ABSTRACT
Smalt is a blue cobalt-coloured glass pigment used in European wall paintings from the early Renaissance period that became a popular choice for artists due to its unique tone, availability, and versatility. The pigment has long been considered as stable in an alkaline environment since it was applied with lime-based techniques and generally retained its colour over time. This perceived stability has meant that aqueous-based alkaline treatments, often involving high pH values, substantial quantities of liquid, and long contact times, have been used in conservation treatments. However, studies of the kinetics of glass deterioration in the past few years have demonstrated that glass is highly reactive in contact with water and substances with both high and low pH values. Research into smalt deterioration in canvas paintings has shown that smalt is susceptible to elemental leaching and physical changes in contact with water and low pH values; however, there is little specific information about the conservation implications of these findings on the treatment of smalt in wall paintings. This experimental study explores several aspects of how aqueous-based alkaline conservation treatments affect smalt grains in lime-based wall paintings: the influence of painting technique, exposure of the pictorial layer to water before treatment, and pH values and contact times during treatment. Replicas representing three different painting techniques were created, exposed to liquid water or water vapour, and treated with three substances commonly used in conservation according to accepted practices in terms of preparation and application. Chemical and physical changes in the pigment were then analysed using scanning electron microscopy with energy dispersive X-ray spectroscopy. Results confirmed that aqueous-based alkaline substances provoke chemical changes as well as physical deterioration in smalt applied in lime-based techniques evidenced by leaching, corrosion, and cracking in the pigment grains. Crucial factors found to influence the impact of treatments on smalt included the previous exposure to humidity of the pictorial layer, the pH value of the treatment, and extended contact times.
Introduction
Smalt is a blue glass pigment that owes its colour to the use of cobalt as a colouring agent. Cobalt-coloured glasses are known from ancient times, with evidence found of their manufacture and use dating back to Mesopotamia and ancient Egypt (Reade, Freestone, and Simpson Citation2003; Delamare Citation2013). However, there are no examples of cobalt glass used as a pigment from the ancient world and there is no existing information about blue glass production in the early treatises on art and technology from the Middle Ages (Freestone Citation1992; Frezzato Citation2011).
The earliest known examples of smalt in European wall paintings date to about 1400 (Delamare Citation2013). By the beginning of the sixteenth century, it was being used in wall paintings by some of the period’s greatest artists, including: Ghirlandaio, Michelangelo, Raphael, Ferrari, Luini, and Correggio (Gallone Citation1991; Barucci Citation2002; Bandini Citation2002; Bersani et al. Citation2010) and was considered as a versatile and stable blue pigment that could be used with lime-based techniques in wall paintings (Mühlethaler and Thissen Citation1993; Merrifield Citation2004). The other principal blue pigments of the period were azurite and ultramarine (Cadogan Citation2002; Eastaugh et al. Citation2004; Frezzato Citation2011; Delamare Citation2013), however neither was ideal in lime-based paintings. Ultramarine could be applied affresco, however, it was generally more sparingly used in wall paintings as it was costly (Plesters Citation1993; Bandini Citation2002; Merrifield Citation2004). Azurite was considered unstable in the alkaline environment and was generally applied a secco with an organic binder (Gettens and Fitzhugh Citation1993; Barucci Citation2002; Merrifield Citation2004; Frezzato Citation2011).
In the past few years, scholarly and scientific study has collectively helped to define general issues connected with the identification, use, and deterioration of smalt in wall paintings. But it has also highlighted the need for further research specifically relating to conservation treatments used on smalt in wall paintings for several reasons: first, most studies about smalt originate from the field of canvas paintings which have different physical and elemental characteristics than lime-based wall paintings; second, studies from the field of glass have highlighted its sensitivity to both acidic and basic solutions; third, treatments used on wall paintings frequently involve the use of aqueous-based solutions with high pH values and long contact times; and fourth, the impact of treatments on the pigment is primarily evaluated based on a visual examination of the surface for colour change.
The composition of historical smalt
Smalt was created through the combination of cobalt minerals, silica, and a flux (an alkali oxide, principally potassium) resulting in a complex material including impurities associated with the raw materials, and/or intentionally added modifiers and/or stabilizers (Gratuze et al. Citation1995; Cagno et al. Citation2008; Delamare Citation2013; Janssens et al. Citation2016; Seccaroni and Haldi Citation2016). ‘Recipes’ and methods of production are recorded in treatises and documents from the sixteenth century onwards (Salerno and Ferroni Citation1999; Santopadre and Verità Citation2006; Borgia and Seccaroni Citation2007).
The elemental composition of historical smalt varies and is usually reported as ranges: silica between 66–72 or 65–71 (wt.% SiO2); potassium (K) between 10-21, 16-21, or 5–40 (wt.% K2O); and cobalt (Co) between 6-7, 2-18, or 5–40 (wt.% CoO) (Giovanoli and Mühlethaler Citation1970; Boon et al. Citation2001; Santopadre and Verità Citation2006; Borgia and Seccaroni Citation2007; Daniilia and Minopoulou Citation2009; Robinet et al. Citation2011b; Berrie Citation2015; Panighello et al. Citation2016). The composition of glass directly impacts its physical qualities and stability (Robinet et al. Citation2013). Historical smalt is not a standard material and there are sometimes significant differences in composition and stability of ‘smalts’ from the same period.
Triggers and mechanisms of glass deterioration
In the past 50 years, many studies have focused on glass deterioration, often originating in the fields of industrial glass or manufacturing. This research has shown that glass is a reactive material that deteriorates in primarily two different ways depending on availability of water, the composition of the glass, and the pH of the solution with which it is in contact. The first process, ‘leaching’, ‘lixiviation’, or ‘selective dissolution’ begins with the adsorption and diffusion into the glass of molecular water (Dal Bianco et al. Citation2005; Santopadre and Verità Citation2006; Kuniki-Goldfinger Citation2008), followed by an ion-exchange in which primarily alkali ions (K+ and Na+) are replaced by hydrogen (H+) or hydronium (H3O+) ions (Boon et al. Citation2001; Sterpenich and Libourel Citation2006; Kuniki-Goldfinger Citation2008). This reaction generally occurs in solutions with a pH value of <9, yet the exact pH value at which it occurs is not fixed but varies based on the composition of the glass and environmental conditions (Gentaz et al. Citation2011). The loss of the alkali ions through the ion exchange process leads to the development of an alkali-depleted zone often called a ‘silica gel layer’ (Messiga and Riccardi Citation2006; Santopadre and Verità Citation2006; Kunicki-Goldfinger Citation2008; De Ferri et al. Citation2012), generally defined as an open and porous silica network (Melcher and Schreiner Citation2006; Kuniki-Goldfinger Citation2008; Schalm et al. Citation2009; Gentaz et al. Citation2011) or as a micro-cracked zone (Tournié et al. Citation2008).
The second deterioration process is ‘congruent dissolution’, ‘corrosion’, or ‘etching’ that initiates when a substance with a pH value of >9 comes into contact with glass and the Si-O bonds in the glass structure are disrupted by hydroxide (OH–) ions causing the glass to dissolve (El-Shamy and Douglas Citation1972; Freestone Citation1992; Santopadre and Verità Citation2006; Vilarigues and de Silva Citation2006; Kunicki-Goldfinger Citation2008; Tournié et al. Citation2008; Gentaz et al. Citation2011; Crundwell Citation2014). Pitting and corrosion precede an overall breakdown of the glass surface (El-Shamy and Douglas Citation1972; Santopadre and Verità Citation2006; Gentaz et al. Citation2011). Other constituent elements of the glass are also released as the silica structure is dissolved (Boon et al. Citation2001; Spring et al. Citation2005).
The deterioration of smalt
The most intensive research about smalt in the past few years has come from the field of canvas paintings where questions relating to visible colour changes were noted. Findings have revealed insight into the structure of cobalt ions, where each cobalt (Co2+) ion is positioned at the centre of a coordination complex featuring a tetrahedral formation with four oxygens, balanced by two alkali ions that neutralize the electrical charge. This tetrahedral coordination creates the characteristic colour in cobalt glasses, where the Co2+ ion is best stabilized by potassium (K+) (Boon et al. Citation2001; Borgia and Seccaroni Citation2007; Robinet et al. Citation2011a; Delamare Citation2013; Berrie Citation2015; Janssens et al. Citation2016).
Research has furthermore expanded the understanding of triggers, mechanisms, and kinetics of physical and elemental changes, and has highlighted the crucial role of water and pH values on deterioration (Boon et al. Citation2001; Altavilla and Ciliberto Citation2004; Spring et al. Citation2005; Robinet et al. Citation2011a, Citation2011b, Citation2013; Janssens et al. Citation2016). The crucial chemical balance that guarantees the colour of smalt is affected by the loss of alkali during leaching which occurs in the presence of water or oil in the pH <9 environment. When alkali ions are lost, the coordination structure of the Co2+ ions shifts from tetrahedral to octahedral, resulting in a subsequent change in the optical qualities of the pigment (Robinet et al. Citation2011a; Cianchetta et al. Citation2012; Robinet et al. Citation2013; Janssens et al. Citation2016). Boon et al. (Citation2001) determined that the threshold for a perceptible shift in the optical properties of the pigment in deteriorated smalt occurred when the ratio of Co:K is 1:1. The physical structure is also affected by shrinkage in the glass network due to the relative differences in size between the alkali ions (K+ and Na+) and the H+ or H3O+ ions that replace them (Kunicki-Goldfinger Citation2008), and the creation of a structurally unstable gel layer susceptible to hydration and dehydration cycles with the associated expansion and contraction of the layer leading to cracking (Santopadre and Verità Citation2006; Cagno, Janssens, and Mendera Citation2008; Kunicki-Goldfinger Citation2008; Tournié, Ricciardi, and Colomban Citation2008).
This research primarily addresses the underlying causes and mechanisms of colour change in smalt on microscopic and macroscopic levels in canvas paintings. The pictorial layer of canvas paintings is characterized by pH values in the acidic range and water is generally used in small quantities both in original painting technique and in conservation. Conversely, the pictorial layer in wall paintings is generally in the alkaline range of pH values, and there is substantially more water used both in the original painting technique and in conservation treatments.
There has only been a limited number of studies published about smalt in lime-based wall paintings. Studies published by Ajò et al. (Citation2004) and Santopadre and Verità (Citation2006) remain the main sources of information in the literature today, with other studies addressing smalt applied with other techniques in wall paintings (Daniilia and Minopoulou Citation2009; Bersani et al. Citation2010, Citation2014). Although smalt has been considered stable in wall paintings, changes in the tone of surfaces are noted in the literature but are often inconsistent and varied: some describe them as discoloured, turning grey, appearing lighter, darker, or ‘weathered’ (Ajò et al. Citation2004; Santopadre and Verità Citation2006; Daniilia and Minopoulou Citation2009). Santopadre and Verità (Citation2006) address the deterioration of smalt on a microscopic level in the alkaline environment of a lime-based wall painting and they raise questions about the implications for conservation, however, specifically addressing the issue of aqueous-based alkaline conservation treatments was beyond the scope of the study.
Alkaline substances used in conservation treatments
Moderately to highly alkaline substances are regularly used in the treatment of wall paintings executed with lime-based techniques since they are considered to be compatible with the constituent materials of the painting; calcium hydroxide (Ca(OH)2) has a pH of ≅12.6 at 20°C (National Lime Association Citation2007), and a lime-based painting after carbonatation has a pH value of around 8 (Mattei et al. Citation2008). Most pigments traditionally used in lime-based wall paintings are considered stable in high pH values. Pigments sensitive to the alkaline environment, such as copper-based blues and greens were generally applied a secco with organic binders such as casein, egg, or glue to avoid alteration (Eastaugh et al. Citation2004).
The most common reagents used in conservation treatments are ammonium bicarbonate (NH4HCO3, pH ≅ 8.2) and ammonium carbonate ((NH4)2CO3, pH ≅ 9.2), to remove unwanted material (intentionally applied such as coatings and previous conservation treatments, and unintentionally deposited such as dirt, dust, and soot), and barium hydroxide (Ba(OH)2, pH ≅ 13) to address issues with salts and decohesion of the wall support.
Research objective of this project
Since smalt does not generally change colour when applied with lime-based techniques and smalt surfaces generally do not exhibit colour changes after treatment, aqueous-based alkaline treatments have been widely considered as safe and effective. Yet studies about smalt and glass deterioration clearly demonstrate how glass in contact with high pH solutions results in a loss of material, rather than in a change in colour. Thus, evaluation of treatment based on visual examination is not sufficient. Additionally, treatments usually involve using large quantities of water and long contact times. The proven reactivity of glass in water brings into question the consequences of these types of treatments on the glass structure of the pigment.
The present research investigates the physical and elemental impact of aqueous-based alkaline conservation treatments on smalt in lime-based wall paintings, and the potential contribution of pH values, methods of application, and contact times used during conservation treatment to the effect on the pigment. The primary purpose of this experiment was to try to arrive at preliminary results that were indicative of the behaviour of the applied pigment in response to the exposure to water and to alkaline conservation treatments. Since the number of variables in the study of smalt in wall paintings is great (considering the multitude of painting techniques and different types of water exposure that can be found in wall paintings in situ), one of the purposes of this study was to isolate some of the variables to identify situations (or combinations of variables) that both reflected some of the deterioration seen in authentic case studies, but also to identify combinations that appear to provoke the most severe responses. In this way, more focused research can proceed in the future (such as to determine more precisely the impact of different contact times and concentration of solutions).
Materials and methods
Nine replicas were prepared following information reported in the literature and observed in wall paintings from the sixteenth century in Canton Ticino, Switzerland. The replicas were prepared on terracotta tiles (40 × 19 × 5 cm) consisting of an arriccio base layer, and an intonaco layer composed of lime putty (CTS ‘Grassello di Calce’ Code 01191500/573; pH value 12.20 measured with a Hach portable pH meter P4C301) and sand (arriccio 0-4 mm; intonaco 0-1 mm). Three different lime-based painting techniques were chosen and are described based on the state of the intonaco (the final plaster layer) at the time at which it was painted: Technique A – Paint applied to a freshly laid intonaco; Technique B – Paint applied on partially set intonaco six hours after it was applied; and Technique C – Paint applied on an intonaco that had set for 90 days. The differentiating factors amongst the lime-based techniques are primarily the humidity in the system and the degree to which the intonaco was set at the time of paint application.
The tiles were all painted using the same commercially produced smalt (‘Kremer “Smalte” <120 µm’), mixed with deionized water, lime water, and lime putty. The elemental composition of historical smalt varies considerably in terms of the concentrations of the main constituent elements (K, Co, and silica) and grain size, whereas modern industrial pigments have a more consistent composition and grain size. The average chemical composition of the Kremer pigment obtained on several grains using SEM-EDX is the following: SiO2 = 70 wt%; Na2O = 13 wt%; K2O = 10 wt%; Al2O3 = 1 wt%; CoO = 6 wt%. The grain size value range is between 60 and 120 µm. This pigment was chosen for the experiment to make it more standard and reproduceable. Although the actual composition of the Kremer pigment differs somewhat from a historical smalt (in that it has a generally higher concentration of Na), its behaviour in response to water exposure and treatment give indicative results due to its nature as a glass.
All replicas were allowed to set for at least eight months after painting (a minimum of 240 days) to ensure carbonatation, with the exact number of days depending on the technique and type of subsequent exposure. Carbonatation was checked using phenolphthalein (C20H14O4) as an indicator to gauge alkalinity, applied to a freshly exposed cross section of replica wet with deionized water.
Preliminary exposure to water
Water is always present in wall paintings in different forms, and it has been demonstrated that glass is highly reactive with water in any form, and thus even small amounts of water (even atmospheric water) can have an effect (Carmona et al. Citation2006; Vilarigues and de Silva Citation2006; Gentaz et al. Citation2011). For this reason, the replicas were exposed to water before treatment. The replicas were divided into three sets, with each set containing one replica representing each of the three techniques (A, B, C as described in ‘Materials and Methods’). Set 1 (Replicas 1A, 1B, 1C) was kept as a control; Set 2 (Replicas 2A, 2B, 2C) was exposed to liquid water to simulate rising damp conditions; and Set 3 (Replicas 3A, 3B, 3C) was exposed to water vapour in a high RH environment (see ).
Table 1. Conditions and procedure for exposure of replicas to water in different forms.
Treatment
Three aqueous-based, alkaline substances commonly used in conservation were chosen: ammonium bicarbonate, ammonium carbonate, and barium hydroxide. Although there are other substances used in wall painting conservation, these were chosen because they represent a range of basic pH values, and because they are aqueous-based and commonly applied in poultices with high liquid content with long contact times. The substances were prepared as follows and pH was measured (with a Hach portable P4C301 pH-meter at room temperature, 25°C) and applied in cellulose pulp (Arbocel® 200) on Japanese paper: Section I of each replica was left as a control (no treatment applied); in Section II, deionized water [H2O; pH 7.6] was applied with a contact time of 6 h; in Section III, ammonium bicarbonate [NH4HCO3; pH 8.2] was prepared at a 10% concentration in deionized water and was applied with a contact time of 1 h; in Section IV, ammonium carbonate [(NH4)2CO3; pH 9.2] was prepared at a 10% concentration in deionized water and was applied with a contact time of 1 h; in Section V, ammonium carbonate [(NH4)2CO3; pH 9.2] was prepared at a 10% concentration in deionized water and was applied with a contact time of 6 h. After 15 days, a pause which is recommended in the literature (Matteini Citation1991, Citation2010; Bandini et al. Citation1986), samples were taken, then barium hydroxide [Ba(OH)2; pH 13] was prepared at a 6% concentration in deionized water and was applied with a contact time of 6 h. All replicas were left to set for several weeks before samples were taken for analysis. shows a summary of pre-treatment exposure and treatment of replicas.
Table 2. Organization of replicas by technique, water exposure (pre-treatment), and treatment.
Evaluation
To provide a context for microscopic data, surfaces of all replicas were investigated in direct and raking light, and with a digital hand-held microscope to observe: the tone of the observed area; morphology of the surface; signs of surface deterioration and losses; and the size of pigment grains and their distribution in the pictorial layer.
Samples measuring 1–4 mm were taken from each section of all the replicas (for a total of 54 samples), mounted in SpeciFix™ epoxy resin, and prepared as cross-sections by hand using a wet ground method with a variable speed rotary disc and increasingly fine papers. Samples were checked with an optical microscope under visible (Vis) incident light in bright-field (to ensure the exposure of the face of the sample, to check the degree of polish and for possible residue from preparation, and to verify that the sample featured an appropriate group of smalt grains). Before graphite coating, the cross-sections were cleaned using compressed air followed by an ethanol swab. They were then carbon coated and examined with a field emission gun Tescan Mira 3XMU-series FE-SEM equipped with an EDAX microanalytical system. Operating conditions were as follows: accelerating voltage 20 kV, beam current 40 mA, with a working distance of 15.8 mm.
Samples were observed to define stratigraphy and distribution of grains. As it would not have been possible to observe the same applied smalt grains before and after treatment, it was decided that the most important variable to consider was the treatment of applied pigment, and so samples were taken from the replicas before and after treatment and were prepared in the same manner to establish continuity in the overall group of samples considered. Individual smalt grains were evaluated based on their physical appearance and elemental characteristics. Evidence of cracking and signs of corrosion were noted. Grains demonstrating a uniform physical appearance with a uniform elemental composition based on SEM-EDX measurements were considered intact. Grains featuring visibly differentiated core and rim areas in backscattered electron mode (BSE), and confirmed by SEM-EDX readings were considered ‘zoned’.
Results
Surface evaluation after water exposure
All replicas were exposed to water until they exhibited a change in the colour or tone of the surface that was visible to the naked eye. These changes were evaluated based on a visual inspection for two reasons: first, theoretically the deterioration of smalt in contact with water is tied to leaching behaviour resulting in a change in the appearance of the glass (in terms of tone and intensity). It has been shown that at a certain point once enough grains have altered, there is a visible change in tone on the painted surface (Boon et al. Citation2001; Cianchetta et al. Citation2012); and second, the evaluation of the deterioration of smalt in wall paintings is often determined through a visual inspection and analysis of the surface, so monitoring visible changes is important.
In the present experiment, the most significant surface changes after exposure to water were noted in Replicas 2A, 2B, and 2C (all techniques exposed to liquid water); Replicas 1C, 2C, and 3C (paint applied on set plaster) surfaces demonstrated the most striking changes in tone and general homogeneity of the paint layer, followed by Replicas 1B, 2B, and 3B (paint applied to partially set plaster). The surfaces of Replicas 1A, 2A, and 3A (paint applied to fresh plaster) demonstrated the least visible change.
Microscopic investigation of samples
The pictorial layer in all the replicas measured between 100 and 200 µm and featured smalt grains measuring 60–120 µm. Differences in the stratigraphy of the samples attributed to technique were as expected: the samples from Replicas 1A, 2A, and 3A (paint applied to fresh plaster) showed a material continuity between the intonaco and pictorial layer; samples from all other replicas showed a developed carbonatation line. The main physical and elemental deterioration phenomena that were noted in all samples were zoning, cracking, and corrosion. The phenomena in were observed both individually and in different combinations.
Table 3. Observed deterioration phenomena in smalt grains.
A methodology for using SEM-EDX data to understand the process of smalt deterioration through mapping the ratio of elemental concentrations is presented in the literature (Boon et al. Citation2001; Daniilia and Minopoulou Citation2009; Robinet et al. Citation2011a). SEM-EDX readings from this experiment were examined using the following ratios: Na2O/SiO2; K2O/SiO2; CoO/SiO2.
Section I: control
Samples were taken from the control sections of all replicas, representing the three different painting techniques (A, B, C) and exposure to two different forms of water (liquid or vapour) as summarized in . These results served as an indicative baseline for the appearance of smalt as applied for each replica. The pigment grains, in all the painting techniques represented (A, B, C), demonstrated a first stage of deterioration that developed around the edges of the grains as coronitic microtextures (). The composition of the singular grains shows a consistent trend with the ratio of alkali/silica remaining between 0.2 and 0.15 from the centre of the grain (0 µm) to the border of the unaltered portion of the grain, that then diminishes sharply in correspondence with the altered rim ((D–F)).
Figure 1. Coronitic microtextures in smalt grains from Section I in replicas from set 1 (no water exposure pre-treatment). A: Replica 1A.I Freshly applied intonaco; B: Replica 1B.I Partially set intonaco; C: Replica 1C.I Set intonaco; D: Compositional profile of the grain in A; E: Compositional profile of the grain in B; F: Compositional profile of the grain in C.
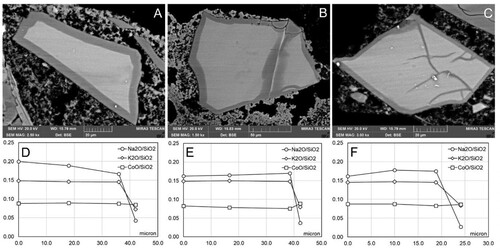
Section II: deionized water (contact time 6 h)
One section of each replica was treated with a poultice of deionized water for six hours (6 h) to evaluate whether there was a reaction in the pigment to water alone, and to compare it to aqueous-based alkaline substances and their effect on applied smalt. The results demonstrated that this treatment does not bring about evident changes in the microtexture of the smalt grains, but it is possible to note a change in the chemical composition in the pigment that presents as a variation in the Na content compared to the control replicas as shown in the compositional profiles from the centre (0 µm) towards outer edge of the grains ((C–E)). In particular, the Na varies from 0.20–0.15, and in some cases, tends towards zero. For the grains in which the value of Na tends towards zero, the coronitic microtexture is no longer present. This type of treatment could be considered as selective in terms of the leaching of alkalis since the K content remains almost unchanged. The observed behaviour is the same for the three different painting techniques.
Figure 2. Examples of microtextures in smalt grains treated with deionized water from Section II (Replica 1A: freshly applied intonaco; no water exposure pre-treatment). A: Smalt grain with a thin coronitic microtexture (1) and an apparently intact grain (2); B: Smalt grain with notable coronitic microtexture and development of a network of microcracks (3); C: Compositional profile of grain 1 in A; D: Compositional profile of grain 2 in A; E: Compositional profile of the grain in B.
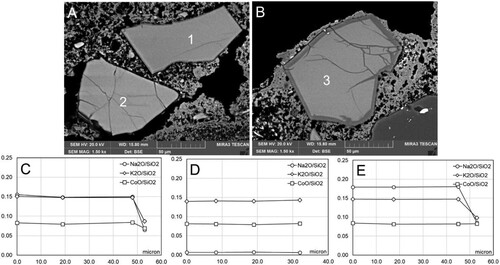
Section III: ammonium bicarbonate (contact time 1 h)
No significant difference was noted amongst the grains in the different replicas. The smalt grains examined from the sections treated with ammonium bicarbonate for one hour (1 h) exhibited a fair amount of zoning (). Pigment grains were observed that featured coronitic microtextures of different thicknesses as demonstrated in compositional profiles measured from the centre (0 µm) towards the outer edge of smalt grains ((D–F)), and apparently intact grains were noted, in which there was a marked reduction in the content of Na (). This situation is very similar to that which was observed in samples from Section II that were treated with deionized water. A marked number of grains demonstrated different types of cracking. Some exhibited cracks (Type I, ) located in the rim zone in zoned grains. Others showed the first signs of tiny cracks (Type III, ) in the central area of the glass. This suggests that the presence of water vapour activates the corrosion process on the edges of the pigment grains and the signs of this process first manifest as irregularities in the edges of the grain, before resulting in the appearance of microfractures and secondary porosity. In terms of the chemical composition of the grains, a slight loss in alkalis was noted; this initiates in the centre of the grain and extends to the outer edge of the grain, where the loss is more noticeable as shown in the compositional profile below ((B)).
Figure 3. Examples of microtextures in smalt grains from Section III (Replica 1B: partially set intonaco; no water exposure pre-treatment). A: Grain with slightly developed rim; B: Grain with well-developed rim; C: Apparently intact grain; D: Compositional profile of smalt grain in A; E: Compositional profile of smalt grain in B; F: Compositional profile of smalt grain in C.
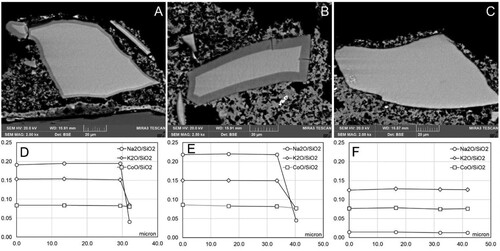
Section IV: ammonium carbonate (contact time 1 h)
There was no significant difference noted between the grains in the different replicas. Most of the smalt grains in the sections treated with ammonium carbonate (1 h) exhibited coronitic microtextures () or were shown through SEM-EDX to have lost their Na content as demonstrated in the compositional profile from centre (0 µm) towards outer edge of the smalt grains ((C,G,H,I)). Grains exhibited cracks (Type I, ) in the outer band in zoned grains as well as tiny cracks (Type III, ) in the central zone of the glass. The treatment with ammonium carbonate on replicas previously exposed to water vapour produced an initial corrosion around the edge of the grain, accompanied by microfractures and concentric compositional zoning. The compositional transverses shown in the diagrams of clearly demonstrate the variation of alkali content in the grains that diminish from the centre towards the outer edge of the grains ((C,G,H,I)).
Figure 5. Examples of microtextures in smalt grains from Section IV in different replicas. A: Replica 1B (partially set intonaco; no water exposure pre-treatment); B: Replica 1B (partially set intonaco; no water exposure pre-treatment); C: Compositional profile of smalt grain in B; D: Replica 2B (partially set intonaco; exposure to liquid water); E: Replica 2B (partially set intonaco; exposure to liquid water); F: Replica 3B (set intonaco, exposure to liquid water); G: Compositional profile of smalt grain in D; H: Compositional profile of smalt grain in E; I: Compositional profile of smalt grain in F.
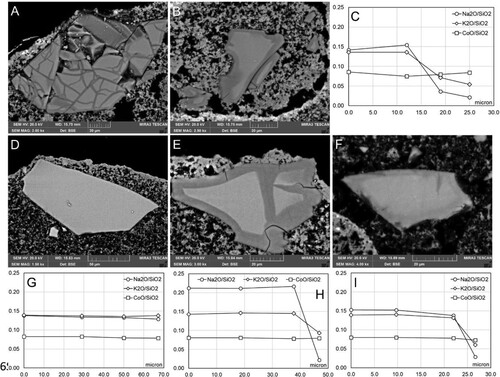
Section V: ammonium carbonate (contact time 6 h) and ammonium carbonate (contact time 6 h)/barium hydroxide (contact time 6 h)
Almost all of the smalt grains in the sections treated with ammonium carbonate (6 h) exhibited either zoning or a loss of only Na from the entire grain as demonstrated in compositional profiles from the centre (0 µm) towards outer edge of smalt grain ((C,D)). The grains that demonstrated an overall loss of Na had a mottled appearance, but no zoning. Grains with zoning and marked reduction in both K and Na exhibited cracks (Types I, II, and III, ) in the glass structure. Corrosion around the edges of the grains was noted in all samples ().
Figure 6. Examples of microtextures in smalt grains from Section V in different replicas. A: Replica 3A (freshly applied intonaco; exposure to water vapour) Section V.a (ammonium carbonate 6 h); B: Replica 3A (freshly applied intonaco; exposure to water vapour) Section V.a (ammonium carbonate 6 h); C: Compositional profile of smalt grain in A; D: Compositional profile of smalt grain in B; E: Replica 3B (partially set intonaco; exposure to water vapour) Section V.a (ammonium carbonate 6 h); F: Replica 3B (partially set intonaco; exposure to water vapour) Section V.b (ammonium carbonate 6 h/barium hydroxide 6 h); G: Compositional profile of smalt grain in E; H: Compositional profile of smalt grain in F.
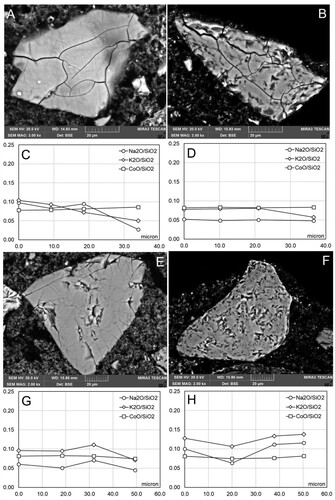
Smalt grains in the samples treated with ammonium carbonate (6 h) and barium hydroxide (6 h) exhibited a wide variety of deterioration phenomena with all grains either demonstrating zoning or an overall loss of Na. All types of corrosion (I, II, and III, ) were noted. Smalt grains from replicas that had previously been exposed to high RH values exhibited a notable overall physical and chemical change involving a physical breakdown of the glass structure ((G,H)). The process active in the transformation of the pigment grains is the corrosion which brings an increase in the secondary porosity of the grains. This determines a non-linear trend in the compositional variations in the individual grains, and a general leaching of the alkalis evidenced in the percentage values for K and Na that are much lower in respect to those of the original material.
Discussion
In this study, the analysis of the results considered four important factors that potentially contributed to the effect of alkaline treatments on smalt in lime-based wall paintings: the physical and elemental qualities of the pigment, the original technique and characteristics of the paint layer, the condition of the painting in terms of its state of conservation, and the parameters and duration of the treatment.
Pigment: physical and elemental qualities
The role of grain size in reactivity is noted in the literature, with smalt grains appearing to deteriorate at different rates depending on their dimensions (Boon et al. Citation2001; Santopadre and Verità Citation2006; Panighello et al. Citation2016). In this experiment, the relationship between the size of pigment grains and different treatments applied to portions of replicas was not specifically taken into consideration because the granulometric distribution of the pigment used falls in a too narrowly defined dimensional range (60–120 µm). However, the phenomenon can begin to be understood based on the principles relating to relative surface area and the behaviour of glass in contact with water, whereas the greater the surface to volume ratio, the greater the reactivity of the glass (El-Shamy and Douglas Citation1972; Jain Citation2000).
Smalt in wall paintings from the Renaissance demonstrate a wide range of grain sizes: Bersani et al. (Citation2010) report that in Correggio’s paintings in the Cathedral of Parma smalt grains measured between 30 and 40 µm; and Santopadre and Verità (Citation2006) report grains between 15–80 µm and 15–60 µm respectively in two different wall paintings. Sometimes precise measurements are not specified in reports, yet dimensions are defined as notable: the smalt used by Ghirlandaio in the Coronation of the Virgin in Santa Maria Novella Church in Florence was described as ‘large crystals’ (‘grossi cristalli’, Bandini Citation2002); and the smalt in Bernardino Luini’s Madonna col Bambino e Sant’Antonio Abate, was described as ‘large fragments of smalt’ (‘Smalto in grossi frammenti … ’, Gallone Citation1991, 14). Ajò et al. (Citation2004) report both ‘coarse’ and ‘fine’ smalt grains in the later work of Ciro Ferri. Smalt grains found in wall paintings are generally larger than those used in canvas paintings from the same period; reported in the literature as between 5–30 µm (Panighello et al. Citation2016); 10–50 µm (Robinet et al. Citation2011a); 10–40 µm (Kugler et al. Citation2013); and 2–70 µm (Boon et al. Citation2001). This may help to explain why smalt deterioration is more notable in canvas paintings since the smaller grains would be more susceptible to deterioration.
Original technique: characteristics of the paint layer
This experiment, featuring painting techniques in which the pigment smalt may have been used in the past, has demonstrated that substantial variations do not exist in the microtextural and compositional consequences tied to the deterioration of the smalt grains based on painting technique.
Condition of the paint
The physical and elemental stability of the paint layer and the ‘condition’ of the grains before treatment was shown to be important in this experiment. As this is difficult to assess in authentic paintings, it is useful to consider information from laboratory replicas. Samples from control replicas 1A, 1B, and 1C (not previously exposed to water) were compared after treatment with those from replicas that had been exposed to water in different forms (either liquid or vapour). Results showed that some of the grains with a substantial leached layer before treatment demonstrated less relative corrosion after a long treatment than grains from replicas not previously exposed to water; suggesting that leaching due to exposure to water (liquid or vapour) made a difference in the later resistance of the smalt grains to alkaline treatments (especially in the case of water vapour).
This variation is perhaps attributable to the existence of a silica-rich layer in coronitic microtextures on the outer surface of the glass (sometimes called the ‘gel layer’). It has been suggested that this layer may serve a protective function by influencing the process of ion exchange, and that it may in some cases either slow or stop the deterioration process (El-Shamy et al. Citation1972; Santopadre and Verità Citation2006; Gentaz et al. Citation2011). In any case, it is an important, yet difficult to define, issue in wall paintings which are complex physical and chemical systems, often located in church buildings or on exterior walls where it is difficult to regulate the environmental parameters to which they are exposed (substantial and unpredictable shifts in temperature, relative humidity, unregulated exposure to atmospheric pollutants and particulate matter), and reconstruct their conservation histories (restoration treatments in the past involving cleaning and/or consolidation and application of protective coatings). In addition, diffusion processes from the lime matrix to the smalt pigment grains (and vice versa) should be taken into consideration; this topic deserves more in-depth study in future research.
Treatment: parameters and duration
The particulars of application of treatments also appear to influence their impact on smalt in lime-based wall paintings. The smalt grains in this experiment exposed to the ammonium bicarbonate (pH ≅ 8.2) tended to be less affected by exposure than the grains treated with ammonium carbonate (pH ≅ 9.2) after 1 h. The grains exposed for 6 h to ammonium carbonate demonstrated similar types of deterioration to the results after 1 h, but with more intensive corrosion. The grains exposed to ammonium carbonate for 6 h, then barium hydroxide (pH ≅ 13) for 6 h demonstrated substantial deterioration (corrosion).
Glass deteriorates in different ways based on the pH value of the solution with which it is in contact, with leaching occurring in contact with pH values of <9 and congruent dissolution or corrosion in contact with pH values of >9. Calcium hydroxide, present in the plaster layer and often used in paint application, has a pH value of ≅12.6 at 20°C (National Lime Association Citation2007). However, the pH value of the surface reduces as it carbonates. A paint layer applied a secco (with glue) and a carbonated lime-based wall painting surface has a pH value closer to 8 (Mattei et al. Citation2008). Thus, smalt applied in lime-based techniques theoretically undergoes physical and elemental changes when it is initially applied as it is exposed to water and high pH values. Yet at some point equilibrium is reached in the wall painting system. Although unstable climate could in theory provoke further changes, the greatest danger to this equilibrium would be prolonged exposure to water and unusually high pH values.
Results of the present experiment showed that the progression of deterioration was not always linear and predictable based on the substance and contact time used in treatment; yet recognizable changes fall on a continuum of deterioration from undamaged to completely changed (physically and chemically) between the contact times of 1 and 6 h ( and ). There were also differences in the ways that cracking and corrosion occurred in the grains. Some zoned grains showed signs of cracking in correspondence to the rim zone. Some grains presented an elementally uniform composition according to SEM-EDX, yet showed extensive cracking (Type II, ).
The condition of smalt, even when applied in different painting techniques, is heavily dependent on its previous exposure to water, as seen in the comparison of the replicas in Set 1 (no previous exposure to water) and those in Set 3 (exposed to water vapour). Additionally, this appeared to significantly affect the behaviour of the pigment in respect to the typology of treatments and to the contact times used to apply them. In fact, the most critical conditions were observed for Replicas 3A, 3B, and 3C, where the surfaces were subjected for six hours to ammonium carbonate and then for six hours to barium hydroxide. In these cases, there were significant examples of pronounced corrosion accompanied by a non-linear and compositional variability in the pigment grains mostly in terms of the alkali content ().
Some grains that underwent treatments with shorter contact times often showed uneven deterioration corresponding to areas where they were partially covered by the paint layer and partially exposed to the surface. Any potential ‘protective’ role of the paint layer for smalt may be especially important in wall paintings, as grains of pigment that are coarsely ground such as smalt tend to ‘sit’ higher in the paint layer (Cornale et al. Citation2005) and thus are theoretically more directly exposed to substances applied to the surface. It is also possible that in some cases, the qualities (compactness, intactness) of the paint layer afford some limited protection or buffer to grains from substances applied to the surface. The specific ‘protective’ role of the binder is complicated and unresolved in lime-based wall paintings as well as the aforementioned possible diffusion processes; comparatively, it has been shown that smalt in a paint layer applied in an organic binder (glue) was susceptible to deterioration by humidity and atmospheric gas (Altavilla and Ciliberto Citation2004).
Examples found in the published literature demonstrate that treatments are commonly applied to wall painting surfaces with a variety of contact times that can range from several minutes (Colalucci Citation1986, Citation1991) to several hours (Bandini et al. Citation1986; Giovannoni Citation1990; Matteini Citation2010) and with different methods of application depending on the individual painting and the purpose of the intervention. The results of the research presented here suggest that although reactions occurred even in shorter contact times of one hour, there is a significant difference in the degree of reaction of smalt grains to contact times lasting longer than one hour.
Conclusions
Conservation treatments involve a conscious decision to upset the equilibrium of a system to rectify an identified and documented problem needing attention. Although the application of aqueous-based alkaline treatments are widely considered to be safe on wall paintings containing smalt, the information in the literature, and the results of the experiment presented here highlight several potentially negative consequences of these treatments. Based on the analysis of the information gathered, it is possible to propose ways in which damage can be minimized, mitigated, or avoided through modifying methods and materials used in treatments. More specifically, the following issues are critical: controlling the pH values of substances applied; modifying the method of application of treatments to limit saturation and contact time; reducing the overall volume of water used; monitoring and maintenance of smalt surfaces; and reconsidering methods of evaluating the efficacy of treatment.
Treating smalt
The pH value of a substance in contact with smalt has been proven to be important. Alkaline treatments react with the surface of smalt grains. For intact grains, this brings about a loss of material. For deteriorated grains, alkaline treatments disrupt the altered outer layer of the glass, raising two issues: the first is that this layer may potentially serve some protective role in preserving the glass core and so its disruption may trigger a new cycle of deterioration once a fresh surface is exposed; the second is that it should be considered as original material from an ethical point of view, as it is in the literature about glass conservation (Koob Citation2006). The fact that glass deterioration occurs at both ends of the pH scale (<9 and >9) must be considered when planning treatment to avoid damage to the pigment during treatment and in the future.
A common method of applying treatments to wall paintings is cellulose pulp poultices as they ensure good and regular contact between the wall surface and the solution being used in treatment for an extended period of time. However, it is precisely this intensity and duration of contact that appears to be detrimental for smalt. In the experiment presented here, it was clear that longer contact times led to increased deterioration of grains overall (with an acknowledged variation within the samples). In cases where it is determined that an aqueous-based treatment is necessary, it would perhaps be possible to explore using poulticing materials with a higher retention value to better control the direct contact of the surface with an aqueous-based solution and limit the absorption of water into the system that extends the time that the pigment is in contact with the solution.
Although the volume of water used in conservation treatments was not a measured parameter in this experiment, information in the literature confirms that smalt in contact with water brings deterioration (El-Shamy and Douglas Citation1972; Kunicki-Goldfinger Citation2008). This suggests that in any treatment, the volume of water applied and the rate of replenishment of the solution is important; the amount of water used will directly affect the degree to which there will be available H+ ions in the solutions that can interact with smalt, and the time it will require for the water to exit the system. Limiting the amount of water that is introduced through treatment would minimize the solution that the pigment can react with, an issue also addressed in the literature about the conservation of glass (Römich et al. Citation2000; Murcia-Mascaros et al. Citation2008), and already raised as a potential issue in the conservation of smalt in canvas paintings (Spring et al. Citation2005).
Monitoring and maintaining smalt surfaces in good order is also important to the conservation of smalt in lime-based wall paintings. Although historic buildings often have issues in terms of environmental humidity that are difficult to solve, it is important to note that for smalt surfaces, the failure of a window seal or gutter leading to the ingress of water into a building, issues with groundwater, and/or rapid changes in temperature and relative humidity near or around the wall painting can cause deterioration of the painted surface. For this reason, monitoring of climatic parameters would be important since it has been shown that even atmospheric moisture can affect the pigment (Cianchetta et al. Citation2012) and surfaces even in the same building can deteriorate in different ways based on the type of moisture in the environment (Santopadre and Verità Citation2006).
Finally, a common method of evaluating the efficacy of treatments on smalt surfaces is based on a visual inspection of the surface to identify changes in tone or saturation of colour. The deterioration of smalt is complicated and does not always manifest in colour change that would be perceptible to the naked eye. It is especially important for smalt in lime-based wall paintings, that when alkaline conservation treatments are used, the fact that there are no visible changes to the surface does not guarantee that the treatment has not provoked changes in the pigment. Therefore, better methods must be developed to monitor changes that reflect the present understanding of glass materials in general and smalt in particular (more specifically that it deteriorates differently when exposed to both <9 and >9 pH values). Until a suitable method is developed, a cautious approach should be adopted in approaching the evaluation of alkaline treatments and methods other than those based on a purely visual and tone-based survey to judge the efficacy of conservation treatments should be considered.
Issues for further research
Several issues that arose in the course of the present experiment are worth exploring in future research: the effect of solution concentration in treatment, elemental variation in historical smalt, and secondary deterioration products.
The influence of the concentration of the treatment solution on smalt is not clear. In this experiment, ammonium bicarbonate and ammonium carbonate were prepared to a concentration of 10% and barium hydroxide was prepared at 6%. Although the concentration of the solution depends on the perceived need in each intervention, ammonium bicarbonate and ammonium carbonate are sometimes used at percentages ranging from 2-10%, and sometimes used as a saturated solution (with a concentration of between 24% and 35%) (Bandini et al. Citation1986; Colalucci Citation1986). It would be important to better understand how this variable affects smalt when it is treated in wall paintings.
Another important issue for historical smalt would be to investigate the impact of elemental variation in different smalts since the original composition of glass has been proven to be crucial in determining its vulnerability to forces and agents of deterioration (Messiga and Riccardi Citation2006; Murcia-Mascaros et al. Citation2008). In the early Renaissance, smalt was still a non-standard material with elemental variation, that might be an important factor influencing how individual ‘smalts’ are more or less susceptible to deterioration.
Finally, it would be important to investigate secondary weathering products and the release of certain elements during the leaching process that can cause other problems in the system. Salt formation caused by the release of alkali from the pigment has been shown to be an issue in deteriorated smalt surfaces in canvas paintings, with the formation of carbonates and sulphates of calcium, sodium, or potassium on the painting surface (Dal Bianco et al. Citation2005; Spring et al. Citation2005; Carmona, Villegas, and Fernandez Navarro Citation2006; Vilarigues and de Silva Citation2006; Kunicki-Goldfinger Citation2008; Van Loon et al. Citation2011; Van der Snickt et al. Citation2016). As salts in wall paintings are an especially important issue in conservation, the study of this phenomenon in wall paintings would be a valuable contribution.
Acknowledgements
The authors would like to thank the anonymous reviewers who read this paper for their helpful and constructive comments and suggestions.
Disclosure statement
No potential conflict of interest was reported by the author(s).
References
- Ajò, D., U. Casellato, E. Fiorin, and P. A. Vigato. 2004. “Ciro Ferri’s Frescoes: A Study of Painting Materials and Technique by SEM-EDS Microscopy, X-ray Diffraction, Micro-FTIR and Photoluminescence Spectroscopy.” Journal of Cultural Heritage 5: 333–348.
- Altavilla, C., and E. Ciliberto. 2004. “Decay Characterization of Glassy Pigments: an XPS Investigation of Smalt Paint Layers.” Applied Physics A. 79: 309–314.
- Bandini, F. 2002. “Gli affreschi di Domenico Ghirlandaio nella cappella Tornabuoni in Santa Maria Novella a Firenze.” In Materiali e Tecniche nella pittura murale del quattrocento: Storia dell’arte, indagini diagnostiche e restauro verso una nuova prospettiva di ricerca, Vol. II, edited by F. Cardinali, 351–359. Rome: Documentazione preliminare al Convegno Internazionale 20-22 Febbraio 2002.
- Bandini, F., G. Botticelli, M. Danti, M. Matteini, and A. Moles. 1986. “The Restoration of Domenico Ghirlandaio’s Frescoes in the Cappella Maggiore of Santa Maria Novella in Florence: Problems, Practical Work, Results.” Studies in Conservation 31 (1): 186–189.
- Barucci, L. 2002. “Gli azzuri della Loggia di Psiche.” In Raffaello: La loggia di Amore e Psiche alla Farnesina, edited by R. Varoli-Piazza, 157–179. Milan: Silvana Editoriale.
- Berrie, B. 2015. “Mining for Color: New Blues, Yellows, and Translucent Paint.” Early Science and Medicine 20: 308–334.
- Bersani, D., M. Berzioli, S. Caglio, A. Casoli, D. Cauzzi, P. Lottici, L. Medeghini, G. Poldi, and P. Zannini. 2010. “Il Blu di Smalto in affreschi: il caso del Correggio nella cupola del Duomo di Parma.” PATRON: Atti del convegno Air Reflessioni e Trasparenze Diagnosi e Conservazione di Opere e Manufatti Vetrosi. Bologna, 205–223.
- Bersani, D., M. Berzioli, S. Caglio, A. Casoli, P. Lottici, L. Medeghini, G. Poldi, and P. Zannini. 2014. “An Integrated Multi-Analytical Approach to the Study of the Dome Wall Paintings by Correggio in Parma Cathedral.” Microchemical Journal 11: 80–88.
- Boon, J., K. Keune, J. van der Weerd, M. Geldof, and J. R. J. van Asperen de Boer. 2001. “Imaging Microspectroscopic, Secondary Ion Mass Spectrometric and Electron Microscopic Studies on Discoloured and Partially Discoloured Smalt in Cross-Sections of 16th Century Paintings.” Chimia 55: 952–960.
- Borgia, I., and C. Seccaroni. 2007. “L’azzurro di smalto nella pittura e nelle fonti italiane del XV e XVI secolo”. OPD 17, Note di restauro, 152–164.
- Cadogan, J. 2002. “Osservazioni sulla tecnica del Ghirlandaio.” In Materiali e Tecniche nella pittura murale del quattrocento: Storia dell’arte, indagini diagnostiche e restauro verso una nuova prospettiva di ricerca, Vol. I, edited by F. Cardinali, 361–363. Rome: Documentazione preliminare al Convegno Internazionale 20–22 Febbraio 2002.
- Cagno, S., K. Janssens, and M. Mendera. 2008. “Compositional Analysis of Tuscan Glass Samples: In Search of raw Material Fingerprints.” Analytical and Bioanalytical Chemistry 391: 1389–1395.
- Carmona, N., M. Villegas, and J. Fernandez Navarro. 2006. “Characterisation of an Intermediate Decay Phenomenon of Historical Glasses.” Journal of Material Science 41: 2339–2346.
- Cianchetta, I., I. Colantoni, F. Talarico, F. d’Acapito, A. Trapananti, C. Maurizio, S. Fantacci, and I. Davoli. 2012. “Discoloration of the Smalt Pigment: Experimental Studies and ab Initio Calculations.” Journal of Analytical Atomic Spectrometry 27: 1941–1948.
- Colalucci, G. 1986. “Michelangelo in the Sistine Chapel: Painting Technique and Technique of Restoration.” Studies in Conservation 31 (sup. 1): 46–47.
- Colalucci, G. 1991. “The Frescoes of Michelangelo on the Vault of the Sistine Chapel: Original Technique and Conservation.” In The Conservation of Wall Paintings, edited by S. Cather, 103–136. Los Angeles: The Getty Conservation Institute.
- Cornale, P., L. Maritan, C. Mazzoli, and R. Piovesan. 2005. “Affresco e Mezzofresco: Studio sperimentale e procedure analitiche per la caratterizzazione delle techniche pittoriche.” Scienza e Beni culturali XXI: 687–696.
- Crundwell, F. K. 2014. “The Mechanism of Dissolution of Minerals in Acidic and Alkaline Solutions: Part II Application of a new Theory to Silicates, Aluminosilicates and Quartz.” Hydrometallurgy 149: 265–275.
- Dal Bianco, B., R. Bertoncello, L. Milanese, and S. Barison. 2005. “Glass Corrosion Across the Alps: A Surface Study of Chemical Corrosion of Glasses Found in Marine and Ground Environments.” Archaeometry 47 (2): 351–360.
- Daniilia, Sister, and E. Minopoulou. 2009. “A Study of Smalt and Red Lead Discolouration in Antiphonitis Wall Paintings in Cyprus.” Applied Physics A 96: 701–711.
- De Ferri, L., D. Bersani, P. Colomban, P. Lottici, G. Simon, and G. Vezzalini. 2012. “Raman Study of Model Glass with Medieval Compositions: Artificial Weathering and Comparison with Ancient Samples.” Journal of Raman Spectroscopy 43 (11): 1817–1823.
- Delamare, F. 2013. Blue Pigments: 5000 Years of Art and Industry. London: Archetype Publications.
- Eastaugh, N., V. Walsh, T. Chaplin, and R. Siddall. 2004. The Pigment Compendium. Amsterdam: Elsevier Butterworth-Heinemann.
- El-Shamy, T., and R. W. Douglas. 1972. “Kinetics of the Reaction of Water with Glass.” Glass Technology 13 (3): 77–80.
- El-Shamy, T., J. Lewins, and R. W. Douglas. 1972. “The Dependence on the pH of the Decomposition of Glasses by Aqueous Solutions.” Glass Technology 13 (3): 81–87.
- Freestone, I. 1992. “Theophilus and the Composition of Medieval Glass.” In Materials Issues in Art and Archaeology Symposium, 740. San Francisco, CA. Materials Research Society.
- Frezzato, F. ed. 2011. Cenino Cennini, Il Libro dell’Arte. Vicenza: Neri Pozza Editore.
- Gallone, A. 1991. “Studio analitico del colore di affreschi staccati- Pinacoteca di Brera- Milano, 22.10.1991.” Polytecnico di Milano-Istituto di Fisica. 14.
- Gentaz, L., T. Lombardo, C. Loisel, A. Chabas, and M. Vallotto. 2011. “Early Stage of Weathering of Medieval-Like Potash-Lime Model Glass: Evaluation of Key Factors.” Environmental Science and Pollution Research 18: 291–300.
- Gettens, R., and E. W. Fitzhugh. 1993. “Azurite and Blue Verditer.” In Artists’ Pigments: A Handbook of Their History and Characteristics, Vol. 2, edited by A. Roy, 23–33. Washington: National Gallery of Art.
- Giovannoni, S. 1990. “Restauri nel Refettorio della SS. Annunziata in Firenze,” Le Pitture Murali. Firenze. 289–290.
- Giovanoli, R., and B. Mühlethaler. 1970. “Investigation of Discoloured Smalt.” Studies in Conservation 15 (1): 37–44.
- Gratuze, B., I. Soulier, J. Barrandon, and D. Foy. 1995. “The Origin of Cobalt Blue Pigments in French Glass from the Thirteenth to the Eighteenth Centuries.” In Trade and Discovery: The Scientific Study of Artefacts from Post-Medieval Europe and Beyond, edited by D. Hook, and D. Gaimster, 123–133. London: British Museum Press.
- Jain, V. 2000. Chemical Durability of Nuclear Waste Glasses – a Review. San Antonio, TX: Center for Nuclear Waste Regulatory Commission. https://www.nrc.gov/docs/ML0613/ML061310303.pdf.
- Janssens, K., G. Van Der Snickt, M. Alfeld, P. Noble, A. van Loon, J. Delaney, D. Conover, J. Ziebel, and J. Dik. 2016. “Rembrandt’s ‘Saul and David’ (c. 1652): use of Multiple Types of Smalt Evidenced by Means of non-Destructive Imaging.” Microchemical Journal (126): 515–523.
- Koob, S. 2006. Conservation and Care of Glass Objects. London: Archetype Publications in association with the Corning Museum of Glass.
- Kugler, V., M. Spring, and J. Hudson. 2013. "Quantitative SEM-EDX Analysis of Smalt Pigment Under Variable Pressure Conditions." Microscopy and Microanalysis 19 (Suppl 2): 1428–1429.
- Kunicki-Goldfinger, J. 2008. “Unstable Historic Glass: Symptoms, Causes, Mechanisms and Conservation.” Reviews in Conservation 9: 49.
- Mattei, E., G. de Vivo, A. De Santis, C. Gaetani, C. Pelosi, and U. Santamaria. 2008. “Raman Spectroscopic Analysis of Azurite Blackening.” In Proceedings of the XX Italian Conference on Raman Spectroscopy and Non Linear Effects, Catania, Italy, 27–29 June 2007. 39, 2, 302–306.
- Matteini, M. 1991. “In Review: An Assessment of Florentine Methods of Wall Painting Conservation Based on the Use of Mineral Treatments.” In The Conservation of Wall Paintings, edited by S. Cather, 103–136. Los Angeles: The Getty Conservation Institute. http://hdl.handle.net/10020/gci_pubs/conserv_wall_paintings.
- Matteini, M. 2010. “Inorganic Treatments for Cleaning, Consolidation and Protection of Mural Paintings: Tradition and Innovation”. Science in Conservation Conference, 1–16.
- Melcher, M., and M. Schreiner. 2006. “Leaching Studies on Naturally Weathered Potash-Lime-Silica Glasses.” Journal of Non-Crystalline Solids 352: 368–379.
- Merrifield, M. 2004. The Art of Fresco Painting in the Middle Ages and the Renaissance. Mineola: Dover Publications.
- Messiga, B., and M. P. Riccardi. 2006. “Alteration Behaviour of Glass Panes from the Medieval Pavia Charterhouse (Italy).” Journal of Cultural Heritage 7: 334–338.
- Mühlethaler, B., and J. Thissen. 1993. “Smalt.” In 1993. Artists’ Pigments: A Handbook of Their History and Characteristics. Vol. 2, edited by A. Roy, 113–130. Washington: National Gallery of Art.
- Murcia-Mascaros, S., P. Foglia, M. L. Santarelli, C. Roldán, R. Ibañez, A. Muñoz, and P. Muñoz. 2008. “A new Cleaning Method for Historic Stained Glass Windows.” Journal of Cultural Heritage 9: e73–e80.
- National Lime Association Lime Terminology, Standards & Properties. 2007. Accessed June 2020. www.lime.org/publications.
- Panighello, S., A. Kavčič, K. Vogel-Mikus, N. Tennent, A. Wallert, S. Hočevar, and J. Elteren. 2016. “Investigation of Smalt in Cross-Sections of 17th Century Paintings Using Elemental Mapping by Laser Ablasion.” Microchemical Journal 125: 105–115.
- Plesters, J. 1993. “Ultramarine Blue, Natural and Artificial.” In 1993. Artists’ Pigments: A Handbook of Their History and Characteristics, Vol. 2, edited by A. Roy, 37–61. Washington: National Gallery of Art.
- Reade, W., I. Freestone, and S. J. Simpson. 2003. “Innovation or Continuity? Early First Millennium BCS Glass in the Near East: The Cobalt Blue Glasses from Assyrian Nimrud.” In AIHV Annales du 16 Congrès.
- Robinet, L., M. Spring, and S. Pagès-Camagna. 2011a. “Investigation of the Loss of Colour in Smalt on Degradation in Paintings Using Multiple Spectroscopic Analytical Techniques.” Lisbon 2011 conference. 1–8.
- Robinet, L., M. Spring, and S. Pagès-Camagna. 2013. “Vibrational Spectroscopy Correlated with Elemental Analysis for the Investigation of Smalt Pigment and Its Alteration in Paintings.” Analytical Methods. doi:10.1039/c3ay40906f.
- Robinet, L., M. Spring, S. Pagès-Camagna, D. Vantelon, and N. Trcera. 2011b. “Investigation of the Discoloration of Smalt Pigment in Historic Paintings by Micro-X-ray Absorption Spectroscopy at the Co K-Edge.” Analytical Chemistry 83: 5145–5152.
- Römich, H., E. Jägers, M. Torge, W. Müller, and K. Adam. 2000. “This Text Originally Appeared as Chapter 5, ‘Reinigung – eine Gratwanderung’.” In Restaurierung und Konservierung historischer Glasmalereien, edited by A. Wolff, 101–128. Mainz: Verlag Philipp von Zabern. http://www.cvma.ac.uk/conserv/cleaning.html.
- Salerno, C., and S. Ferroni. 1999. “Pigmenti a base di vetro nella pittura rinascimentale e barocca da riccettari muranesi editi ed inediti.” Rivista della Stazione Sperimentale del Vetro 6: 293–302.
- Santopadre, P., and M. Verità. 2006. “A Study of Smalt and Its Conservation Problems in two Sixteenth Century Wall Paintings in Rome.” Studies in Conservation 51 (1): 29–40.
- Schalm, O., V. Van der Linden, P. Frederickx, S. Luyten, G. Van der Snickt, J. Caen, D. Schryvers, et al. 2009. “Enamels in Stained Glass Windows: Preparation, Chemical Composition, Microstructure and Causes of Deterioration.” Spectrochimica Acta Part B 64: 812–820.
- Seccaroni, C., and J. Haldi. 2016. Cobalto, zaffera, smalto dall’antichità al XVIII secolo, Laboratorio Tecnografico ENEA-Frascati.
- Spring, M., C. Higgitt, and D. Saunders. 2005. “Investigation of Pigment-Medium Interaction Process in oil Paint Containing Degraded Smalt.” National Gallery Technical Bulletin 26: 56–70.
- Sterpenich, J., and G. Libourel. 2006. “Water Diffusion in Silicate Glasses Under Natural Weathering Conditions: Evidence from Buried Medieval Stained Glasses.” Journal of Non-Crystalline Solids 352: 5446–5451.
- Tournié, A., P. Ricciardi, and Ph. Colomban. 2008. “Glass Corrosion Mechanisms: A Multiscale Analysis.” Solid State Ionics 179: 2142–2154.
- Van der Snickt, G., S. Legrand, J. Caen, F. Vanmeert, M. Alfeld, and K. Janssens. 2016. “Chemical Imaging of Stained -Glass Windows by Means of Macro X-ray Fluorescence (MA-XRF) Scanning.” Microchemical Journal 124: 615–622.
- Van Loon, A., P. Noble, and J. Boon. 2011. “White Hazes and Surface Crusts in Rembrandt’s Homer and Related Paintings.” Lisbon Conference 2011. 1–10.
- Vilarigues, M., and R. de Silva. 2006. “Characterization of Potash-Glass Corrosion in Aqueous Solution by Ion Beam and IR Spectroscopy.” Journal of Non-Crystalline Solids 352: 5368–5375.