Abstract
New Zealand's bovine tuberculosis (TB) control programme has greatly reduced the burden of tuberculosis on the farming industry, from 11% of mature cattle found with TB at slaughter in 1905 to <0.003% in 2012/13. New Zealand implemented TB control measures in cattle from the mid-twentieth century, and later in farmed deer. Control was based on established methods of tuberculin testing of herds, slaughter of suspect cases, and livestock movement control. Unexplained regional control failures and serious disease outbreaks were eventually linked to wildlife-vectored infection from the introduced Australian brushtail possum (Trichosurus vulpecula), which also triggered a wildlife disease complex involving a range of introduced species. This paper reviews the progressive elucidation of the epidemiology of Mycobacterium bovis in New Zealand's wildlife and farmed livestock, and the parallel development of research-led, multi-faceted TB control strategies required to protect New Zealand's livestock industries from damaging infection levels. The adoption of coordinated national pest management strategies, with increasingly ambitious objectives agreed between government and industry funders, has driven a costly but very successful management regime targeted at controlling TB in the possum maintenance host. This success has led to initiation of a strategy designed to eradicate TB from New Zealand's livestock and wildlife, which is considered a realistic long-term prospect.
Introduction
This paper reviews the history of New Zealand's bovine tuberculosis (TB) control programme up to 2013. It summarises the key research, operational developments and systematic observations that have improved understanding of the epidemiology of the disease in New Zealand, led to better disease control decision-making and improved the cost-effectiveness of control programmes, especially in light of the emergence of wildlife-vectored disease as a significant factor in New Zealand. Ultimately, the success of the New Zealand control programme has been firmly based on intensive control of the disease in populations of the introduced Australian brushtail possum (Trichosurus vulpecula), the main wildlife maintenance host and vector of TB in New Zealand.
The introduction of a national test and slaughter programme for cattle initially made major progress towards eradicating TB from New Zealand's herds. However, the emergence of TB in possums as well as other wild animal species in a number of locations reversed these early gains and had a major impact on the government-led TB control programme, leading to requirements for farmers to fund an increasing share of disease control costs. This in turn led to the formation of the Animal Health Board (AHB) (subsequently re-named TBfree New Zealand Ltd from July 2013) as an independent organisation solely responsible and accountable for developing and implementing government and industry-approved strategies and plans for controlling TB in New Zealand. National Pest Management Strategies (subsequently termed National Pest Management Plans under the Biosecurity Law Reform Act 2012) were developed from a science base but also took account of observational and operational knowledge, organisational capability and the practical needs of farmers.
A unique body of strategically applied research and development has been instrumental in helping to solve complex problems of the epidemiology and disease transmission to livestock of TB in wildlife. This research has supported the development of cost-effective, environmentally acceptable tools and tactics for wildlife disease surveillance and control. The application of smart software and geospatial tools to disease modelling, data analysis and programme management has led to continuing improvements in operational design and delivery, enabling increasingly challenging disease control objectives to be achieved.
Bovine tuberculosis in New Zealand – the early years
Cattle were first introduced into New Zealand in 1814 (Hopkirk Citation1935). Historical records from the United Kingdom and Europe indicate that TB in cattle was widespread by the start of the nineteenth century (Francis Citation1947). Unrestricted trade in live cattle during this time undoubtedly led to the international spread of bovine TB (Smith Citation2012), including to New Zealand. It is unknown when bovine TB was first introduced into New Zealand, but as early as 1880 the disease was well established, with between 4% and 7% of cattle slaughtered in Wellington considered infected with M. bovis, as were large numbers of young cattle in Taranaki (Laing Citation1964). In 1893 bovine TB became a notifiable disease in New Zealand, with clinically affected animals being condemned and compensation paid to owners at one-half the market value (Anonymous Citation1905).
Further legislation in 1894 enabled the appointment of inspectors with powers to condemn diseased meat and affected stock brought to slaughter (Laing Citation1970). In the absence of an effective control programme, bovine TB had become widespread, causing a substantial proportion of cattle to be affected with clinical disease and frequent condemnation of carcasses at slaughter (). As well as discovering the tubercle bacillus in 1882, Robert Koch also developed tuberculin which was shown in 1891 to be a valuable diagnostic skin test agent in animals (Sakula Citation1982). In 1911, a Privy Council instigated Royal Commission reported conclusive evidence that the bovine tubercle bacillus (M. bovis) had a very broad host range and was transmissible to humans (Francis Citation1959). Importantly, it also provided sufficient evidence on which to base future bovine TB control programmes. The intradermal caudal-fold tuberculin test became the primary test for bovine TB control programmes in New Zealand and elsewhere, with voluntary tuberculin testing and slaughter of test-positive (reactor) cattle starting in New Zealand in 1922. However uptake was generally poor and sporadic, mainly because compensation paid for the slaughter of reactor cattle was much less than market value as well as farmers' perceptions that a high proportion of reactors sent to slaughter were false positives (Laing Citation1955).
Table 1. Number of cases of tuberculosis identified in cattle and pigs at export meat works and abattoirs in New Zealand during a 12-month period in 1904–1905 (from Anonymous Citation1905).
TB control programme from 1930–1969
Up to 1956, TB testing of cattle remained voluntary and TB control was ineffectual, being based mainly on inspection of dairy herds by government stock inspectors and slaughter of clinical cases, with only limited skin testing (Davidson Citation2002). The annual prevalence of TB in cattle for the period 1930–1951, as measured by cattle found with TB lesions at slaughter and dairy cattle condemned as clinical TB cases, increased from 0.44% of 3.77 million cattle in 1930/31 to 1.02% of 4.8 million cattle in 1950/51. Over this 20-year period an average of 35,500 cattle per year were found with TB lesions at slaughter or condemned as clinical TB cases. During the same period, TB lesions were found in 8.3–18.3% (mean 13.4%) of domestic pigs slaughtered annually (from between 438,000 and 1,080,000 pigs per annum) (Anonymous Citation1930–1950). As domestic pigs were commonly raised on skim milk as a by-product from cream production, this indicates that excretion of M. bovis into cow's milk was widespread. Nevertheless, by the 1940s several groups of fresh milk supply dairy herds were being regularly tested and TB was eradicated from herds supplying some smaller towns (Laing Citation1955).
Compulsory TB testing was introduced for fresh milk supply herds in 1956, and for herds supplying milk for factory processing in 1961 (Davidson Citation2002). By 1970, all dairy cattle were under compulsory TB testing, and this was progressively extended over the next 5 years to beef herds. This heralded the start of systematic control of bovine TB in New Zealand. The progressive update and expansion of cattle TB testing reflected concerns arising in the 1950s and 1960s that levels of TB and brucellosis infection in cattle herds could adversely affect New Zealand's export trade in beef and dairy products (Davidson Citation2002). A similar concern was expressed in Australia in 1966 and 1967 which led to the introduction of the successful Brucellosis and Tuberculosis Eradication Campaign (Lehane Citation1996).
TB control programme 1970–1994
Limited response to test and slaughter
The numbers of cattle reactors to tuberculin testing (all skin-test positive cattle were deemed reactors and sent to slaughter) and infected herds fell relatively rapidly following the introduction of formal test and slaughter policies for dairy herds. By March 1971, 27,905 herds comprising 2.9 million cattle had passed two consecutive clear tuberculin tests and were considered free of TB (Anonymous Citation1971). This decline was consistent with results from Australia (Cousins and Roberts Citation2001), North America (Essey and Koller Citation1994) and Western Europe (Reviriego Gordejo and Vermeersch Citation2006) following the widespread introduction of tuberculin test and slaughter policies. However in New Zealand there were some notable exceptions to this rapid clearance of infection. Comparison of reactor rates from whole-herd TB tests in dairy cattle in the Buller district of the West Coast region with those for the rest of New Zealand for the period 1962 to March 1968 shows both a higher reactor rate at initial herd tests, and a much slower decline in reactor rates at each subsequent whole-herd test for the Buller area (Anonymous Citation1968, ).
Table 2. Number of dairy cattle tested and percentage that tested positive (reactor) for tuberculosis (TB) between 1962 to 31 March 1968 in the Buller district of the West Coast region and the rest of New Zealand (from Anonymous Citation1968; J. Edington2, pers. comm.).
In seeking an explanation for the low clearance rates in Buller district herds, the possum came under suspicion, because M. bovis had been isolated from a possum in the district in 1967 (Ekdahl et al. Citation1970). However at that time beef cattle were not being tested, and were often mixed with non-lactating dairy cattle, so possums with TB could not be confirmed as the source of infection for dairy cattle. To resolve that confounding factor, all beef and dairy cattle over 6 weeks of age in the Buller district were tested every 3 months, with immediate slaughter of both skin-test positive cattle and any cattle not presented for test. Hygiene measures such as cleaning and disinfection of water troughs and milking sheds were also introduced for infected dairy herds (N BeatsonFootnote1 pers. comm.).
Despite the resulting eight whole-herd tests per herd between January 1970 and December 1971, and the extra hygiene measures, Footnote incidence of TB in Buller district cattle remained high. This is illustrated by data from the Buller South area (). Here, the annual incidence of TB reactors remained above 17% in 1970 and 1971. An average of 57% of these TB reactors had visible lesions of TB at slaughter. The percentage of herds that were infected at December 1971 was 82%, the same as in March 1970. These outcomes clearly demonstrated that common grazing of non-lactating dairy cattle with beef cattle, within-herd infection, or cattle movement were not the fundamental causes of local persistence of TB infection in cattle.
Figure 1. Percentage of total cattle tested for tuberculosis (TB) that were test-positive and sent for slaughter (red bars) and percentage of herds (n=24) that were infected with TB (blue triangles) in the Buller South area (mean combined cattle population 4,474) of the West Coast region of New Zealand, from 1970–1980. Blue lines represent linear trends in percentage infected herds before (solid line) and after (dashed line) commencement of intensive possum control in autumn 1972 (black arrow). (J. Edington2, pers. comm.).
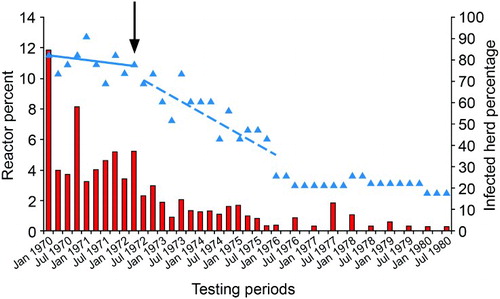
In 1971, a trial to determine whether possums with TB were a source of infection for cattle was undertaken in the West Coast region. Twenty-nine test-negative calves were grazed in a paddock from which 12% of possums had been found with TB lesions some 6 months earlier. After 6 months grazing in the paddock, the calves were retested, with 26/29 being test-positive, and 16 having gross lesions of TB at slaughter. This strongly implicated possums as a source of infection for cattle (Davidson Citation1976). As a result, in late 1971 possums were killed throughout the Buller district and bodies submitted for necropsy, histology and culture for M. bovis (Adlam Citation1977). Tuberculous possums were identified on, or adjacent to, many farms with infected herds. Intensive possum control was therefore implemented in the Buller district between 1972 and 1978. The annual incidence of TB reactors in the Buller-South area subsequently fell from 17% in 1971 to 4.0% by January 1976. The percentage of infected herds fell from 82 to approximately 30% over the same time period, and then declined to 22% by June 1976 (). Similar reductions in reactor rates and herd infection levels were observed throughout the rest of Buller district following possum control (Davidson Citation1976). Although not formally confirmed until much later, this provided compelling evidence that TB in possums had become a major source of spillback infection for cattle.
Emergence and recognition of the problem of TB in wildlife
Being highly valued for their fur and generally considered harmless, possums were successfully introduced and established at Riverton in Southland in 1858 to provide the basis for a fur trade. Further introductions and redistributions were freely made throughout New Zealand over the next 50 years, eventually leading to a ubiquitous national presence (Pracey Citation1974; Clout and Ericksen Citation2000). The relevant ecology and biology of possums in New Zealand have been comprehensively reviewed in a series of papers (Montague Citation2000) which also documents the emergence of possums as a serious environmental pest in New Zealand.
Possum densities in forest and bush adjacent to farmland in the Buller district were surveyed between late 1940 and 1960 and identified both that the overall distribution of possums was increasing and that their regional densities in favourable (forested) habitats were increasing (Pracy Citation1997). Retrospectively, it is apparent that by the 1960s, possum densities in some areas were close to carrying capacity and well above the threshold subsequently suggested for TB establishment and maintenance (Barlow Citation1991a, b; Caley et al. Citation1999), including near Mokihinui where possums with TB were first identified in 1967. Both then (and now) it was not unusual for cattle to graze in close proximity to either patchy or extensive possum habitat. Although possums are primarily herbivorous, they are opportunistic omnivores and have been observed eating meat, carrion (Nugent et al. Citation2000), bird's eggs, hatchlings and insects (Brown et al. Citation1993; Cowan Citation2001). Nugent and Whitford (Citation2007) have shown that possums will occasionally eat muscle and lymph node tissue from carcass remnants of pig and deer, and it has been suggested that such scavenging is the mechanism by which TB became established in possum populations (Barron et al. Citation2013).
It is also plausible that infected populations could have resulted from possums feeding on tuberculous cattle carcasses, as prior to the introduction of formal test and slaughter programmes, dairy cattle with clinical TB symptoms were often condemned and slaughtered on farms. It is therefore probable that during the 1950s and 1960s the increasing possum population in the Buller district would have had access to tuberculous cattle carcasses following poorly managed slaughter and disposal of clinical TB cattle cases on farms (Laing Citation1955). Alternative hypotheses as to how possums became infected include close contact with infectious cattle, or through ingestion of other TB contaminated food sources.
Possums were later identified as a true maintenance host of TB (Morris and Pfeiffer Citation1995). They appear to be highly susceptible to M. bovis infection. Identified natural routes whereby possums become infected include respiratory, ingestion or percutaneous (Adlam Citation1977; Coleman and Cooke Citation2001; Jackson Citation2002). Intra-specific transmission of infection in possums is considered to be largely through close contact between adults, at den sites or as a result of fighting and dominance behaviours associated with mating (Nugent et al. Citation2015). Most infected possums die within 6 months of becoming infected (Cowan Citation2001; Nugent et al. Citation2013), though possums with TB lesions have survived for 12 months and at least one survived for 22 months (Jackson Citation1995). Transmission of infection to young possums in their mother's pouch has also been described, and infected migrating juveniles may play a role in spreading infection to other possum populations (Morris and Pfeiffer Citation1995). Tuberculous possums are commonly found clustered spatially and temporally, with often large distances between infected groups (Coleman Citation1988). The prevalence of TB in infected possum populations is generally low (<5%), though a localised prevalence of 53% has been recorded (Coleman et al. Citation1999). The overall patchiness and low prevalence of disease means that it is often difficult to detect TB in possum populations through surveys.
Although there was no evidence of TB establishing in possums before the 1960s, despite many millions being killed by fur trappers, TB was found in many different localities between 1967 and 1981. By the early 1980s, multiple different DNA strain types had been identified among 83 M. bovis isolates from possums. Isolates of the same type were usually found in the same geographic area, indicating the disease established in possum populations separately on several occasions (Collins et al. Citation1986). Other species were also involved in the emergence of TB as a wildlife disease in New Zealand. Tuberculosis was identified histologically in a wild red deer (Cervus elaphus) in 1954 (Anonymous Citation1955) and in feral pigs (Sus scrofa) in 1964 (Ekdahl et al. Citation1970). Tuberculosis was subsequently identified in ferrets (Mustela furo) (de Lisle et al. Citation1993), stoats (Mustela erminea), feral cats (Felis catus) (Ragg et al. Citation1995), hedgehogs (Erinaceus europaeus) (Lugton et al. Citation1995), a wild rabbit (Oryctolagus cuniculus) (Gill and Jackson Citation1993) and hares (Lepus europaeus) (Coleman and Cooke Citation2001). Originally, wild deer were considered a possible TB maintenance host (Morris and Pfeiffer Citation1995), but possums with TB were eventually shown to be the source of most infection in wild deer (Lugton et al. Citation1998; Nugent Citation2005). Feral pigs, ferrets, stoats, hedgehogs and feral cats can all become infected from scavenging tuberculous wildlife carcasses (). These scavenger species are all considered to be spillover hosts under the conditions and at the densities at which they usually occur in the wild in New Zealand (Nugent Citation2011). Ferrets may also act as a maintenance host in areas where they are at high densities (Caley and Hone Citation2004). Wild deer are also considered spillover hosts at the low densities (mostly <5/km2) found in New Zealand (Nugent and Fraser Citation1993).
Figure 2. Direct and indirect pathways for spread of bovine tuberculosis between and within species in New Zealand. Bold arrows indicate a main source or route of infection; brown depicts direct transmission, green depicts indirect transmission via scavenging or investigation of tuberculous carcasses and offal, and red indicates that the source of infection is unknown but likely to be by direct means.
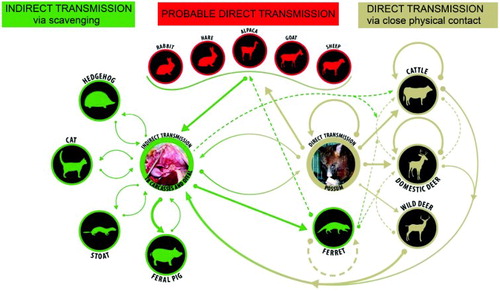
In New Zealand, any wildlife species that is a direct source of TB for cattle or farmed deer is termed a TB vector. Possums are undoubtedly the most important TB vector, but ferrets have also been implicated as vectors of infection for cattle and farmed deer (Boyd Citation1996), and possibly other domestic species. There may be rare instances when other wildlife species fulfil the role of a TB vector.
The discovery of TB in possums in 1967 also coincided with very active periods of commercial harvesting of wild deer (Nugent and Fraser Citation1993) and possum trapping for fur (Clout and Barlow Citation1982). Unwanted possum carcasses left after skinning, together with discarded deer heads and offal, would have provided further sources of tuberculous carrion, not only for possums (Davidson Citation1976), but also for scavenging feral pigs, ferrets and stoats and cats.
Once TB became established in a few localised possum populations, the scene was set for a cycle of infection as shown in , involving transmission from possum to possum, from possums to cattle, as well as to domestic and wild deer. Dead possums with TB provide a source of infection for scavenger species and potentially for cattle and deer investigating carcasses with open lesions. Evidence from DNA typing studies of M. bovis using restriction endonuclease analysis supports this cycle of infection between different hosts. The same DNA type was found in domestic animals and wildlife from the same area (Collins et al. Citation1988; de Lisle et al. Citation1995).
The increasing number of locations at which TB was found in possums indicated that infection was spreading within the wildlife population through extensive, rugged and forested terrain as displayed in and . Tuberculous possums then became a source of infection for cattle at these new locations. While most juvenile possums remain within 2 km of their natal home range, between 20% and 30% disperse up to 5 km, and there is a record of a juvenile migrating 41 km from its natal site (Cowan and Clout Citation2000; Cowan Citation2001). Although most dispersal is by juveniles, adults also sometimes shift home ranges. Tuberculosis was therefore likely to have been transferred from one location to another through a series of migrations by infected possums (Pfeiffer et al. Citation1995), whereby infected migrants have established infection in a new local possum population, which then triggered further waves of expanding infection. Additionally, or alternatively, infection may also have been spread through a series of spillover-spillback transmission events involving possums, wild deer, feral pigs and then back to possums as shown in .
Figure 3. Locations, estimated area in hectares and dates when possums with tuberculosis were first found in New Zealand, until 1980 (from Anonymous Citation1976, Citation1978; Adlam Citation1977; Batcheler and Cowan Citation1988), together with the type of possum control undertaken during the period 1972–1980. Information collated by PG Livingstone from a range of internal MAF reports. The information contained in this Figure uses GIS mapping and therefore provides an accurate representation of the area where infection was found during the period 1967–1980.
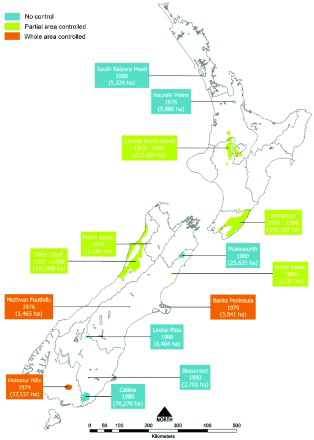
Figure 4. Boundary locations of areas in which tuberculosis (TB) was identified, or was strongly suspected to be present, in possums in New Zealand, showing the maximum size of the areas during 1980 (dark blue), 1994 (green), 2005 (sky blue), and the additional area identified in 2012 (orange). A number of the areas were subsequently declared free of TB or their size was reduced following the eradication of TB from the possum population. These changes are not shown on the map. This Figure uses the 1980 boundary from and then uses additional information compiled by PG Livingstone to define the boundaries for 1994, 2005 and 2012, based on GIS mapping.
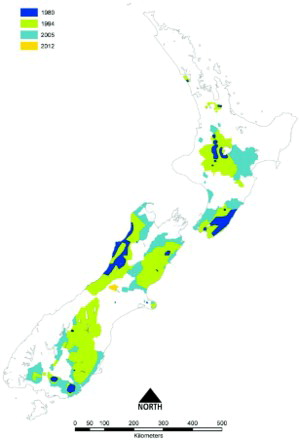
The spread of apparent possum-vectored TB in cattle indicated that most local expansion was through contiguous forest or bush habitats (PG Livingstone, unpublished data). Infection then appeared to spread to farmland from possum habitat along the edges of rivers and watercourses and from bush-pasture margins. At a number of sites where possums were not being controlled in the central North Island and Wairarapa in the mid-1980s, TB appeared to spread through possum populations in habitat adjacent to rivers and streams at between 3 and 5 km per year (Batcheler and Cowan Citation1988).
More broadly, the rate at which TB spread geographically within wildlife populations has been estimated by examining the locations where possums with TB were found after the disease was detected in previously uninfected cattle herds for which livestock infection sources could be considered highly unlikely (). By measuring the straight line distances between the new infected herd/possum location and the closest previously known TB possum location, this analysis suggested that TB was capable of spreading through wildlife populations in extensive forest-covered rugged country at 1.8–2.3 km/year. In less rugged country with a mixture of native and exotic forest, estimated rates were between 1.4 and 4 km/year, while in more open or tussock covered country, they were 2.5–5 km/year. The accuracy of these estimates would be affected by geographic features impacting on the route of travel on the one hand, and by delays in detecting possums with TB on the other.
Table 3. Locations, dates and Euclidean (straight line) distances between a newly located possum infected with tuberculosis (TB) and the closest known possums with TB through contiguous possum habitat, for various locations in New Zealand (unpublished data, PG Livingstone).
Initial impact of TB in wildlife on the TB control programme:
By 1976, 6.5% of the 38,500 cattle herds being tested nationally were classified as infected with M. bovis (Adlam Citation1977). More than half of these infected herds were located in areas where TB was known to be widespread within the possum population, notably around the central North Island, the Wairarapa, and the West Coast of the South Island, despite these areas only accounting for a small proportion of the national herds under test. In addition, isolated pockets of possums with TB had been identified in the Hauraki Plains, Banks Peninsula, Matiri Valley, Methven foothills and the Hokonui Hills in Southland () (Adlam Citation1977).
The wide separation between many of the areas with infected possums indicates TB became established in possum populations as a result of multiple spillover events (Davidson Citation1976) and not just by simple expansion of disease fronts through contiguous wildlife populations. In effect, the possum infection scenario first observed in the Buller district was repeated elsewhere in suitable environments, eventually at possibly up to 50 separate locations (Morris and Pfeiffer Citation1995). It is also notable that in the Northland, Gisborne and Taranaki regions where TB was endemic in cattle herds, there was no reported spread of infection from cattle to wildlife. In these three regions in the 1960s and 1970s there was an absence or rarity of wild deer. Given wild deer are a spillover host, it has been postulated that in some instances, they may have been the crucial conduit by which TB was initially transmitted from livestock to possums (Nugent Citation2011). Their absence in these regions may provide an explanation for the lack of wildlife infection there.
In response to the escalating problem of possums with TB acting as a source of infection for cattle herds, wide-scale possum control programmes were initiated in 1972 (Davidson Citation1991). By 1977, possum control was being undertaken by the New Zealand Forest Service (and Agricultural Pest Destruction Boards (Adlam Citation1977) at a cost of approximately $3 million per year. In those areas where isolated pockets of possums with TB were identified, intensive control was usually undertaken over the whole site. In larger areas, however, limited funding often meant that control was imposed only on affected farmland and adjacent forest margins to protect cattle herds (). Possum control, combined with TB testing of beef and dairy herds, resulted in a halving of reactor rates (the term widely used in New Zealand for the proportion of tested animals with a positive skin-test reaction), from 33.7 per 10,000 cattle tested in the 1975/76 financial year to 14.1 per 10,000 in 1980/81 (). Numbers of infected herds also fell significantly over this period () such that by 1980, the number of infected cattle herds had fallen to 540 with an annual infected herd prevalence (AIHP) of approximately 2%.
Figure 5. Number of cattle tested for tuberculosis that had a positive skin-test reaction (reactors) per 10,000 animals tested between 1975/76 and 1980/81. Information collected by PG Livingstone from various historical documents relating to the then Animal Health Division of the Department of Agriculture.
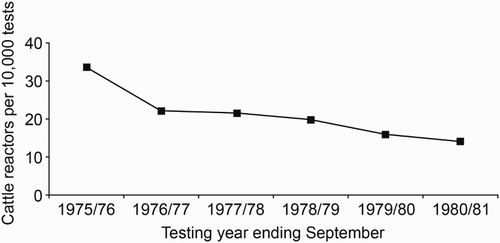
Figure 6. Number of cattle (blue bars) and deer (green bars) herds infected with tuberculosis in New Zealand for the period 1976/77 to 2012/13, with annual expenditure (NZ$ million) on vector control (red line) over the same period. Initially this was for possum control and management, but subsequently increasing amounts were spent on monitoring possum density (from 1998), and wildlife surveillance (from 2006). Figure reproduced from Hutchings et al. (Citation2013), with permission.
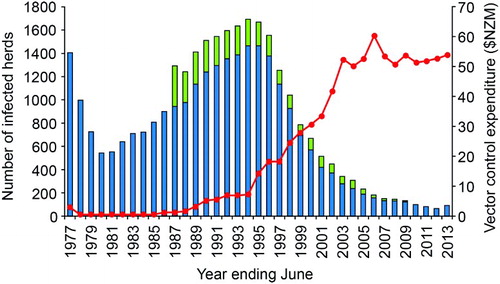
However post 1980 there was a marked increase in the size of areas in which TB had been identified in possums as shown in , indicating that the extent and intensity of control undertaken during the 1970s was insufficient to eradicate infection from possum populations. By 1980, possums with TB had been identified at a further seven separate locations: two in the North Island (south Kaipara and Turangi) and five in the South Island (Molesworth, North Waiau, Lindis Pass, Catlins and Beaumont) as shown in . Infected possum populations were by then estimated to occupy 1.1 million ha, or 4% of New Zealand's land area.
In view of the apparent success of the possum control programme in the mid-1970s, and due to pressure on funds from the Ministry of Agriculture and Fisheries, possum control funding was reduced (Tweddle and Livingstone Citation1994) to approximately $500,000 per year between 1978 and 1984 (PJ O'HaraFootnote3, pers.comm.). Numbers of infected cattle (and later deer) herds subsequently increased relatively rapidly, despite continued intensive TB testing: from 540 in June 1980 to 1,694 in June 1994 (), with an AIHP of 3.8% and an annual infected herd incidence rate of 1.4%. In the 1993/94 financial year, there were 6,328 cattle reactors, with 52% having typical gross lesions of TB at slaughter, and 1,907 deer reactors with 12% having typical TB lesions at slaughter.
In response to the three-fold increase in numbers of infected herds and TB reactors, government funding for possum control was gradually restored from 1984, reaching $3 million by 1989 (Anonymous Citation1989; O'Neil and Pharo Citation1995) and $7.3 million by 1993/94 (). During the period 1987–1990, most of the renewed possum control effort was targeted at stopping the northern and westward spread of possums with TB from the central North Island, by means of a low possum-density “buffer” 150 km long by 3–8 km wide stretching from the Whanganui River in the south-west to the Waikato River in the north-east. This proved to be largely successful in protecting the important dairying areas of the Waikato, but the high cost of the buffer resulted in there being little funding available for controlling possums with TB in other areas. By 1994, the total area where possums were considered to be TB maintenance hosts had expanded to 7.13 million ha (). Much of this increase reflected both a genuine expansion of the area occupied by possums with TB, and also a greater awareness, investigation and reporting of TB, resulting in discovery of infection in new areas where it had been present but undetected before 1980. Further foci of previously undetected tuberculous possums were often revealed through investigation of intransigent M. bovis infection in cattle herds.
Involvement of other domestic or semi-domestic species
Tuberculosis was identified in domestic sheep on the West Coast of the South Island in 1970 (Davidson et al. Citation1981) and in the central North Island in 1975 (Cordes et al. Citation1981). In both cases, the local possum population was heavily infected with TB. In 1986, a survey identified that 7.2% of feral goats in the West Coast region had TB (Sanson Citation1988). While there have been other occasional, isolated findings of TB in sheep and goats, these species are not considered to play any significant role in the TB problem, other than as spillover hosts.
The rise of deer farming, on the other hand, became a significant factor in the spread and control of bovine TB. In 1978, TB was identified for the first time in farmed deer in the West Coast region (Beatson and Hutton Citation1981). At that time, deer farming was a new and rapidly growing venture, largely based on live capture of wild deer. These deer were sometimes captured from areas which were later shown to have TB infection in possums. Capture of infected deer thus gave rise to serious infection levels in some of the new deer herds in both islands, leading to voluntary TB testing of deer herds from 1978, which only became compulsory in 1990. These observations support the hypothesis that despite being spillover hosts at the low densities at which they occur in the wild in New Zealand, deer can also be a maintenance host for TB when farmed at high densities of hundreds per km2. The strong demand for deer meant that both captured and farm-bred deer were transported over large distances, often without effective TB control precautions. This led to an increase in the number of infected deer herds throughout the country. Of more importance strategically, was that TB farmed deer were considered to be the source of infection for uninfected possum populations in at least four localities; Te Puna (O'Neil Citation1990), Fortification (Livingstone Citation1990), Mackenzie Basin (de Lisle et al. Citation1995) and Tikokino/Waipa (Mackereth Citation1993), between 1980 and 1989. This also supports the hypothesis that wild deer with TB may have been a source of infection for possums in some localities. Compulsory TB testing for deer helped prevent further such events (Tweddle and Livingstone Citation1994; Davidson Citation2002).
In 2000, TB was identified in a domestic alpaca (Vicugna pacos; Anonymous Citation2000), but subsequently has been only rarely identified in this host.
TB control programme 1995–2013
Advent of National Pest Management Strategy
By 1994, concerns about the rapid expansion in the total area affected by possums with TB and increasing levels of infection in livestock, despite increasing possum control, prompted more concerted action. The Biosecurity Act 1993 provided a new mechanism for the establishment of a National Pest Management Strategy for bovine TB control.
The strategy introduced a simple herd and area status classification (Livingstone et al. Citation2004), together with movement control requirements for cattle and deer in defined areas. Beef and dairy farmers contributed funding to the strategy via levies on production, which paid for all cattle testing and compensation for TB reactor cattle slaughtered, the latter being limited to 65% of their market value (Anonymous Citation1995). In contrast, in return for lower industry levies, deer farmers elected to meet their own testing costs directly and to receive only carcass proceeds from slaughtered TB reactor deer (Anonymous Citation2001).
As an aid to planning for control of possums and other wildlife TB vectors, New Zealand was classified into TB Vector Risk Areas (VRA) and TB Vector Free Areas (VFA). A VRA was defined as a geographical area in which TB had either been identified in a wildlife maintenance host (possums), or was strongly suspected to be present in a wildlife maintenance host based on epidemiological findings from infected cattle and deer herds. Defining the presence of a wildlife maintenance host and determining the boundaries of a VRA often began by finding infection in cattle or farmed deer for which there was no logical epidemiological explanation (Tweddle and Livingstone Citation1994). As part of the epidemiological investigation into the cause of the infection, wild animals may be sampled from the property contiguous with the newly infected herd as well as adjacent areas of land. Sometimes TB was found in wildlife via passive surveillance that included nationwide post-mortem examination of wild deer and pigs commercially hunted and sold to game packing houses, and ad-hoc reporting by possum trappers. The finding of TB in spillover hosts or in specific cohorts of cattle or farmed deer can be used to better define areas to enable intensive sampling of possums for necropsy and where necessary, laboratory examination. When TB is found in possums within a specified location, then the extent of TB possum infection needs to be defined. Tuberculin testing of all herds in the locality, together with sampling of scavenger species present in the wider area provides a means of identifying areas for further intensive possum surveillance (Livingstone Citation1990; Ryan et al. Citation2006). Defining the boundaries of the new VRA depend upon a number of factors including: i) locations of known possums with TB as well as other TB wild and domestic animals species within the general area, ii) noting areas where the status of wildlife is still unknown, iii) taking account of potential possum and other wild animal movements, iv) geographical features that may restrict possum movements. The remainder of the country where TB maintenance hosts are not present or suspected is classed as VFA. (Livingstone et al. Citation2004)
The main objectives of the National Pest Management Strategy (within 5 years) were to: i) reduce the AIHP in VFA from 0.7–0.2%; ii) to reduce the AIHP in VRA from 17–11%; and iii) to prevent the expansion of VRA (Anonymous Citation1995). The thrust of the strategy was thus to prevent the spread of TB to possums and other wildlife outside VRA boundaries, and to control possums within VRA in ways that reduced the likelihood of transmission of M. bovis infection from possums to cattle and deer herds. The strategy also identified the three critical components for success in the New Zealand TB control programme as: TB test and slaughter of reactors, control of movement of cattle and deer from infected herds as well as declared areas where the AIHP rate was ≥1%, and reduction and maintenance of the possum population in VRA at low densities.
The herd and area classifications of the strategy, coupled with the introduction of area movement control and setting of reactor compensation, were quickly assimilated by farmers, as they helped to clarify herd TB testing requirements and provided incentives to better manage TB risk in livestock management, movement and purchasing decisions. New strategy funding agreements saw funds available for controlling TB vectors doubling to $14 million in 1995, with further increases to $33 million in 2001 ().
The delineation of VRA enabled possum control to be targeted at boundaries to contain the spread of possums with TB into adjacent VFA. The balance of possum control funding was prioritised to areas with the highest numbers of vector-related herd infections.
Initial research support
Steps towards a research framework to support the National Pest Management Strategy had begun as early as 1989 when the AHB developed a portfolio of TB and possum-related research to address New Zealand's escalating TB problems. This portfolio included short-term research into the epidemiology of TB in possum and other wildlife populations, improved means of controlling possums, and improvements in diagnostic testing for cattle and deer. It also included medium-term research on the development and evaluation of various vaccines, including BCG-based vaccines for cattle, farmed deer and possums, as well as long-term research focused on biological control of possums (Anonymous Citation1995).
This portfolio formed the basis of a research programme overseen by the National Science Strategy Committee for Possums and TB Control, under the auspices of the Ministry of Research, Science and Technology (Atkinson and Wright Citation1993). The AHB took responsibility for funding short-term, applied research projects while the Ministry of Agriculture and Fisheries and the Foundation for Research, Science and Technology funded more of the medium- and long-term research.
The research achieved a number of important outcomes, including the following. Approval of the γ-interferon test for ancillary serial and parallel diagnostic tests on cattle (Ryan et al. Citation2000) addressed issues of false positive and false-negative reactivity to the skin test. Identification that the human vaccine BCG was protective for farmed deer and cattle against TB (Buddle et al. Citation2006; Griffin et al. Citation2006) potentially offered another avenue for controlling TB in cattle and deer herds should possum control fail. A practical means of indexing possum density (Warburton Citation1996) provided a method for measuring possum control effectiveness, enabled control objectives to be set and facilitated the introduction of commercial, performance-based possum control contracts. Clarification of the epidemiology of TB in possum populations and their role in spreading infection to cattle and deer (Morris and Pfeiffer Citation1995; Paterson and Morris Citation1995; Sauter and Morris Citation1995) provided for better planning and targeting of possum control. In addition, there was on-going enhancement of knowledge on the host status of possums (Caley et al. Citation1999); ferrets (Caley et al. Citation2001; Caley and Hone Citation2005), and wild deer (Lugton et al. Citation1998), and the identification that ferrets were probably a TB vector for cattle and farmed deer (Livingstone Citation1996; Ragg et al. Citation2000). Continued development of possum-TB models (Barlow Citation2000) assisted in determining population density levels required to eradicate TB from possum populations, and formed a basis for newer disease eradication models.
Expansion of the National Pest Management Strategy 2002–2011
Implementing the findings from this early research, together with a range of managerial and strategic programme improvements, provided the AHB with confidence to initiate major up-scaling of the National Pest Management Strategy in March 1999. By then, the objective of reducing the AIHP of infected herds in VRA to 10% had been met, but the expansion of possums with TB from VRA had continued and the AIHP within VFA was 0.5% (compared with the 0.2% objective), although cattle TB reactor numbers had usefully fallen by 50% nationwide. The objective initially proposed for the revised strategy was to reduce the AIHP in New Zealand to 0.2% within a 10-year time frame. Following discussion with stakeholders, iterative analysis of policy issues, and debate over the time frame, a proposal to amend the strategy was developed and submitted to the Minister of Biosecurity in May 2001 (Anonymous Citation2001). The key objective was to achieve the 0.2% AIHP by June 2013.
Although formal approval of this amendment was delayed until 2004 (to enable resolution of funding share issues) increased vector control funding to more than $50 million per year became available from industry and central and regional government from 2002/03, enabling the main vector control components of the strategy to be introduced. The priorities for vector control funding were: containment of wildlife infection by establishing and maintaining very low possum densities around the boundaries of VRA to prevent possums with TB spreading; reduction and maintenance of possum populations at very low densities on the majority of farmland and adjacent forest areas within VRA to limit herd infection; and local eradication of TB from possum populations where this was both achievable and likely to be more cost-effective in the long-term than simply suppressing TB in possums to low levels (Livingstone et al. Citation2004).
Implementation of the expanded strategy saw the number of TB reactors and infected herds fall () more rapidly than forecast. shows the expansion in boundaries and increase in number of VRA that occurred from 1980–2012. The expansion peaked at 10.5 million ha or 39% of New Zealand's land area in 2004. Most of the increase in area identified between 1994 and 2004 occurred due to expansion of the boundaries of all the major VRA. During the period 1994–2000, wildlife with TB were identified in 10 new discrete VRA: at Opuatai, Maungatautari, East Whareorino, Mangaporau, Papaiti and Coastal Manawatu/Whanganui in the North Island; and at Springfield, Tai Tapu, Roxburgh and the Blue Mountains in the South Island. In some of the smallest VRA such as Opuatai, Springfield and Tai Tapu, maintaining possum densities at low levels for 5–7 years was apparently sufficient to eradicate TB from the possum populations, with no subsequent recurrence of TB infection in livestock (Anonymous Citation2009).
No further expansion of VRA occurred from June 2004 until July 2011, when possums and feral pigs with TB were identified in the Rolleston Range in Canterbury (Anonymous Citation2013). Following further investigations to define the extent of the wild animal infection, the Rolleston Range was formally recognised as a new VRA in July 2012 (). The source of infection for this new VRA is unknown, but was most probably either infected wild animals migrating across the Southern Alps via a low pass from the West Coast, or liberation of infected wild pigs or deer from the West Coast, and/or disposal of their heads and carcasses, into the catchments of the Rolleston Range.
Over an 18-year period, spanning 1993/94 to 2011/12, the AHB identified and recorded herd breakdowns (new herd infections) each year (data from a National Livestock Database and later a Disease Management Information System, TBfree New Zealand). During this period, as areas were more accurately classified as either VRA or VFA, the attributable fraction of herd breakdowns associated with herds located in VRA increased, and there was a parallel increase in the population attributable fraction, i.e. that component of the overall disease risk directly attributable to a defined single factor (Rockhill et al. Citation1998). In this case, the population attributable fraction indicated that breakdowns occurring in the VRA were as a consequence of a source of infection that was only found within VRA. Thus in 1993/94, 88% of herd breakdowns occurred in the areas designated as VRA at that time, but only 55% of the breakdowns could be ascribed to a source located within a VRA, largely as a result of there being substantial areas containing TB wildlife that had not yet been identified and categorised as VRA. In 2000/01, the corresponding figures were 91 (95%CI=88–93)% and 64%, respectively. By 2005/06, when most, if not all areas with TB established in wildlife had been categorised correctly as VRA, 95 (95%CI=92–97)% of herd breakdowns occurred in VRA, with 80% of these ascribed to a source within the VRA, such as TB infected wildlife. By 2011/12, 98 (95%CI=94–99)% of herd breakdowns occurred in VRA, and the population attributable fraction indicated that 91% of these could be ascribed to a source within the VRA. The only difference between VRA and VFA was the respective presence or absence of TB in wildlife.
Continued research support
During the period 2002–2011, a substantial programme of research and epidemiological predictive modelling of disease was funded by both the AHB and Foundation for Research, Science and Technology. Some of the main outcomes included the following. Increased clarity around the spillover host status of wild deer (Nugent Citation2005) and feral pigs (Nugent et al. Citation2012b) demonstrated that large-scale possum control resulted in TB levels in wild deer and pigs declining toward zero, strongly indicating that TB levels in possums must also have declined to very low levels. Feral pigs were identified as being particularly sensitive sentinels for detecting TB in possums (Nugent et al. Citation2002), with a lower relative utility of ferrets and wild deer in this role (Nugent and Whitford Citation2008). Continued work on behaviour and ecology of possums at low densities to assist with cost-effective maintenance of low possum densities (Nugent et al. Citation2015). Further development and refinement of TB-possum models for predicting the levels of possum control required to eradicate TB from possums (Ramsey and Efford Citation2005) and the outcomes of other potential management scenarios (Ramsey and Efford Citation2010). Development of a “Proof of Freedom” framework combining prediction from the TB-possum model above and Bayesian modelling of multiple sources of TB surveillance data for estimating the probability that TB has been eradicated from a defined possum population (Anderson et al. Citation2013).
Disease control programmes were also augmented by key technological developments over the same period. These included the following. Development of an oral bait BCG vaccine for possums (Aldwell et al. Citation2003) which had an efficacy of >80% in a free-living infected possum population in which possums were captured for vaccination (Tompkins et al. Citation2009). The oral bait BCG vaccine was developed as insurance in case other control methods became unavailable or failed to achieve the required reduction in possum densities to eradicate TB. Free-choice oral vaccination of wild possums has yet to be proven to be operationally effective. However, the expected higher cost of vaccine baits relative to toxic baits and the regular recruitment of unvaccinated young is likely to make oral vaccination less cost-effective than lethal control. Development and implementation of a γ-interferon serial test using defined antigens for cattle where a high specificity was required (Buddle et al. Citation2003), leading to further reduction in slaughter of false positives to the intradermal caudal fold tuberculin test. Development and implementation of variable number of tandem repeats as a new DNA strain typing method for M. bovis (Price-Carter et al. Citation2011), which provided a test that was technically easier to perform and read than the restriction endonuclease analysis (Collins Citation2011) typing method. Implementation of a DNA amplification test for detecting TB in cattle and deer lesions (Bean et al. Citation2007), which enabled lesions caused by infection with M. avium subspecies paratuberculosis to be readily separated from M. bovis lesions in deer.
Research also continued into environmental, non-target, and human health effects of using sodium fluoroacetate (1080) as a possum toxin (Eason et al. Citation2011). This helped address actual and publically perceived risks of large-scale use of 1080 and supported a major reassessment of the toxin by New Zealand's Environmental Risk Management Authority. As a result of this reassessment process, 1080 was approved for continued use in aerial application for controlling possums (Anonymous Citation2007). This research also contributed to the strong support for continued use of aerial 1080 from the Parliamentary Commissioner for the Environment (Wright Citation2011). Other related work included the evaluation of a deer repellent for use on 1080 baits to reduce incidental deer mortality (Speedy Citation2005), and investigation of lower aerial application rates of baits to maintain cost-effective control (Nugent et al. Citation2011, Citation2012a). In addition, research continued to identify and evaluate new toxins or toxin formulations for controlling possums (Eason et al. Citation2010).
This broad body of research, coupled with continuous improvement in operational efficiency and uniformity of control coverage, indicated that possum densities could be reduced to near zero over very large areas at an affordable cost, using a combination of ground-based control on farmland and aerial 1080 baiting of adjacent extensive forests. The epidemiological research and associated modelling indicated that there was an extremely low likelihood of TB persisting for more than 7 years at such low possum densities (Nugent et al. Citation2015). Combined with increased confidence that the key tools needed to attain those low densities would continue to be available for at least the medium term, and the development of a suite of fall-back possum control alternatives, there was increased conviction that regional or even national scale eradication of TB from both wildlife and domestic livestock was feasible.
The next stage
By 2005, the reduction in the number of infected herds was exceeding forecast expectations. In 2006, following a major technical assessment, the AHB began a second review of the National Pest Management Strategy with a working objective of eradicating TB from wildlife. By then, TB had been eradicated from possum populations in 10 small VRA with a combined area of 115,000 ha. As in the first review, there were several years of extensive discussion with stakeholders, along with detailed analysis of policy issues and the development and costing of alternative TB management or eradication scenarios. Eventually, a proposal to amend the strategy was developed and submitted to the Minister of Agriculture in September 2009 (Anonymous Citation2009). The key new objective of the third strategy was to eradicate TB from possums and other wildlife across 2.5 million ha (25% of the VRA) by July 2026.
Towards a new paradigm: eradication of TB in wildlife
By December 2011, a combination of livestock test and slaughter programmes, risk-based movement control and extensive possum control as well as wildlife surveillance had reduced the AIHP to ≤0.2%, some 18 months ahead of the forecast date. For the 2011/12 financial year, there were 722 cattle and 250 deer TB reactors, with 16% and 4% confirmed tuberculous respectively (Anonymous Citation2012). At June 2012, there were 66 infected cattle and deer herds () and the AIHP was 0.18%. Further, between 1 July 2011 and 30 June 2013, eradication of TB from wildlife was considered to have been achieved across a total of approximately 500,000 ha (Anonymous Citation2012, Citation2013), and the VRA status for those management areas was revoked. Thus at 30 June 2013, there were 18 VRA with a combined area of 9.8 million ha (Anonymous Citation2013).
However, the number of infected herds and the AIHP increased to 92 and 0.21% respectively in 2012/13, but close investigation revealed livestock movement and the establishment of new dairy herds as the main driver of this increase. While this confirmed the continuing disease risks posed by movement of cattle, application of effective test and slaughter regimes were expected to clear infection from the majority of these herds within 18 months. Had infection been vector-related, then eradication of infection from both the cattle herds and the adjacent possum populations would have been a much more costly and time consuming process. Containment and eventual eradication of TB in possums is thus both the priority and the challenge for New Zealand's TB control programme, notwithstanding continuing occasional livestock movement-related outbreaks in cattle herds.
It is instructive to compare New Zealand's achievements with those of England which has similar livestock TB testing, slaughter and movement control policies applied under high levels of expertise. The key difference is that culling of the Eurasian badger (Meles meles), England's equivalent TB wildlife reservoir and main TB vector, was only undertaken on a reactive basis using variable methods between 1974 and 1997. There has been no badger control since then apart from a large-scale trial between 1998 and 2005, which had some implementation problems (Donnelly et al. Citation2003). Over the last 20 years most metrics of cattle TB in England have increased, some of them in an exponential fashion. In the 2013 calendar year, England had over 26,000 TB reactors slaughtered. Further, the “…rolling average proportion of live cattle herds under restriction as a result of culture and/or lesion confirmed positive bTB breakdowns rose from just under one percent in 2000 to just over five percent in 2012.” (Anonymous Citation2014). The area considered affected by tuberculous badgers has expanded from a few isolated foci in the southwest counties of Cornwall and Gloucestershire in the mid-1970s, to an area covering about 30% of England's land mass in 2013 (R. de la Rua DomenechFootnote4, pers. comm.). Given the similar livestock TB control measures that have been instituted in both countries, this comparison shows that, without effective possum control, New Zealand could be facing a similar situation to that of England, with numbers of infected herds in the thousands, tens of thousands of TB reactors, with herds over more than half of New Zealand's land area being prone to wildlife infection.
In summary, the history of TB management in New Zealand initially paralleled that for most other developed countries, moving progressively to more and more stringent test-and-slaughter programmes during the mid-twentieth century. However progress toward TB freedom in livestock was slowed and then halted by the emergence of the problem of TB in wildlife in the 1960s, in contrast to countries free of TB in wildlife. Although some other countries have also suffered from this problem, the central role of the possum is unique to New Zealand. Once that role was recognised, effective techniques for controlling TB via lethal control of possums were developed. When these were then applied in the context of a comprehensive and adequately funded control programme, progress toward TB freedom in New Zealand subsequently resumed. This programme was largely responsible for achieving a >95% reduction in the number of infected cattle and deer herds over the last two decades. The proven effectiveness of the control systems and tools now available and being used, provides confidence that wide-scale eradication of TB from wildlife populations is a realistic prospect, albeit currently at high cost. New Zealand is therefore potentially able to follow Australia as the first country to eradicate TB despite its presence in wildlife.
AHB | = | Animal Health Board |
AIHP | = | Annual infected herd prevalence |
TB | = | Tuberculosis |
VFA | = | Vector free areas |
VRA | = | Vector risk areas |
Acknowledgements
The authors would like to express their appreciation to the following people who have contributed without authorship. Kelly Beuth, Frank Cross, John Edington, Royce Elliott, Campbell Fleury, Lindsay Grueber, Michael Hansen, John Hellstrom, Chris Lester, Peter O'Hara and Terry Ryan, variously provided data or information, data analyses, maps and figures used in this paper. Frank Cross, Michael Davidson, Stu Hutchings, Rosanne Livingstone, William McCook reviewed and commented on early drafts. John Deal and Deirdre Cuff provided assistance with editing and presentation. John Edington and Neil Ranger compiled much of the early West Coast data.
We also would like to express our appreciation to the three reviewers and the New Zealand Veterinary Journal Editorial Board for the positive way that they reviewed our paper and the comments made to improve its scientific rigour and readability. We consider that addressing the concerns identified by the reviewers has made the paper more scientifically robust and readable.
Publication of this paper was financially supported by TBfree New Zealand Ltd.
Notes
1N Beatson, former Ministry of Agriculture's Veterinary Officer for Buller, NZ, 1970–72
2J Edington, AsureQuality veterinarian for the West Coast from 1999–2008.
3PJ O'Hara, former Chief Veterinary Officer of the Ministry of Agriculture and Forestry, Wellington, NZ
4R. de la Rua Domenech, Veterinary Advisor, Defra, London, UK
References
- Adlam GH. Current problems and future developments in disease eradication schemes. New Zealand Veterinary Journal 25, 270–3, 1977
- Aldwell FE, Keen DL, Parlane NA, Skinner MA, de Lisle GW, Buddle BM. Oral vaccination with Mycobacterium bovis BCG in a lipid formulation induces resistance to pulmonary tuberculosis in brushtail possums. Vaccine 22, 70–6, 2003
- Anderson DP, Ramsey DSL, Nugent G, Bosson M, Livingstone P, Martin PAJ, Sergeant E, Gormley AM, Warburton B. A novel approach to assess the probability of disease eradication from a wild-animal-reservoir host. Epidemiology and Infection 141, 1509–21, 2013
- *Anonymous. Department of Agriculture Annual Report. Division of Veterinary Science, Appendix to Journals of House of Representatives. Government Printer, Wellington, New Zealand, 1905
- *Anonymous. Department of Agriculture Annual Report. Division of Veterinary Science, Appendix to Journals of House of Representatives; 1930–1950. Government Printer, Wellington, New Zealand, 1930–1950
- *Anonymous. Department of Agriculture Annual Report. Appendix to Journals of House of Representatives. Government Printer, Wellington, New Zealand, 1955
- *Anonymous. Department of Agriculture Annual Report. Appendix to Journals of House of Representatives. Government Printer, Wellington, New Zealand, 1968
- *Anonymous. Ministry of Agriculture Annual Report. Appendix to Journals of House of Representatives. Government Printer, Wellington, New Zealand. Pp 27–8, 1971
- *Anonymous. Tuberculosis Seminar. Ministry of Agriculture, Government Printer, Wellington, New Zealand, 1976
- *Anonymous. Tuberculosis eradication scheme. Surveillance 5 (2), 12–4, 1978
- *Anonymous. Ministry of Agriculture and Fisheries Annual Report, Appendix to Journals of House of Representatives. Government Printer, Wellington, New Zealand, 1989
- *Anonymous. Proposed National Pest Management Strategy for Bovine Tuberculosis. https://gazette.govt.nz/notice/id/1995-go7564 (accessed 24 September 2014). New Zealand Gazette Office, Wellington, New Zealand, 1995
- *Anonymous. Animal Health Board Annual Report for the year ending 30 June 2000. http://www.tbfree.org.nz/Portals/0/2014AugResearchPapers/AHB%20Annual%20Report%202000.pdf (accessed 20 September 2014). TBfree New Zealand, Wellington, NZ, 2000
- *Anonymous. Bovine Tuberculosis Pest Management Strategy 2001–2013. An amendment proposal prepared by the Animal Health Board Incorporated. http://www.tbfree.org.nz/Portals/0/2014AugResearchPapers/Bovine%20Tuberculosis%20Pest%20Management%20Strategy%202001-2013.pdf (accessed 20 September 2014). TBfree New Zealand, Wellington, NZ, 2001
- *Anonymous. Environmental Risk Management Authority Decision: Application for the Reassessment of a Hazardous Substance under Section 63 of the Hazardous Substances and New Organisms Act 1996. Name of Substance(s): Sodium Fluoroacetate (1080) and Formulated Substances containing 1080. http://www.epa.govt.nz/Publications/1080-Decision-document-with-amendments.pdf (accessed 26 June 2014). Environmental Risk Management Authority, Wellington, NZ, 2007
- *Anonymous. National Bovine Tuberculosis Pest Management Strategy: An amendment proposal prepared by the Animal Health Board Incorporated. http://www.tbfree.org.nz/Portals/0/2014AugResearchPapers/Anonymous.%20National%20Bovine%20Tuberculosis%20Pest%20Management%20Strategy%20-%20Amendment%20Proposal%20Sept%202009.%20Animal%20Health%20Board,%20Wellington,%20New%20Zealand,%202009.pdf (accessed 20 September 2014). TBfree New Zealand, Wellington, NZ, 2009
- *Anonymous. Animal Health Board Annual Report 2011/12. http://www.tbfree.org.nz/Portals/0/Annual%20Report%20web.pdf (accessed 26 June 2014). TBfree New Zealand, Wellington, NZ, 2012
- *Anonymous. Animal Health Board Annual Report 2012/13. http://www.tbfree.org.nz/Portals/0/AHB%20Annual%20Report%20E-book%202012-13final.pdf (accessed 26 June 2014). TBfree New Zealand, Wellington, NZ, 2013
- *Anonymous. The strategy for achieving Officially Bovine Tuberculosis-Free status for England. https://www.gov.uk/government/uploads/system/uploads/attachment_data/file/300447/pb14088-bovine-tb-strategy-140328.pdf (accessed 17 August 2014). Department for Environment, Food and Rural Affairs, London, UK, 2014
- Atkinson PH, Wright DE. The formulation of a national strategy for biological control of possums and bovine Tb. New Zealand Journal of Zoology 20, 325–8, 1993
- Barlow N. Control of endemic bovine Tb in New Zealand possum populations - results from a simple model. The Journal of Applied Ecology 28, 794–809, 1991a
- Barlow N. A spatially aggregated disease/host model for bovine Tb in New Zealand possum populations. The Journal of Applied Ecology, 28, 777–93, 1991b
- *Barlow ND. Models for Possum Management. In: TL Montague (eds). The Brushtail Possum: Biology, Impact and Management of an Introduced Marsupial. Pp 208–19. Manaaki Whenua Press, Lincoln, New Zealand, 2000
- Barron MC, Nugent G, Cross ML. Importance and mitigation of the risk of spillback-transmission of Mycobacterium bovis infection for eradication of bovine tuberculosis from wildlife in New Zealand. Epidemiology and Infection 141, 1394–406, 2013
- *Batcheler CL, Cowan PE. Review of the status of the possum in New Zealand. http://www.landcareresearch.co.nz/__data/assets/pdf_file/0009/78750/Review_Status_Possum_NZ.pdf (accessed 24 September 2014). Landcare Research, Lincoln, NZ, 1988
- Bean DC, Hills A, Ryan TJ, Aitken J. Evaluation of the BD ProbeTec ET system for direct detection of Mycobacterium bovis in veterinary specimens. Journal of Clinical Microbiology 45, 3434–5, 2007
- *Beatson NS, Hutton JB. Tuberculosis in farmed deer in New Zealand. In: Proceedings of a Deer Seminar for Veterinarians. New Zealand Veterinary Association, Wellington, New Zealand. Pp 143–51, 1981
- *Boyd P. Vector control and reactor history at Haldon Station. In: Proceedings of a Workshop organised by the Possum and Bovine Tuberculosis Control National Science Strategy Committee, Landcare Research Ltd, Lincoln. Pp 16–7. http://www.tbfree.org.nz/Portals/0/2014AugResearchPapers/Vector%20control%20and%20reactor%20history%20at%20Haldon%20Station.pdf (accessed 24 September 2014). TBfree New Zealand, Wellington, NZ, 1996
- Brown K, Innes J, Shorten R. Evidence that possums prey on and scavenge bird's eggs, birds and mammals. Notornis 40, 169–77, 1993
- Buddle BM, McCarthy AR, Ryan TJ, Pollock JM, Vordermeier HM, Hewinson RG, Andersen P, de Lisle GW. Use of mycobacterial peptides and recombinant proteins for the diagnosis of bovine tuberculosis in skin test-positive cattle. Veterinary Record 153, 615–20, 2003
- Buddle BM, Wedlock DN, Denis M. Progress in the development of tuberculosis vaccines for cattle and wildlife. Veterinary Microbiology 112, 191–200, 2006
- Caley P, Hone J. Disease transmission between and within species, and the implications for disease control. Journal of Applied Ecology 41, 94–104, 2004
- Caley P, Hone J. Assessing the host disease status of wildlife and the implications for disease control: Mycobacterium bovis infection in feral ferrets. Journal of Applied Ecology 42, 708–19, 2005
- Caley P, Hickling G, Cowan P, Pfeiffer D. Effects of sustained control of brushtail possums on levels of Mycobacterium bovis infection in cattle and brushtail possum populations from Hohotaka, New Zealand. New Zealand Veterinary Journal 47, 133–42, 1999
- Caley P, Hone J, Cowan PE. The relationship between prevalence of Mycobacterium bovis infection in feral ferrets and possum abundance. New Zealand Veterinary Journal 49, 195–200, 2001
- Clout MN, Barlow ND. Exploitation of brushtail possum populations in theory and practice. New Zealand Journal of Ecology 5, 29–35, 1982
- *Clout MN, Ericksen K. Anatomy of a disastrous success: the brushtail possum as an invasive species. In: TL Montague (ed). The Brushtail Possum: Biology, Impact and Management of an Introduced Marsupial. Pp 1–9. Manaaki Whenua Press, Lincoln, New Zealand, 2000
- Coleman JD. Distribution, prevalence, and epidemiology of bovine tuberculosis in brushtail possums, Trichosurus vulpecula, in the Hohunu Range, New Zealand. Journal of Australian Wildlife Research 15, 651–63, 1988
- Coleman JD, Cooke MM. Mycobacterium bovis infection in wildlife in New Zealand. Tuberculosis 81, 191–202, 2001
- Coleman JD, Cooke MM, Jackson R, Webster R. Temporal patterns in bovine tuberculosis in a brushtail possum population contiguous with infected cattle in the Ahaura valley Westland. New Zealand Veterinary Journal 47, 119–24, 1999
- Collins DM. Advances in molecular diagnostics for Mycobacterium bovis. Veterinary Microbiology 151, 2–7, 2011
- Collins DM, de Lisle GW, Gabric DM. Geographic distribution of restriction types of Mycobacterium bovis isolates from brush-tailed possums (Trichosurus vulpecula) in New Zealand. Journal of Hygiene (London) 96, 431–8, 1986
- Collins DM, Gabric DM, de Lisle GW. Typing of Mycobacterium bovis isolates from cattle and other animals in the same location. New Zealand Veterinary Journal 36, 45–6, 1988
- Cordes DO, Bullians JA, Lake DE, Carter ME. Observations on tuberculosis caused by Mycobacterium bovis in sheep. New Zealand Veterinary Journal 29, 60–2, 1981
- Cousins DV, Roberts JL. Australia's campaign to eradicate bovine tuberculosis: the battle for freedom and beyond. Tuberculosis 81, 5–15, 2001
- Cowan PE. Advances in New Zealand mammalogy 1990–2000: Brushtail possum. Journal of The Royal Society of New Zealand 31, 15–29, 2001
- *Cowan PE, Clout MN. Possums on the move: activity patterns, home ranges and dispersal. In: TL Montague (ed). The Brushtail Possum: Biology, Impact and Management of an Introduced Marsupial. Pp 24–34. Manaaki Whenua Press, Lincoln, New Zealand, 2000
- Davidson RM. The role of the Opossum in spreading tuberculosis. New Zealand Journal of Agriculture 133, 21–5, 1976
- *Davidson RM. Tuberculosis in possums. Surveillance 18 (5), 16, 1991
- Davidson RM. Control and eradication of animal diseases in New Zealand. New Zealand Veterinary Journal 50 (Suppl. 3), 6–12, 2002
- Davidson RM, Alley MR, Beatson NS. Tuberculosis in a flock of sheep. New Zealand Veterinary Journal 29, 1–2, 1981
- de Lisle GW, Crews K, de Zwart J, Jackson R, Knowles GJE, Paterson KD, Mackenzie RW, Waldrup KA, Walker R. Mycobacterium bovis infections in wild ferrets. New Zealand Veterinary Journal 41, 148–9, 1993
- de Lisle GW, Yates GF, Collins DM, MacKenzie RW, Crews KB, Walker R. A study of bovine tuberculosis in domestic animals and wildlife in the MacKenzie Basin and surrounding areas using DNA fingerprinting. New Zealand Veterinary Journal 43, 266–71, 1995
- Donnelly CA, Woodroffe R, Cox DR, Bourne J, Gettinby G, Le Fevre AM, McInerney JP, Morrison WI. Impact of localised badger culling on tuberculosis in British cattle. Nature 426, 834–7, 2003
- Eason CT, Fagerstone KA, Eisemann JD, Humphrys JR, Lapidge SJ. A review of existing and potential New World and Australasian vertebrate pesticides with a rationale for linking use patterns to registration requirements. International Journal of Pest Management 56, 109–25, 2010
- Eason CT, Miller A, Ogilvie S, Fairweather A. An updated review of the Toxicology and Ecotoxicology of 1080 in relation to its use as a pest control tool in New Zealand. New Zealand Journal of Ecology 35, 1–20, 2011
- Ekdahl MO, Smith BL, Money DFL. Tuberculosis in some wild animals in New Zealand. New Zealand Veterinary Journal 18, 44–5, 1970
- Essey MA, Koller MA. Status of bovine tuberculosis in North America. Veterinary Microbiology 40, 15–22, 1994
- *Francis J. Bovine Tuberculosis Including a Contrast with Human Tuberculosis. P 220, Staples Press Limited, London, UK, 1947
- Francis J. The work of the British Commission on Tuberculosis, 1901–1911. Tubercle 40, 124–32, 1959
- Gill JW, Jackson R. Tuberculosis in a rabbit: a case revisited. New Zealand Veterinary Journal 41, 147, 1993
- Griffin JFT, Mackintosh CG, Rodgers CR. Factors influencing the protective efficacy of a BCG homologous prime-boost vaccination regime against tuberculosis. Vaccine 24, 835–45, 2006
- Hopkirk CSM. Livestock of New Zealand with Special Reference to Disease Past and Present. Reprinted July 1935, of the Report of the Melbourne (1935) meeting of the Australian and New Zealand Association for the Advancement of Science. Section L- Veterinary Science, P 340, 1935
- Hutchings SA, Hancox N, Livingstone PG. A strategic approach to eradication of bovine TB in wildlife in New Zealand. Transboundary and Emerging Disease 60 (Suppl. 1), 85–91, 2013
- *Jackson R. Transmission of tuberculosis (Mycobacterium bovis) by possums. PhD Thesis, Massey University, Palmerston North, NZ. Pp 241 & 251, 1995
- Jackson R. The role of wildlife in Mycobacterium bovis infection of livestock in New Zealand. New Zealand Veterinary Journal 50, 49–52, 2002
- Laing ADMG. The history and progress of the town milk supply tuberculin testing scheme. New Zealand Veterinary Journal 3, 138–43, 1955
- Laing ADMG. Some historical notes on the veterinary profession in New Zealand: Part 1. New Zealand Veterinary Journal 12, 67–71, 1964
- Laing ADMG. The history of meat hygiene and inspection in New Zealand up to the formation of the meat division of 1963. New Zealand Veterinary Journal 18, 241–3, 1970
- *Lehane R. Beating the odds in a big country: the eradication of bovine brucellosis and tuberculosis in Australia. Pp 24––8. CSIRO Publishing, Victoria, Australia, 1996
- *Livingstone PG. Epidemiology of tuberculosis: a national perspective. Proceedings of a Deer Course for Veterinarians, No. 7, Deer Branch of the New Zealand Veterinary Association. Pp 8–17, 1990
- *Livingstone PG. Overview of the ferret problem. In: Proceedings of a Workshop Organised by the Possum and Bovine Tuberculosis Control National Science Strategy Committee, Landcare Research Ltd, Lincoln. Pp 2–6 http://www.tbfree.org.nz/Portals/0/2014AugResearchPapers/Livingstone%20PG.%20Overview%20of%20the%20ferret%20problem.%20In%20Proceedings%20of%20a%20Workshop%20Organised%20by%20the%20Possum%20and%20Bovine%20Tuberculosis%20Control%20National%20Science%20Strategy%20Committee.pdf (accessed 24 September 2014) TBfree New Zealand, Wellington, NZ, 1996
- *Livingstone PG, Ryan TJ, Hancox N. Deer TB control in New Zealand. Proceedings of the First World Deer Veterinary Congress, No. 21, Deer Branch of the New Zealand Veterinary Association. Pp 98–101, 2004
- Lugton IW, Johnstone AC, Morris RS. Mycobacterium bovis infection in New Zealand hedgehogs (Erinaceus europaeus). New Zealand Veterinary Journal 43, 342–5, 1995
- Lugton IW, Wilson PR, Morris RS, Nugent G. Epidemiology and pathogenesis of Mycobacterium bovis infection of red deer (Cervus elaphus) in New Zealand. New Zealand Veterinary Journal 46, 147–56, 1998
- *Mackereth G. The Waipa endemic area: The epidemiological picture. Proceedings of a Deer Course for Veterinarians, No. 10, Deer Branch of the New Zealand Veterinary Association. Pp 222–8, 1993
- *Montague TL. The Brushtail Possum: Biology, Impact and Management of an Introduced Marsupial. Manaaki Whenua Press, Lincoln, New Zealand, 2000
- Morris RS, Pfeiffer DU. Directions and issues in bovine tuberculosis epidemiology and control in New Zealand. New Zealand Veterinary Journal 43, 256–65, 1995
- *Nugent G. The role of wild deer in the epidemiology and management of bovine tuberculosis in New Zealand. PhD Thesis, Lincoln University, Lincoln, NZ, 2005
- Nugent G. Maintenance, spillover and spillback transmission of bovine tuberculosis in multi-host wildlife complexes: A New Zealand case study. Veterinary Microbiology 151, 34–42, 2011
- Nugent G, Fraser W. Pests or valued resources? Conflicts in management of deer. New Zealand Journal of Zoology 20, 361–6, 1993
- *Nugent G, Whitford J. Animal Health Board Project No. R-10678 Is residual TB infection in deer and pig populations important? http://www.tbfree.org.nz/Portals/0/2014AugResearchPapers/Nugent%20G,%20Whitford%20J.%20Is%20residual%20Tb%20infection%20in%20deer%20and%20pig%20populations%20important.pdf (accessed 20 September 2014). TBfree New Zealand, Wellington, NZ, 2007
- *Nugent G, Whitford J. Animal Health Board Project No. R-10652 Relative utility of TB hosts as sentinels for detecting TB. http://www.tbfree.org.nz/Portals/0/2014AugResearchPapers/Nugent%20G,%20Whitford%20J.%20Relative%20utility%20of%20Tb%20hosts%20as%20sentinels%20for%20detecting%20Tb.pdf (accessed 20 September 2014). TBfree New Zealand, Wellington, NZ, 2008
- *Nugent G, Sweetapple P, Coleman J, Suisted P. Possum feeding patterns; dietary tactics of a reluctant folivore. In: Montague, TL (ed). The Brushtail Possum. Biology, Impact, and Management of an Introduced Marsupial. Pp 10–23. Manaaki Whenua Press, Lincoln, NZ, 2000
- Nugent G, Whitford J, Young N. Use of released pigs as sentinels for Mycobacterium bovis. Journal of Wildlife Diseases 38, 665–77, 2002
- Nugent G, Warburton B, Thomson C, Sweetapple P, Ruscoe W. Effect of prefeeding, sowing rate and sowing pattern on efficacy of aerial 1080 poisoning of small-mammal pests in New Zealand. Wildlife Research 38, 249–59, 2011
- Nugent G, Warburton B, Thomson C, Cross ML, Coleman MC. Bait aggregation to reduce cost and toxin use in aerial 1080 baiting of small mammal pests in New Zealand. Pest Management Science 68, 1374–9, 2012a
- Nugent G, Whitford J, Yockney I, Cross ML. Reduced spillover transmission of Mycobacterium bovis to feral pigs (Sus scofa) following population control of brushtail possums (Trichosurus vulpecula). Epidemiology and Infection 140, 1036–47, 2012b
- Nugent G, Yockney I, Whitford J, Cross ML. Mortality rate and gross pathology due to tuberculosis in wild brushtail possums (Trichosurus vulpecula) following low dose subcutaneous injection of Mycobacterium bovis. Preventive Veterinary Medicine 109, 168–75, 2013
- Nugent G, Buddle BM, Knowles GJE. Epidemiology and control of Mycobacterium bovis infection in brushtail possums (Trichosurus vulpecula), the primary wildlife host of bovine tuberculosis in New Zealand. New Zealand Veterinary Journal 63 (Suppl. 1), 28–41, 2015
- *O'Neil BD. Control measures to contain a tuberculosis breakdown in a deer herd. Proceedings of a Deer Course for Veterinarians, No. 7, Deer Branch of the New Zealand Veterinary Association. Pp 43–8, 1990
- O'Neil BD, Pharo HJ. The control of bovine tuberculosis in New Zealand. New Zealand Veterinary Journal 43, 249–55, 1995
- Paterson BM, Morris RS. Interactions between beef cattle and simulated tuberculous possums on pasture. New Zealand Veterinary Journal 43, 289–93, 1995
- Pfeiffer DU, Hickling GJ, Morris RS, Patterson KP, Ryan TJ, Crews KB. The epidemiology of Mycobacterium bovis infection in brushtail possums (Trichosurus vulpecula Kerr) in the Hauhungaroa Ranges, New Zealand. New Zealand Veterinary Journal 43, 272–80, 1995
- *Pracy LT. Introduction and liberation of the opossum (Trichosurus vulpecula) into New Zealand. New Zealand Forest Service Information Series 45. 2nd Edtn. P 28. New Zealand Forest Service, Wellington, NZ, 1974
- *Pracy LT. New Zealand possum population density distribution maps, 1948–50 and 1961–63. http://www.landcareresearch.co.nz/__data/assets/pdf_file/0009/76824/possum-distribution-maps.pdf (accessed 24 September 2014). Landcare Research Archive Collection, Lincoln, NZ, 1997
- Price-Carter M, Rooker S, Collins DM. Comparison of 45 variable number tandem repeat (VNTR) and two direct repeat (DR) assays to restriction endonuclease analysis for typing isolates of Mycobacterium bovis. Veterinary Microbiology 150, 107–14, 2011
- Ragg JR, Moller H, Waldrup KA. The prevalence of bovine tuberculosis (Mycobacterium bovis) infections in feral populations of cats (Felis catus), ferrets (Mustela furo) and stoats (Mustela erminea) in Otago and Southland, New Zealand. New Zealand Veterinary Journal 43, 333–7, 1995
- Ragg JR, Mackintosh CG, Moller H. The scavenging behaviour of ferrets (Mustela furo), feral cats (Felis domesticus), possums (Trichosurus vulpecula), hedgehogs (Erinaceus europaeus) and harrier hawks (Circus approximans) on pastoral farmland in New Zealand: Implications for bovine tuberculosis transmission. New Zealand Veterinary Journal 48, 166–75, 2000
- *Ramsey DSL, Efford M. Animal Health Board Project Report No. R-10619 Eliminating TB – results from a spatially explicit stochastic model. http://www.tbfree.org.nz/Portals/0/2014AugResearchPapers/Ramsey%20DSL,%20Efford%20M.%20Eliminating%20Tb%20-%20results%20from%20a%20spatially%20explicit,%20stochastic%20model.pdf (accessed 20 September 2014). TBfree New Zealand, Wellington, NZ, 2005
- Ramsey DSL, Efford MG. Management of bovine tuberculosis in brushtail possums in New Zealand: predictions from a spatially explicit, individual-based model. Journal of Applied Ecology 47, 911–9, 2010
- Reviriego Gordejo FJ, Vermeersch JP. Towards eradication of bovine tuberculosis in the European Union. Veterinary Microbiology 112, 101–9, 2006
- Rockhill B, Newman B, Weinberg C. Commentary: Use and misuse of population attributable fractions. American Journal of Public Health 88, 15–9, 1998
- Ryan TJ, Buddle BM, de Lisle GW. An evaluation of the gamma interferon test for detecting bovine tuberculosis in cattle 8–28 days after tuberculin skin testing. Research in Veterinary Science 69, 57–61, 2000
- Ryan TJ, Livingstone PG, Ramsey DSL, de Lisle GW, Nugent G, Collins DM, Buddle BM. Advances in understanding disease epidemiology and implications for control and eradication of tuberculosis in livestock: The experience from New Zealand. Veterinary Microbiology 112, 211–9, 2006
- Sakula A. Robert Koch: centenary of the discovery of the tubercle bacillus. Thorax 37, 246–51, 1982
- *Sanson RL. Tuberculosis in goats. Surveillance 15 (2), 7–8, 1988
- Sauter CM, Morris RS. Behavioural studies on the potential for direct transmission of tuberculosis from feral ferrets (Mustela furo) and possums (Trichosurus vulpecula) to farmed livestock. New Zealand Veterinary Journal 43, 294–300, 1995
- Smith NH. The global distribution and phylogeography of Mycobacterium bovis clonal complexes. Infection, Genetics and Evolution 12, 857–65, 2012
- *Speedy C. Field trials and operational results of a deer repellent for 1080 possum baits. New Zealand Journal of Forestry 50, 27–30, 2005
- Tompkins DM, Ramsey DSL, Cross ML, Aldwell FE, de Lisle GW, Buddle BM. Oral vaccination reduces the incidence of tuberculosis in free-living brushtail possums. Proceedings of the Royal Society B 276, 2987–95, 2009
- Tweddle NE, Livingstone PG. Bovine tuberculosis control and eradication programs in Australia and New Zealand. Veterinary Microbiology 40, 23–39, 1994
- *Warburton B. Trap-catch for monitoring possum populations. http://www.tbfree.org.nz/Portals/0/2014AugResearchPapers/Warburton%20B.%20Trap-catch%20for%20monitoring%20possum%20populations.%20Manaaki%20Whenua%20-%20Landcare%20Research%20contract%20report%20no.%20LC9596060,%20Lincoln%20NZ,%201996.pdf (accessed 20 September 2014). TBfree New Zealand, Wellington, NZ, 1996
- *Wright, J. Evaluating the use of 1080: Predators, Poisons and Silent Forests. http://www.pce.parliament.nz/assets/Uploads/PCE-1080.pdf (accessed 26 June 2014). Parliamentary Commissioner for the Environment, Wellington, NZ, 2011