W., G. L. Velthof, O. Oenema, K. J. Van Groenigen, and C. Van Kessel. 2010. “Towards an Agronomic Assessment of N2O Emissions: A Case Study for Arable Crops.” European Journal of Soil Science 61 (6): 903–913. https://doi.org/10.1111/j.1365–2389.2009.01217.x.
- Venterea, R. T., A. D. Halvorson, N. Kitchen, M. A. Liebig, M. A. Cavigelli, S. J. D. Grosso, P. P. Motavalli, et al. 2012. “Challenges and Opportunities for Mitigating Nitrous Oxide Emissions from Fertilized Cropping Systems.” Frontiers in Ecology and the Environment 10 (10): 562–570. https://doi.org/10.1890/120062.
- Wang, J., H. Akiyama, K. Yagi, and X. Yan. 2018. “Controlling Variables and Emission Factors of Methane from Global Rice Fields.” Atmospheric Chemistry and Physics 18 (14): 10419–10431. https://doi.org/10.5194/acp–18–10419–2018.
- Wang, J., P. Ciais, P. Smith, X. Yan, Y. Kuzyakov, S. Liu, L. Tingting, and J. Zou. 2023. “The Role of Rice Cultivation in Changes in Atmospheric Methane Concentration and the Global Methane Pledge.” Global Change Biology 29 (10): 2776–2789. https://doi.org/10.1111/gcb.16631.
- Watanabe, A., K. Ikeya, N. Kanazaki, S. Makabe, Y. Sugiura, and A. Shibata. 2014. “Five Crop Seasons’ Records of Greenhouse Gas Fluxes from Upland Fields with Repetitive Applications of Biochar and Cattle Manure.” Journal of Environmental Management 144:168–175. https://doi.org/10.1016/j.jenvman.2014.05.032.
- Yamamoto, A., H. Akiyama, M. Kojima, and A. Osaki. 2019. “Nitrous Oxide Emissions from an Andosol Upland Field Amended with Four Different Types of Biochars.” Nutrient Cycling in Agroecosystems 113 (3): 323–335. https://doi.org/10.1007/s10705–019–09983–2.
- Yamamoto, A., H. Akiyama, T. Naokawa, Y. Miyazaki, Y. Honda, Y. Sano, Y. Nakajima, and K. Yagi. 2014. “Lime–Nitrogen Application Affects Nitrification, Denitrification, and N2O Emission in an Acidic Tea Soil.” Biology and Fertility of Soils 50 (1): 53–62. https://doi.org/10.1007/s00374–013–0830–6.
- Zhang, Y., M. Huang, K. Yu, Y. Xie, Y. Wang, J. Wu, F. Zheng, et al. 2023. “Decreased CH4 Emissions Associated with Methanogenic and Methanotrophic Communities and Their Interactions Following Fe(iii) Fertiliser Application in Rice Paddies.“ Geoderma. 431. https://doi.org/10.1016/j.geoderma.2023.116375.