ABSTRACT
Mosses are a common type of organism found colonising stone in both the natural and built environment. People responsible for managing and conserving historic buildings often worry that mosses can cause biodeterioration, but there is very little research to date on what controls moss growth and the nature and magnitude of their impact. To help fill this knowledge gap, research has been carried out on limestone walls at Kelmscott Manor, the churchyard at Chipping Norton, and in the laboratory on limestone test blocks, to examine the impact of microclimate variations on both the initial colonisation and spread of mosses, and the impact of moss colonisation on temperature, relative humidity, and moisture conditions. The research used a combination of non-destructive and invasive tests to determine the impact of mosses on moisture relations through direct impacts on surface moisture, water absorption capacity, and open porosity. From the results presented, we can infer that mosses have an impact on water uptake and retention on mortar and limestone at both the surface and subsurface level, and that they may play a role in ‘shielding’ the underlying substrate from light rainfall events. The experimental data also suggests that in certain cases, removing mosses may have more of an impact on moisture regimes than leaving them in situ.
Introduction
Mosses are one common form of organisms which grow at the lithobiontic interface. Previous studies have investigated and characterized the impact of a range of organisms growing on cultural heritage and have defined the lithobiontic interface as the zone of interaction between organisms and their underlying substrates (McIlroy de la Rosa, Warke, and Smith Citation2012). Research on organisms such as lichens, algae, and bacteria growing at the lithobiontic interface has revealed a range of biodeteriorative and bioprotective impacts (Slavík et al. Citation2016; Morando et al. Citation2017). The relationship between these organisms and their substrates may be complex and multilayered, and the influence may not be entirely bioprotective or biodeteriorative, but rather the true impact may lie somewhere on a spectrum between these two end points. The importance of understanding the lithobiontic interface for conserving and managing built cultural heritage has been previously explored, identifying the impact of the growth of organisms on stone-built cultural heritage to the weathering, durability, and lifespan of built heritage (Smith and McCabe Citation2008). Mechanisms of biodeterioration at the lithobiontic interface have been characterized primarily for lichens, with an understanding that they can have both direct and indirect chemical and physical impacts (Fontaine, Hendrickx, and Clercq Citation2015). Specific impacts include direct impacts which contribute to biodeterioration such as pitting, erosion, and the deposition of acidic by-products from metabolic processes, while some indirect impacts of colonization have been reported to have bioprotective effects, such as reducing temperature fluctuations at the stone surface and freeze-thaw effects as well as providing a physical barrier for airborne pollutants (McIlroy de la Rosa, Warke, and Smith Citation2012, Citation2013). According to previous research on epilithic and endolithic lichens on Portland and Botticino limestones, lichen hyphae have been seen to extend 2.0–3.0 mm beneath the surface and thus the lithobiontic interface includes not only on surface, but also subsurface interactions (Morando et al. Citation2017). Findings from lichens may also have relevance to mosses as these subsurface penetration and anchoring mechanisms are also present in moss morphology and could potentially be a reason why moss fragments are seen within the stone substrate even after cleaning (Slavík et al. Citation2016). While mosses are evidenced to have wide impacts on their underlying substrates, including but not limited to biochemical decomposition and accelerated physical degradation, this project focuses on the direct impacts of moisture and saturation.
The spatial extent of the lithobiontic interface is of particular interest when discussing the impacts of moisture interactions for mosses, due to the nature of their growth patterns and morphology. Moss growth patterns fall broadly within two major categories with distinct characteristics (). Pleurocarpous mosses are freely branched and amorphous, with prostrate habit and lateral growth. They are often described as ‘carpeting’ and are more firmly attached to the substrate. Acrocarpous mosses are unbranched and have an ascending habit, with a circular form. They are often described as ‘cushions’ and are more loosely attached to the underlying substrate. All forms of mosses are attached to the substrate by small structures known as rhizoids. This project primarily analyses the impact of the species Brachythecium rutabulum (Schimper 1853), which is described by the British Bryological Society as one of the commonest mosses (in the United Kingdom and worldwide) and is found in a wide range of habitats including natural and urban environments although it is especially common on wood and stone (BBS Field Guide Citation2010, 747). This species of moss has a pervasive biogeographical spread and is a common species of moss widely found in temperate and subpolar climatic zones.
Figure 1. Acrocarpous and pleurocarpous moss morphology on a cementitious mortar capped wall at Kelmscott Manor, Lechlade UK. Photo by authors
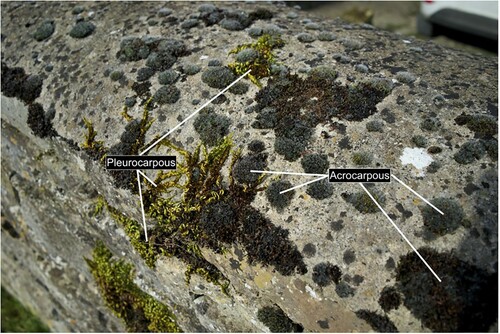
While this project primarily analyses the impact of the species Brachythecium rutabulum, mosses share certain similarities in morphology and habit which means that at least some of the identified impacts are likely to be applicable to mosses in general. When observing the impacts of moss morphology on moisture and the built environment, the adaptations for surviving drought and routine desiccation are particularly significant. Mosses differ from vascular plants in the nature of their water uptake and circulation, as they lack the tissue structures which transport water and other nutrients. According to previous research, most dryland mosses have evolved to require desiccation and during desiccated periods, ‘shoot tissues lose virtually all liquid water and can dry to 5-10% of dry mass and water potentials of −100 MPa’ (Coe and Sparks Citation2014, 294). Not only can mosses tolerate periods of extreme drought, but some species of mosses have evolved to retain moisture during brief rainfall events. The use of the species Sphagnum mosses is documented throughout World War One for absorptive properties and ability to retain ‘16-20 times its own weight in liquid, as compared to 4–6 times of cotton’ (Rotherham and Griffiths Citation2013, 202). This species of moss gained widespread use as a field dressing and was harvested for its absorptive properties.
Although there has been relatively little scientific research on the impact of mosses on built cultural heritage, the advice and management strategies from built heritage bodies and local councils (UK) broadly agree on the treatment of mosses on built heritage structures. Advice from local heritage bodies and councils broadly addresses two key issues with mosses. The first is that moss should be treated as general debris, with adverse effects primarily on roof structures, guttering, and drainage. Their treatment as general debris can be extended to areas where they may not be aesthetically pleasing to viewers, such as walls, decorative assemblages, and external structures such as gates (Historic Environment Scotland Advisory Standards Citation2005; Eklund Citation2013; Edinburgh World Heritage Historic Home Guide Citation2019). Of course, the removal of mosses can have a dramatic effect on the aesthetics and presentation of a cultural heritage asset and may be a consideration in the treatment and management strategy of the structure. Past management strategies of rural heritage have indicated that the presence of mosses is beneficial to the aesthetic presentation of a site, and their presence at the abandoned monastery of Dryburgh Abbey particularly conveyed values such as ‘age’ and ‘authenticity’ (Douglas-Jones et al. Citation2016). In this case, the removal of mosses would be detrimental to the presentation and overall impact of the heritage site. In these rural heritage sites, the relationship between organisms and the underlying structures are seen as inseparable. The second piece of advice from local councils and heritage bodies is relevant to the relationship between moisture and mosses. They often describe mosses as ‘retaining moisture’ and ‘causing moisture,’ which accelerates the deterioration of the underlying substrate and recommend removal. These two terms are often used interchangeably, but when examined more closely have very different meanings. The description of mosses ‘retaining moisture’ is linked to the original use of Sphagnum mosses and their uptake of moisture from external sources. When describing this effect on built heritage, it may be tied to micro and macro-environmental fluctuations, such as relative humidity or rainfall. However, the description of mosses as ‘causing moisture,’ on the other hand, has a very different meaning. The use of this description may be misleading because it implies that through the growth and colonisation of mosses they create inherently moist and wet environments when as described above they regularly undergo desiccation.
Aims and objectives
The primary objective of this project is to determine if the presence of mosses impacts moisture regimes on their underlying substrates in any way. Non-destructive and non-invasive tests in the field and laboratory have been chosen to examine the following research questions:
How does moss growth influence microclimate fluctuations, and similarly, how do microclimate fluctuations and aspect influence moss growth?
How does moss growth influence evaporation rates and water uptake at surface and subsurface levels?
Materials, sites, and methods
Materials
This project used a mixture of laboratory and on-site analyses to investigate the role of mosses on moisture relations on limestone and cementitious mortar. The laboratory portion of this research project uses a set of weathered limestone blocks from a previous research project (Eklund n.d.). In 2010, 16 Portland limestone blocks were exposed as part of a project on bioreceptivity in a grassland area at Wytham Woods, near Oxford (516′12.7″N 19′58.8″W) and were appropriated for this research project in 2017. The cubes (15 × 15 × 15 cm) were cut from two types of Portland limestone – fine-grained Jordan Basebed (JB) and coarse grained, fossil encrusted Bowers Roach (BR). In total, twelve JB and four BR blocks were exposed with bedding planes horizontal on a geotextile in an open field setting. The cubes were left on site to weather naturally and collected in autumn 2017, after seven years of exposure. For the purposes of this project, six JB and two BR blocks were recovered along with the original one JB and one BR control blocks which were stored over the same period in a controlled environment laboratory facility in Oxford. The field investigations included cementitious mortar capping on limestone walls at Kelmscott Manor, Lechlade UK (), microclimate monitoring of structural walls at Kelmscott Manor, and local ooidal limestone from Chipping Norton.
Sites
The laboratory analyses were carried out at the Oxford Resilient Buildings and Landscapes Laboratory (OxRBL) in the School of Geography and the Environment, University of Oxford. This laboratory allowed for a temperature and relative humidity stable environment for testing, as well as storage for the control blocks in the stone storage facility. The field analyses took place at Kelmscott Manor, Lechlade UK and Chipping Norton UK. Currently owned by the Society of Antiquaries of London (acquired 1962), the main house at Kelmscott Manor was built c. 1570 for Richard Turner, with later additions c. 1670, 1871, and 1896 (Historic England listing 1199373). The main building is built from uncoursed limestone rubble walls, and the boundary walls consist of coursed, roughly hewn limestone with lime mortar capping. Mosses grow luxuriantly at this site including at the base of north-facing walls (pleurocarpous), and on the tops of mortar-capped boundary walls (acrocarpous). Additionally, there is extensive pleurocarpous and acrocarpous moss growth on the roof tiles on all buildings at the site. There has been no regular cleaning or removal of mosses at this site because William Morris described Kelmscott as existing symbiotically with nature, and his philosophy for the buildings emphasized Kelmscott organically intertwining with nature over time. Kelmscott Manor was chosen as a research site due to the association with ‘rural’ and ‘countryside’ characteristics, as it was William Morris’ summer country house, and for William Morris’ own philosophy for the manor itself. The test area at the Chipping Norton site was a small area of dry-stone walling adjacent to the Grade I listed church, St. Mary the Virgin, located in the North-West area of Chipping Norton. The dry-stone walling provided a flat surface for testing, and also had small colonies of acrocarpous mosses. Again, the test area is comprised of local limestone, medium-grained and ooidal in nature (Chipping Norton Limestone).
Methods
Due to the historic nature of the sample sites, non-invasive and non-destructive test methods used in previous cultural heritage research have been adapted for this project (Viles, Sternberg, and Cathersides Citation2011; Eklund et al. Citation2013; Wilhelm et al. Citation2016).
This project uses a mixture of monitoring and direct analyses of moisture uptake and saturation to explore the controls on moss growth and their impacts on moisture relations. Microclimate monitoring took place at Kelmscott Manor, UK and Chipping Norton in summer 2019, using four iButton® (DS1923 Hygrochron Temperature/Humidity Data Logger) devices to monitor the microclimate differences between areas with and without moss cover (Tonon et al. Citation2019). At Kelmscott Manor, the devices were placed on south- and north-facing walls. Areas were chosen at high (1 m from base) and low (0.5 m from base) wall positions on the same wall, with the low wall devices placed directly next to moss colonization. The aim of this monitoring experiment was to determine the impact of aspect, relative humidity, and temperature on the colonization of mosses, and to determine if microclimatic conditions impacted the bioreceptivity of certain surfaces. It was theorized that cooler areas with higher relative humidity would be more receptive to moss growth than hotter areas with lower relative humidity.
Microclimate monitoring at Chipping Norton, UK took place over summer 2019 using a modified methodology to investigate the impacts of mosses on surface microclimates. Four iButton® devices were placed on a horizontal surface with two of the iButton® devices covered with B. rutabulum growth and two of the devices left bare. The aim of this monitoring experiment was to mimic the natural state of a moss-covered wall, and to determine if the presence of mosses impacted the temperature fluctuations and relative humidity of the underlying substrate in any way.
Field experiments were also devised to examine the surface moisture of areas covered with mosses compared to bare stone. In Chipping Norton, the handheld Protimeter (GE Protimeter Surveymaster™ dual-function moisture meter Model: BLD5360) device was used in resistance mode to simply measure the %Wood Moisture Equivalent (%WME) of areas of bare stone directly next to areas with moss colonisation. The moss-colonised areas beside the bare stone simply had the prongs pushed into the center of each growth with the prongs perpendicular to the substrate. Five repeat measurements were taken on each measured area, and average values calculated. In the laboratory environment, bare or moss-covered surfaces of sample blocks were measured with the Protimeter to determine the baseline ‘dry’ measurement. The surface of each sample was then flooded with 15 mL of water. The handheld Protimeter device was then used at periodic intervals of five minutes, with the surface measured five times in the same spot and using the same bedding direction (following the protocol of Eklund et al. Citation2013.) The measurements were repeated until the initial ‘dry’ measurement was reached or three hours had elapsed.
Karsten tube tests were used to compare the water absorption characteristics of areas covered with mosses, weathered stone without moss, and bare/unweathered stone (BS EN 16302 Citation2013) This is a well-established experiment within the field of cultural heritage used as a proxy measurement for water absorption (Blaeuer, Vergès-Belmin, and Franzen Citation2012; Hendrickx Citation2013; Vandevoorde et al. Citation2013; Scatigno et al. Citation2016a, Citation2016b; Ruiz, Flores, and Prieto Citation2019). The measurements took place on-site at Kelmscott Manor using the cementitious mortar capped wall shown in , at Chipping Norton using the top of the drystone walling constructed from local ooidal limestone, and in the laboratory environment using the limestone test blocks. This experiment measured the impact of both pleurocarpous and acrocarpous mosses. The Karsten tube tests used the standard method to measure water absorption, but also used a modified version of the implemented contact sponge method (i-CSM) method as described by Scrivano and Gaggero (Citation2017). In contrast to the i-CSM method, which uses a known weighted device to press a wetted sponge onto stone which is then weighed, the method used in this project used a wetted sponge with a known amount of water (3 mL) applied to a dry cushion of acrocarpous moss. A Karsten tube was then fitted over the wetted cushion of acrocarpous moss and then the test was conducted according to the standard. This method was used to measure the difference in Karsten tube results from areas which had moss colonisation (dry) and moss colonisation (wet).
This project analysed a large sample set of Karsten tube tests (total of N=82 tests.) The aim of using such a large sample set of tests was to determine the relative reliability and variance of Karsten tube tests on a variety of different substrates (weathered and unweathered limestone and cementitious mortar), and to investigate the possibility of using the i-CSM methodology on moss growth. The Karsten tube tests were applied to five different treatments of substrates (Unweathered no moss, Weathered no moss, Weathered moss present but removed, Weathered moss present, and Weathered moss present contact sponge method) to determine if there were any statistically significant differences in the volume of water absorbed.
Open porosity of sample blocks with and without moss growth was measured using the standard BS EN Citation1936 (Citation2006) in the laboratory. This test was modified from the standard as the sample blocks were not dried in the oven prior to the analyses, they were only left to acclimate to the lab environment for a period of one month before testing. This change was made because the live moss growing on the sample blocks would not have survived the oven drying period, and live moss was required for subsequent testing on the sample blocks.
Results
How does aspect and microclimate influence moss growth: Kelmscott Manor temperature and relative humidity data
At Kelmscott Manor, mosses were observed to grow primarily on roof structures, boundary walls, and at the base of structural walls. Shaded areas were more likely to have prevalent moss growths, as were north-facing walls and roof structures. Both acrocarpous and pleurocarpous mosses were observed to colonise Kelmscott Manor, with Brachythecium rutabulum at the base of structural walls, and Grimmia pulvinata (Smith 1807) and Homalothecium sericeum (Hedw.) Bruch, Schimp. and W. Guembel. on the tops of mortar-capped boundary walls, . Grimmia pulvinata and possibly Hypnum tectorum (Kindberg ex Brotherus 1907) were also observed on the roof tiles of all buildings at the site.
As expected, the influence of aspect on microclimate at Kelmscott Manor () shows the south-facing walls reaching higher temperatures than the north-facing walls. The absolute readings for the daily maximum reach much higher on the south-facing walls than the north-facing walls, and they also have much higher daily ranges. The S Wall High iButton® data in particular reached very high temperatures, much higher than commensurate readings of air temperature at the nearby Cirencester Met Office weather station (Location: 51.078, −1.994) at the same time and date.
Microclimate monitoring was undertaken at Kelmscott Manor, Gloucestershire (July-August 2019), and Chipping Norton, Oxfordshire (July-August 2019). Relative humidity drift is a common problem with iButton® Hygrochrons, especially in areas with higher relative humidity readings. In cases where values above 100% RH were recorded, they have simply been treated as 100% RH ().
Figure 2. Kelmscott Manor relative humidity microclimate monitoring (July–August 2019), influence of aspect and wall height on daily relative humidity fluctuations.
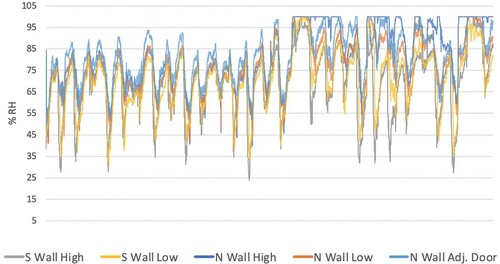
Figure 3. Kelmscott Manor temperature microclimate monitoring (July–August 2019), influence of aspect and wall height on daily temperature fluctuations.
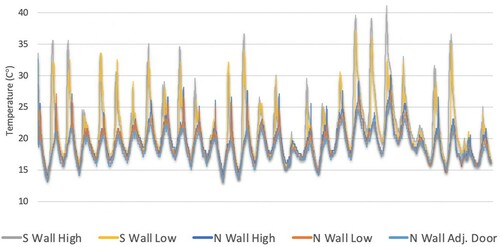
Figure 4. Chipping Norton relative humidity microclimate monitoring (July–August 2019), influence of biological cover on relative humidity fluctuations.
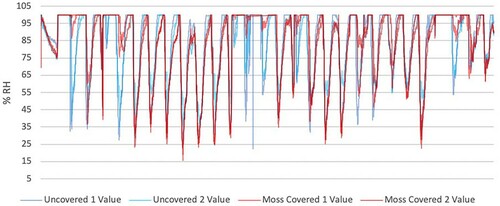
Figure 5. Chipping Norton relative humidity microclimate monitoring (July–August 2019), influence of biological cover on relative humidity fluctuations. Conditional formatting to indicate dry conditions (red) <70% RH and wet conditions (blue) 85% RH. Grey indicates invalid data.
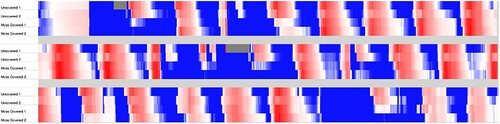
There appears to be a moderate effect of aspect and wall height on relative humidity fluctuations at Kelmscott Manor. As we can see in and from the daily fluctuations in relative humidity at this site, the relative humidity on the north-facing walls is relatively higher than that of the south-facing walls. We would expect to see wetter conditions on north-facing walls as they are exposed to fewer sunlight hours per day. Additionally, the north-facing walls are observed to have relatively more stable relative humidity than south-facing walls. These wetter and more stable conditions may encourage mosses to grow on north-facing walls. This typical growth pattern was observed at Kelmscott Manor, where the mosses primarily grew on north-facing walls and roof structures and were especially prevalent along the base of north-facing walls.
These findings may explain why mosses prefer to inhabit certain areas of Kelmscott Manor and not others. Other factors which would regularly affect bioreceptivity, such as substrate acidity and texture, and the amount of rainfall and airborne pollutants are fairly similar in all areas at Kelmscott Manor. Both the relative humidity and temperature data at Kelmscott Manor appear to suggest more favorable microclimates at the base of north-facing walls.
How does moss growth influence microclimate fluctuations: Chipping Norton relative humidity and temperature data
A short monitoring experiment explored the effect of moss coverage on the underlying substrate in Chipping Norton (July-August 2019) by comparing iButton® data on bare stone surfaces and directly adjacent areas with live moss growth placed on top of the iButton® devices.
As with the previous microclimate monitoring exercise, RH data >100% were transformed to 100% to remove any drift/ oversaturation values when the RH sensors become affected by liquid water. illustrates that both the uncovered and moss-covered areas were routinely saturated during rainfall events. Additionally, they appeared to reach higher relative humidity than recorded at Kelmscott Manor over the same period (), most likely due to the positioning of the iButton® devices. Whereas at Kelmscott Manor the devices were fixed to vertical walls, at Chipping Norton they were placed on flat horizontal limestone surfaces. The higher relative humidity readings at Chipping Norton may be due to the small iButton® aperture being flooded with water during rainfall events.
There was a statistically significant difference between groups (Uncovered 1, Uncovered 2, Covered 1, and Covered 2) as determined by one-way ANOVA (p = 0.000995, which is significant at p < 0.05). Here we see significant differences in the mean daily ranges in relative humidity between the four treatments over the monitoring period July-August 2019.
visualizes the relative humidity data from Chipping Norton using conditional formatting in order to illustrate the time lag in response between covered and uncovered areas. The horizontal bars represent the passage of time between July-August 2019, with red areas representing low RH% (<80% RH) and blue areas indicating high RH% (>80% RH). Here we can see a significant difference in the Covered and Uncovered iButton® devices in the time that it takes for moisture to reach the iButton® aperture, as well as the amount of time that it takes for each iButton® to reach ‘dry’ measurements. Since the uncovered devices have no surface covering they sense an increase in RH% very quickly. The covered ones, on the other hand, lag behind the uncovered ones. This is likely due to the moss covering the aperture on the iButton® and shielding it from the effects of light rain, dew, or mist. This shielding effect is particularly evident in the last few weeks in July 2019, where we can see that there were some periods of light rain. The uncovered devices have a longer duration of high RH% values, and the covered ones have a delayed and much shorter timespan in the high RH% range.
Conversely, the shielding effect of mosses on their substrates also appears to increase the duration of high RH% readings in periods of heavy rainfall. This is evident in beginning of July, middle of July, and end of August during the monitoring period. We can see that there is a delayed response from the covered iButton® devices in initially sensing the increase of RH%; however, once those areas become completely saturated (heavy rainfall events), they take much longer to dry than the uncovered devices.
In comparison to the RH% results from the uncovered and covered iButton® devices, there appears to be no such shielding effect with regards to temperature (). Both treatments result in broadly similar results, with the differences in average maximum, minimum, and daily temperature ranges being statistically insignificant.
Figure 6. Chipping Norton temperature microclimate monitoring (July–August 2019), influence of biological cover on temperature.
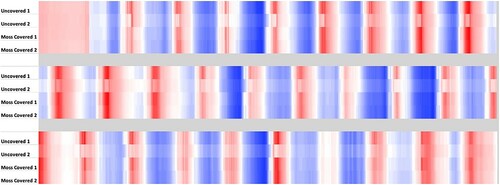
Overall, the results from this monitoring experiment showed that each iButton® showed different RH trends, with no significant difference in temperature. The moss-covered iButton® devices seemed to have lags in both wetting and drying after rainfall periods. The results from this experiment most likely underestimate moss impacts, as there had to be an air gap between the moss cover and the iButton®. Improvements in methodology in future experiments could provide further, more representative data.
How does moss growth influence water uptake and evaporation rates: moss data
This experiment compared the impact of moss growth on water retention and the evaporation rate on the weathered 15 cm Portland limestone cubes. By repeatedly weighing each cube or block over a 24-hour period, the amount of water retained by the block at different time periods was obtained by subtracting the initial dry weight. All blocks used in this experiment with the exception of Block 5 had been weathered in a natural environment. Although the sample size is small, the results show that the blocks with moss growth retain more water than the blocks without moss growth (). The blocks with moss growth initially absorb much more water, and also retain the water for a longer period of time, with Block 1 only reaching its initial dry weight after drying in the lab environment for 24 h.
Does moss growth influence water uptake at a surface: Protimeter data
These experiments investigated the influence of water uptake at the surface using the 15 cm cubes of weathered Portland stone. plots median values of surface moisture measurements taken in the laboratory environment before and after flooding the surface of blocks to determine the drying time for blocks with and without moss coverage. Standard errors were used to determine the whisker length. Jordan Basebed blocks covered with moss had high standard errors as each of the four blocks had varying degrees of biological growth coverage. Although blocks were chosen with similar amounts of moss coverage, they were not identical which may have led to a difference in the surface moisture levels.
Figure 8. Surface moisture measurements using capacitance (handheld Protimeter) in %Wood Moisture Equivalent comparing Bowers Roach and Jordan Basebed limestone blocks with and without moss growth (N = 8).
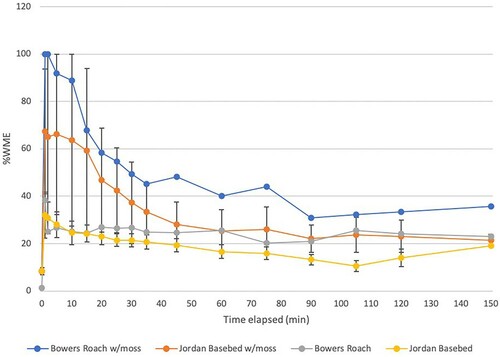
depicts a large difference in the amount of water initially taken up by both the Bowers Roach and Jordan Basebed blocks with moss growth and those without moss growth. Surface moisture levels peak and then drop quickly until around 30 min, when the measurements appear to stabilize somewhat, reaching their initial dry measurements 2.5 h after the initial surface flooding.
Does moss growth influence water uptake at subsurface level: Karsten test data
plots median values from all 82 Karsten tube tests, with standard errors used as the whisker values. These results were obtained in the laboratory environment using unweathered samples (N = 24), and in the field at Kelmscott Manor (N = 3) and Chipping Norton (N = 57). The unweathered Portland limestone samples in the laboratory environment absorbed the least amount of water during the tests. The substrates that absorbed by far the most water were the areas that had moss growth which had been manually removed before testing. Areas with moss (removed or otherwise) absorbed more water than areas without moss, whether these areas had been weathered or not. Additionally, certain areas of the ‘Weathered, no moss’ areas had lichen growth and these areas did not show any significant increases in water uptake in comparison to those that were weathered and had moss growth. This suggests that one of the underlying factors for water absorption is the growth of moss itself.
Figure 9. Karsten tube tests from field and lab (N = 82), showing water uptake from different substrate conditions.
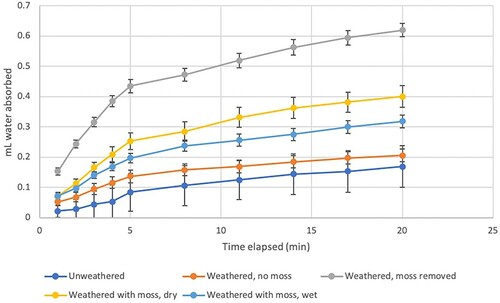
Additionally, one-way ANOVA using Karsten tube median values (5 treatments: Unweathered no moss, Weathered no moss, Weathered moss present but removed, Weathered moss present, Weathered moss present contact sponge method) gives a significant result at p < 0.05, with a p-value of <0.00001. We can see that there are significant differences in the 5 Karsten tube treatments, with significant differences between the unweathered substrates, and the weathered? substrates with and without moss.
Discussion
Limitations and scope of the project
The microclimate monitoring for this project was limited to a small area in England and done for a short period of time. For more robust findings, these experiments could be repeated and monitored for a longer period of time. This project focuses on limestone and cementitious mortar, and future research should investigate other material types such as slate and bricks. While the results show significant findings when regarding the moisture regimes of mosses and moisture regimes, more testing should be done to increase the confidence level. However, the methodology and analyses used in this project illustrate the suitability of non-destructive tests in the laboratory and field. Such tests are simple, easy to deploy, and can be tailored to different substrates. They are also suitable for many different environments and can be undertaken without disturbing the aesthetics of cultural heritage sites (for instance, long-term monitoring using iButton® devices is relatively discreet).
Recommendations for further research
This project showed some significant initial findings that could be explored further in future research. In particular, the differences between covered and uncovered areas and the impact on moisture beneath biological growths has potential to be explored in future projects. The relationship between mosses and moisture, while appearing straightforward, may be more complex than initially imagined. Further research particularly on the impacts of moss removal, moisture, and built heritage has the potential to be explored.
There is additional scope to evaluate the differences in moisture regimes when comparing the two distinct growth forms of mosses. This research project mostly examined the pleurocarpous growth type, defined as carpeting, with amorphous edges, and in particular the species B. rutabulum. This project also investigated the impact of acrocarpous mosses on water absorption through the Karsten tube tests, and future projects could further explore the impact of acrocarpous mosses and their circular, cushion-like forms. These two growth types are visually distinct from one another, and also have demonstrated differences in growth rates and patterns – therefore one might surmise the effect on substrates may be different. The influence of upright cushions and the absorption capabilities of water as compared to the flatter growth type is another question to be explored in future research.
Relevance to built heritage management strategies
Previous research and advice given to built heritage caretakers has suggested that mosses simply create wet environments, and that they also prefer wetter climates to flourish and grow. From the data presented in this paper, we can confirm that mosses prefer to inhabit wetter and darker environments, (differences in moss cover on North versus South walls at high and low levels at Kelmscott Manor). However, to say that mosses simply create wet environments, saturate their substrates, and cause moisture to linger on substrates may be overstating a more complex relationship. From the microclimate data at Chipping Norton which compared the results from bare stone and moss-covered stone, we can see that mosses may in fact shield the substrate from lighter periods of rain and moisture. Not only did the presence of moss delay the impact of relative humidity spikes, but in light rainfall events the presence of moss shortened the impact of moisture on the underlying substrate creating a drier underlying substrate than the bare surfaces.
When we further explored the impact of mosses on surface and subsurface water retention and uptake using a range of other methods, they fell broadly in line with previous research. Results from the water uptake and absorption tests (Karsten tube and modified BS EN Citation1936:Citation2006), as well as surface moisture retention (Protimeter experiment) showed that in all cases the presence of moss increased the overall amount of water absorbed and lengthened the amount of time for each sample to return to baseline moisture measurements. Whether by the mechanisms of their growth, such as their rhizoids or deposition of acidic metabolic by-products, or their overall morphology above the substrate surface, mosses appear to have an impact on the uptake and evaporation of water.
The results presented here have relevance for recommendations for moss management strategies and removal. For built heritage located in drier climates, the removal of mosses may not be necessary for the overall health and preservation of the site. Keeping in mind the results from the covered and uncovered microclimate monitoring experiment with iButton® devices at Chipping Norton, we could see a buffering effect that may be beneficial to buildings located in drier climates. Moreover, the growths appeared to have a negligible effect on surface temperature fluctuation and absolute temperature measurements, so fears regarding physical deterioration regarding temperature fluctuations may be allayed.
For built heritage located in wetter climates and for those with dramatic and invasive growths, the traditional advice may still apply. Despite this advice, the management strategy at Kelmscott Manor has conventionally followed William Morris’ conservation philosophy, which allowed the Manor to coexist symbiotically with the natural environment. Mosses and other species of lower plants have been allowed to colonise the roof structures, walls, and other structures at Kelmscott Manor and yet there do not appear to be any great deterioration or damp problems present in these areas.
The findings from this project showed evidence that the presence of mosses can prolong the effects of intense rainfall on their underlying surfaces () as well as increase overall water uptake (). In these cases the presence of mosses may be detrimental to the underlying substrates and may accelerate processes of deterioration. For areas with delicate decorations and any substrates that are sensitive to water, such as frescoes or wall paintings, particular care should be taken to prevent moss growth from occurring. However, it is important to keep in mind that moss growth will not occur unless the underlying environmental conditions are suitable. In most cases, controlling and monitoring the environmental conditions in the interior of built heritage will prevent mosses and other undesirable biological growths from growing. Results from the water uptake (Karsten tube) experiments also suggest that prevention of moss growth may be the best method to prevent further deterioration of the substrate through water absorption, since the findings indicate that the substrate with the highest amount of water absorption were the areas that previously had moss growth which had been manually removed. Once moss is established it may cause more harm than good to remove it.
Conclusions
Key findings
As suggested by previous research and literature, mosses prefer to inhabit areas that have higher RH% values, and smaller fluctuations in RH% and temperature.
Mosses may play a role in ‘shielding’ the underlying substrate from light rainfall events, while prolonging surface wetness in more intense rainfall events.
Mosses have an impact on water uptake and retention at both the surface and subsurface level.
The findings from this project suggest that the relationship between mosses and moisture is more complex than usually portrayed. From previous research, most sources suggested that the presence of mosses indicated that the underlying substrate was likely to be wetter than those substrates without mosses. The results gained from the experiments presented in this paper show that this may not be the case, and that in certain cases substrates covered with mosses may shelter underlying surfaces from brief rainfall events.
Additional tests (e.g. Karsten tube tests) illustrated that the impact of removing mosses may be more inherently damaging than leaving them in situ. These initial findings suggest that more research should be undertaken to fully understand the moisture regimes surrounding mosses and cultural heritage ().
Table 1. List of analyses, equipment, and sites: Does microclimate influence moss growth?
Table 2. List of analyses, equipment, and sites: How does moss growth influence moisture regimes?
Table 3. Average daily range %RH Kelmscott Manor (June–August 2019).
Table 4. Average daily temperature (C°) max, min, and range at Kelmscott Manor (June–August 2019).
Table 5. Average daily range %RH comparing covered and uncovered iButton® devices, Chipping Norton (June–August 2019).
Table 6. Average daily temperature (C°) max, min, and range at Chipping Norton, Covered vs. Uncovered (June–August 2019).
Disclosure statement
No potential conflict of interest was reported by the author(s).
Additional information
Funding
References
- Blaeuer, C., V. Vergès-Belmin, and C. Franzen. 2012. “Simple Field Tests in Stone Conservation.” In International Congress on the Deterioration and Conservation of Stone, 22–26. New York: Columbia University.
- British Bryological Society. 2010. “Mosses and Liverworts of Britain and Ireland. A Field Guide.” In British Bryological Society, edited by I. Atherton, S. Bosanquet, and M. Lawley. Plymouth: Latimer Trend & Co. LTD.
- BS EN 16302. 2013. “Conservation of Cultural Heritage. Test methods. Measurement of Water Absorption by Pipe Method.”
- BS EN 1936. 2006. “Natural Stone Test Methods. Determination of Real Density and Apparent Density, and of Total and Open Porosity.”
- Coe, K. K., and J. P. Sparks. 2014. “Physiological Ecology of Dryland Biocrust Mosses.” Photosynthesis in Bryophytes and Early Land Plants, Advances in Photosynthesis and Respiration 37: 291–308. doi:10.1007/978-94-007-6988-5.
- Douglas-Jones, R., J. J. Hughes, S. Jones, and T. Yarrow. 2016. “Science, Value and Material Decay in the Conservation of Historic Environments.” Journal of Cultural Heritage 21: 823–833. doi:10.1016/j.culher.2016.03.007.
- Edinburgh World Heritage: Historic Home Guide. 2019. Edinburgh World Heritage. https://ewh.org.uk/wp-content/uploads/2018/02/EWH-Roofs-Guide.pdf.
- Eklund, J. 2013. Biological Growth on Masonry: Identification and Understanding. INFORM Guide for Historic Environment Scotland. https://www.engineshed.scot/publications/publication/?publicationId=5057d101-9e6e-49e3-92b6-a595009defc6.
- Eklund, J., H. Zhang, H. A. Viles, and T. Curteis. 2013. “Using Handheld Moisture Meters on Limestone: Factors Affecting Performance and Guidelines for Best Practice.” International Journal of Architectural Heritage 7 (2): 207–224. doi:10.1080/15583058.2011.626491.
- Fontaine, L., R. Hendrickx, and H. Clercq. 2015. “Deterioration Mechanisms of the Compact Clay-Bearing Limestone of Tournai Used in the Romanesque Portals of the Tournai Cathedral (Belgium).” Environmental Earth Sciences 74: 3207–3221. doi:10.1007/s12665-015-4358-y.
- Furness, S. B., and J. P. Grime. 1982. “Growth Rate and Temperature Responses in Bryophytes: An Investigation of Brachythecium Rutabulum.” Journal of Ecology 70 (2): 513–523.
- Hendrickx, R. 2013. “Using the Karsten Tube to Estimate Water Transport Parameters of Porous Building Materials.” Materials and Structures 46: 1309–1320. doi:10.1617/s11527-012-9975-2.
- Historic Environment Scotland. 2005. Advisory Standards of Conservation and Repair for the Historic Environment in Scotland. https://www.historicenvironment.scot/media/5118/advisory-standards-repair.pdf.
- McIlroy de la Rosa, J. P., M. Casares Porcel, and P. A. Warke. 2013. “Mapping Stone Surface Temperature Fluctuations: Implications for Lichen Distribution and Biomodification on Historic Stone Surfaces.” Journal of Cultural Heritage 14 (4): 346–353. doi:10.1016/j.culher.2012.09.006.
- McIlroy de la Rosa, J. P., P. A. Warke, and B. J. Smith. 2012. “Lichen-induced Biomodification of Calcareous Surfaces: Bioprotection Versus Biodeterioration.” Progress in Physical Geography 37 (3): 325–351. doi:10.1177/0309133312467660.
- Morando, M., K. Wilhelm, E. Matteucci, L. Martire, R. Piervittori, and S. E. Favero-longo. 2017. “The Influence of Structural Organization of Epilithic and Endolithic Lichens on Limestone Weathering.” Earth Surface Processes and Landforms 42: 1666–1679. doi:10.1002/esp.4118.
- Morillas, H., M. Maguregui, E. Gallego-Cartagena, G. Huallparimachi, I. Marcaida, I. Salcedo, and F. Astete. 2019. “Evaluation of the Role of Biocolonizations in the Conservation State of Machu Picchu (Peru): The Sacred Rock.” Science of the Total Environment 654: 1379–1388. doi:10.1016/j.scitotenv.2018.11.299.
- Robinson, S. A., and M. J. Waterman. 2014. “Sunsafe Bryophytes: Photoprotection from Excess and Damaging Solar Radiation.” Advances in Photosynthesis and Respiration 37: 113–130. doi:10.1007/978-94-007-6988-5.
- Rotherham, I. D., and C. Griffiths. 2013. War and Peat. Landscape Archaeology and Ecology (10).
- Ruiz, L., V. Flores, and E. Prieto. 2019. “In Situ Assessment of Superficial Moisture Condition in Façades of Historic Buildings Using Non-Destructive Techniques.” Case Studies in Construction Materials 10: 1–14. doi:10.1016/j.cscm.2019.e00228.
- Scatigno, C., S. Gaudenzi, M. P. Sammartino, and G. Visco. 2016a. “A Microclimate Study on Hypogea Environments of Ancient Roman Building.” Science of the Total Environment 566–567: 298–305. doi:10.1016/j.scitotenv.2016.05.050.
- Scatigno, C., C. Moricca, C. Tortolini, and G. Favero. 2016b. “The Influence of Environmental Parameters in the Biocolonization of the Mithraeum in the Roman Masonry of Casa di Diana (Ostia Antica, Italy).” Environmental Science and Pollution Research 23: 13403–13412. doi:10.1007/s11356-016-6548-x.
- Scrivano, S., and L. Gaggero. 2017. “Non-Invasive Analytical Technique to Address Water Uptake on Stone Surfaces: The Implemented Contact Sponge Method (i-CSM).” Journal of Cultural Heritage 28: 9–15. doi:10.1016/j.culher.2017.05.013.
- Slavík, M., J. Bruthans, M. Filippi, J. Schweigstillová, L. Falteisek, and J. Řihošek. 2016. “Biologically-Initiated Rock Crust on Sandstone: Mechanical and Hydraulic Properties and Resistance to Erosion.” Geomorphology 278: 298–313. doi:10.1016/j.geomorph.2016.09.040.
- Smith, B. J., and S. McCabe. 2008. “Understanding the Decay of Stone-Built Cultural Heritage.” Progress in Physical Geography 32 (4): 439–461. doi:10.1177/0309133308098119.
- Tonon, C., S. E. Favero-Longo, E. Matteucci, R. Piervittori, P. Croveri, and L. Appolonia. 2019. “Microenvironmental Features Drive the Distribution of Lichens in the House of the Ancient Hunt, Pompeii, Italy.” International Biodeterioration and Biodegradation 136: 71–81. doi:10.1016/j.ibiod.2018.10.012.
- Urmi, E., and N. Schnyder. 2007. “Mapping Bryophytes: A Review.” Lindbergia 33: 40–54.
- Vandevoorde, D., V. Cnudde, J. Dewanckele, L. Brabant, D. Bouw, V. Meynen, and E. Verhaeven. 2013. “Validation of in Situ Applicable Measuring Techniques for Analysis of the Water Adsorption by Stone.” Procedia Chemistry 8: 317–327. doi:10.1016/j.proche.2013.03.039.
- Viles, H., T. Sternberg, and A. Cathersides. 2011. “Is Ivy Good or Bad for Historic Walls?” Journal of Architectural Conservation 17 (2): 25–41.
- Wilhelm, K., H. Viles, E. Winter, O. Burke, S. Engelstaetter, and K. Z. Coyte. 2016. “Catastrophic Limestone Decay at the Central Sanctuary of Iupiter Dolichenus at Dülük Baba Tepesi in South Turkey: Causes and Implications for Future Conservation.” Conservation and Management of Archaeological Sites 19: 3–29. doi:10.1080/13505033.2016.1291025.