Abstract
A series of N-benzhydryl protected α-aminophosphonates with α-phenyl, α-(1-naphtyl), α-(9-anthryl) or α-(1-pyrenyl) substituents was synthesized by the Kabachnik–Fields condensation of diphenylmethylamine (benzhydrylamine), the corresponding aryl aldehyde and a dialkyl phosphite under MW irradiation. X-ray studies performed at low temperatures for a few of these α-aminophosphonates confirmed the presence of unusually short intramolecular Cα–Hδ+ ··· δ+H–Cperi contacts.
Graphical Abstract
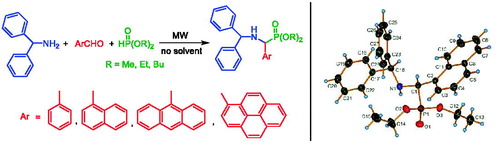
Introduction
α-Aryl-α-aminophosphonates are the P-analogues of α-aryl-α-amino acids. They play an important role as enzyme inhibitors, antibiotics, antiviral or anticancer agents.[Citation1-4] The synthesis of α-aryl-α-aminophosphonates can be carried out in two main routes: by the Kabachnik–Fields condensation,[Citation5-9] or by the Pudovik (aza-Pudovik) reaction.[Citation10-13] Although, the Pudovik addition, where a >P(O)H reagent, such as a dialkyl phosphite is added to the C = N double-bond of imines, is simpler and widely applied, the Kabachnik–Fields condensation, in which an amine, an aldehyde or ketone and a >P(O)H species react in a one-pot manner, is also of an increased interest. Both reaction types were performed in the presence of a catalyst and/or a solvent.[Citation14] Nowadays, greener accomplishments have come to the front, from which the catalyst- and solvent-free microwave (MW)-assisted variations are to be mentioned.[Citation14-18]
The benzhydryl group may be utilized as an N-protecting group in organic and peptide chemistry.[Citation19-21] The easy introduction and elimination of this moiety, as well as the stability and the good crystallization properties of the products make this unit useful for preparative chemists. Up to now, only three cases were reported for the Kabachnik–Fields-type syntheses of α-aryl-α-aminophosphonates containing an N-benzhydryl group.[Citation22-24] In one case the condensation of benzhydrylamine, 1-naphthaldehyde and diethyl phosphite was carried out in the presence of montmorillonite KSF catalyst in a kitchen MW oven.[Citation22] A scandium tris(dodecylsulfate)-catalyzed synthesis of α-aryl-α-aminophosphonates was also described, where triethyl phosphite was applied as the P-component.[Citation23] Benzhydrylamine was also reacted with formylbenzoic acid and dimethyl phosphite, where 3-oxoisoindolin-1-ylphosphonate was synthesized in a moderate yield.[Citation24] In most cases, these derivatives were synthesized by the Pudovik reaction of α-aryl imines with dialkyl phosphites,[Citation25,Citation26] also providing new information on the peculiar proximity H atoms.[Citation26]
In this paper, the synthesis of α-aryl-α-aminophosphonates by the solvent- and catalyst-free Kabachnik–Fields condensation of benzhydrylamine, aromatic aldehydes and dialkyl phosphites was revisited under MW irradiation. The investigation involved the preparation of the dialkyl α-aryl-α-(diphenylmethylamino)methanephosphonates, and the determination of the X-ray structures of a few of these products at low temperatures.
Results and discussion
Kabachnik–Fields reaction of benzhydrylamine, benzaldehyde and dialkyl phosphites
The title phospha-Mannich condensation starting from benzhydrylamine, benzaldehyde and dialkyl phosphites was performed under solvent-free microwave (MW)-assisted conditions (Scheme 1). Using 1 equivalent of dimethyl phosphite (DMP), diethyl phosphite (DEP) or dibutyl phosphite (DBP) at 100 °C for a reaction time of 1 h, conversions of 87%, 89% and 63%, respectively, were obtained. In these cases, the imine intermediate 2 could also be detected in the reaction mixtures. Complete conversion required a 1.1 equivalents quantity of the dialkyl phosphites. After an irradiation of 1 h at 100 °C, the methyl and ethyl aminophosphonates (1a and 1b) were obtained in yields of 88% and 90%, respectively. Efficient synthesis (91% yield) of the butyl product (1c) required a reaction temperature of 120 °C.
The products (1a–c) obtained after column chromatography were characterized by 31P, 13C and 1H NMR spectral data.
Kabachnik–Fields reaction of benzhydrylamine, 1-naphthaldehyde and dialkyl phosphites
A similar series of the phospha-Mannich reaction was carried out applying 1-naphtylaldehyde as the oxo component (Scheme 2). The situation was somewhat more complicated as in the previous case discussed above. With this sterically more hindered aryl aldehyde, complete conversions required somewhat more forcing conditions. At the same time, beside imine 3, the other possible intermediate, hydroxyphosphonate 5 could be pointed out from the mixture by 31P NMR and LC-MS. Moreover, the corresponding monoester aminophosphonic derivative 6 was also present in the mixtures. Experimental data are listed in . Using 1 equivalent of DMP at 100 °C/1 h, the proportion of components 3, 4, 5 and 6 was 15:67:15:3 (, entry 1). Increasing the quantity of DMP to 1.2 equivalents, not much change could be observed (, entry 2). However, the application of 1.5 equivalents of DMP led to a proportion of 82% of the desired aminophosphonate 4a (, entry 3). Repeating the experiment marked by entry 1 of at 120 °C, the proportion of product 4 was almost the same, but no imine 3 was present, while the relative quantity of the monoester by-product (6) increased (, entry 4). Preparation of the ethyl derivative (4b) was more appropriate at 120 °C (, entry 5 vs. entry 6). An increase in the quantity of DEP to 1.5 equivalents was useful, as again a relative proportion of 90% of product 4b could be attained, but without imine 3 (, entry 8). Butyl aminophosphonate 4c was also synthesized best at 120 °C for 1 h using 1.5 equivalents of DBP (, entry 11). α-Aminophosphonates 4a, 4b and 4c were obtained in 80%, 84% and 90% yields, respectively. Structure of the products was characterized as above. Identification data of the imines (HRMS), as well as the α-hydroxyphosphonates and the α-aminophosphonate monoesters (31P NMR and HRMS) are listed in Tables S1, S2 and S3, respectively.
Table 1. The condensation of benzhydrylamine, 1-naphthaldehyde and dialkyl phosphites.
Kabachnik–Fields reaction of benzhydrylamine, 9-anthraldehyde and dialkyl phosphites
9-Anthraldehyde proved to be a somewhat more hindered model in Kabachnik–Fields reaction with benzhydrylamine and dialkyl phosphites, as only lower proportions of the corresponding α-aminophosphonates (8a–c) could be obtained. The same type of by-products, α-hydroxyphosphonate 9, and monoester 10 could be found in the mixtures (Scheme 3 and ). As can be seen from the data, the conditions explored in the previous sets of experiments were proved to be the most suitable also for the preparation of the anthryl-substituted aminophosphonates 8a–c. The methyl-, ethyl- and butyl esters could be prepared in yields of 73%, 78% and 82%, respectively (, entries 3, 7 and 11, respectively).
Table 2. The condensation of benzhydrylamine, 9-anthraldehyde and dialkyl phosphites.
Table 3. The condensation of benzhydrylamine, 1-pyrenecarbaldehyde and dialkyl phosphites.
Kabachnik–Fields reaction of benzhydrylamine, 1-pyrenecarbaldehyde and dialkyl phosphites
The phospha-Mannich condensations of 1-pyrenecarbaldehyde, benzhydrylamine and dialkyl phosphites were performed at the conditions found suitable in the previous experiments suggesting 1.5 equivalents of the dialkyl phosphite and 100–120 °C (Scheme 4, ). In these cases, no unreacted imine remained in the mixture, and the corresponding α-aminophosphonates 11a–c were obtained in yields of 68%, 80% and 88%, respectively (, entries 1–3).
Scheme 4. Kabachnik–Fields reaction of benzhydrylamine, 1-pyrenecarbaldehyde and dialkyl phosphites.
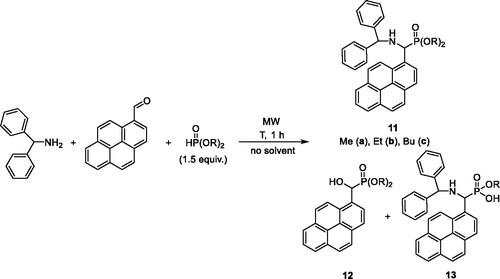
A few of the reactions were also carried out on conventional heating. Based on our results, the MW-assisted variations reached somewhat (by <10%) higher conversions as compared to the control experiments (see footnote “c” of ).
Altogether 12 α-aminophosphonates (1a–c, 4a–c, 8a–c and 11a–c) were prepared and characterized by 31P, 13C and 1H NMR spectroscopy, as well as by HRMS data. Apart from the four ethyl esters (1b, 4b, 8b and 11b), all derivatives are new.
X-ray diffraction study of the ethyl α-aryl-α-aminophosphonates
Results of the redetermination of the crystal structures of three ethyl α-aminophosphonates 4b, 8b and 11b at low temperatures (c.f. Table S4) can be summarized as leading to the same dimensions and conclusions as from those from the earlier, mostly room-temperature structure determinations.[Citation26] Neither phase transitions nor any relevant changes occur in the 173–295 K temperature range for 4b, 8b and 11b. As a measure of the similarity of these three pairs of independent structures at two sets of temperatures (295 K and 173 K) for 4b, 8b and 11b is provided by a least-square fit[Citation27] of the Caryl–Cα –N moiety. The results of the fitting can be summarized by the r.ms. deviations for the respective models. The values are 0.002 Å for 4b, 0.005 Å for 8b, and 0.004 Å for 11b (c.f. Figs. S1, S2 and S3, respectively). These indicate that the low temperature structures are practically the same as reported.[Citation26] This applies for the basic packing motifs (c.f. Table S5), too. α-Aminophosphonates 4b and 11b form N − H ··· O = P bridged dimers, while structure 8b shows a C − H ··· O = P contact that links molecules into chains (c.f. Figs. S4, S5 and S6).
As far as the important H atom positions are concerned, these are intriguing either from the point of view of C − Hδ+ ··· δ+H − C bonding, or due to the hybridization state of the N atom. In a neutral molecule, the latter depends on the nature of the distal substituent atom vis à vis the Cα atom. As a result e.g. in 8b the symmetric peri-position H atom distances become similarly short: the Cα−H···H − Cperi is dH(1)···C−Hδ+···δ+H−C···H(4) = 1.80(2) Å, while the N − H···H − Cperi2 is dH(14)···C−Hδ+···δ+H−C···H(10) = 2.00(2) Å.
The history of short C–H ··· H–C contacts begun with experimental proofs. Extreme short (1.754(4) Å and 1.713(3) Å) non-bonded H ··· H distances were reported by Ermer and Mason.[Citation28] An even shorter (1.617(3) Å) such non-bonded distance was found using neutron diffraction, as well.[Citation29] Theoretic and experimental results suggested that such short distances may well be resulting from attractive C − Hδ+···δ+H − C type interactions.[Citation30,Citation31] The theory was increasingly used to explain whether the experimentally observed distances are of attractive, or of repulsive nature.[Citation30,Citation32-36] The H–H bonding was confirmed in nitroimidazole derivatives[Citation33] and in biphenyl.[Citation36] A DFT, ab initio, QTAIM and ELF study concluded that stability of a few branched alkanes is due to H – H bonds,[Citation37] however, atom–atom short contacts still remain a field of ongoing discussions.[Citation38]
Conclusions
The MW-assisted, catalyst- and solvent-free Kabachik–Fields reaction afforded the α-aminophosphonates bearing sterically demanding aryl groups efficiently in a one-pot manner. The products obtained were characterized by 31P, 13C and 1H NMR spectroscopy, as well as by HRMS data. Eight of the 12 α-aminophosphonate derivatives are new.
Redetermination of some of the crystal structures at 173 K temperatures verifies results and conclusions based on earlier data. Thus, we conclude that no phase change nor any structural change occur in the 295–173 K temperature range in these crystals. The very short intramolecular C − Hδ+ ··· δ+H − C contacts persist and their quality, as inferred from other studies too, is of cohesive nature. They are contributing to stabilization of the shape, conformation and dimensions of these molecules in their crystals.
Experimental section
General procedure for the synthesis of α-aminophosphonates
The mixture of 0.17 mL (1.00 mmol) of benzhydrylamine, 1.0 mmol of aldehyde (benzaldehyde: 0.10 mL, 1-naphtaldehyde: 0.14 mL, 9-anthracenecarboxaldehyde: 0.20 g or 1-pyrenecarboxaldehyde: 0.23 g) and 1.0/1.2/1.5 mmol of dialkyl phosphite (dimethyl phosphite 0.092/0.11/0.14 mL, diethyl phosphite: 0.13/0.16/0.19 mL, dibutyl phosphite: 0.20/0.23/0.29 mL) was irradiated in a sealed tube at 100–120 °C for 60–75 min in a CEM Discover Microwave reactor equipped with a pressure controller. The crude product was purified by flash column chromatography using silica gel, and dichloromethane-methanol 97:3 as the eluent. See the Supplementary Information for the characterization data of products 1a–c, 4a–c, 8a–c and 11a–c.
Supplemental Material
Download MS Word (2.1 MB)Additional information
Funding
References
- Kukhar, V. P.; Hudson, H. R. Aminophosphonic and Aminophosphinic Acids: Chemistry and Biological Activity, Wiley, Chichester, 2000. ISBN:0-471-89149-5.
- Mucha, A.; Kafarski, P.; Berlicki, Ł. Remarkable Potential of the α-Aminophosphonate/Phosphinate Structural Motif in Medicinal Chemistry. J. Med. Chem. 2011, 54, 5955–5980. DOI: 10.1021/jm200587f.
- Hudson, H. R.; Lee, R. J. A Brief Review of the Anticancer Activity of α-Aminophosphonic Acid Derivatives and a Report on the in Vitro Activity of Some Dialkyl α-Aryl-(or Heteroaryl)-α-(Diphenylmethylamino)Methanephosphonates. Phosphorus, Sulfur Silicon Relat. Elem. 2014, 189, 1149–1155. DOI: 10.1080/10426507.2014.905781.
- Tajti, Á.; Keglevich, G. The Importance of Organophosphorus Compounds as Biologically Active Agents. In Organophosphorus Chemistry, Keglevich, G. Ed.; de Gruyter, Berlin, 2018; pp 53–65.
- Kabachnik, M. I.; Medved, T. Y. New Synthesis of Aminophosphonic Acids. Dokl. Akad. Nauk SSSR 1952, 83, 689–692.
- Fields, E. K. The Synthesis of Esters of Substituted Amino Phosphonic Acids. J. Am. Chem. Soc. 1952, 74, 1528–1531. DOI: 10.1021/ja01126a054.
- Cherkasov, R. A.; Galkin, V. I. The Kabachnik–Fields Reaction: synthetic Potential and the Problem of the Mechanism. Russ. Chem. Rev. 1998, 67, 857–882. DOI: 10.1070/RC1998v067n10ABEH000421.
- Zefirov, N. S.; Matveeva, E. D. Catalytic Kabachnik-Fields Reaction: New Horizons for Old Reaction. Arkivoc 2008, 1–17. DOI: 10.3998/ark.5550190.0009.101.
- Keglevich, G.; Bálint, E. The Kabachnik–Fields Reaction: Mechanism and Synthetic Use. Molecules 2012, 17, 12821–12835. DOI: 10.3390/molecules171112821.
- Pudovik, A. N. Addition of Dialkyl Phosphites to Unsaturated Compounds. A New Method of Synthesis of β-Keto Phosphonic and Unsaturated α-Hydroxyphosphonic Esters. Dokl. Akad. Nauk SSSR 1950, 73, 499–502.
- Pudovik, A. N. Addition of Dialkyl Phosphites to Imines. New Method of Synthesis of Esters of Amino Phosphonic Acids. Dokl. Akad. Nauk SSSR 1952, 83, 865–868.
- Ali, T. E.; Abdel-Kariem, S. M. Methods for the Synthesis of α-Heterocyclic/Heteroaryl-α-Aminophosphonic Acids and Their Esters. Arkivoc 2015, 246–287. DOI: 10.3998/ark.5550190.p009.112.
- Ali, T. E.; Abdel-Kariem, S. M. Methods for Synthesis of N-Heterocyclyl/Heteroaryl-α-Aminophosphonates and α-(Azaheterocyclyl)Phosphonates. Arkivoc 2016, 183–211. DOI: 10.3998/ark.5550190.p009.519.
- Bálint, E.; Tajti, Á.; Tripolszky, A. Synthesis of α-Aminophosphonates by the Kabachnik–Fields reaction and by the Pudovik reaction. In Organophosphorus Chemistry, Keglevich, G., Ed.; de Gruyter: Berlin, 2018; pp 108–147. DOI: 10.1515/9783110535839-006.
- Kafarski, P.; Górniak, M. G.; Andrasiak, I. Kabachnik-Fields Reaction under Green Conditions—A Critical Overview. Curr. Green Chem. 2015, 5, 218–222. DOI: 10.2174/2213346102666150109203606.
- Keglevich, G.; Szekrényi, A. Eco-Friendly Accomplishment of the Extended Kabachnik–Fields Reaction; a Solvent- and Catalyst-Free Microwave-Assisted Synthesis of α-Aminophosphonates and α-Aminophosphine Oxides. Lett. Org. Chem. 2008, 5, 616–622. DOI: 10.2174/157017808786857598.
- Tibhe, G. D.; Reyes-Gonzales, M. A.; Cativiela, C.; Ordóñez, M. Microwave-Assisted High Diastereoselective Synthesis of α-Aminophosphonates under Solvent and Catalyst Free-Conditions. J. Mex. Chem. Soc. 2012, 56, 183–187. DOI: 10.29356/jmcs.v56i2.319.
- Bálint, E.; Tajti, Á.; Ádám, A.; Csontos, I.; Karaghiosoff, K.; Czugler, M.; Ábrányi-Balogh, P.; Keglevich, G. The Synthesis of α-Aryl-α-Aminophosphonates and α-Aryl-α-Aminophosphine Oxides by the Microwave-Assisted Pudovik Reaction. Beilstein J. Org. Chem. 2017, 13, 76–86. DOI: 10.3762/bjoc.13.10.
- McOmie, J. F. W. Protective Groups in Organic Chemistry; Plenum Press: London, 1973. DOI: 10.1007/978-1-4684-7218-9.
- Takahashi, D.; Yano, T.; Fukui, T. Novel diphenylmethyl-Derived Amide Protecting Group for Efficient Liquid-Phase Peptide Synthesis: AJIPHASE. Org. Lett. 2012, 14, 4514–4517. DOI: 10.1021/ol302002g.
- Saudi, M.; van Aerschot, A. A Straightforward Diphenylmethyl Protection Method and Deprotection of Some Pyrimidine Nucleosides. Molecules 2013, 18, 8524–8534. DOI: 10.3390/molecules18078524.
- Yadav, J. S.; Reddy, B. V. S.; Madan, C. Montmorillonite Clay-Catalyzed One-Pot Synthesis of α-Amino Phosphonates. Synlett 2001, 2001, 1131–1133. DOI: 10.1055/s-2001-15162.
- Manabe, K.; Kobayashi, S. Facile Synthesis of α-Amino Phosphonates in Water Using a Lewis Acid–Surfactant-Combined Catalyst. Chem. Commun. 2000, 669–670. DOI: 10.1039/b000319k.
- Ordonez, M.; Tibhe, G. D.; Zamudio-Medina, A.; Viveros-Ceballos, J. L. An Easy Approach for the Synthesis of N-Substituted Isoindolin-1-Ones. Synthesis 2012, 44, 569–574. DOI: 10.1055/s-0031-1289680.
- Hudson, H. R.; Lee, R. J.; Matthews, R. W. 1-Amino-1-Aryl- and 1-Amino-1-Heteroaryl-Methanephosphonic Acids and Their N-Benzhydryl-Protected Diethyl Esters: Preparation and Characterization. Phosphorus, Sulfur Silicon Relat. Elem 2004, 179, 1691–1709. DOI: 10.1080/10426500490466274.
- Hudson, H. R.; Czugler, M.; Lee, R. J.; Woodroffe, T. M. Extremely Short H···H Distances and Intermolecular Hydrogen-Bonding Patterns of Dialkyl α-Aryl-α-(Diphenylmethylamino)Methanephosphonates. Phosphorus, Sulfur Silicon Relat. Elem. 2016, 191, 469–477. DOI: 10.1080/10426507.2015.1091833.
- Macrae, C. F.; Bruno, I. J.; Chisholm, J. A.; Edgington, P. R.; McCabe, P.; Pidcock, E.; Rodriguez-Monge, L.; Taylor, R.; van de Streek, J.; Wood, P. A. Mercury CSD 2.0– New Features for the Visualization and Investigation of Crystal Structures. J. Appl. Crystallogr. 2008, 41, 466–470. DOI: 10.1107/S0021889807067908.
- Ermer, O.; Mason, S. A. Extremely Short Non-Bonded H … H Distance in Two Derivatives of Exo, Exo-Tetracyclo[6.2.1.13,6.02,7]Dodecane. J. Chem. Soc. Chem. Commun. 1983, 53–54. DOI: 10.1039/c39830000053.
- Ermer, O.; Mason, S. A.; Anet, F. A. L.; Miura, S. S. Ultrashort Nonbonded Hydrogen…Hydrogen Distance in a Half-Cage Pentacyclododecane. J. Am. Chem. Soc. 1985, 107, 2330–2334. DOI: 10.1021/ja00294a023.
- Matta, C. F.; Hernandez-Trujillo, J.; Tang, T.-H.; Bader, R. F. W. Hydrogen–Hydrogen Bonding: A Stabilizing Interaction in Molecules and Crystals. Chem. Eur. J. 2003, 9, 1940–1951. DOI: 10.1002/chem.200204626.
- Bodige, S. G.; Sun, D.; Marchand, A. P.; Namboothiri, N. N.; Shukla, R.; Watson, W. H. Short H· · ·H Distances in Norbornene Derivatives. J. Chem. Crystallogr. 1999, 29, 523–530. DOI: 10.1023/A:1009584416307.
- Wolstenholme, D. J.; Cameron, T. S. Comparative Study of Weak Interactions in Molecular Crystals: H − H Bonds vs Hydrogen Bonds. J. Phys. Chem. A 2006, 110, 8970–8978. DOI: 10.1021/jp061205i.
- Paul, A.; Kubicki, M.; Jelsch, C.; Durand, P.; Lecomte, C. R-Free Factor and Experimental Charge-Density Analysis of 1-(2'-Aminophenyl)-2-Methyl-4-Nitroimidazole: A Crystal Structure with Z' = 2. Acta Crystallogr. B Struct. Sci. 2011, 67, 365–378. DOI: 10.1107/S0108768111022683.
- Grabowski, S. J.; Pfitzner, A.; Zabel, M.; Dubis, A. T.; Palusiak, M. Intramolecular H···H Interactions for the Crystal Structures of [4-((E)-but-1-Enyl)-2,6-Dimethoxyphenyl]Pyridine-3-Carboxylate and [4-((E)-Pent-1-Enyl)-2,6-Dimethoxyphenyl]Pyridine-3-Carboxylate; DFT Calculations on Modeled Styrene Derivatives. J. Phys. Chem. B 2004, 108, 1831–1837. DOI: 10.1021/jp0351829.
- Matta, C. F. Hydrogen–hydrogen bonding: the non-electrostatic limit of closed-shell interaction between two hydrogen atoms. A critical review. In Hydrogen Bonding—New Insight, (Challenges and Advances in Computational Chemistry and Physics Series), Grabowski, S., Ed.; Dordrecht: Springer, 2006; pp 337–375. DOI: 10.1007/978-1-4020-4853-1_9.
- Hernández-Trujillo, J.; Matta, C. F. Hydrogen–Hydrogen Bonding in Biphenyl Revisited. Struct. Chem. 2007, 18, 849–857. DOI: 10.1007/s11224-007-9231-5.
- Monteiro, N. K.; Firme, C. L. Hydrogen–Hydrogen Bonds in Highly Branched Alkanes and in Alkane Complexes: A DFT, ab Initio, QTAIM, and ELF Study. J. Phys. Chem. A 2014, 118, 1730–1740. DOI: 10.1021/jp500131z.
- Lecomte, C.; Espinosa, E.; Matta, C. F. On Atom–Atom `Short Contact' Bonding Interactions in Crystals. IUCrJ 2015, 2, 161–163. DOI: 10.1107/S2052252515002067.