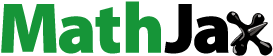
ABSTRACT
Medieval wall paintings in the church of the monastery of St. John in Müstair (Switzerland) have been exposed to several fires over the last centuries. Non-invasive (hand-held-XRF) and microinvasive (PLM, SEM-EDX, XRPD, µ-Raman, and SERS) techniques were used to analyse 14 painted fragments from the Romanesque (c. 1200 CE) painting cycle recovered from the attic of the church above younger Gothic vaulting in order to study the microstratigraphy of the painted surfaces and determine alterations and newly formed products, with the aim of understanding if the darkening was directly attributable to contact with fire. Scientific, historical, and bibliographical research have shown that alteration processes in the pigments from these paintings are not directly linked with fires, except possibly for two lead-based painted fragments. This indicates that the action of the historically documented fires in the church was not uniform and did not affect all areas of the paintings equally.
Introduction and aim of the research
The Benedictine Convent of St. John in Müstair is located in the southeast part of the Swiss canton of Grisons, close to the border with Italy. In 1983, it was inscribed on the UNESCO World Heritage List. Dendrochronological dates obtained from the remnants of wooden wall plates from the roof of the church indicate that its construction was completed around 775 CE (Hurni, Orcel, and Tercier Citation2007). It has been assumed that the mandate for the establishment of the monastery came from Charlemagne, who was crowned King of the Lombards in 774, and wanted to control the alpine routes between the Kingdom of the Franks and the Italian peninsula (Goll, Exner, and Hirsch Citation2007). The monastery occupies a strategic position for the control of the mountain passes of Umbrail, Fuorn, and Resia, which lead respectively to Valtellina, Engadina, and the Austrian upper Inn valley.
The main church is located on the eastern side of the complex and preserves wall paintings dating to the Carolingian period. Sometime during the twelfth century, the monastery was converted into a convent. After this event, in around 1200 CE, the apses and the eastern wall were decorated with new wall paintings, created on a new plaster over the pre-existing one (Goll, Exner, and Hirsch Citation2007).
Over the centuries, several fires occurred in the monastery and consequently the church was notably damaged three times. The first fire is attested as occurring in the first half of the tenth century and affected the the part of the church featuring the Carolingian wall paintings. The same cycle was probably affected by another fire in the first quarter of the eleventh century. In 1492, Gothic vaulting was erected inside the church. Part of the early Medieval wall paintings came thus to be located above the vaults, in the attic of the church. The Gothic vaults protected the interior of the church when the last fire broke out in 1499. This fire therefore affected only the roof and the attic, and those portions of the wall paintings located there (Goll, Exner, and Hirsch Citation2007). In 1908, the fire-damaged Romanesque and Carolingian wall paintings located above the Gothic vaults in the attic were removed and taken to the Swiss National Museum in Zurich, where they remain to this day (Wüthrich Citation1980; Flühler-Kreis Citation2002).
Past and recent research on the condition and composition of the paint layers of the Carolingian and Romanesque pictorial cycles (Emmenegger Citation1986; Mairinger and Schreiner Citation1986; Leandri Citation2018; Cavallo et al. Citation2020) revealed a general alteration and deterioration of the painted surfaces. These processes have been described as ‘fading’, ‘blackening’, ‘converting’, and ‘loss’ of pigments. Several causes have been proposed. Emmenegger (Citation2002) pointed out that the removal of limewash and plaster which covered the Carolingian wall paintings caused the loss of the uppermost paint layers. He also mentions that water ingress after fires damaged the roof (Citation1986). Mairinger and Schreiner (Citation1986) suggested that lead white could have been converted to yellow lead oxide (massicot) through the heat of the fire. The action of fire is also proposed as an explanation for the lack of yellow and brown earth colours on the Carolingian wall paintings in the nave of the church by Emmenegger (Citation2002). However, no systematic and specific scientific analyses have been attempted until now. Furthermore, the focus of the aforementioned studies lay on the Carolingian paintings, since they are the most altered and damaged. Although the environmental conditions to which the Carolingian and Romanesque paintings have been subjected are identical, the painting techniques and later treatments are fundamentally different. This means that the insights gained into the alteration and deterioration processes and mechanisms of the Carolingian wall paintings cannot be directly applied to the Romanesque ones without consideration of the material and technical differences between these two groups of paintings.
Fire is one of the most serious factors affecting the integrity of materials and structures of built heritage and can generate rapid and irreversible damage (Calia et al. Citation2016). A clear understanding of the alteration and deterioration processes occurring in the world-renowned painting cycles of the monastery is crucial in order to devise a long-term conservation strategy. It makes a big difference if the observed phenomena are due to singular events such as fires, which have ceased to act on the paintings, or if other, still active factors are involved. While the Carolingian paintings in the monastery church have been subjected to detailed analysis in the past as already mentioned, specific data on the deterioration of the Romanesque paintings is still lacking. Therefore, a research project was devised to determine the mineralogical composition and alteration products associated with the possible mechanisms behind the observed chromatic alterations of the Romanesque paintings and their microstratigraphic distribution (Cavallo et al. 2012). The scope of the research is to try to understand the extension of the altered surfaces at a microstratigraphic level discriminating whether the painted surfaces were altered by the action of the fires and/or by environmental factors such as exposure to light, alkaline environment of the plaster, or (inadequate) painting technique. Lead, copper, and iron-based pigments were selected as they represent the classes of painting materials mostly used in the Medieval cycle and which are more prone to alteration ().
Table 1. Pigments used in the Medieval mural paintings in the church of the monastery of St. John in Müstair (Switzerland) as reported in previous studies (Emmenegger Citation1986; Mairinger and Schreiner Citation1986; Leandri Citation2018).
The pigments considered in this study
Copper-based pigments. The pigments malachite [Cu2(CO3)(OH)2] and azurite [Cu3(CO3)2(OH)2] are products of copper ore alteration, found in nature in cupriferous ores. The minerals may darken if heated at temperatures in the range of 200–400°C, forming tenorite (CuO) (Frost et al. Citation2002; Baraldi and Bensi Citation2006). The almost complete decomposition of these pigments occurs at T = 600°C (Rutherford and West Fitzhugh Citation1993). The most frequent alteration is the chromatic modification from blue to green linked to the alteration of azurite into malachite or to one of the three isomers of basic copper chloride (atacamite, paratacamite, or clinoatacamite) (Mattei et al. Citation2008). The transformations of azurite into black products such as copper sulphide (CuS, covellite) or copper oxide (CuO, tenorite) are less frequent and consequently less studied. The formation of tenorite can be due to two different causes: the alkaline environment or heat (Gutscher et al. Citation1989; Mattei et al. Citation2008; Coccato, Moens, and Vandenabeele Citation2017).
Lead-based pigments. Since ancient times, these pigments have been used both as natural minerals and as synthetic products after heat treatment. Lead white, when heated at low temperatures, forms massicot; at higher temperatures minium and then litharge are formed (Rutherford, Kuhn, and Chase Citation1993) according to the following process (Baraldi and Bensi Citation2006; Calia et al. Citation2016; Coccato, Moens, and Vandenabeele Citation2017).
The darkening of lead-based pigments is mainly due to the formation of plattnerite (β-PbO2). The reaction is attributed to different causes, such as the exposure of lead to oxidising agents, the action of microorganisms, or the formation of galena (PbS) as a reaction product from the interaction of the pigment and sulphur from the atmosphere, microorganisms, or other pigments (Coccato, Moens, and Vandenabeele Citation2017; Vagninia et al. Citation2018). The formation of the minerals plattnerite (β-PbO2) and anglesite (PbSO4) after exposure of lead-based pigments to light and pollutants is also reported. Therefore, the factors controlling these alteration processes are environmental parameters, including humidity, temperature, and sulphate ion concentration (Aze et al. Citation2007).
Iron-based pigments. Natural earth pigments are the most used class of painting materials since prehistory, due to their availability and physical–chemical stability. Thermal transformation of (yellow-brown) goethite (α-FeOOH) into (red) hematite (α-Fe2O3) was an intentional practice over the centuries. This led to the modification of the mineralogical characteristics and physical–chemical properties of the original material (Gualtieri and Venturelli Citation1999). The transformation is topotactic and starts approximately at T = 200°C, according to the following reaction (Cavallo et al. Citation2018)
Red ochre pigment, on the contrary, can undergo alterations in the clay component (Baraldi and Bensi Citation2006). The thermal instability of yellow ochre and the wide use of this class of pigments as artificial products make it difficult to distinguish between accidental and intentional transformations when temperatures are over T = 600–650°C.
Another natural pigment that has been widely used since ancient times is green earth (K[(Al, FeIII),(FeII, Mg)] (AlSi3, Si4)O10(OH)2). It is an extremely stable pigment that can be used in all painting media. When exposed to high temperatures, the colour turns into a brown tone forming what is known as burnt green earth. This chromatic alteration may be due to the conversion of most of the iron from a divalent to a trivalent oxidation state. A study carried out on samples of burnt green earth shows that burnt and unburnt earths cannot be differentiated (Grissom Citation1986). Another study reported in the outcome section of the analytical thermography data of the Rufus’ House in the Archaeological Park of Pompeii highlights the transformations induced by high temperatures on a sample of Verona green earth. It was observed that the alteration of the green earth occurs at about 240°C, the same temperature range that influences the passage of goethite into hematite (Baraldi and Bensi Citation2006).
The scientific background shows that it is not possible to make a clear distinction between unintentional (fire action, alteration processes) and intentional (technological process of pigments’ production of Pb- and Fe-based artificial pigments widely used in Medieval times) transformations. In addition, the current research is based on the scientific analysis of (mostly monochrome) painted fragments derived from archaeological excavations () whose size and original placement in the figurative scene is unknown. This does not allow possible iconographic correlations (i.e. a blue drape). However, the microstratigraphic analysis (lateral continuity of the altered paint layer, relationship with other paint layers, mineralogical modification of the ground layer, and partial alteration of the pigment) is here used to understand the morphological and compositional features of the pictorial sequences and to formulate hypotheses on the darkening affecting the painted fragments.
Archaeological context of the samples
For the present study, 14 Romanesque painted fragments with iron, lead, and copper-based pigments from the attic of the church were selected (, ). The attic of the church was sealed off from the church through the construction of Gothic vaulting in 1492. The vaulting is lower than the original, flat ceiling of the church, therefore a part of the early Medieval and Medieval wall paintings came to be located above the vaulting. These sections of the paintings were subjected to the same destructive fires as those below the vaults until 1492. After this date, they have been exposed to different conditions from the rest of the painting cycle. This includes differences in climate such as temperature and humidity, as well as exposure to a fire in 1499, which was restricted to the roof of the church (Goll, Exner, and Hirsch Citation2007). As consequence of these events, part of the plaster has fallen off the walls onto the vaults.
Table 2. Description of the selected painted plaster fragments reported in and corresponding materials analysis carried out.
Another event that took place in 1908 and contributed to certain changes and deterioration was when the wall paintings in the attic were removed by strappo- and stacco-methods. The former consists of glueing a portion of canvas to the surface of the paintings and then ripping it off in order to remove the paint layers from the wall. The removed layers are then lined onto another canvas. The stacco technique works in a similar way, but in this case, the paintings are removed together with their plaster support. During this procedure, many fragments fell off the walls and the detached portions of the paintings joined those that came off in the past. The deposits of dirt on the top of the Gothic vaults were investigated archaeologically in 1993. The dirt was removed and sieved, and all recovered artefacts sorted, catalogued, and stored in boxes. This procedure yielded a large amount of painted plaster fragments, which were made available for the present study by the Foundation pro Monastery of St. John and the Archaeological Service of the Canton of Grisons.
Materials and methods
Considering the large number of fragments and the methodological approach already used for other similar studies, the analytical strategy was designed as a combination of preliminary visual observation and non-invasive analysis, followed by the selection of fragments for microstratigraphic research ().
The first step of the work was the opening of about 80 boxes containing painted fragments stored in the attic of the church. The preliminary selection of the fragments was carried out by visual examination. The Romanesque wall painting fragments are easily distinguishable macroscopically from the Carolingian fragments because of the features of the ground layer (plaster). The criteria used for differentiating between the two plaster groups were: colour, aggregate, and surface texture.
Hand-held X-ray spectrometry (HH-XRF) was then carried out in situ for the preliminary selection of painted surfaces (results not reported here) and then for the further selection in order to minimise the number of the samples for microinvasive analysis. A portable Niton Xl3t X-ray spectrometer was used with the following set-up: X-ray tube 50 kV (maximum); Ag anode, 40 mA (maximum); Si Pin diode X-ray detector, resolution 195 eV; minimum atomic number detected Z = 19 (K). HH-XRF was used both for a preliminary screening of the painted fragments and for the in-depth analysis of those selected.
Each of the 14 selected fragments was first observed with a hand-held digital microscope (Dino-lite), then microstratigraphic analysis was carried out combining polarising light microscopy (PLM) both in transmitted (TL) and reflection (RL) mode and scanning electron microscopy coupled with micro-analysis (SEM-EDX). For the observations under PLM, an Axioskop 40 (Zeiss) microscope was used; selected micrographs were captured with a digital camera using the software AxioCam 4.5. SEM-EDX analysis was carried out using a SEM Jeol 5910 LV; EDX IXRF-2000, LV conditions (with the exception of (b) captured in HV conditions using a Field Emission Gun Tescan Mira 3XMU-series with a working distance of 15.8 mm).
X-ray powder diffraction (XRPD) was carried out on small amount of powder taken with a scalpel from the fragments. After grinding it in an agate mortar, randomly oriented samples were prepared and deposited in the hollow of a Si monocrystalline zero-background plate, provided by Assing Spa, Monterotondo (Italy). A Rigaku Miniflex system was used, operating in θ:2θ mode: generator setting 30 kW, 10 mA, Cu anode (CuKα = 1.5418 Å), Ni filter, 2θ range 5–55°, step size 0.02° and scan speed 0.6°min−1. Qualitative phase determination was carried out with QualX2.0 software and the correlated COD database (Altomare et al. Citation2015).
µ-Raman and SERS analysis were also done. A Senterra dispersive Raman spectrometer (Bruker) combined with an Olympus BX51 microscope was used. The instrument is equipped with a CCD detector (1024 × 256 pixels) cooled with a Peltier cell. The analyses were carried out using an excitation wavelength of 785 nm and a 50× long focal lens. The spectra were acquired with a 400 line / mm grid, a laser power from 1 to 50 mW, and acquisition times from 5 to 2.5 s. SERS analysis was carried out by depositing a drop of silver colloidal solution on the surface of the fragment as it is. Silver colloids were prepared by chemical reduction of silver nitrate with sodium citrate, following the Lee and Meisel synthetic protocol (Lee and Meisel Citation1982).
Results
Hand-held X-ray spectrometry (HH-XRF)
shows only the results obtained for the fragments definitely selected and included in this study, after a general survey carried out on a larger number of samples executed in the attic of the church where all the fragments are stored in standard plastic boxes.
Table 3. HH-XRF analysis.
Ca was detected in all the readings since it is associated with the support layer(s) and the lime-based painting technique. The Ca-Kα counts are appreciably lower for the readings no. 14, 15, and 18 corresponding to those where Pb was detected. Fe was detected in all the readings. Fe-Kα higher counts/sec are closely correlated with the presence of orange (readings no. 29, 36, 56) and red (readings no. 23, 59, 65) Fe-based pigments (Fe-oxides and Fe-oxyhydroxides) whilst lower counts may be associated with preparation layers and/or sands used for mortar preparation.
Data collected on dark and black surfaces indicate the presence of Pb (readings no. 14, 15, 18) and Cu (readings no. 20, 21, 22, 26, 28, 31, 35), attesting respectively to the presence of (altered) lead- and copper-based pigments. Cu was also found on fragment 13 characterised by a purple surface (reading no. 61).
Optical and electron microscopy (PLM, SEM-EDX)
The results of the combination of PLM and SEM-EDX analyses are reported in .
Table 4. PLM and SEM-EDX analysis.
Lead-based pigments. The microstratigraphic analysis of the painted fragment no. 1 () indicates the presence of a continuous and homogeneous dark layer ((a)) composed of Pb ((b)), thus suggesting the darkening of Pb-based pigments, over an orange layer composed of Fe-rich mineral pigment mixed with dolomitic lime. The analysis of one of these grains (corresponding to the larger grain outlined in (a,b)) indicates that it features well the morphology and the microstructure of a phyllosilicate mineral whose chemical composition (not reported here) matches that of a biotite mica. The continuity of this layer with the ground (intonaco) and the absence of an evident interface suggest that the orange layer was applied on a wet plaster (Regazzoni et al. Citation2018). The painted fragment no. 2 () exhibits the presence of residues of glue and gauze ((a)). This can be attributed to the aforementioned stacco and strappo procedures carried out in 1908. Under PLM and SEM-EDX, the sequence shows a yellow layer which fades into an underlying orange-red one, and the presence of Pb over the entire area of investigation ((b–d)). Therefore, the pigment was applied ‘in pasta’ (mixed with silicate sands and dolomitic lime binder; (e–i)), an unusual painting technique for lead-based pigments being generally indicated among those incompatible with fresco technique.
Figure 2. Microstratigraphic analysis of fragment 1. (a) micrograph of the painted sequence under visible incident light; (b) BSE image; (c) BSE image (detail) of the orange grain included in the square outline marked on the previous images.
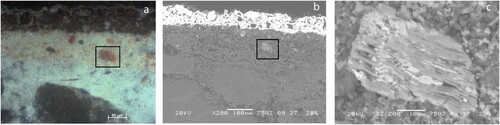
Figure 3. Microstratigraphic analysis of fragment 2. (a) residues of glue and gauzes (portable microscope); (b) micrograph of the painted sequence under visible incident light; (c) BSE image; (d–i) X-ray maps.
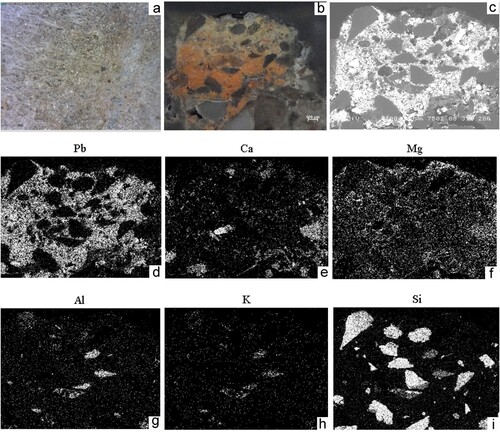
Copper-based pigments. Selected painted plasters (fragments no. 3, 4, 8, 13) – where Cu was detected through HH-XRF as in – were analysed using microscopic techniques. All of the samples indicate the presence of an irregular dark superficial Cu-based layer separated from that below. In particular, the dark Cu-rich layer of fragment no. 3 is in contact with the lime-based ground (intonaco; ), whilst in the fragments no. 4 and 13 ((a)) the Cu-layer is in continuity with a red-purple layer. This red-purple layer features the composition of an organic dye as no chromophore chemical elements were detected (). The Fe counts are too low for justifying the presence of the corresponding mineral pigments. A detailed BSE image ((b)) of the (altered) irregular superficial dark Cu-based layer marked by the presence of bright particles indicates the presence of a grey tone sub-angular grain, approximately 8 µm large, whose chemical composition (results not reported here) suggests the use of (natural) ultramarine (lapis lazuli).
Figure 4. Microstratigraphic analysis of fragment no. 13. (a) micrograph of the painted sequence under visible incident light; (b) BSE image (detail) of the superficial area of the sample.
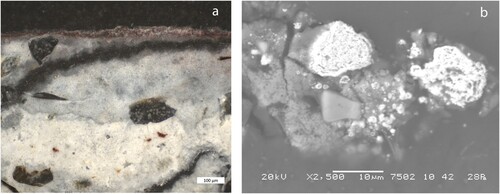
Table 5. Mineralogical analysis (XRPD, µ-Raman, and SERS).
Iron-based pigments. The observation under PLM of sample no. 12 allowed for the detection of a thick orange-brown paint layer ((a)) composed of Fe-bearing pigments (results not reported here) spread within the lime binder ((b)).
X-ray powder diffraction analysis (XRPD)
XRPD analysis () was crucial to determine the mineralogical phases of the painted layers, in order to identify the pigments originally used and/or to highlight any newly formed mineralogical phase due to alteration processes. XRPD was carried out on those samples where darkening of the yellow surface was markedly correlated with the presence of Pb (sample no. 1) and Cu (sample no. 3).
XRPD analysis of fragment no. 1, carried out on the powder taken (mainly) from the black area, identified the minerals plattnerite (β-PbO2), massicot, and cerussite. The presence of calcite, quartz, muscovite, and albite can be associated with the ground layer. Results indicate that the original pigments were not completely transformed into plattnerite suggesting the partial darkening of the fragment. The black compound associated with the darkening of Cu-based pigment is due to the formation of tenorite (CuO). The remaining mineralogical phases (calcite, quartz, orthoclase, muscovite) are associated with the ground layer ().
µ-Raman and SERS
µ-Raman analysis was carried out on the fragments nos. 2 and 4 (), to further determine the mineralogical phases present and identify the nature of the alteration products observed. A Raman spectrum collected on the white-yellow area of the polished cross-section of sample no. 2 ((b)) shows the presence of Raman bands related to massicot (144 cm−1) and white lead (1050 cm−1). The one collected on a red area of the same sample evidences the presence of minium (123, 391, and 549 cm−1) and massicot (144 and 290 cm−1).
Although Raman spectroscopy is optimal in the study of inorganic pigments as it allows obtaining information on chemical compounds and their crystalline structures, it does not allow for a reliable identification of organic dyes. This type of pigment shows a low Raman dispersion and a protracted fluorescence when exposed to the laser beam. Therefore, SERS analysis was carried out on fragment 4, being the most suitable technique for the study of natural dyes in artistic artefacts (Idone et al. Citation2014). The spectrum collected on a red area (not reported here) relates to an organic dye whose precise identification was not accomplished as it does not match with the available databases that were consulted (Bruni, Guglielmi, and Pozzi Citation2011; Pozzi et al. Citation2014; Whitney, Van Duyne, and Casadio Citation2006). The use of high-performance liquid chromatography (HPLC) would be a possible alternative technique for the detection of the nature of the natural dye used (Mills and White Citation1994).
Discussion
Lead-based pigments were only used for the decoration of the painted fragments nos. 1 and 2. The microstratigraphic and mineralogical analysis allowed for the detection of the (original?) pigments used and those which have altered. It is clear that the darkening of the painted surfaces is due to the formation of plattnerite, the mineral phase related to transformations of original Pb-based pigments based on (micro-) environmental exposure. The lead-based pigment was not always uniformly altered into plattnerite (sample 1, ) as it was also found associated with lead white indicating the partial darkening and mineralogical transformation of the original pigment. Lead white and massicot were found in both the samples; massicot is also associated with minium in the red-orange part of the sample. As mentioned earlier, the manufacturing process of these pigments is due to heat treatment. That being said, it is not possible to state if the co-existence of these pigments is intentional or an unintentional consequence of the reaction with the heat generated by the fire. However, the observation of the pigment distribution in sample no. 2 – as highlighted in the micrograph of the cross-section under incident visible light ((b)) – might suggest a non-intentional use, thus supporting the hypothesis of the fire action. Possibly, a lead white pigment was used mixed with a lime binder and subsequently transformed into massicot and minium due to exposure to high temperatures. However, we cannot exclude the intentional use of different Pb-based pigments as the original position of the painted fragment in the wall painting scheme is unknown.
Finally, residues of organic materials (probably glue and gauze) were found on fragment no. 2, presumably used during the removal of wall paintings with the strappo and stacco technique in 1908. This evidence is fundamental as it testifies that until 1900 the fragment was part of the pictorial cycle (Goll, Exner, and Hirsch Citation2007).
Copper-based pigments. The black altered paint layer indicating the presence of Cu was identified on fragments nos. 3, 4, 5, 7, and 9. The darkening is due to the formation of tenorite (CuO) as detected for sample no. 3. This compound, associated with painted surfaces, may be formed as a consequence of the exposure of a Cu-based pigment to high temperatures or due to a reaction to an alkaline environment. In the literature, the use of malachite is mentioned for the Romanesque cycle whilst azurite is not reported (Emmenegger Citation1986; Goll, Exner, and Hirsch Citation2007). According to the analysis of the painted surfaces and of the analytical data reported, the following considerations arise. The reddish-purple layer executed with the use of an organic colourant underneath the Cu-based layer preserves its original integrity and the altered layer composed of tenorite is well separated from the paint layer. In the relevant scientific literature, it is reported that alizarin (from the roots of Rubia tinctorum L.) chars at T = 290°C and sublimes at T = 430°C; otherwise, carminic acid (extracted from various coccid insects) carbonises at lower temperatures, around T = 250°C (Baraldi and Bensi Citation2006).
The geometric separation of the dark and yellow paint decorations in fragments no. 8 and 10 () is very sharp. The dark area is composed of Cu and the yellow one of Fe (), confirming the selective alteration of the pigments used. This behaviour can be explained only considering the instability of the Cu-based pigment used (possibly based on painting technique and/or environmental causes), and the stability of yellow Fe-based pigments. The action of the fire would have led to the conversion of yellow into red. Experimental work carried out on the heat treatment of natural goethite (Cavallo et al. Citation2021) indicates that at T = 250°C the Munsell colour notation changes dramatically from 7.5 YR 6/8 (goethite) to 10R 4/8 (proto-hematite).
Iron-based pigments were used for the decoration of fragments no. 6, 8, 10, 11, 12, and 14 (). The microstratigraphic analysis carried out on samples nos. 8, 11, and 12 () clearly indicate that alteration of the yellow Fe-based pigments did not occur as their yellow-brownish colour is well preserved. Therefore, it is possible to exclude an alteration due to exposure to high temperatures during fires.
Conclusions
The combination of non-invasive and microinvasive analytical techniques shows that the general darkening of Medieval painted fragments at St. John monastery in Müstair cannot be attributed to the action of fires that occurred in the site as reported in the archaeological records but is a consequence of other causes that specifically affect Pb- and Cu-based pigments (light exposure, oxidation processes, alkaline environment of the plaster, and the painting technique used). The microstratigraphic analysis was able to highlight the ways in which darkening is exclusively linked with the paint layers where plattnerite and tenorite occur and did not involve the thermal stability of underlying layers composed of yellow Fe-based pigments and red-purple organic colourant. In addition, the microstratigraphic analysis carried out on yellow Fe-based pigments confirmed that there was not any detectable change of the original colour. Finally, the visual observations of painted fragments (nos. 8, 10) where Cu and Fe were detected respectively on the dark and yellow areas of the same fragment indicate that the contact between the two areas is sharp and no colour changes can be noted in the yellow area, thus excluding possibility of contact with fire for these particular fragments. The co-existence of different Pb-based pigments (lead white, minium, and massicot) in fragment no. 2, on the other hand, leaves open the possibility that this fragment was in contact with fire, possibly suggesting that the effects of fire on the paintings in the attic was not uniform.
The partial (in some cases) alteration of lead white into plattnerite allowed for the recognition of the original pigment used; this was not possible for Cu-based pigments as unaltered grains were not detected.
At St. John monastery, the use of Cu-based pigments is documented for the Romanesque wall paintings, for which the use of malachite is reported. However, the use of a red preparation layer for a green colour as in fragment no. 13 is artistically unusual (thus suggesting the use of azurite?). This consideration might be linked with the evidence found in the analysis of fragment no. 13 where an individual grain of (natural) ultramarine mixed with lime and associated with altered Cu-based pigment (originally green or blue?; (b)) was detected.
The identification of the nature of the red colourant deserves more in-depth analysis also considering the fact that dyes are indicated among the pigments that are not compatible with fresco painting technique because they are not very resistant to light and chemical agents, except for indigo, the only natural dye reported in ancient treatises for wall paintings (Cordaro and Anselmi Citation1978; Schweppe Citation1997; Schweppe and Winter Citation1997). Despite this apparent incompatibility, in Müstair's Carolingian pictorial cycle the presence of madder with protein binder applied on dry plaster has been suggested (Mairinger and Schreiner Citation1986). No natural dyes have hitherto been detected in the Romanesque paintings of Müstair, so this is a new result of this study though its exact characterisation requires additional analytical studies.
Disclosure statement
No potential conflict of interest was reported by the author(s).
References
- Altomare, A., N. Corriero, C. Cuocci, A. Falcicchio, A. Moliterni, and R. Rizzi. 2015. “QualX2.0: A Qualitative Phase Analysis Software Using the Freely Database POW_COD.” Journal of Applied Crystallography 48: 598–603. doi:10.1107/S1600576751002319.
- Aze, S., J. Vallet, M. Pomey, A. Baronnet, and O. Grauby. 2007. “Read Lead Darkening in Wall Paintings: Natural Ageing of Experimental Wall Paintings Versus Artificial Ageing Tests.” European Journal of Mineralogy 19 (6): 882–890.
- Baraldi, P., and P. Bensi. 2006. “Alterazioni delle materie coloranti nelle pitture walli prodotte dalle alte temperature: Fonti storiche ed indagini scientifiche.” In Salvati dalle fiamme. Gli interventi su edifici e oggetti d’ arte danneggiati dal fuoco, edited by G. Jean, 15–29. Atti della giornata di studio. Lugano: SUPSI.
- Bruni, S., V. Guglielmi, and F. Pozzi. 2011. “Historical Organic Dyes: A Surface-enhanced Raman Scattering (SERS) Spectral Database on Ag Lee–Meisel Colloids Aggregated by NaClO4.” Journal of Raman Spectroscopy 42: 1267–1281.
- Calia, A., D. Colangiuli, M. Lettieri, G. Quarta, and M. Masieri. 2016. “Microscopic Techniques and a Multi-analytical Approach to Study the Fire Damage of the Painted Stuccoes from the Petruzzelli Theatre (Bari, Southern Italy).” Microchemical Journal 126: 42–53.
- Cavallo, G., M. Aceto, R. Emmenegger, A. T. Keller, R. Lenz, L. Villa, S. Wörz, and P. Cassitti. 2020. “Preliminary Non-invasive Study of Carolingian Pigments in the Churches of St. John at Müstair and St. Benedict at Malles.” Archaeological and Anthropological Sciences. doi:10.1007/s12520-020-01024-2.
- Cavallo, G., R. Cardani Vergani, L. Gianola, and A. Meregalli. 2012. “Archaeological, Stylistic and Scientific Research on 11th-13th Century A.D Painted Fragments from the San Giovanni Battista Church in Cevio (Switzerland).” Archaeometry 54 (2): 294–310.
- Cavallo, G., F. Fontana, S. Gialanella, F. Gonzato, M. P. Riccardi, R. Zorzin, and M. Peresani. 2018. “Heat Treatment of Mineral Pigment During the Upper Paleolithic in North-East Italy.” Archaeometry 60: 1045–1061.
- Cavallo, G., G. Ischia, R. Zorzin, and S. Gialanella. 2021. “Experimental Analysis on Natural Heated Goethite from Ponte di Veja (Mt Lessini, NE Italy).” Journal of Archaeological Science Reports 36: 102871.
- Coccato, A., L. Moens, and P. Vandenabeele. 2017. “On the Stability of Mediaeval Inorganic Pigments: A Literature Review of the Effect of Climate, Material Selection, Biological Activity, Analysis and Conservation Treatments.” Heritage Science 5 (12): 1–25.
- Cordaro, M., and E. Anselmi. 1978. “Tecniche di esecuzione e materiali costitutivi.” In DIMOS, corso sulla manutenzione di dipinti murali, mosaici e stucchi, parte I modulo I. Roma: Ed. ICR7–42.
- Emmenegger, O. 1986. “Deterioration and Preservation of Carolingian and Mediaeval Mural Paintings in the Müstair Convent (Switzerland), Part III: Techniques and Materials Used and Past Restorations.” In: Contributions to the Bologna Congress 21-26 September 1986, Case Studies in the Conservation of Wall Paintings, preprints, 197–199.
- Emmenegger, O. 2002. “Karolingische und romanische Wandmalerei in der Klosterkirche. Technik, Restaurierungsprobleme, Massnahmen. Die mittelalterlichen Wandmalereien im Kloster Müstair. Grundlagen zu Konservierung und Pflege, edited by A. Wyss, H. Rutishauser, and M. A. Nay, 77–139. vdf Hochschulverlag, Zürich.
- Flühler-Kreis, D. 2002. “Karolingische Wandgemälde aus der Klosterkirche im Schweizerischen Landesmuseum Zürich.” In: Die mittelalterlichen Wandmalereien im Kloster Müstair. Grundlagen zu Konservierung und Pflege, edited by A. Wyss, H. Rutishauser, and M. A. Nay, 63–75. vdf Hochschulverlag, Zürich.
- Frost, R. L., Z. Ding, J. T. Kloprogge, and W. N. Martens. 2002. “Thermal Stability of Azurite and Malachite in Relation to the Formation of Mediaeval Glass and Glazes.” Thermochimica Acta 390: 133–144.
- Goll, J., M. Exner, and S. Hirsch. 2007. Müstair: Le pitture parietali Medievali nella chiesa dell'abbazia, Patrimonio mondiale dell'UNESCO. Zurigo: Neue Zürcher Zeitung.
- Grissom, C. A. 1986. “Green Earth.” In Artists’ Pigments, A Handbook of Their History and Characteristics, edited by R. L. Feller, vol. 1, 41–165. Washington: National Gallery of Art.
- Gualtieri, F., and P. Venturelli. 1999. “In Situ Study of the Goethite-Hematite Phase Transformation by Real Time Synchrotron Powder Diffraction.” American Mineralogist 84: 895–904.
- Gutscher, D., B. Mühlethaler, A. Portmann, and A. Reller. 1989. “Conversion of Azurite Into Tenorite.” Studies in Conservation 34: 117–122.
- Hurni, J. P., C. Orcel, and J. Tercier. 2007. “Zu den dendrochronologischen Untersuchungen von Hölzern aus St. Johann in Müstair.” In: Müstair Kloster St. Johann 4. Naturwissenschaftliche und technische Beiträge, edited by H. R. Sennhauser, 99–116. vdf Hochschulverlag, Zürich.
- Idone, A., M. Aceto, E. Diana, L. Appolonia, and M. Gulmini. 2014. “Surface-enhanced Raman Scattering for the Analysis of Red Lake Pigments in Painting Layers Mounted in Cross Section.” Journal of Raman Spectroscopy 45. doi: 10.1002/jrs.4491.
- Leandri, C. 2018. “I frammenti di intonaco dipinto romanico del monastero di Müstair (GR).” Studio dei materiali e della tecnica esecutiva. Unpublished Bachelor thesis, Scuola Universitaria Professionale della Svizzera Italiana.
- Lee, P. C., and D. Meisel. 1982. “Adsorption and Surface-enhanced Raman of Dyes on Silver and Gold Sols.” The Journal of Physical Chemistry 86 (17): 3391–3395.
- Mairinger, F., and M. Schreiner. 1986. “Deterioration and Preservation of Carolingian and Medieval Mural Paintings in the Müstair Convent (Switzerland), Part II: Materials and Rendering of the Carolingian Wall Paintings.” In: Contributions to the Bologna Congress 21-26 September 1986, Case studies in the Conservation of Wall Paintings, Preprints, 195–196.
- Mattei, E., G. De Vivo, A. De Santis, C. Gaetani, C. Pelosi, and U. Santamaria. 2008. “Raman Spectroscopic Analysis of Azurite Blackening.” Journal of Raman Spectroscopy 39: 302–306.
- Mills, J. S., and R. White. 1994. The Organic Chemistry of Museum Objects (2nd ed., 1–206). Oxford: Butterworth Heinemann.
- Pozzi, F., K. J. van den Berg, I. Fiedlera, and F. Casadio. 2014. “A Systematic Analysis of Red Lake Pigments in French Impressionist and Post-impressionist Paintings by Surface-Enhanced Raman Spectroscopy (SERS).” Journal of Raman Spectroscopy 45: 1119–1126.
- Regazzoni, L., G. Cavallo, D. Biondelli, and J. Gilardi. 2018. “Microscopic Analysis of Wall Painting Techniques: Laboratory Replicas and Romanesque Case Studies in Southern Switzerland.” Studies in Conservation 63 (6): 326–341.
- Rutherford, J. Gettens, H. Kuhn, and W. T. Chase. 1993a. “Lead White.” In Artists’ Pigments, A Handbook of Their History and Characteristics, edited by A. Roy, vol. 2, 67–82. Washington: National Gallery of Art.
- Rutherford, J. Gettens, and E. West Fitzhugh. 1993b. “Azurite and Blue Verditer.” In Artists’ Pigments, A Handbook of Their History and Characteristics, edited by Ashok Roy, vol. 2, 23–36. Washington: National Gallery of Art.
- Schweppe, H. 1997. “Indigo and Woad.” In Artists’ Pigments, A Handbook of Their History and Characteristics, edited by E. W, Fitzhugh, vol. 381–108. Washington: National Gallery of Art.
- Schweppe, H., and J. Winter. 1997. “Madder and Alizarin.” In Artists’ Pigments, A Handbook of Their History and Characteristics, edited by Elisabeth West Fitzhugh, vol. 3, 109–142. Washington: National Gallery of Art.
- Vagninia, M., R. Vivani, E. Viscuso, M. Favazza, B. G. Brunetti, A. Sgamellotti, and C. Miliani. 2018. “Investigation on the Process of Lead White Blackening by Raman Spectroscopy, XRD and Other Methods: Study of Cimabue’s Paintings in Assisi.” Vibrational Spectroscopy 98: 41–49.
- Whitney, A. V., R. P. Van Duyne, and F. Casadio. 2006. “An Innovative Surface-enhanced Raman Spectroscopy (SERS) Method for the Identification of Six Historical Red Lakes and Dyestuffs.” Journal of Raman Spectroscopy 37: 993–1002.
- Wüthrich, L. 1980. Wandgemälde von Müstair bis Hodler. Katalog der Sammlung des Schwezerischen Landesmuseums Zürich, 17–41.Zürich: Verlag Berichtshaus.