Abstract
AIM: To study the association of polymorphisms at five micro-satellite loci with immune responses to a killed Mycobacterium avium subsp. paratuberculosis (Map) vaccine.
METHODS: Merino sheep (504 vaccinates and 430 unvaccinated controls) from a long-term Johne's vaccine trial undertaken on three different properties in the Central Tablelands of New South Wales, Australia, were genotyped for five micro-satellite markers located in three immunologically significant chromosome regions. The marker loci included three from the major histocompatibility complex (MHC), namely DYMS1, OLADRB and SMHCC1; and one each from the solute carrier family 11 member 1 (SLC11A1), OVINRA1, and the interferon-γ (IFN-γ), o(IFN)-γ, gene regions. Associations between immune responses and genetic polymorphisms at the marker loci were examined by analysing both allelic and genotypic effects.
RESULTS: The o(IFN)-γ locus had only two alleles, whereas the other four loci exhibited extensive polymorphism, with the number of alleles ranging from 10 (OVINRA1) to 21 (DYMS1), resulting in 30–92 genotypes per locus. Heterozygosities varied between 37% (o(IFN)-γ) and 87% (SMHCC1), while information on polymorphic contents ranged from 0.31 (o(IFN)-γ) to 0.87 (DYMS1). Each of the three properties exhibited unique allelic and genotypic frequencies. Analysis of immune response data revealed strong antibody and IFN-γ responses as early as 2 months post-vaccination. Immune responses in control animals on all three properties remained consistently low, except for slightly elevated IFN-γ responses at a few time-points on two properties, concomitant with exposure to natural infection. Genotype-phenotype association analyses revealed a number of marker geno types/alleles to be significantly associated with antibody and IFN-γ responses. However, the effects of only five genotypes (one each at DYMS1, OLADRB, SMHCC1, OVINRA1 and o(IFN)-γ) and three alleles (one each at o(IFN)-γ, DYMS1 and OLADRB) on IFN-γ responses were consistent across the three properties.
CONCLUSION: Considering the significance of IFN-γ responses in protection against Map, it is possible that the genotypes/alleles identified might have a role in protective immune responses to natural Map infections, and further studies are warranted to confirm this.
bp | = |
Base pair(s) |
CMI | = |
Cell-mediated immune/immunity |
IFN-γ | = |
Interferon-γ |
Map | = |
Mycobacterium avium subsp. paratuberculosis |
MHC | = |
Major histocompatibility complex |
NRAMP1 | = |
Natural resistance-associated macrophage protein 1 |
OD450 | = |
Optical density at 450 nm |
PBS | = |
Phosphate buffered saline |
PPD | = |
Purified protein derivative(s) |
PTB | = |
Paratuberculosis |
SLC11A1 | = |
Solute carrier family 11 member 1 |
Introduction
Johne's disease, or paratuberculosis (PTB), is a chronic, progressive intestinal disease of ruminants, caused by Mycobacterium avium subsp. paratuberculosis (Map). PTB does not result in high rates of mortality, however it results in significant economic losses primarily attributed to decreased production (milk, bodyweight and wool) and culling. A study in Minnesota, United States of America, found that cows that were light, moderate and heavy faecal shedders of Map produced on average 537, 1,403 and 1,534 kg, respectively, less milk per lactation than cows that did not shed Map (Raizman et al. Citation2007). Reductions up to 15% and 31% in slaughter weight and value, respectively, were reported in dairy cows in Denmark that tested positive for serum antibodies and Map faecal cultures compared with un-infected cows (Kudahl and Nielsen Citation2009). In Australia, the mean annual decrease in gross margin due to PTB over 12 infected sheep flocks was found to vary between 6.4% and 8.5% over a 3-year period, equating to an average reduction in annual income of A$13,715 per farm per year (Bush et al. Citation2006).
Due to the prolonged subclinical nature of the disease, PTB is not usually diagnosed until the onset of the clinical phase, and treatment during this phase is ineffective and expensive. Further, there are no approved drugs to treat PTB in livestock (Harris and Barletta Citation2001). Vaccination against PTB can play an important role in the control of clinical disease, but cannot prevent animals from becoming infected (Gwozdz et al. Citation2000). One possible way of limiting the incidence of the disease is to identify genetic markers for resistance/susceptibility to the disease. Such markers could be employed to cull susceptible animals and to selectively breed resistant animals. Low (0.01) to moderate (0.18) estimates of heritability were previously reported for susceptibility to Map in cattle (Koets et al. Citation2000), goats (Singh et al. Citation1990) and sheep (Hickey et al. Citation2003).
A long-term trial designed to evaluate the efficacy of a whole killed Map vaccine, Gudair (CZ Veterinaria, Porrino, Spain), for the control of PTB in Merino sheep, was carried out in New South Wales, Australia, from 1999 to 2004 (Reddacliff et al. Citation2006). In total, 600 sheep from three different properties were vaccinated, while another 600 constituted the unvaccinated control group. Antibody and IFN-γ responses to vaccination, recorded at various intervals for up to 54 months post-vaccination, revealed variations between individuals in response to the vaccine. These variations offered the opportunity to search for genetic markers to immune responses to vaccination. These responses determine the inherent ability of individuals to protect themselves from the disease represented by the vaccine antigens and hence can be employed as a phenotype for disease resistance in disease association studies involving genes such as those within the MHC (Outteridge et al. Citation1988).
Major histocompatibility complex class I and II gene products play an important role in presentation of antigens essential for the onset of immunological responses. Several studies have investigated the polymorphisms of ovine MHC genes (Dukkipati et al. Citation2006b) and their role in resistance and/or susceptibility to diseases in sheep (Dukkipati et al. Citation2006a). In the studies reviewed, genes from class I and II regions were found to be significantly associated with resistance to a number of diseases, in particular gastrointestinal nematodiasis. In humans, several studies (reviewed by Selvaraj Citation2004) have established the association of various MHC genes (mostly DR and DQ) and susceptibility to pulmonary tuberculosis. Further, it was shown in mice that haplotypes at the H-2 (MHC) locus had a profound effect on the antigen-specific CD4+ T-cell responses, i.e. production of IFN-γ, subsequent to Mycobacterium tuberculosis infection (Kamath et al. Citation2004).
Genes from locations other than the MHC considered to be immunologically important include those coding for IFN-γ, SLC11A1, formerly known as the natural resistance-associated macrophage protein 1 (NRAMP-1), toll-like receptors, and nucleotide oligomerisation binding domain 2. A diallelic microsatellite marker locus, o(IFN)-γ, within the IFN-γ gene, was shown to be associated with resistance to gastrointestinal nematodiasis in sheep (Coltman et al. Citation2001). Alleles at the SLC11A1 gene in humans have been shown to be associated with susceptibility to mycobacterial infections (Awomoyi et al. Citation2002). Also, polymorphisms at an SLC11A1 microsatellite were found to be associated with PTB infection status in cattle (Pinedo et al. Citation2009). Two microsatellites, OVINRA1/NRAMP1 and OVINRA2, were found to be located within the 3' untranslated region of the ovine SLC11A1 region (Pitel et al. Citation1996). Alleles at the OVINRA1 locus were shown to be associated with probable susceptibility/resistance to clinical PTB in two naturally infected fine-wool Merino flocks in Australia (Reddacliff et al. Citation2005).
The objective of this study was to examine the association of polymorphisms at five microsatellite loci located in three immunologically significant chromosomal regions (MHC, IFN-γ and SLC11A1), with immune responses to vaccination against Map in Merino sheep in Australia.
Materials and methods
Phenotypes
Phenotypic data pertaining to antibody and IFN-γ responses recorded in the trial conducted in Australia for up to 54 months post-vaccination, together with blood samples from the trial sheep, were kindly provided by the Department of Primary Industries, New South Wales, Australia. All work involving sheep in this trial was conducted under the approval of the Elizabeth Macarthur Agricultural Institute Animal Ethics Committee, Menangle, NSW, Australia. Details of the experimental flocks, vaccination, and measurement of immune responses were provided in the final report for the project (Reddacliff Citation2005), and are briefly described here.
Experimental flocks
The vaccination trial in Australia was undertaken on three separate properties in the Central Tablelands of New South Wales. All properties were within 100–150 km of the Orange Agricultural Institute and Rural Lands Protection Board in Bathurst. Each property produced at least 800 fine-wool Merino lambs from unrelated ewe flocks (n˜300) that had bred their own female replacements. All three properties had >5% losses per annum due to PTB in susceptible age groups (2–4-year-olds).
Vaccination
On each property, 400 (200 vaccinates and 200 unvaccinated controls) predominantly female lambs were randomly selected for the trial when they were 1–3 months old. One ml of either vaccine (Gudair) or saline was administered to the lambs by S/C injection high on the neck behind the ear, using a 6-mm needle. On Property 1, spring-born lambs were vaccinated in December 1999, while on Properties 2 and 3, autumn-born lambs were vaccinated in June 2000. Vaccinates and controls on each property were grazed together throughout the trial.
Immune responses
Antibody and cell-mediated immune (CMI) responses in vaccinates and controls were assessed at different time-points for up to 54 months post-vaccination. The time-points varied between the properties. On Property 1, immunological responses were first assessed at 12 months post-vaccination, and subsequently at 18, 24, 30, 42 and 54 months post-vaccination. Immunological assessment on Properties 2 and 3 commenced at the time of vaccination. Subsequent assessments on Property 2 were made at 2, 8, 12, 18, 24, 36 and 48 months, while on Property 3 at 2, 8, 12, 18, 24, 36 and 42 months post-vaccination. At each time-point, 10 ml blood was obtained from each sheep into lithium-heparin tubes, and stored at room temperature until cultures for IFN-γ assay were set up, usually within 8 h of blood-sampling.
Cell-mediated immune responses were assessed by measuring the production of IFN-γ in blood in response to stimulation with Map antigens. Stimulation of whole blood with Johnin and avian purified protein derivatives (PPD), phosphate buffered saline (PBS) (negative control), and pokeweed mitogen (positive control), was carried out in separate reactions. Stimulated plasma was held overnight at 4°C, and production of IFN-γ was measured using enzyme immunoassay, using the Bovigam kit (Prionics AG, Zürich, Switzerland). The phenotype used to represent CMI in the current study was the difference between the IFN-γ responses to Johnin and avian PPD, measured as the optical density read at 450 nm (OD450), i.e. Johnin OD450—avian OD450. IFN-γ responses to Johnin and avian PPD on Properties 1 and 2 at time-points 18 and 12 months post-vaccination, respectively, were low, and even the responses to pokeweed mitogen (positive control) were very low, suggesting a problem with the assay. Hence, IFN-γ responses on the two properties at the said time-points were excluded from statistical analyses. Antibody responses were measured using enzyme immunoassay, using a commercial kit, Parachek (Prionics AG). The assay was performed on the plasma from blood samples stimulated with PBS in the IFN-γ assay. The phenotype used to represent humoral immunity was the antibody response measured as OD450 in the enzyme immunoassay.
Genotyping
Blood samples
Heparinised blood samples (0.5–1.0 ml from each animal) were obtained from 934 sheep. These were remnants of samples utilised for immune assays, and were under storage at −20°C for prolonged periods before they were obtained for DNA analysis. By the time blood samples were obtained, some of the sheep had died or been culled due to various reasons. Hence, of the original 600 vaccinates and 600 controls from the three properties, blood samples from only 504 (Property 1 n=171, Property 2 n=165, Property 3 n=168) vaccinates and 430 (Property 1 n=161, Property 2 n=138, Property 3 n=131) controls were available.
DNA extraction
DNA was extracted from the blood samples using a QIAamp DNA blood minikit (Qiagen Pty Ltd, Doncaster, Victoria, Australia). The quality and quantity of DNA were assessed using agarose (0.75%) gel electrophoresis.
Genetic markers employed
The primer sequences, forward primer 5' end fluorescent label for the employed markers, and location of the microsatellites they amplify, are detailed in .
Amplification of microsatellite DNA
The details of amplification of microsatellite DNA are outlined in . Briefly, the target DNA for the five primer pairs was amplified in four PCR (markers DYMS1 and o(IFN)-γ were coamplified). Each 20-μl PCR consisted of 5 nmol of each dNTP, 37.5–45.0 nmol MgCl2, 10 pmol each of forward and reverse primers, 1.25 units of platinum Taq DNA polymerase (Invitrogen Corporation, Carlsbad CA, USA), 2 μl of 10x PCR buffer, and 50–100 ng of template DNA. PCR was performed in a GeneAmp PCR system 9600 (Applied Biosystems, Foster City CA, USA), with an initial hold at 95°C for 5 minutes, followed by 30 cycles (94°C for 30 seconds, annealing temperature for 30 seconds, 72°C for 1 minute), and a final extension at 72°C for 7 minutes. The presence of amplification products and the approximate amount of DNA in the PCR products were assessed using agarose gel (2.5% UltraPure Agarose 1000; Invitrogen Corporation) electrophoresis.
Table 1. Details of markers used to genotype sheep (n=934) from a trial to evaluate a killed Johne's vaccine on three properties in Australia.
Determining PCR product lengths, and scoring genotypes
Employing an ABI3730 genetic analyser (Applied Biosystems), fluorescent labelling at the 5' end of forward primers for the five markers, with four different dyes, facilitated automated determination of the length of the PCR products. For each lamb, PCR products from the four different PCR were diluted and pooled based on their concentrations of DNA, and 1.0 μl of the pooled product used for the capillary run. Resulting chromatogram data from the genetic analyser were analysed using ABI Prism Gene-Mapper Software v3.7 (Applied Biosystems), to obtain the individual genotypes for all the markers, based on the lengths of the amplified products.
Statistical analysis
Phenotypes
The effects of vaccination, property and time on each of the immune response variables (antibody response and IFN-γ response) were analysed using SAS v9.1 (SAS Institute Inc, Cary NC, USA). The linear mixed model (Littell et al. Citation1998) included the fixed effects of treatments (vaccinates and controls), property, time (different time-points between 0 and 54 months post-vaccination), and their interaction, and random intercepts for animals within group within property. A first-order auto-regressive model of covariance error structure was used for repeated measures. LSM (± SE) were obtained for group, property, time-points, and their interactions, and were used to plot the graphs in Figures and 2, using SigmaPlot 10 (Systat Software Inc, Richmond CA, USA). Multiple comparisons among treatment effects were performed, and hence α (0.05) was corrected (αcorr) using a modified procedure for the rate of false discoveries (Narum Citation2006):
where i = 1 up to k comparisons. Associations were significant at αcorr, and considered a trend if αcorr=0.05. The effect of season of vaccination on antibody and IFN-γ responses was also tested. The model included the fixed effects of season (summer and winter), treatment (vaccinates and controls), and their interaction. Only time-points that were common to the three properties (12, 18 and 24 months) were considered.Estimating allelic and genotypic frequencies
Individual genotype data obtained from analysis of chromatograms using GeneMapper software were analysed using PROC ALLELE procedure in SAS/Genetics (SAS Institute Inc), in order to obtain allelic and genotypic frequencies, as well as polymorphic information content, heterozygosity (observed) and allelic diversity (expected heterozygosity), for the markers on different properties.
Test for Hardy-Weinberg equilibrium
Genotypic frequencies were tested for evidence of departure from Hardy-Weinberg equilibrium for each marker on each property as well as for the three properties together, employing a Markov chain method providing an exact test (Guo and Thompson Citation1992), using GENEPOP 3.4 software (http://genepop.curtin.edu.au/; Raymond and Rousset Citation1995a).
Genetic differentiation of properties
Differences in allelic and genotypic frequencies at each marker locus, among the three properties and between controls and vaccinates, within a property, were tested using GENEPOP 3.4. Allelic differences were assessed based on Fisher's exact test (Raymond and Rousset Citation1995b), and a log-likelihood exact test (Goudet et al. Citation1996) was used to compare genotypic frequencies.
Genetic effects on immune responses
The association of genetic polymorphisms at each of the five genetic marker loci on immune responses was tested in two ways, by fitting genotypic and allelic effects, using SAS v9.1. Since the distributions of antibody as well as IFN-γ responses at each time-point were positively skewed, OD450 values for antibody and IFN-γ responses were log (OD450 + 0.00001)-transformed, in order to achieve approximate normalisation of data. The effects of genotypes were tested separately for each marker locus within each property. For each marker, only genotypes occurring in at least five individuals each of vaccinated and control groups were evaluated for their effects. The model fitted was as follows: yijkl=Groupi + Genotypej + Timek + (Group*Genotype)ij + (Group*Time)ik + Animall + eijkl; where yijkl = log-transformed phenotype (antibody or IFN-γ response), Group i = 1, 2 (1 = controls, 2 = vaccinates), Genotype j = 1, 2, 3, .... n (number of genotypes varied for each marker within a property), Time k = 0, 2, .... 54 months post-vaccination (time-points varied between properties), Animal l = 1, 2, . . . random effect of animal l, and eijkl = residual error assumed to have an auto-regressive structure for animall, within a groupi.
LSM (± SE) were obtained for group, genotype, time, group*genotype, and group*time, and were used in multiple comparisons among different treatment effects. For multiple comparisons, α (0.05) was corrected, as described above (comparison of phenotypes), using a modified procedure for the rate of false discoveries (Narum Citation2006). The effects of presence vs absence of marker alleles were determined separately for each allele at each locus within each property. For each marker, only alleles occurring in at least six individuals each of vaccinated and control groups were evaluated for their effects. For determining the effect of a particular allele, individuals possessing at least a single copy of that allele were classified as ‘allele 1’ (allelic presence), while the individuals that lacked at least a single copy of the allele as ‘allele 2’ (allelic absence). The model employed was similar to that used for determining the genotypic effects (described above), except that ‘Genotypej’ in the equation was replaced with ‘Allelej’. Genotypes/alleles with significant (p<0.05) effects were summarised based on the consistency of their effects across the three properties, and categorised into ‘most likely’ and ‘probable’. ‘Most likely’ genotypes/alleles had a similar (either low or high) and significant (p<0.05) effect across all three properties, while ‘probable’ genotypes/alleles had a significant (p<0.05) and similar (either low or high) effect on two properties and either a similar but non-significant (p>0.05) effect or was absent (had insufficient numbers for evaluation) on the other property.
Results
Immune responses
The interaction of property, group (vaccinates vs controls) and time had a significant (p<0.01) effect on antibody responses as well as on IFN-γ responses. The trends of antibody and IFN-γ responses in vaccinates as well as controls on the three properties are depicted in , respectively. Antibody responses in control sheep from all three properties were fairly constant, and remained persistently low throughout the experimental period.
Figure 1. Trends of (a) antibody, and (b) interferon-γ (IFN-γ) responses (LSM ± SE) in sheep vaccinated with a killed Johne's vaccine (---) or unvaccinated (- - -) on three properties (


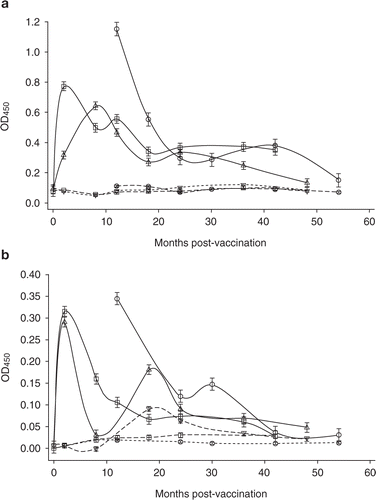
Antibody responses in vaccinates on Property 1 were higher at 12 and 18 months post-vaccination compared with those on Properties 2 and 3 (p<0.01). Antibody responses in vaccinates on Property 3 peaked earlier, and were higher at 2 months post-vaccination compared with those in vaccinates on Property 2 (p<0.01).
The overall IFN-γ responses in vaccinates on Property 1 differed from those in vaccinates on Properties 2 and 3 (p<0.01). IFN-γ responses in vaccinates on Properties 2 and 3 peaked by 2 months post-vaccination. On Property 2, IFN-γ responses returned to almost baseline levels by 8 months, and a second peak was evident at 18 months. On Property 3, IFN-γ responses decreased by 12 months to a lower but still higher level compared with that for the control group (p<0.01), that persisted for up to 24 months post-vaccination. Unvaccinated controls on Property 1 had consistently low IFN-γ responses throughout the experimental period. In contrast, in controls, the IFN-γ responses on Properties 2 and 3 were consistently higher during 18–48 months post-vaccination compared with those on Property 1. The overall antibody and IFN-γ responses (over the time-points of 12, 18 and 24 months studied) in lambs vaccinated during summer (Property 1) were higher compared with those vaccinated during winter (Properties 2 and 3) (p<0.01).
Marker alleles and genotypes
Genotypic results are summarised in and . Except for the o(IFN)-γ locus, that had only two alleles, all other four loci exhibited extensive polymorphisms, and the number of alleles ranged between 10 (OVINRA1) and 21 (DYMS1), and their corresponding genotypes ranged between 30 and 92. Heterozygosities varied between 37% (o(IFN)-γ) and 87% (SMHCC1), while information on polymorphic contents ranged from 0.31 (o(IFN)-γ) to 0.87 (DYMS1).
Genotypes at the five loci investigated were found to be mostly in Hardy-Weinberg equilibrium (p>0.05), with a few exceptions. DYMS1 genotypes on Properties 2 and 3 and OLADRB genotypes on Property 1 deviated from Hardy-Weinberg equilibrium (p<0.05). While excess heterozygosity was the cause for deviation at DYMS1 on Property 3, deficiency of heterozygosity resulted in departure from Hardy-Weinberg equilibrium at DYMS1 on Property 2 and at OLADRB on Property 1.
Table 2. Allelic and genotypic information pertaining to DYMS1, OLADRB and SMHCC1 loci in sheep from a trial to evaluate a killed Johne's vaccine on three properties (P1, P2, P3), and overall for the three properties, in Australia.
Table 3. Allelic and genotypic information pertaining to OVINRA1 and o(interferon)-γ (o(IFN)-γ) loci in sheep from a trial to evaluate a killed Johne's vaccine on three properties (P1, P2, P3), and overall for the three properties, in Australia.
Results from the tests for allelic and genotypic differences between properties as well as those between vaccinates and controls on each property are summarised in . Consideration of results over five marker loci indicated strong genetic (both allelic and genotypic) differences among the three properties, and genetic similarity between vaccinates and controls within each property.
Table 4. Allelic and genotypic differences between properties and groups within properties, pertaining to five microsatellite marker loci in sheep from a trial to evaluate a killed Johne's vaccine on three properties (P1, P2, P3) in Australia.
Table 5. Marker genotypes and alleles found to have significant and consistent effects on interferon-γ (IFN-γ) responses in vaccinated sheep from a trial to evaluate a killed Johne's vaccine on three properties (P1, P2, P3) in Australia.
Genetic effects on immune responses
The effects of marker genotypes/alleles on antibody as well as IFN-γ responses in controls on all three properties were found to be non-significant (p>0.05). Hence, only the allelic and genotypic effects in vaccinates are presented here.
Effects on antibody responses
Mean antibody responses (LSM, back-transformed to normal scale) for different genotypes and alleles at the five marker loci in vaccinates on the three properties are shown in Supplementary and 2Footnote 1 , respectively. Several genotypes/alleles at the marker loci investigated were found to significantly influence antibody responses to Johne's vaccination, some with increasing and others with decreasing effects. However, the effects of all such genotypes/alleles were inconsistent across the three properties. Genotypes/alleles found to be significantly associated with either high or low antibody responses on one or two properties either had opposite effects or were absent (had insufficient numbers for evaluation) on the other property/properties.
Effects on IFN-γ responses
Mean IFN-γ responses (LSM, back-transformed to normal scale) for different genotypes and alleles at the five marker loci in vaccinates on the three properties are shown in Supplementary Figures 3 and 4Footnote 1 , respectively. Several genotypes/alleles at all five marker loci were found to possess a significant effect on IFN-γ responses. However, the effects of only a few genotypes/alleles were consistent across the three properties. Genotypes/alleles at different marker loci possessing significant and consistent effects on IFN-γ responses are listed in . A genotype at the DYMS1 locus and an allele at the o(IFN)-γ locus were most likely to influence IFN-γ responses. Similarly, four genotypes, one at each of the OLADRB, SMHCC1, OVINRA1and o(IFN)-γ loci, and two alleles, one each at DYMS1 and OLADRB loci, probably influenced IFN-γ responses.
Discussion
This study examined the association of polymorphisms at five microsatellite loci with immune responses to vaccination against Map in Merino sheep. The trend in antibody production observed during the first 10 months post-vaccination was comparable to that seen in two live-attenuated vaccine response studies (Juste et al. Citation1994; Begg and Griffin Citation2005). Neither of those studies monitored antibody responses beyond 10 months post-vaccination. Also, consistent with the current findings, a previous study that monitored immune responses for 10 months post-vaccination found IFN-γ responses to peak by 6 weeks before returning to baseline levels by 10 months (Begg and Griffin Citation2005). The specificity and reliability of the Parachek and Bovigam kits used to measure antibody and IFN-γ responses, respectively, were demonstrated in several studies (Stewart et al. Citation2004; Begg and Griffin Citation2005).
In the current study, both antibody and IFN-γ responses in vaccinates on Property 1 were consistently higher than those in vaccinates on Properties 2 and 3. Individuals on Property 1 were vaccinated in summer (December), while those on Properties 2 and 3 were vaccinated in winter (June). The difference in responses between the properties could be due to an effect of season of vaccination, with sheep vaccinated in summer producing high initial responses that might have prevailed during the subsequent periods. However, no such effect of season on immune responses to vaccination against Map was reported in a different study in sheep (Goddard et al. Citation2000). Control sheep on Properties 2 and 3 exhibited consistently higher IFN-γ responses during 18–48 months post-vaccination compared with those on Property 1. It is likely that natural infection in flocks on Properties 2 and 3 might have resulted in these higher responses. This can be substantiated by the fact that control sheep on Properties 2 and 3 had a high prevalence of shedding Map in faeces during this period (Reddacliff et al. Citation2006).
High polymorphism at the three MHC marker loci (Table ) and the OVINRA1 locus (Table ) observed in the current study was consistent with that reported for DYMS1 (Buitkamp et al. Citation1996), OLADRB (Blattman and Beh Citation1992; Gruszczynska et al. Citation2002b), SMHCC1 (Gruszczynska et al. Citation2002a), and OVINRA1 (Pitel et al. Citation1996; Reddacliff et al. Citation2005; Worley et al. Citation2006) loci. In the current study, only two alleles (124 base pairs (bp) and 128 bp) were identified at the o(IFN)-γ locus, which is consistent with findings from several studies (Schmidt et al. Citation1996; Crawford and McEwan Citation1998; Reddacliff et al. Citation2005). However, alleles of sizes 126 and 130 bp were found in Soay sheep (Coltman et al. Citation2001), while a single 122-bp allele was found fixed in a large structured population (n=922) of wild sheep (Worley et al. Citation2006).
A notable feature with regard to the three MHC and the OVINRA1 loci in the current study was the presence of rare genotypes/alleles (<1.0% frequency; Tables and ). These rare genotypes/alleles indicated that the rate of mutation of microsatellites (number of rare alleles) varied between loci within the MHC, with the rate of mutation at DYMS1 being considerably higher in comparison with that at SMHCC1 and OLADRB. Rare genotypes/alleles, especially at MHC loci, have been reported previously (Buitkamp et al. Citation1996; Gruszczynska Citation1999). It has been hypothesised that rare MHC alleles would provide a selective advantage to the individuals possessing them, compared with individuals with more frequent alleles, in responding to a new variant of an invading parasite (Froeschke and Sommer Citation2005).
Genotypes at the five loci investigated, with a few exceptions (DYMS1 on Properties 2 and 3, and OLADRB on Property 1), were found to be in Hardy-Weinberg equilibrium. As the three flocks investigated in the current study had been run on a self-replacement basis for several decades (Reddacliff et al. Citation2006), it is likely that the exceptions observed might be due to balancing selection at coding loci linked to the microsatellites. Sourcing replacement rams externally might have also contributed to departure from Hardy-Weinberg equilibrium.
Perusal of results from tests for allelic and genotypic differentiation of properties (Table ) suggests that each property was distinct in terms of allelic and genotypic frequencies. The genetic differences between properties were the result of variation in both the number and frequency of genotypes/alleles within each marker locus. As the flocks on the three properties were independent, with self-replacement for several decades, the observed genetic differences among the properties are unsurprising.
The current study, which relied on data from a trial conducted earlier in Australia, had some inherent limitations. Blood samples for the current study were obtained nearly 4 years after the start of the original vaccine trial. By that time, several sheep, especially in the control group, were lost (either culled or deceased), and of the original 1,200 sheep, samples could be obtained from only 934. The majority of the dead animals were diagnosed positive for PTB. Attempts to recover DNA from formalin-fixed tissues from those sheep were unsuccessful. Genetic information from those sheep would have made this study more effective, and eliminated possible bias. Also, immune response time-point data varied among the properties, e.g. on Property 1 the first recording was obtained at 12 months post-vaccination. Because of this and the observed genetic differences (Table ) between the three properties, genetic associations with phenotypes could not be tested using data pooled across the three properties. Such pooled analysis would have greatly increased the power of analysis. Also, using a single measure, such as the value at a maximum response time-point or area under the curve of the immune response, rather than using data across all time-points (as done in the current study), was considered. The effects of genotypes/alleles at two marker loci (o(IFN)-γ and OVINRA1) were tested using area under the curve as the phenotype, and the results were similar to those presented here. However, the data from the study had missing observations at one or more time-points for several individuals, hence the current model, that considered data across all time-points, was chosen.
This study revealed significant and consistent effects of genotypes/alleles (Table ) at five marker loci located within the MHC, SLC11A1 and IFN-γ gene regions, on IFN-γ responses in sheep vaccinated against Map. Considering the role of those gene products in eliciting immune responses, these associations are not surprising. Four of these markers are located within the functional genes. DYMS1 is located within the 5' region of the class II Ovar-DYA gene, and polymorphisms at the locus were associated with gastrointestinal nematodiasis (Buitkamp et al. Citation1996). SMHCC1 is located in the Ovar-Mhc I gene region (Groth and Wetherall Citation1994), and polymorphisms at the locus were associated with gastrointestinal nematodiasis in two different studies (Buitkamp et al. Citation1996; Charon et al. Citation2001). Marker OVINRA1 is located in the 3' untranslated region of the ovine SLC11A1 gene, and a study in Merino sheep revealed possible associations of alleles 162 and 160 with susceptibility and resistance, respectively, to clinical PTB (Reddacliff et al. Citation2005). However, in the current study, those two alleles had non-significant and inconsistent effects on antibody as well as IFN-γ responses.
The diallelic marker o(IFN)-γ locus, located within the IFN-γ gene, had a significant and consistent effect on IFN-γ responses. Individuals with genotype 128/128 had significantly higher IFN-γ responses than those with 124/124 and 124/128, on Properties 1 and 3 (on Property 1, the effect was similar but non-significant). Conversely, it can be said that allele 124 had a dominant reducing effect on IFN-γ responses, that was evident in the tests for allelic effects. Positive associations of alleles at this locus with gastrointestinal nematodiasis have been reported in three different studies. While the larger (128 bp) and smaller (124 bp) alleles at the locus were found to be associated with nematode resistance and susceptibility, respectively, in a study in New Zealand (Crawford and McEwan Citation1998), the reverse was true in two other studies involving Soay (Coltman et al. Citation2001) and Texel (Sayers et al. Citation2005) sheep. Marker OLADRB, located in intron 5 of class II pseudogene Ovar-DRB2 (Blattman and Beh Citation1992), was found to be in linkage disequilibrium with the functional gene Ovar-DRB1 (Schwaiger et al. Citation1996).
In addition to the genotypes/alleles listed in Table , a number of others at those loci were also found to possess significant effects on immune responses, but their effects were inconsistent across the three properties. It is possible that environmental variations such as season of vaccination, prevalence of natural Map infection, and exposure to Mycobacterium avium species, might have resulted in differential expression of coding regions linked to the microsatellite loci. Genotype-by-environment interaction was also reported in a study on association of polymorphism of MHC class II genes with resistance/susceptibility to footrot in sheep (Escayg et al. Citation1997). It is interesting to note that none of the marker genotypes/alleles had significant and consistent effects on antibody responses in the current study.
It is important to consider how, in the current study, the observed significant effects of marker genotypes/alleles on immune responses might impact on the protection of sheep against natural Map infection. CMI responses play an important role in protection against Map, and are usually evident relatively early in infection, and remain active for months or years (Clarke Citation1997). It had been shown that IFN-γ responses, a good indicator of CMI, varied between animals considered immune and susceptible to experimental infection with Map (Gwozdz et al. Citation2000). IFN-γ responses in immune sheep were consistently and significantly higher than those in susceptible sheep between Weeks 9–36 post-infection. However, immune responses to a vaccine containing either live-attenuated or killed pathogens constitute a complex phenomenon, probably influenced by several genes.
Strong long-lasting CMI responses, measured using a lymphocyte transformation test using peripheral blood mononuclear cells, have been induced in goats vaccinated with a killed vaccine (Singh et al. Citation2007). It is apparent from the results of earlier studies (Juste et al. Citation1994; Gwozdz et al. Citation2000) that vaccination induced higher immune responses compared with those seen in sheep experimentally infected with Map. However, it is unclear if animals able to elicit high or low immune responses to vaccination can also show immune responses at corresponding levels in response to natural or artificial infection. Also, it is not known whether the production of IFN-γ induced by vaccination involves the same immune effector cells activated following natural infection. If so, it is likely that animals able to elicit high immune responses to vaccination can more effectively combat Map infections, while those with poor responses are likely to be susceptible to infection.
The possible confounding effect of the vaccine's adjuvant on the genetic associations observed in this study must also be considered. Gudair vaccine has a new-generation oil adjuvant. Like any adjuvant, it will potentiate an immune response to the vaccine's antigens. However, it is unknown whether this potentiation is uniform across all individuals or whether it is variable from individual to individual. If the latter scenario is correct, the observed genetic associations may be confounded. Consequently, the role of the identified genotypes/alleles in immune responses to natural Map infection should be further studied, in order to determine their possibility as markers for susceptibility/resistance to the disease.
In conclusion, this study has provided an insight into genetic polymorphisms at five marker loci within the MHC, SLC11A1 and IFN-γ gene regions, and their influence on immune responses to vaccination of sheep against Map. Extensive polymorphisms were evident at the loci investigated, and five genotypes and three alleles possessing a significant and consistent effect on IFN-γ responses to vaccination were identified. However, considering the complexity of the protective immune response to a vaccine, further studies are warranted to determine the role of these markers in the immune response to natural Map infection.
69154supplementary_figures.pdf
Download PDF (200.9 KB)Acknowledgements
Financial support for the project from Meat & Wool New Zealand, and the award of a doctoral scholarship by Massey University, New Zealand, to the first author are gratefully acknowledged. The authors are thankful to Meat and Livestock Australia, the New South Wales Department of Primary Industries, and the University of Sydney, for providing access to blood samples and immunological data utilised in the study.
Notes
References
- Awomoyi , AA , Marchant , A , Howson , JMM , McAdam , KPWJ , Blackwell , JM and Newport , MJ . 2002 . Interleukin-10, polymorphism in SLC11A1 (formerly NRAMP1), and susceptibility to tuberculosis . Journal of Infectious Diseases , 186 : 1808 – 1814 .
- Begg , DJ and Griffin , JFT . 2005 . Vaccination of sheep against M. paratuberculosis: immune parameters and protective efficacy . Vaccine , 23 : 4999 – 5008 .
- Blattman , AN and Beh , KJ . 1992 . Dinucleotide repeat polymorphism within the ovine major histocompatibility complex . Animal Genetics , 23 : 392 – 392 .
- Buitkamp , J , Filmether , P , Stear , MJ and Epplen , JT . 1996 . Class I and class II major histocompatibility complex alleles are associated with faecal egg counts following natural, predominantly Ostertagia circumcincta infection . Parasitology Research , 82 : 693 – 696 .
- Bush , RD , Windsor , PA and Toribio , JA . 2006 . Losses of adult sheep due to ovine Johne's disease in 12 infected flocks over a 3-year period . Australian Veterinary Journal , 84 : 246 – 253 .
- Charon , KM , Moskwa , B , Kuryl , J , Gruszczynska , J and Rutkowski , R . 2001 . Relationship between polymorphism in locus OMHC1 (MHC class I) and resistance to nematodes in Polish Heatherhead sheep . Animal Science Papers and Reports , 19 : 285 – 292 .
- Clarke , CJ . 1997 . The pathology and pathogenesis of paratuberculosis in ruminants and other species . Journal of Comparative Pathology , 116 : 217 – 261 .
- Coltman , DW , Wilson , K , Pilkington , JG , Stear , MJ and Pemberton , JM . 2001 . A microsatellite polymorphism in the gamma interferon gene is associated with resistance to gastrointestinal nematodes in a naturally-parasitized population of Soay sheep . Parasitology , 122 : 571 – 582 .
- Crawford , AM and McEwan , JC . 1998 . Identification of Animals Resistant to Nematode Parasite Infection , Wellington, , NZ : Ovita .
- Dukkipati , VSR , Blair , HT , Garrick , DJ and Murray , A . 2006a . ‘Ovar-Mhc’ — Ovine major histocompatibility complex: Role in genetic resistance to diseases . New Zealand Veterinary Journal , 54 : 153 – 160 .
- Dukkipati , VSR , Blair , HT , Garrick , DJ and Murray , A . 2006b . ‘Ovar-Mhc’ — Ovine major histocompatibility complex: Structure and gene polymorphisms . Genetics and Molecular Research , 5 : 581 – 608 .
- Escayg , AP , Hickford , JGH and Bullock , DW . 1997 . Association between alleles of the ovine major histocompatibility complex and resistance to footrot . Research in Veterinary Science , 63 : 283 – 287 .
- Froeschke , G and Sommer , S . 2005 . MHC class II DRB variability and parasite load in the striped mouse (Rhabdomys pumilio) in the Southern Kalahari . Molecular Biology and Evolution , 22 : 1254 – 1259 .
- Goddard , PJ , Fawcett , AR , Macdonald , AJ and Reid , HW . 2000 . The behavioural, physiological and immunological responses of lambs from two rearing systems and two genotypes to exposure to humans . Applied Animal Behaviour Science , 66 : 305 – 321 .
- Goudet , J , Raymond , M , De Meeüs , T and Rousset , F . 1996 . Testing differentiation in diploid populations . Genetics , 144 : 1933 – 1940 .
- Groth , DM and Wetherall , JD . 1994 . Dinucleotide repeat polymorphism within the ovine major histocompatibility complex class I region . Animal Genetics , 25 : 61 – 61 .
- Gruszczynska , J . 1999 . Polymorphism of the OLA-DRB1 (MHC class II) gene in German Merino sheep . Annals of Warsaw Agricultural University, Animal Science , 35 : 125 – 132 .
- Gruszczynska , J , Charon , KM , Swidarek , W and Sawera , M . 2002a . Microsatellite polymorphism in locus OMHC1 (MHC class I) in Polish Heath sheep and Polish Lowland sheep (Zelazna variety) . Journal of Applied Genetics , 43 : 217 – 222 .
- Gruszczynska , J , Swidarek , W , Charon , KM , Kuryl , J , Cieslak , D and Pierzchala , M . 2002b . Microsatellite polymorphism of pseudo-gene OLA-DRB2 in intron 5 in two Polish sheep breeds . Animal Science Papers and Reports , 20 : 67 – 72 .
- Guo , SW and Thompson , EA . 1992 . Performing the exact test of Hardy-Weinberg proportions for multiple alleles . Biometrics , 48 : 361 – 372 .
- Gwozdz , JM , Thompson , KG , Manktelow , BW , Murray , A and West , DM . 2000 . Vaccination against paratuberculosis of lambs already infected experimentally with Mycobacterium avium subspecies paratuberculosis . Australian Veterinary Journal , 78 : 560 – 566 .
- Harris , NB and Barletta , RG . 2001 . Mycobacterium avium subsp. paratuberculosis in veterinary medicine . Clinical Microbiology Reviews , 14 : 489 – 512 .
- Hickey , SM , Morris , CA , Dobbie , JL and Lake , DE . 2003 . Heritability of Johne's disease and survival data from Romney and Merino sheep . Proceedings of the New Zealand Society of Animal Production , 63 : 179 – 182 .
- Juste , RA , Garcia Marin , JF , Peris , B , Saez de Ocariz , C and Badiola , JJ . 1994 . Experimental infection of vaccinated and non-vaccinated lambs with Mycobacterium paratuberculosis . Journal of Comparative Pathology , 110 : 185 – 194 .
- Kamath , AB , Alt , J , Debbabi , H , Taylor , C and Behar , SM . 2004 . The major histocompatibility complex haplotype affects T-cell recognition of mycobacterial antigens but not resistance to Mycobacterium tuberculosis in C3H mice . Infection and Immunity , 72 : 6790 – 6798 .
- Koets , AP , Adugna , G , Janss , LLG , van Weering , HJ , Kalis , CHJ , Wentink , GH , Rutten , VPMG and Schukken , YH . 2000 . Genetic variation of susceptibility to Mycobacterium avium subsp. paratuberculosis infection in dairy cattle . Journal of Dairy Science , 83 : 2702 – 2708 .
- Kudahl , AB and Nielsen , SS . 2009 . Effect of paratuberculosis on slaughter weight and slaughter value of dairy cows . Journal of Dairy Science , 92 : 4340 – 4346 .
- Littell , RC , Henry , PR and Ammerman , CB . 1998 . Statistical analysis of repeated measures data using SAS procedures . Journal of Animal Science , 76 : 1216 – 1231 .
- Narum , SR . 2006 . Beyond Bonferroni: Less conservative analyses for conservation genetics . Conservation Genetics , 7 : 783 – 787 .
- Outteridge , PM , Windon , RG and Dineen , JK . 1988 . An ovine lymphocyte antigen marker for acquired resistance to Trichostrongylus colubriformis . International Journal for Parasitology , 18 : 853 – 858 .
- Pinedo , PJ , Buergelt , CD , Donovan , GA , Melendez , P , Morel , L , Wu , R , Langaee , TY and Rae , DO . 2009 . Candidate gene polymorphisms (BoIFNG, TLR4, SLC11A1) as risk factors for paratuberculosis infection in cattle . Preventive Veterinary Medicine , 91 : 189 – 196 .
- Pitel , F , Lantier , I , Gellin , J , Elsen , JM and Lantier , F . 1996 . Two polymorphic microsatellite markers close to the ovine NRAMP gene . Animal Genetics , 27 : 60 – 61 .
- Raizman , EA , Fetrow , J , Wells , SJ , Godden , SM , Oakes , MJ and Vazquez , G . 2007 . The association between Mycobacterium avium subsp. paratuberculosis fecal shedding or clinical Johne's disease and lactation performance on two Minnesota, USA dairy farms . Preventive Veterinary Medicine , 78 : 179 – 195 .
- Raymond , M and Rousset , F . 1995a . GENEPOP (version 1.2): population genetics software for exact tests and ecumenicism . Journal of Heredity , 86 : 248 – 249 .
- Raymond , M and Rousset , F . 1995b . An exact test for population differentiation . Evolution , 49 : 1280 – 1283 .
- Reddacliff , LA . 2005 . Field Evaluation of OJD Control Using Gudair , Sydney, , Australia : Meat and Livestock Australia Limited . http://ses.library.usyd.edu.au/bitstream/2123/936/1/OJD.009%20Final%20report.pdf (accessed 07 July 2010)
- Reddacliff , LA , Beh , K , McGregor , H and Whittington , RJ . 2005 . A preliminary study of possible genetic influences on the susceptibility of sheep to Johne's disease . Australian Veterinary Journal , 83 : 435 – 441 .
- Reddacliff , LA , Eppleston , J , Windsor , P , Whittington , R and Jones , S . 2006 . Efficacy of a killed vaccine for the control of paratuberculosis in Australian sheep flocks . Veterinary Microbiology , 115 : 77 – 90 .
- Sayers , G , Good , B , Hanrahan , JP , Ryan , M and Sweeney , T . 2005 . Intron 1 of the interferon gamma gene: Its role in nematode resistance in Suffolk and Texel sheep breeds . Research in Veterinary Science , 79 : 191 – 196 .
- Schmidt , P , Ludt , C , Kuhn , CH and Buitkamp , J . 1996 . A diallelic tetranucleotide repeat (GT3)5 or 6, within intron 1 of the ovine interferon-γ gene . Animal Genetics , 27 : 437 – 438 .
- Schwaiger FW Maddox J Ballingall K Buitkamp J Crawford AM et al. The ovine major histocompatibilty complex The Major Histocompatibility Complex Region of Domestic Animal Species Schook LB Lamont SJ CRC Press Florida, , USA 1996 121 176 In
- Selvaraj , P . 2004 . Host genetics and tuberculosis susceptibility . Current Science , 86 : 115 – 121 .
- Singh , N , Kala , SN , Vihan , VS and Singh , SV . 1990 . Genetic study on the susceptibility to Johne's disease in goats . Indian Journal of Animal Sciences , 60 : 1163 – 1165 .
- Singh , SV , Singh , PK , Singh , AV , Sohal , JS , Gupta , VK and Vihan , VS . 2007 . Comparative efficacy of an indigenous ‘inactivated vaccine’ using highly pathogenic field strain of Mycobacterium avium subspecies paratuberculosis ‘Bison type’ with a commercial vaccine for the control of Capriparatuberculosis in India . Vaccine , 25 : 7102 – 7110 .
- Stewart , DJ , Vaughan , JA , Stiles , PL , Noske , PJ , Tizard , MLV , Prowse , SJ , Michalski , WP , Butler , KL and Jones , SL . 2004 . A long-term study in Merino sheep experimentally infected with Mycobacterium avium subsp. paratuberculosis: clinical disease, faecal culture and immunological studies . Veterinary Microbiology , 104 : 165 – 178 .
- Worley , K , Carey , J , Veitch , A and Coltman , DW . 2006 . Detecting the signature of selection on immune genes in highly structured populations of wild sheep (Ovis dalli) . Molecular Ecology , 15 : 623 – 637 .
- 1 http://www.sciquest.org.nz/node/69154