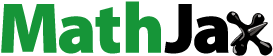
ABSTRACT
With the occasion of the exhibition Fatti come nuovi (20 October 1985–12 January 1986), where the most prominent artworks of the Poldi Pezzoli Museum in Milan were shown after restoration, a multidisciplinary project was launched between the Museum and the Italian Montedison group. This project aimed at evaluating several conservation products used at the time in ceramic and glass conservation, such as adhesives and retouching varnishes. In the frame of the project, two-component epoxy adhesives (Plastogen® EP, HXTAL NYL-1), acrylates (Paraloid® B-72, Plastogen® G), vinyl acetate dispersions (Vinavil®, Arkolyn), a polyester resin and cellulose nitrate adhesives (HMG, AGO® Goldsiegel) were studied in terms of their mechanical properties and colour change upon artificial ageing by UV-VIS radiation. Since then, the samples were kept in the dark. Almost 30 years later, the samples have been re-evaluated for changes in solubility and colour upon natural ageing.
Introduction
In the mid-1980s, an interdisciplinary collaboration between the company Montedison and the Museum Poldi Pezzoli aimed at the evaluation of several conservation products as used in ceramic and glass conservation at that time, such as adhesives and retouching varnishes. Strength, colour stability, and hardness were tested before and after artificial ageing. The results, obtained and published in 1985 in the catalogue Fatti come nuovi (Agnini, Venchiarutti, and Massa Citation1985), presented an early characterisation approach to solve the problem of obtaining extensive and articulated information on the products used in conservation. At the time the project was carried out, a small range of conservation products for ceramics and glass existed in Italy and only very few specific publications and literature about these products were accessible (Down Citation1984); relevant publications now available post-date the original study (Down Citation1996, Citation2015; Derschau and Unger Citation1998). In the frame of the collaboration, a range of products commonly used by conservators in the 1970s and 1980s was chosen for evaluation focussing particularly on conservation aspects.
When choosing an adhesive for the conservation of ceramic and glass objects, the following points should be considered: (i) reversibility, (ii) non-yellowing, (iii) applicability, (iv) sufficient cohesion without shrinkage, and (v) chemical and mechanical stability (Down Citation1984; Feller Citation1994; Horie Citation2010). It should also retain its properties in various environmental conditions. For ceramics, in particular, adhesives should have the correct density, depending on the porosity of the ceramic. In the case of glass, the refractive index (RI) of the adhesive should match the RI of the glass and it remain clear and colourless. To minimise the need to restore the artefacts a second time, adhesives must be durable and their physical properties have to remain stable upon ageing for at least 100 years (Feller Citation1994; Koob Citation2006). Finally, conservation treatments should be reversible at any time, without causing damage to the original objects.
Stability is indeed one of the main issues when choosing a material for conservation. Both in the conservation community and in the academic and industrial literature, authors have devoted themselves to the evaluation of the effect of ageing on materials’ properties. It is particularly interesting in this respect to consult the conservation literature, which is more interested in the long-term stability of these materials due to the longer useful life that is expected for them as opposed to most products used for industrial purposes. Also, the parameters of interest are different to some extent; for industrial uses, retaining the mechanical properties of a material over time is usually much more relevant than maintaining its appearance e.g. colour (Down Citation1984).
In this initial stage of the project, which started in the 1980s and is also reported here, several adhesives were studied in terms of their mechanical properties and colour change upon artificial ageing by UV-VIS radiation. The range of adhesives chosen features epoxy resins, acrylates, polyvinyl acetates, and cellulose nitrate, which represent the most typical adhesives used at the time in Italy for ceramic and glass restoration.
The stability of these materials has been studied in the past. The tendency of epoxy resins to yellow over time has been a main concern since their appearance in glass conservation, when they became interesting due to their ability to solve some of the issues posed by other adhesives such as acrylic, cellulose nitrate, and polyvinyl acetate (Down Citation1984). Down carried out in-depth analyses of a broad range of epoxy resins used as adhesives in glass conservation. Her 1984 study focused on the natural dark ageing evaluation of 55 commercially available epoxy resins, particularly on the effects of thermal ageing on yellowing. This allowed her to report changes in colour not caused by light-induced reactions. Based on yellowing curves (UV-VIS absorption data) she was able to estimate the life expectancy for a range of epoxy resins, which was lower than 20 years for most of the products, and between 20 and 100 years for the best ones, thus showing that the non-linear, multi-phase yellowing due to thermal effects is a non-negligible contribution to the overall colour change upon ageing. In later work (Down Citation1996), Down showed that, while the intensity of light exposure determines the service life expectancy of any epoxy resin (all tested resins yellowing under high-intensity light), under low intensity irradiation (closer to ideal museum conditions), the life expectancy is similar to the natural dark ageing results. This work also documented the effect that small changes in the formulation (not detectable by using FTIR analysis) can have on the long-term stability of some epoxy resins.
HXTAL NYL-1 was among the most durable epoxy resins both under low- and high-intensity irradiation. Depending on their structure and the presence of additives, epoxy resins are differently prone to ageing, and previous research has studied the mechanisms and species appearing upon ageing, and the chromophores that may be behind yellowing (Luoma and Rowland Citation1986; Zhang Citation1994; Fulton Citation1998; Mailhot Citation2005). In this respect, HXTAL NYL-1 has been repeatedly documented to have the lowest tendency to yellowing (Coutinho Citation2009; Sideridou, Vouvoudi, and Papadopoulos Citation2016). Even in this case, the lighting conditions for the restored objects should be considered if yellowness is to be avoided. Regarding the removal of epoxy resins, dichloromethane, tetrahydrofuran, and chloroform, among others, have been proposed as the best solvents to swell and mechanically remove epoxy resin adhesives, although the best solvent is dependent on the particular resin being considered (Down Citation2001). Generally, UV-aged samples were noted as being easily removable.
In Down’s comprehensive work on polyacrylates and polyvinyl acetates from the 1980s (Down Citation2015), samples were aged in the dark for around 25 years and 12–15 years under light exposure, and then were evaluated for a number of changes, among them pH, strength, and yellowing. The study showed that light ageing causes a faster yellowing than dark ageing for the tested polyvinyl acetates; the best values are obtained for UHU® All-Purpose Clear Adhesive (homopolymer with cellulose nitrate), which remains clear throughout. In the overall performance (pH, volatiles, mechanical properties, and yellowing), polyvinyl acetate homopolymers are acidic, cohesively strong, brittle, and have fair to poor yellowing properties. The copolymers fare better than the homopolymers, since they are more neutral, flexible, show medium strength, and yellow slightly less. In general, vinyl acetate/ethylene copolymers perform better regarding the studied parameters.
The same trend with respect to yellowing under light exposure was observed for the acrylates, but lower absolute values (also for the initial colour) and reduced yellowing changes were measured for these materials in comparison to the polyvinyl acetates. Other authors studied the thermal (Lazzari and Chiantore Citation2000) and photooxidative (Chiantore and Lazzari Citation2001) ageing of a range of acrylic and methacrylic resins, both homo and copolymers, regarding their ageing reactions (chain scission, crosslinking) and appearance of new functional groups. In the case of photochemical ageing, studies were carried out under a range of different lightning conditions (Melo Citation1999; Bracci and Melo Citation2003; Favaro Citation2006). These studies focused on the use of acrylic resins as protective coatings on stone, and thus were generally more interested in the outdoor ageing. The study of more controlled recipes in these studies, as opposed to Down’s study of commercial products mentioned above, offers the possibility of discovering structure–property relationships which are not obscured by different recipes. In Down’s study, a range of additives were found in the different products which potentially influence their performance; in fact, not all products based in the same structure fare equally in this study. The study offers, however, valuable information on the behaviour of real, commercial conservation products.
The first documented use of cellulose nitrate for the conservation of museum objects dates back to the nineteenth century (Horie Citation2010) and it has since been used as adhesive, consolidant, and coating in numerous applications. However, stability issues have been well known since it was first synthesised (Selwitz Citation1988). These problems have been of concern for archivists, conservators, and conservation scientists for more than 30 years, particularly when cellulose nitrate is present in films (Edge Citation1990) or in bulk objects (Quye Citation2011; Elsässer Citation2021). Cellulose nitrate coatings have been documented to remain soluble and uncoloured after long natural ageing (Shashoua, Bradley, and Daniels Citation1992), as long as they are protected from extreme conditions (Reedy Citation1999). Considering advantages and disadvantages of its use, there are applications in which cellulose nitrate coatings (e.g. where protection against certain sulphur-containing pollutants is key) are still a valid alternative (Grissom Citation2013); however, substitutes are appearing (Dandridge Citation2005; Marquardt Citation2015).
In these studies, yellowness and the identification of the new chemical species appearing upon degradation under different conditions were the focus of research. Only in a few cases was the removability reported (Melo Citation1999; Down Citation2001; Favaro Citation2006).
The Poldi Pezzoli Museum has been engaged for a long time in the conservation of the works of art of its collection. Help came in 1984 from the industrial group Montedison to carry out the restoration of decorative art objects, in particular ceramics and glasses. This group not only financed the restoration, but also provided research in its own laboratories on the materials to be used for the restoration. It also financed the catalogue and the exhibition Fatti come nuovi (20 October 1985–12 January 1986), where the most prominent artworks of the museum in Milan were shown after restoration (). The museum has a broad collection of northern Italian and Netherlandish/Flemish paintings. The collection houses as well weaponry, glassworks, ceramics, jewellery, clocks, textiles, and furnishing. The collection of ceramics and glass of the Museo Poldi Pezzoli is composed of exquisite objects of different ages and provenience. The ceramic collection includes pottery, majolica, and Eastern European porcelain. Among the glass collection, there are glasses of Venetian production, others falling within the category façon de Venise, and some pieces from Germany and Bohemia.
Figure 1. Satyr, manufacturer Doccia (Ginori, Italy), second half of the eighteenth century, museum collection Poldi Pezzoli Milan (Italy) before conservation in 1984 (left) and present state (right). Credit: Poldi Pezzoli Museum (1984 and 2012).
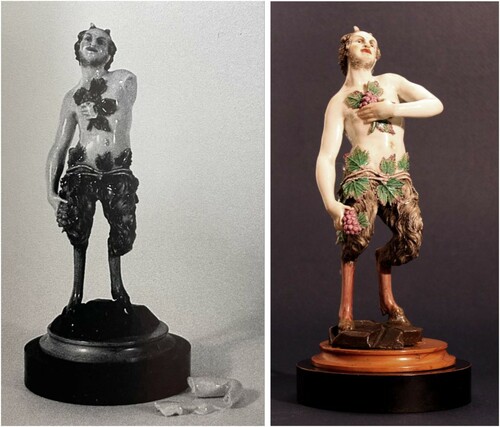
Upon completion of the project, samples of the chosen adhesives for the study were kept in the dark. Around 30 years later, the same samples have been re-evaluated for their changes in solubility, colour, and chemical changes. The present work investigates the impact in terms of yellowing and reversibility of both artificial and natural ageing applied to the same set of conservation materials.
The current work aims at re-evaluating the samples aged in 1984, which feature materials still used today. Using scientific methods to evaluate the effect of the natural ageing in the dark on colour and solubility of the areas not artificially aged in 1984, and compare, where possible and relevant, the changes with those measured on the artificially aged samples (1984), which have now aged naturally in the dark. This paper presents the efforts made to ensure the comparability of the colour analysis for naturally aged samples after three decades, and to study the solubility and reversible character of artificially and naturally aged samples at present, an additional study to which there was no counterpart in 1984. With the purpose of identifying the major components of the adhesives, FTIR analysis has also been performed.
Experimental methods
Sample preparation
The preparation and the evaluation of the samples of some products used for the restoration of the ceramics and glass collection in the Poldi Pezzoli Museum were conducted in the Research Center LARAC S.p.A. in Castellanza by Varese, a branch of the Montedison Group.
In 1984, a series of 13 conservation products were applied on glass plates (Agnini, Venchiarutti, and Massa Citation1985). See and for photographs of the samples taken in 1984, before ageing, and in 2012, respectively. For the analyses, the products were applied on glass specimens with a special tool to a thickness of about 30 µm and were cured, according to the manufacturers’ instructions.
Figure 2. Samples T73, T74, and T75 in 1984, before ageing. Upon visual examination in 1984, all samples appeared transparent.
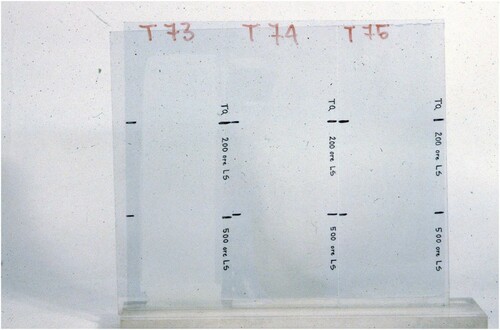
Figure 3. Samples T58, T59, PM9, T74, and T75 with colour chart in 2012 (used in the calibration of all photographs taken in 2012). The samples artificially aged in 1984 are shown here after almost 30 years additional natural ageing.
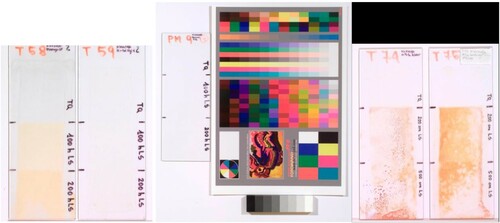
The curing process, carried out at room temperature (RT), was checked by means of hardness measurements carried out later, and this also allowed the final hardness of the samples to be known. Measurements were carried out with the Gardner/Sward Rocker pendulum hardness tester, which provides a relative scale of values where the higher the number, the higher the hardness.
Artificial and natural ageing
In 1984, the samples were aged artificially for 100, 200, and/or 500 h under an OSRAM Vitalux GUR 53 (300 Watt, 220 Volt) on a rotating plate at 17 cm distance from the light source; during the ageing, the maximum temperature of the rotating plate was 60°C (Agnini, Venchiarutti, and Massa Citation1985). reports the studied samples, classified according to their chemical nature. For all materials, samples both not aged, and aged for 200 h were available. For most materials, samples aged for 100 h or 500 h were also available. For those materials only aged up to 200 h, the test was intended as a first assessment, as is the case for the epoxy resins and of the Paraloid® product (Agnini, Venchiarutti, and Massa Citation1985). The colour of the samples was measured, and their mechanical properties tested, before and after ageing.
Table 1. Samples and solvents used for the solubility and reversibility methods.
From 1984 (artificial light ageing study) to 2012, all samples were stored in stable museum object storage conditions. They were kept as they were in the dark in a closed cabinet (ca. 20°C and about 40% RH), in an acid free paper box, and wrapped separately in acid free paper.
The results gathered in this work are mostly based on the evaluation of the naturally aged references (TQ) and the 200 h samples, since these are common to all products throughout. Where available, the evaluation of the 100 and 500 h samples has also been included; this was particularly useful in the case of the 500 h samples for both the solubility and reversibility tests and for the colour measurements.
Conservation products
The product T67 is a polyvinyl acetate glue in acetone solution and as such presents the best level of transparency after hardening. The products T70, T71, and T72 are vinyl acetate dispersions in water, specially developed by Vinavil SpA (Montedison, Italy) as adhesives for porous materials such as wood, leather, and ceramic. The various formulations differ in their curing time and/or their bonding capacity depending on the support previously mentioned. The product T73 is a vinyl acetate dispersion currently used in ceramic conservation. The products T74 from the UK and T75 from Germany are two cellulose nitrate adhesives in solution suitable for leather, wood, ceramic, etc. (see ).
Due to the limited amount of material available, not every test could be performed on every sample. Areas named TQ (tal quale or ‘as it is’ in English) were not artificially aged in 1984 but were studied in 2012 as naturally aged samples. The samples T70-T72 differ in their curing time and/or their bonding capacity depending on the type of support used (e.g. wood, leather, and ceramic).
Solubility and reversibility tests
In order to assess the reversibility of the material after both natural (using the non-artificially aged regions of the 1984 samples) and artificial ageing, two methods were designed. The first (Method A, ‘solubility test’) attempts to deliver a reproducible value for the degree of solubility of the material in the chosen solvent. This method has the advantage of being repeatable under laboratory conditions, and the disadvantage of not being representative of the work of the conservators (an insoluble product can nevertheless be removed from an object by swelling it in the appropriate solvent and removing it mechanically). In order to account for the latter disadvantage, a second method was used, which is similar to previous tests ((de la Rie Citation1988; Bracci and Melo Citation2003) and references cited therein). Method B (‘reversibility test’) evaluates the removal of the material with a cotton swab dampened in a precise amount of solvent and describes its success by counting the number of times that the procedure needs to be repeated to remove the material. This method of evaluation is partly subjective. However, the comparison of both methods, which complement each other, enables a more consistent evaluation of the reversibility of the different conservation products.
For each material, a solvent was chosen according to the decision made by conservators for both the solubility and reversibility tests (see and description below). The criteria used for the choice of solvents included the ease of penetration and ability to swell resins and took into account the experience of the conservator with the materials. This led to the selection of acetone and dichloromethane (DCM). In some cases, the use of warm water (60°C) was favoured for Method B, in order to avoid migration of material into the ceramic.
Method A: solubility test
A mass m0 of each sample was scratched from the glass slide and placed into a cleaned, pre-weighted vial. The amount taken was similar for each sample (≈10 mg). 2 mL of solvent (as specified in ) was then added to the vial. The test tubes were then closed and stirred magnetically for 30 min at RT.
After this time, the solution was filtered on a pre-weighted filter (mF; 55 mm, 2–3 µm pore size). Vial, filter, and funnel were then washed. The filter was then left to dry at RT for at least 2 h (covered to avoid dust deposits), and the insoluble fraction was calculated (minsol).
The filtrate solution (acetone or DCM) was evaporated to dryness at 60°C in an oven and then cooled down at RT, protected from dust. The soluble fraction of polymer was determined by subtraction (msol). All the masses for each single experiment were measured at a similar %RH. The solubility and insolubility percentage are defined as %SOL = [msol/m0]*100 and %INS = [minsol/m0]*100. Following this procedure, the total mass recovered was over 70%.
In most cases, the amount of material was sufficient to perform only one test for each polymer and ageing time; otherwise, average values with the associated errors are presented. The balance accuracy was ±1 mg, which involves a potential error of approximately 10–20% for a 10 mg sample. This unusually high error is only acceptable when considering the minute amounts of material available for the tests (thin films on glass slides). Most of the material available for each sample was used for the solubility and reversibility tests.
Method B: reversibility test
A cotton swab was dampened with a precise amount of solvent drops with the help of a pipette, so that the swab became completely dampened by the solvent. This required a different number of drops for each swab (water: 6, acetone: 9, dichloromethane: 10 drops); for each solvent, the same number of drops was always used.
Five similar areas were chosen for each sample; each was treated in the same way in order to test the repeatability of the procedure.
The dampened swab was lightly pressed against the chosen area, without rolling or any further mechanical action other than the light pressure described, and left there for a short period; this was repeated five times per sample area and solvent (‘Level 1’). The sample was then allowed to dry, and the remaining material was examined visually and with the help of a scalpel, scratching gently. In cases where material was still present, the procedure was repeated again (five additional such ‘pressings’ for Level 2 followed by drying and evaluation, and so on). The reversibility was then visually inspected and the level at which removal had been successful was recorded.
If Level 6 was reached without complete removal of the conservation material, the procedure was repeated in precisely the same way but this time using a scalpel (size no. 15) in order to mechanically remove the material by slight scratching after allowing it to swell in the solvent; again levels 1–6 were defined. The results shown in correspond to the procedure and number of levels used to achieve adhesive removability and are named levels 1 to 6, -swab or -scalpel.
Table 2. Results of the reversibility tests (Method B).
This method allowed removal of adhesives regardless of their solubility in the chosen solvent, and to quantitatively assess their removal in a setup relevant to conservation practice. Insoluble adhesives were thus first softened by the solvent and then lowered with a scalpel. The use of the scalpel offers greater precision than other mechanical instruments both in selecting the area to be cleaned and in choosing the right amount of force, and it allows the deposit to be removed more effectively with greater control.
All the tests were designed together with the conservator, Elena Agnini, who carried out the conservation in 1984 on the original objects.
Colour measurements
In 1984, a Gardner XL 10A colorimeter was used and results were expressed in Hunter Lab colour space. The colour measurements were performed in transmission using a 0° - 180° geometry (Agnini, Venchiarutti, and Massa Citation1985). A 2° observer and CIE illuminant C with colour temperature 6774 K were chosen for the calculation of the colour parameters. Illuminant C was used to represent daylight in 1984 but today is no longer used as such. According to the Lambert–Beer law, the sample thickness has an influence on light absorbed in it. Therefore, the colour measured in transmission relies on the thickness of the samples. Since the samples have all been prepared to the same thickness the transmission measurements can be used for comparison of the colour. An uncoated glass slide was used as filter for the reference measurements in transmission modes.
For the measurements in 2012, the experimental setup and the conditions were kept as similar as possible to those in 1984. To obtain transmission spectra with a wavelength range between 400 and 700 nm, a Zeiss PDA VIS (MCS621 VIS II) spectroscope was connected to a Zeiss Axio Imager.A2 m UV/VIS microscope. The spectra were acquired with the SpectraVision software by A.S. & Co. The photographic documentation was conducted with a Jenoptik – ProgRes CFcool digital camera (see ). The transmission measurements were taken with the light source at an angle of 180° (DIN Citation5033-Citation7 Citation1983). A transparent glass slide was also used as a reference standard for the transmission measurements. For calculation of the colour coordinates in the CIE L*a*b* (DIN EN ISO 11664-Citation4, Citation2012) a D65 illuminant (daylight with colour temperature 6500 K) was used which has the most similar emission spectra to illuminant C used for the measurements in 1984 (Agnini, Venchiarutti, and Massa Citation1985). However, a 10° observer was used.
Figure 4. A cellulose nitrate sample artificially aged in 1984, during colour measurement using the microscope Axio Imager.A2m. The area where the colour measurement was taken is marked with a circle. Photograph taken in 2012.
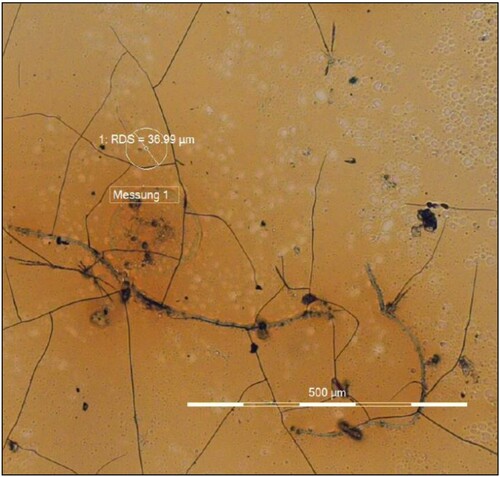
In order to enable a comparison between Hunter Lab and CIE L*a*b* colour spaces the Hunter Lab values (LH, aH, bH without *) were converted to the CIE L*a*b* colour space. For the calculation, the Hunter Lab values were first transformed into the XYZ tristimulus colour space and then to CIE L*a*b* with the following formulas: for Hunter Lab to XYZ (‘Hunter L, a, b Color Scale’ Citation2008); and for CIE L*a*b* to XYZ (DIN EN ISO 11664-Citation4 Citation2012). For the calculations of XYZ and L*a*b* values a 2° observer with illuminant C was chosen, with Xn = 98.04, Yn = 100.00 and Zn = 118.11 as reference values.
(1)
(1)
(2)
(2) where
and
and
.
Once the values of 1984 (originally in the Hunter Lab colour space) were transformed to CIE L*a*b* it was possible to compare the b* values, which represent the colour changes from blue to yellow (from negative to positive). ΔE*ab, ΔL*, Δa*, and Δb* were calculated according to the CIE standard (DIN EN ISO 11664-Citation4 Citation2012).
For the analyses performed in 2012, the error of the colour values was calculated as standard deviation of multiple measurements. The errors of the CIE L*a*b* measurements were between 0.1 and 6.3 for L*, 0.01 to 0.38 for a* and 0.04 to 1 for b*, except for artificially aged areas of T74 and T75 which had higher errors between 13 and 21 for L*, 6 and 15 for a* and 13 to 29 for b*. These samples were suffering from much greater heterogeneities after ageing (see ), which is the reason for the large standard deviations.
For the analyses performed in 1984, there were no associated error values available (Agnini, Venchiarutti, and Massa Citation1985).
ATR-FTIR analysis
The samples were analysed by means of ATR-FTIR in order to identify the main component present in each conservation material. A small amount of each material was sampled with a clean scalpel. The infrared spectra were recorded with an IR spectrometer model ‘Spectrum One’ (Perkin Elmer) with the Universal ATR Sampling Accessory, in the range of 4000–550 cm−1. The resolution was 4 cm−1. The obtained spectra of the samples were compared with reference spectra from commercial (S.T. Japan) or customer databases, or with the IRUG Database. FTIR also helps evaluate the extent of chemical change upon ageing. The results of these analyses will be published elsewhere.
Results and discussion
Characterisation of the main polymeric component by means of FTIR
Using FTIR, the main components of the products tested were characterised. This study aimed solely at the identification of the polymeric component; differences between the artificially and naturally aged samples will be published elsewhere at a later point. The results of the spectroscopic analysis of the samples are provided and discussed below and sorted by the chemical composition of the adhesives.
Epoxy resins (T58: Plastogen® EP, T59: HXTAL NYL-1, T77: Uhu® Plus Endfest)
According to the literature (Tennent Citation1979; Down Citation1984), Plastogen® EP and Uhu® Plus Endfest are both epoxy resins resulting from the reaction of the diglycidyl ether of bisphenol A (DGEBA) with an amine hardener. The latter is a fatty polyamide of high amine value in the case of T77; dibutyl phthalate (DBP) and silica have also been reported as resin additives in this product (Down Citation1984). The reported structure is confirmed in the infrared spectra () of samples T58 (Plastogen® EP) and T77 (Uhu® Plus Endfest) as based in the main bands of the spectra (Chen, Bulkin, and Pearce Citation1982; Zhang Citation1994), namely a broad band at around 3390–3320 cm−1 (N-H and O-H stretching), a weak band at 3040 cm−1 (Ar C-H stretching), bands at 2925 and 2870–2855 cm−1 with several shoulders (C-H symmetric and asymmetric stretching of CH2 and CH3), 1608 and 1510 cm−1 (Ar ring modes: quadrant stretching and semicircle stretching of p-benzene ring, respectively), 1462 cm−1 (asymmetric deformation CH3 and CH2 def), several weak bands at 1417, 1385, and 1364 cm−1 (corresponding to Ar ring mode and CH3 deformations, respectively), 1295 cm−1 (C-N stretching, Ar amine), a strong, broad band at 1240–1235 cm−1 (C(Ar)-O-C and C-N stretching, Ar amine), 1184 cm−1 [C-H in-plane deformation (Chen, Bulkin, and Pearce Citation1982) or Ar ring mode (Zhang Citation1994)], 1106 and 1038 cm−1 (C-O stretching alkyl and aryl, respectively), and 830 cm−1 (C-H Ar, out of plane deformation of p-benzene ring). The band at 1726 cm−1 could correspond to the reported DBP which, as mentioned earlier, is present in these products; its content seems to be higher in sample T77 (Toja Citation2012).
The sample T59 (HXTAL NYL-1) shows a different spectrum than the previous samples. The spectrum is dominated by a very intense band at 1087 cm−1, bands in the C–H stretching region (2934, 2861 cm−1), and further bands at 1448, 1370, 747, and 3396 cm−1. All bands characteristic of an aromatic moiety that were visible in the spectra of samples T58 and T77 (3040, 1608, 1510, 1240, 1184, 1038, and 830 cm−1) are notably missing. The Material Safety Data Sheet for HXTAL NYL-1Footnote1 and more recent literature (Sideridou, Vouvoudi, and Papadopoulos Citation2016) describe the major components of HXTAL NYL-1 as being an epoxy resin with CAS number 30583-72-3, namely hydrogenated bisphenol-A (Part A) lacking aromatic groups, and, in Part B, the hardener, a substance with CAS number 39423-51-3 in a concentration of up to 92% (an amine-terminated polyether) and an imidazole (Down Citation1996). In the final, cured epoxy resin T59, therefore, ether groups and aliphatic moieties prevail, explaining the spectrum and the differences asymmetric deformation the other epoxy resins.
Acrylates (PM9: Paraloid® B-72, N80a: Plastogen® G)
Sample PM9 corresponds to Paraloid® B-72, which has been reported to be a methylacrylate (MA) – ethylmethacrylate (EMA) copolymer with 30/70 w/w % (Favaro Citation2006); it should be noted, however, that the proportion of the monomers, as well as the material composition, are known to have changed over time (Horie Citation2010). The reported structure is in accordance with the FTIR spectrum (), with characteristic bands at 1723 cm−1 (C=O stretch), 1476 cm−1 [C-H, α-CH3, asymmetric deformation, EMA (Favaro Citation2006)], 1448 cm−1 (C-H, CH3 in COOR, asymmetric deformation, EMA), 1387 cm−1 (CH3 in COOR, symmetric deformation, EMA), 1368 cm−1 (C-H, α-CH3, symmetric deformation, EMA), 1266 and 1236 cm−1 (C-O stretching, MA), shoulders at 1190 and 1163 cm−1, and a band at 1142 cm−1 (C(=O)O-C and C-C(=O)-O asymmetric stretching, MA and EMA), 1024, 861, and 753 cm−1 (diverse rocking and wagging deformations) and match with spectra previously reported. The sample N80a, described by the producer as a methacrylate, fits well with the reference spectra of a polymethyl methacrylate, as based on bands at 2994 cm−1 (C-H, CH3 asymmetric stretching), 2951 cm−1 (C-H, CH2 asym st), shoulders at 2930 and 2846 (C-H, asymmetric stretching of CH3 and CH2, respectively), 1724cm−1 (C=O stretching), 1483 and 1435 cm−1 (C-H, CH2 asymmetric and symmetric deformations in-plane), 1447 and 1386 cm−1 (C-H, CH3 asymmetric and symmetric deformations in-plane), 1240 cm−1 ([C-C(=O)-O] stretching), 1190 and 1144 (-O-C- stretching) (Chércoles Asensio Citation2009) and further bands at 986, 841, and 749 cm−1 (Learner Citation2004).
Polyvinyl acetates (T67: Vinavil® S, T70: Vinavil® Adesivo Universale, T71: Vinavil® Rapid, T73: Arkolyn 1110)
The spectra of the naturally aged samples T67, T70, T71, and T73 () show the typical bands of polyvinyl acetate. The most useful bands for the identification are those at 1731 (C=O stretching), 1433 (C-H, asym CH3 deformation), 1371 (C-H, symmetric CH3 stretching), 1225 (C-O st), and 1018 cm−1 (C-O st). In addition, a band at 748 cm−1 can be observed, which is not always present in reference PVAc spectra consulted, and which corresponds to a phthalate plasticiser, probably DBP (Toja Citation2012). Further bands indicating the presence of phthalate can be found at 1601, 1581, 1472 (shoulder), 1120, and 1075 cm−1. These bands are more intense in the spectra of T70 and T73, less so in T71, and are not visible in T67. All samples show otherwise a very similar spectrum, except for sample T71-TQ, which shows an additional band at around 1595 cm−1, whose intensity changes considerably throughout the sample. The origin of this band could not yet be identified with certainty, but it could be indicative of the presence of sodium carboxymethylcellulose (Down Citation2015). As described by Agnini, Venchiarutti, and Massa (Citation1985), the difference among the different Vinavil® products is both their curing time as well as their adequacy and adjustment to the different substrates.
Cellulose nitrates (T74: HMG adhesive, T75: AGO® Goldsiegel Kleber)
The spectra of the naturally aged samples (‘TQ’) show for both materials cellulose nitrate as the main component (), as based among other bands on those at 1642 (NO2, asymmetric stretching), 1276 (NO2, symmetric stretching), 829 (O-NO2, symmetric stretching) cm−1 (Wei Citation2018); only small differences are visible. The main difference observed is the higher intensity of the band at 1714 cm−1 for sample T74 (HMG adhesive), which points to a higher phthalate plasticiser content in this product. The phthalate plasticiser content is further supported by the presence of bands at 2964, 1490, and 650 cm−1, and shoulders at 1600, 1581, and 1073 cm−1 (Toja Citation2012).
Solubility and reversibility
The results of the tests are discussed below, arranged according to the main component of the adhesive products, and shown in . In , grey shading indicates %SOL and white shading % INS, respectively, the fraction of the aged material which was found to be soluble (%SOL) or insoluble (%INS) in the so-called solubility test.
Figure 9. Results of the solubility test (Method A; grey: % SOL and white: % INS; see text and Experimental methods). Different solvents were used for each case (epoxy resins: DCM; acrylates, vinyl acetates, and cellulose nitrates: acetone; see text). The TQ areas were not artificially aged in 1984 but were so when the analyses were carried out in 2012. TQ: not artificially aged, only naturally aged; the numerals 100, 200, and 500 indicate the number of hours of artificial aging undergone by the samples in 1984.
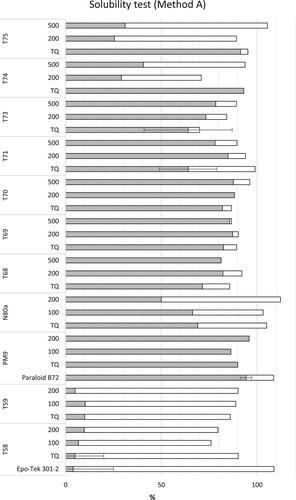
Epoxy resins (T58 Plastogen® EP, T59 HXTAL NYL-1)
Method A (solubility test)
Both studied materials (Plastogen® EP, HXTAL NYL-1) are, as can be expected of epoxy resins, insoluble in DCM (less than 10% SOL with respect to the starting mass). This insolubility remains almost constant with ageing time, as shown in . The results of a fresh sample of Epo-Tek (‘Epo-Tek 301-2’) are also presented in the graph for comparison; this test served as a measure of the reversibility of the fresh material before ageing.Footnote2 This material, new and unaged, was also shown to be insoluble in DCM.
Method B (reversibility test)
Following Method B for the epoxy resins, the sole use of DCM or H2O / 60 °C (without scratching) was unsuccessful for T58, T59, and T77, as could be expected from the results of the solubility tests (Method A). By scratching, after the application of the solvent (whether DCM or warm water), the removal of the material was facilitated. For all samples, it was easier to remove the material after treatment with DCM than after treatment with water ().
The artificially aged samples (‘200’) of T58 and T59 were slightly more difficult to remove with DCM than the TQ areas (naturally aged; ); the biggest difference in removability could be observed for T59 (from level 1-scalpel for TQ to level 3-scalpel for ‘200’). However, sample T77 shows the opposite removability (from level 2-scalpel for TQ to level 1-scalpel for ‘200’). This change shows the advantage of the reversibility test approach as opposed to the solubility test; the change in the polymer network is probably too small to change the solubility in the latter but seems enough to change the performance of the material during the swelling and/or mechanical removal. As mentioned in the Introduction, UV-aged samples are generally more easily removed (Down Citation2001). In the present work, this ability seems to be dependent on the specific product tested. Indeed, some authors cited by Down (unpublished report by Scott in Down Citation2001) reported an increase in the time to separate two glued glass slides with chlorinated solvents. The particular ageing conditions (natural, artificial; lightning conditions) undergone by the sample may also play a role.
Acrylates (PM9: Paraloid® B-72, N80a: Plastogen® G)
Method A (solubility test)
Paraloid® B-72 is soluble in the chosen solvent (acetone) () without major changes in the solubility upon ageing (the results of an unaged sample of Paraloid® B-72,Footnote3 prepared in 2012, are also presented in the graph). Plastogen® G shows a different behaviour: after natural ageing its solubility is not complete, as indicated by the evaluation of TQ, for which only around 70% of the original material is solubilised. The solubility in acetone decreases with artificial ageing and goes down to 50%.
Method B (reversibility test)
This test could only be performed on sample PM9 (i.e. Paraloid® B-72) using acetone. The solubility of this product was still very high, as shown by the results obtained using Method A. The solubility of the samples TQ and 200 are very similar despite the different ageing undergone in 1984. After only Level 1-swab using Method B, the adhesive layer could be entirely removed ().
Vinyl acetates (T67-T72 different Vinavil® products, T73 Arkolyn 1110 Rapido)
Method A (solubility test)
For these samples, Method A was performed in acetone. The solubility of some of the naturally aged samples in this solvent (see TQ of T71, T73, ) was lower (ca. 30–40%) than for the samples aged artificially in 1984 for 200 and 500 h of irradiation time (Agnini, Venchiarutti, and Massa Citation1985); for these materials, the samples which were submitted to artificial ageing were mostly soluble at the time of analysis. For the rest of the samples (T68, T69, and T70), no major differences were found in the behaviour between artificially and naturally aged samples, solubility being high. T68 showed in this respect an intermediate behaviour between the latter (T69 and T70) and the former samples (T71 and T73), the artificially aged samples being slightly more soluble compared to the only naturally aged ones.
Method B (reversibility test)
For this test, H2O / 60 °C was chosen to soften the material. When carried out without scratching, warm water was not enough to remove the material in areas naturally aged (TQ) of samples T67, T70, T71, and T73. In general, this was also the case for areas artificially aged (200 and 500 h). Only for sample T71 (for 500 h artificial ageing) could the material be removed with difficulty (Level 5-swab, see ).
Interestingly, for samples T70, T71, and T73, the removal was slightly harder upon increase in artificial ageing time (from naturally aged sample TQ to 200 and 500 h artificial ageing), as opposed to the observation for the Method A tests using acetone.
Cellulose nitrate (T74 HMG, T75 AGO® Goldsiegel)
Method A (solubility test)
The solubility of the naturally aged (TQ) areas for cellulose nitrate materials in acetone was maintained upon dark storage (). Artificially aged samples, however, were less than 50% soluble. It should be noted that there is a high error associated to the measurements of the cellulose nitrate samples. Due to the small amount of material available the test could only be performed once (owing to the advanced degradation stage of the samples, see ).
Method B (reversibility test)
Naturally aged areas (TQ) of sample T74 (HMG) could be easily removed at level 2-swab using a cotton swab dampened with acetone, without the need for using a scalpel. The removal was hindered in artificially aged samples. The solubility was further reduced for 200 h of ageing (Levels 2–5-swab of treatment needed to be reached) and 500 h of artificial ageing time (Level 4-swab, see ). The high variability in the 200 h aged sample is due to the advanced stage of degradation of the sample and the heterogeneous sample surface. The reason for the heterogeneity of the surface is unknown but is likely linked to the sample ageing (see ). For the artificially aged samples, presence of darker spots of aged material on the surface of the coatings prevented the complete removal of the material. The use of a scalpel after application of the solvent was necessary to completely remove the material (Level 1-scalpel both for 200 and 500 h artificial ageing).
Colour change
The available sets of values obtained from the colour analyses enable several comparisons that are of interest to conservators. First, the set of values from 1984 (Agnini, Venchiarutti, and Massa Citation1985) gives a measure of the change in colour induced by artificial ageing of the different products. Second, the set of values of 2012 evaluates the colour change occurring upon natural ageing by investigating the areas named TQ. These areas were not artificially aged in 1984 but have been naturally aged in the dark during 28 years until 2012. The comparison of colour values for TQ from 1984 for a specific product with the values for the same samples in 2012 helps to identify the materials less prone to colour change upon natural ageing. In this work, however, the direct calculation of a ΔE*ab between these values was not considered due to the methodological differences in the colour measurements of 1984 and 2012. Instead, a comparison of the absolute values of b* for both sets of values led to some interesting observations, as will be discussed below.
Additionally, it has to be noted that the colour changes observed for the samples upon artificial light ageing (UV-VIS radiation) cannot be compared to the behaviour upon natural ageing in the dark (i.e. through the comparison of samples ‘TQ 1984 vs. TQ 2012’ vs. ‘TQ 1984 vs. 200/500 h 1984’). This is due to the differences in ageing mechanisms and subsequent chemical reactions induced in each case.
Results from 1984. Change in colour upon artificial ageing
The results for the colour analyses of 1984 are summarised in . In 1984, all ΔE*ab values were below 5, even for 500 h artificial ageing, except for sample T58, the epoxy resin Plastogen® EP (Agnini, Venchiarutti, and Massa Citation1985). As a comparison, the other epoxy resin tested, HXTAL, showed a negligible colour change upon 200 h of artificial ageing. Sample T74, the HMG heat waterproof cellulose nitrate adhesive, shows the next biggest colour change upon ageing (500 h), with a ΔE*ab of 3. For both T58 and T74, the change in ΔE*ab is mainly due to a positive change in Δb*, i.e. a yellowing. Regarding the colour of the fresh material before ageing, the b* values for the vinyl acetates T70 and T73 are higher than for the rest of the samples. A similar slight colour change as for all the other samples (again excluding the already mentioned T58 and T74), was, however, measured after artificial ageing. The ΔE*ab and Δb* values are also shown in and .
Figure 10. ΔE*ab between TQ and 200 h of the samples from 1984 (white) and 2012 (grey). For samples T74 and T75, the 2012 values are associated with a high error due to the heterogeneity of the samples (see and Experimental section).
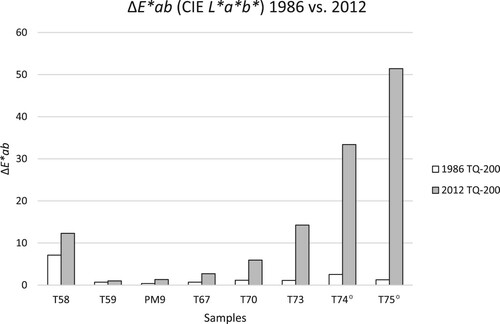
Figure 11. Δb* between TQ and 200 h of the samples from 1984 (white) and 2012 (grey). For samples T74 and T75, the 2012 values are associated with a high error due to the heterogeneity of the samples (see and Experimental section).
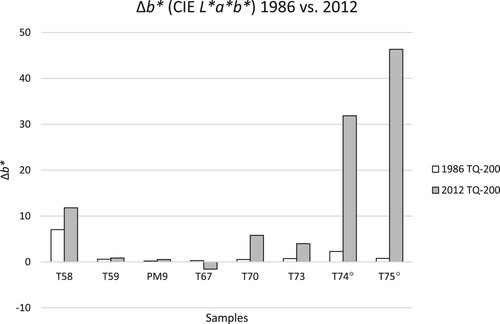
Table 3. Results of the 1984 colour analyses after artificial ageing (100:100 h, 200:200 h, 500:500 h).
Results from 2012. Effect of natural ageing on the samples
In , the values of the 2012 colour measurements are given (see also and ). For most samples, the main changes in ΔE*ab are due to a change in Δb* in both 1984 (Agnini, Venchiarutti, and Massa Citation1985) and 2012. The ΔE*ab between TQ and the area of the sample artificially aged for 200 h is the smallest for PM9 (Paraloid®) and the epoxy resin T59; this is true for both sets of values (1984 and 2012).
A comparison of the b* values of the TQ areas () shows that the variability of the b* data for the TQ of both sets of values (1984 and 2012) is similar, ranging between 0.6–2.3 and −4.1–2.0, respectively. Low absolute values (close to zero) for both sets of samples suggest that the naturally ageing did not have much effect on the yellowing of the products. This agrees with the colourless appearance that these samples still displayed in 2015,Footnote4 after almost 30 years of natural ageing in the dark.
For the samples T58 and T59, 28 years of additional natural ageing had a smaller effect than the initial artificial ageing (). In both ageing steps, sample T58 shows a much higher colour difference compared to T59 (both epoxy resins). Upon further natural ageing in the dark, the epoxy resin T58 further yellows, as do most epoxy resins (Down Citation1984). Interestingly, the sample T59 (HXTAL NYL-1) does not show this behaviour, which is supported by past studies (Down Citation1984, Citation2001; Sideridou, Vouvoudi, and Papadopoulos Citation2016); it was less affected by the 200 h artificial aging and remained almost colourless after long-term ageing in the dark. The different structure of T59 as opposed to T58, particularly the lack of aromatic moiety in the diglycidyl ether, is likely the reason for the higher stability of HXTAL NYL-1 to light exposure (Sideridou, Vouvoudi, and Papadopoulos Citation2016).
The vinyl acetates and the cellulose nitrates all show a significant increase in the ΔE*ab values after natural ageing, particularly in the latter case. For these samples, the ΔE*ab values between the TQ-areas and the artificially aged areas increased by over 30 for T74 and over 50 for T75 between 1984 and 2012. The exposure to the light source used in 1984 seems to have triggered processes which further proceeded in the darkness, and which also rendered the samples insoluble (see above).
In the case of the vinyl acetate T67, the increase in ΔE*ab is, unlike for all other samples, not due to an increase of the Δb* value (yellowing) but to a combined decrease in ΔL* as well as in Δb*. T67 is the only polyvinyl acetate tested that did not display bands attributable to additives in its FTIR spectrum.
PM9 proved to have the highest stability in terms of artificial and natural ageing, which confirm previously reported results well accepted among the conservation community (Horie Citation2010).
It should be reminded at this point that the artificial ageing performed in 1984 cannot be used to predict the colour changes which would take place under natural ageing in the dark. This is particularly important when considering samples T74 and T75 for which discrepancies between the colour changes associated with natural and artificial ageing were mentioned earlier.
Conclusions
Upon dark natural ageing, HMG heat water-proof adhesive (T74a), AGO® Goldsiegel Kleber (T75a), and Paraloid® B-72 (PM9), as well as the vinyl acetates Vinavil® NPC stella bianca (T69) and Vinavil® Adesivo Universale (T70), were easily removed using solvents; in comparison, other vinyl acetates such as Vinavil® S (T67), Vinavil® Rapid (T71), and Arkolyn 1110 Rapido (T73) showed a lower solubility upon natural ageing. The acrylate Plastogen® G (N80a), a homopolymer of methyl methacrylate, is less soluble than the MA/EMA copolymer Paraloid® B-72, whether after artificial or natural ageing in the dark. Paraloid® B-72 is known for remaining soluble and not degrading significantly in typical indoor exposure conditions; as such, it has been classified as a Feller Class A material (Horie Citation2010). Among the epoxy resins, HXTAL NYL-1 did not significantly change in colour upon dark ageing after 28 years, regardless of whether the sample had been artificially aged in 1984 or not.
The epoxy resins Plastogen® EP (T58), HXTAL NYL-1 (T59), and Uhu® Plus Endfest (T77) could only be removed completely by mechanical action after softening in DCM, as expected for this material group. Here, a slight easing of the removal process of artificially aged samples could be noticed for T77, while in T58 and T59 the TQ areas aged in the dark were easier to remove than their artificially aged counterparts. Interestingly, of all the samples tested, only the cellulose nitrates showed a considerable difference in solubility between the naturally and the artificially aged samples in 2012.
The present results obtained on the naturally aged samples can be considered relevant for understanding the ageing of adhesives used on ceramics located in areas protected from light (e.g. inner parts, areas within cracks). Considering the overall results of this study, Paraloid® B-72 shows the lowest yellowing over time by simultaneously having good solubility and reversibility properties. This study also shows that natural ageing of unexposed areas (TQ) over 28 years in the dark had a small, if any, impact on the colour of the studied conservation products. The artificially aged samples underwent further degradation over 28 years in the dark that can be evidenced by much stronger discolorations, which cannot be prevented by optimised storage conditions.
Further work will consider the exposure of the samples to controlled lighting museum conditions, and a subsequent re-evaluation of the colour and reversibility of the materials. Complementary analysis of original material used for the conservation of the museum objects in 1984 should also be performed. The evaluation of the extent of chemical change upon ageing by ATR-FTIR analyses is forthcoming.
Acknowledgements
The preparation and the evaluation of the samples 1984–1986 was conducted in the research center LARAC S.p.A. in Castellanza (Montedison, Italy) by Diego Venchiarutti, Giorgio Pin, Franco Gulani, and Vincenzo Massa. The authors are indebted to Jorge Chamón, Cristian Mazzon, and Isabella del Gaudio for reviewing the manuscript and visual evaluation of the samples in 2015. The authors would especially like to thank the University of Applied Science Potsdam for the use of the ATR-FTIR spectrometer. The 2012 analyses presented in this work were carried out in the Rathgen Research Laboratory of the National Museums Berlin, which is here gratefully acknowledged.
Disclosure statement
No potential conflict of interest was reported by the author(s).
Notes
1 HXTAL Adhesive, LLC., revision date 11/10/10; a most recent document can be downloaded from https://www.msdsdigital.com/hxtal-nyl-1-epoxy-adhesive-part-resin-part-b-hardener-msds (Accessed September 9, 2021).
2 It should be kept in mind that the composition of this sample is not necessarily the same as that of the original samples of 1984.
3 Röhm and Haas.
4 In 2015, all samples were visually examined separately by four different people; of all the samples in this study, only N80a and N80b showed a distinct yellow hue.
References
- Agnini, E., G. P. Venchiarutti, and V. Massa. 1985. “Interventi di restauro su ceramiche e vetri. Caratterizzazioni di prodotti per restauro di ceramiche.” In ‘Fatti come nuovi’. Restauri di oggetti d’arte applicate nel Museo Poldi Pezzoli, edited by M. T. Balboni Brizza, A. Mottola Molfino, and A. Zanni, 40–49. Centro Di: Milano.
- Bracci, S., and M. J. Melo. 2003. “Correlating Natural Ageing and Xenon Irradiation of Paraloid® B72 Applied on Stone.” Polymer Degradation and Stability 80 (3): 533–541. doi:10.1016/S0141-3910(03)00037-5.
- Chen, C. S., B. J. Bulkin, and E. M. Pearce. 1982. “New Epoxy Resins. II. The Preparation, Characterization, and Curing of Epoxy Resins and Their Copolymers.” Journal of Applied Polymer Science 27 (9): 3289–3312. doi:10.1002/app.1982.070270909.
- Chércoles Asensio, R. 2009. “Analytical Characterization of Polymers Used in Conservation and Restoration by ATR-FTIR Spectroscopy.” Analytical and Bioanalytical Chemistry 395 (7): 2081–2096. doi:10.1007/s00216-009-3201-2.
- Chiantore, O., and M. Lazzari. 2001. “Photo-Oxidative Stability of Paraloid Acrylic Protective Polymers.” Polymer 42 (1): 17–27.
- Coutinho, I. 2009. “Studies of the Degradation of Epoxy Resins Used for the Conservation of Glass.” In Holding it All Together, Ancient and Modern Approaches to Joining, Repair and Consolidation, edited by J. Ambers, 127–133. London: Archetype Publications.
- Dandridge, P. 2005. “The Exhibition of Unlacquered Silverat the Metropolitan Museum of Art.” Journal of the American Institute for Conservation 44 (3): 175–183. doi:10.1179/019713605806082257.
- de la Rie, E. R. 1988. “Photochemical and Thermal Degradation of Films of Dammar Resin.” Studies in Conservation 33 (2): 53. doi:10.2307/1506303.
- Derschau, D., and A. Unger. 1998. “Epxydharz-Restaurierungen – zum Problem der Entfernung.” Restauro 104 (7): 486–493.
- DIN 5033-7. 1983. Farbmessung – Meßbedingungen für Körperfarben. Berlin: Beuth Verlag.
- DIN EN ISO 11664-4. 2012. Farbmetrik – Teil 4: CIE 1976 L*a*b* Farbenraum (ISO 11664-4:2008). Berlin: Beuth Verlag.
- Down, J. L. 1984. “The Yellowing of Epoxy Resin Adhesives: Report on Natural Dark Ageing.” Studies in Conservation 29 (2): 63–76.
- Down, J. L. 1996. “The Yellowing of Epoxy Resin Adhesive: Report on High-Intensity Light Ageing.” Studies in Conservation 31 (4): 159–170.
- Down, J. L. 2001. “Review of CCI Research on Epoxy Resin Adhesives for Glass Conservation.” Studies in Conservation 46 (Suppl. 1): 39–46. doi:10.1179/sic.2001.46.Supplement-1.39.
- Down, J. L. 2015. “The Evaluation of Selected Poly(Vinyl Acetate) and Acrylic Adhesives: A Final Research Update.” Studies in Conservation 60 (1): 33–54. doi:10.1179/2047058414Y.0000000129.
- Edge, M. 1990. “Mechanisms of Deterioration in Cellulose Nitrate Base Archival Cinematograph Film.” European Polymer Journal 26 (6): 623–630.
- Elsässer, C. 2021. “Selection of Thermal, Spectroscopic, Spectrometric, and Chromatographic Methods for Characterizing Historical Celluloid.” Journal of Applied Polymer Science 138 (21): 50477. doi:10.1002/app.50477.
- Favaro, M. 2006. “Evaluation of Polymers for Conservation Treatments of Outdoor Exposed Stone Monuments. Part I: Photo-Oxidative Weathering.” Polymer Degradation and Stability 91 (12): 3083–3096. doi:10.1016/j.polymdegradstab.2006.08.012.
- Feller, R. L. 1994. Accelerated Aging: Photochemical and Thermal Aspects. Marina del Rey, CA: Getty Conservation Institute. (Research in conservation, 4).
- Fulton, M. I. 1998. “Colour Development and Luminescence Phenomena in Epoxy Glasses.” Polymers for Advanced Technologies 9: 9.
- Grissom, C. A. 2013. “Evaluation of Coating Performance on Silver Exposed to Hydrogen Sulfide.” Journal of the American Institute for Conservation 52 (2): 82–96. doi:10.1179/0197136013Z.0000000007.
- Horie, C. V. 2010. Materials for Conservation: Organic Consolidants, Adhesives and Coatings. London: Butterworths.
- ‘Hunter L, a, b Color Scale’. 2008. Application Note, 8(9). Accessed 10 December 2019. https://www.hunterlab.se/wp-content/uploads/2012/11/Hunter-L-a-b.pdf.
- Koob, S. P. 2006. Conservation and Care of Glass Objects. London: Archetype Publications. Accessed 9 June 2021. https://www.talasonline.com/Conservation-and-Care-Of-Glass-Objects.
- Lazzari, M., and O. Chiantore. 2000. “Thermal-Ageing of Paraloid Acrylic Protective Polymers.” Polymer 41 (17): 6447–6455.
- Learner, T. J. S. 2004. Analysis of Modern Paints. Los Angeles: Getty Conservation Institute.
- Luoma, G. A., and R. D. Rowland. 1986. “Environmental Degradation of an Epoxy Resin Matrix.” Journal of Applied Polymer Science 32 (7): 5777–5790. doi:10.1002/app.1986.070320710.
- Mailhot, B. 2005. “Study of the Degradation of an Epoxy/Amine Resin, 1: Photo- and Thermo-Chemical Mechanisms.” Macromolecular Chemistry and Physics 206 (5): 575–584. doi:10.1002/macp.200400395.
- Marquardt, A. E. 2015. “Protecting Silver Cultural Heritage Objects with Atomic Layer Deposited Corrosion Barriers.” Heritage Science 3 (1): 37. doi:10.1186/s40494-015-0066-x.
- Melo, M. J. 1999. “Photodegradation of Acrylic Resins Used in the Conservation of Stone.” Polymer Degradation and Stability 66 (1): 23–30. doi:10.1016/S0141-3910(99)00048-8.
- Quye, A. 2011. “Accelerated Ageing to Study the Degradation of Cellulose Nitrate Museum Artefacts.” Polymer Degradation and Stability 96 (10): 1934–1939.
- Reedy, C. L. 1999. “Evaluation of Three Protective Coatings for Indoor Silver Objects.” Armerican Institute of Conservation Objects Specialty Group Postprints 6: 41–69.
- Selwitz, C. 1988. Cellulose Nitrate in Conservation. Marina del Rey: Getty Conservation Institute.
- Shashoua, Y., S. M. Bradley, and V. D. Daniels. 1992. “Degradation of Cellulose Nitrate Adhesive.” Studies in Conservation 37: 113–119.
- Sideridou, I. D., E. C. Vouvoudi, and G. D. Papadopoulos. 2016. “Epoxy Polymer HXTAL NYL-1TM Used in Restoration and Conservation: Irradiation with Short and Long Wavelengths and Study of Photo-Oxidation by FT–IR Spectroscopy.” Journal of Cultural Heritage 18: 279–289. doi:10.1016/j.culher.2015.09.005.
- Tennent, N. H. 1979. “Clear and Pigmented Epoxy Resins for Stained Glass Conservation: Light Ageing Studies.” Studies in Conservation 24: 153–164.
- Toja, F. 2012. “The Degradation of Poly(Vinyl Acetate) as a Material for Design Objects: A Multi-Analytical Study of the Effect of Dibutyl Phthalate Plasticizer. Part 1.” Polymer Degradation and Stability 97 (11): 2441–2448. doi:10.1016/j.polymdegradstab.2012.07.018.
- Wei, R. 2018. “Effect of Plasticizer Dibutyl Phthalate on the Thermal Decomposition of Nitrocellulose.” Journal of Thermal Analysis and Calorimetry 134 (2): 953–969. doi:10.1007/s10973-018-7521-3.
- Zhang, G. 1994. “Studies on Oxidative Photodegradation of Epoxy Resins by IR-ATR Spectroscopy.” Journal of Applied Polymer Science 54 (4): 419–427. doi:10.1002/app.1994.070540402.