Abstract
The control and eventual eradication of bovine tuberculosis (TB) poses major challenges in New Zealand, given the variety of wildlife species susceptible to TB, many of which are capable of onwards transmission of Mycobacterium bovis infection. Here we discuss the role of feral ferrets (Mustela furo), focussing on potential transmission or risk pathways that have implications for management of TB. Firstly inter-specific transmission to ferrets. Ferrets scavenge potentially infected wildlife, including other ferrets, thus prevalence of TB can be amplified through ferrets feeding on tuberculous carcasses, particularly brushtail possums (Trichosurus vulpecula). Secondly intra-specific transmission between ferrets. The rate of ferret-ferret transmission depends on population density, and in some places ferret densities exceed the estimated threshold for disease persistence. TB can therefore potentially be maintained independently of other sources of infection. Thirdly transmission from ferrets to other wildlife. These include the main wildlife maintenance host, brushtail possums, that will occasionally scavenge potentially tuberculous ferret carcasses. Fourthly transmission from ferrets to livestock. This is considered to occur occasionally, but the actual rate of transmission has never been measured. Fifthly geographical spread. M. bovis-infected ferrets can travel large distances and cause new outbreaks of TB at locations previously free of TB, which may have caused an expansion of TB-endemic areas.Ferrets play a complex role in the TB cycle in New Zealand; they are capable of contracting, amplifying and transmitting M. bovis infection, sometimes resulting in ferret populations with a high prevalence of TB. However, ferret population densities are usually too low to sustain infection independently, and transmission to other wildlife or livestock appears a rarer event than with possums. Nevertheless, management of ferrets remains a key part of the National Pest Management Strategy for TB. Control is prudent where M. bovis-infected ferret populations exist in high numbers, to reduce the onward transmission risk of any self-sustained infection to livestock. When ferret numbers are well below the theoretical disease maintenance threshold, ferret control is still sometimes warranted because of the animals’ ability to acquire infection when young and, through dispersal, transport it outside TB-endemic areas. Ferrets can also be used as disease sentinels for TB, especially in areas where alternative sentinel species are rare or expensive to survey, and when sampling of possums is not cost-effective.
Introduction
Estimating the extent of transmission of Mycobacterium bovis within and between wildlife hosts is a prerequisite for effective control of bovine tuberculosis (TB) in livestock (de Lisle et al. Citation2005a; Gavier-Widen et al. Citation2009; Palmer et al. Citation2012). In New Zealand, concerns centre primarily on four species, all of which are introduced: brushtail possums (Trichosurus vulpecula), wild deer (mainly Cervus elaphus), wild (originally feral) pigs (Sus scrofa) and feral ferrets (Mustela furo) (Nugent Citation2011). All four species are frequently infected and play varying roles in both the persistence and spread of disease at a landscape scale (Nugent Citation2011; Anderson et al. Citation2013; Yockney et al. Citation2013), thus complicating efforts to control and ultimately eradicate TB from agricultural systems. Understanding the routes and frequencies of M. bovis transmission among these species is crucial in designing efficient and effective programmes for disease management (Renwick et al. Citation2007; Yockney et al. Citation2013).
In this paper we first review historical data and research on the role of ferrets as a host and potential vector of TB in New Zealand. This includes a synthesis of relevant information about the ecology of ferrets relevant to TB management, and a summary of what is known or suspected about the various pathways for intra- and inter-specific transmission and geographic spread of TB involving ferrets, placing particular emphasis on ferrets as part of a multi-host complex. We evaluate the importance of these pathways in terms of the main risks or impacts posed by ferrets in relation to the management needs for TB control in New Zealand. Finally we evaluate the potential use of ferrets as sentinels for disease surveillance to support TB management. Where considered necessary to address the aims of this paper, we present previously unpublished research contracted by New Zealand's TB management agency (TBfree New Zealand).
The epidemiology of TB and its relationship to ferret ecology
History and prevalence of TB in ferrets
Tuberculosis in feral ferrets was first recorded in Taumaranui, in the Manawatu-Wanganui region of New Zealand, in 1982 (de Lisle et al. Citation1993). In the late 1980s, TB was recorded in ferrets from the Marlborough region (de Lisle et al. Citation1993) and in 1990 in the Mackenzie Basin in the South Island (Walker et al. Citation1993). By 1993, it was considered by New Zealand's Chief Veterinary Officer that there was increasing epidemiological evidence that, in rabbit-prone areas, ferrets were acting as vectors of TB for cattle and farmed deer, and they were implicated in the rapid expansion of the TB-affected area in North Canterbury (O'Hara Citation1993). Subsequent observations indicated that wherever ferrets overlapped with tuberculous cattle and possums, the disease was also present in the ferrets (Ragg et al. Citation1995a; Ragg and Walker Citation1996; Caley Citation1998). It soon became apparent there was a strong relationship between disease prevalence in ferrets and the density of tuberculous possum populations (Caley et al. Citation2001). Beginning in the early 1990s, research into the basic population ecology, behaviour and disease status of ferrets began to elucidate their role in TB transmission (Ragg Citation1997; Morley Citation1999; Byrom Citation2001). Early reviews of epidemiology of TB in wildlife in New Zealand provided some insight into the nature of the disease not only in possums but in other wildlife hosts including ferrets. (Morris et al. Citation1994; Morris and Pfeiffer Citation1995)
Historically, the prevalence of macroscopic TB lesions in ferrets ranged from 0 to 66% across several sites in New Zealand (Caley Citation1998; Caley et al. Citation2001; Caley and Hone Citation2005). However, before instigation of intensive lethal control of possums (and ferrets) for TB management, gross lesion prevalences between 15% and 20% appear to have been the norm for ferret populations in TB-endemic regions of the South Island (Ragg et al. Citation1995a; Byrom Citation2004; Byrom et al. Citation2008). As with most wildlife cross-sectional surveys based on visual necropsy data alone, gross diagnosis generally underestimates the true prevalence of M. bovis infection in ferrets, and estimates of prevalence based on bacterial culture of key tissues from necropsied ferrets tend to be substantially higher than those based on visual diagnoses alone. In samples where both culture and lesion status have been reported, 27–90% of M. bovis culture-positive ferrets have been cited as showing no visible lesions (NVL) (Lugton et al. Citation1997a; Caley et al. Citation1998; Byrom et al. Citation2008). High proportions of culture-positive ferrets with NVL may reflect cursory necropsies being undertaken in studies where all samples were being submitted for bacteriological culture regardless of lesion status. In general, feral ferret surveys reported in New Zealand over the last decade have often recorded a high proportion of individuals with NVL among culture positive cases (de Lisle et al. Citation2005a, Citationb, Citation2008). By contrast to the New Zealand situation, there are few data available for the progenitor wild species in Europe; the polecat (Mustela putorius). Just a single culture-positive TB case with NVL was recorded among 24 polecats surveyed from TB-endemic regions of Great Britain by Delahay et al. (Citation2007).
In New Zealand, possum control (even without ferret control) has been reported to reduce TB infection among sympatric ferret populations: Caley et al. (Citation2001) recorded an 80% reduction in M. bovis infection among ferrets in the years immediately following localised possum control alone (compiled results from several study sites). In line with that, the imposition of large-scale possum control nationally since the late 1990s (either with or without concurrent effort to control ferrets) has seen major reductions in M. bovis infection in ferrets overall, from the 15% to 20% noted above as the norm in TB-endemic areas in the early 1990s, to <1% now (33 infected ferrets from 3,782 surveyed in 2010/11; Anonymous Citation2011a). As an example from one of the areas in which TB in ferrets has been most studied, the Mackenzie Basin in the central South Island, prevalence of TB in ferrets has fallen to near zero over the last two decades since active management of TB in wildlife was instigated. The Mackenzie Basin comprises semi-arid grassland and tussock habitats with, in the 1990s, a high density of rabbits (up to 1,900/km2; Moller et al. Citation1997) consequently supporting a high density of ferrets (up to 5/km2; Moller et al. Citation1996). At that time, prevalence of TB among ferrets was high, with more than 15% of animals with culture-confirmed M. bovis infection (Walker et al. Citation1993). Since then, sustained and intensive possum control has been imposed over an area of 5,000 km2. In 2010/11 over 1,000 ferrets were necropsied but only one M. bovis culture-positive ferret was recorded (Anonymous Citation2011a). However, it is not possible to definitively attribute that decline solely to possum control, because some modest ferret control and/or TB surveillance of ferrets was imposed in conjunction with possum control, and because rabbit numbers declined greatly after the introduction of a rabbit calicivirus in 1997, which by itself is likely to have resulted in lower ferret densities overall (Norbury et al. Citation2003).
The prevalence of TB among ferret populations in TB-endemic areas appears to increase with ferret age (Ragg Citation1997; Lugton et al. Citation1997b; Byrom et al. Citation2008) (). The youngest known infected ferrets are about 1.75 months old, with models fitted to the age-specific prevalence data suggesting a low or zero prevalence in newborn kits (Caley and Hone Citation2002, Citation2004). In TB-endemic areas not subject to intensive population control of possums, M. bovis culture-positive prevalence among adult ferrets can reach 100% by 12 months of age (Caley and Hone Citation2002). Overall, disease is more prevalent among male ferrets than females (Ragg Citation1997; Lugton et al. Citation1997b; Caley et al. Citation1998). The instantaneous rate of new M. bovis infections in ferrets in TB-endemic areas has been estimated to vary from 0.09 to 7.9 per year (Caley and Hone Citation2002), with male ferrets acquiring new M. bovis infections at 2.2 times the rate of females. Sex-specific differences in prevalence of TB are most likely due to male ferrets being more likely to find and/or scavenge carrion than females, due to their larger home ranges (Lugton et al. Citation1997b; Ragg Citation1998).
Figure 1. Estimated age-specific prevalence of tuberculosis (TB) in a sample of 407 ferrets (Mustela furo) from Molesworth Station in the Marlborough region of New Zealand. Age classes are monthly below 1 year, then pooled for 16–18 months old and >23 months. Age-specific prevalence of TB was estimated by ageing all infected ferrets and a random selection of uninfected ferrets, using the latter to estimate the age distribution for all uninfected ferrets (from Nugent and Whitford Citation2008).
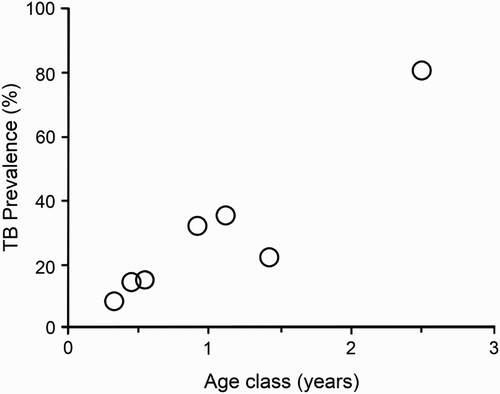
Disease characteristics in ferrets
In feral M. bovis-infected ferrets, gross tuberculous lesions occur most commonly in lymph nodes draining the alimentary tract (34.5%) but rarely in the lungs (2.9%) (Ragg et al. Citation1995b), indicating relatively little pulmonary pathology in this species. This indicates that ferrets probably acquire infection primarily via the oral route under natural conditions, most likely through ingestion of infected material (Lugton et al. Citation1997a, Citationb). In support of this, experimental infection studies have confirmed ubiquitous development of M. bovis infection and representative TB pathogenesis following oral exposure of captive ferrets to inocula of virulent M. bovis (Cross et al. Citation2000a, Citationb). In the wild, ferrets most likely contract M. bovis infection through scavenging tuberculous carrion (particularly possum carcasses) and/or by killing M. bovis-infected prey from the age of weaning (Smith et al. Citation1995; Ragg et al. Citation2000; Caley and Hone Citation2002). Ferrets are known to cannibalise, so ferret-to-ferret (intra-specific) transmission is also possible via that route (Ragg et al. Citation2000; McAuliffe Citation2001; Byrom Citation2004).
The main sites of M. bovis pathology in feral ferrets are the retropharyngeal and mesenteric (jejunal) lymph nodes, associated with the upper- and mid-alimentary tract, respectively. This pattern of lesion distribution is analogous to that observed in similarly sized carnivorous/scavenging meerkats (Suricata suricatta) in Africa (Drewe et al. Citation2009), although in the absence of the pulmonary disease observed in meerkats. In ferrets, macroscopic alimentary tract TB lesions are usually characterised by enlargement and erythema of the nodes, with gross lymphadenopathy and extensive liquefactive necrosis a feature of advanced disease (Lugton et al. Citation1997a). These clinical features have been successfully emulated in captive ferrets by feeding them small (<1 cm3) offal samples containing high doses of virulent M. bovis (i.e. >106 bacteria) (Qureshi et al. Citation1997, Citation1999), reinforcing the suggestion of ingestion of material bearing large numbers of bacilli (such as tuberculous carrion) as the predominant mode of infection in the wild.
Infected ferrets excrete M. bovis bacilli more readily than pigs or deer, most commonly via the oral cavity, with 23% of oral swabs culturing positive, and tracheobronchial swabs, faeces, urine and mammary glands also being positive (Lugton et al. Citation1997b; Qureshi et al. Citation2000). In experimental infection studies, tuberculous ferrets survived for at least 20 weeks post-infection, despite substantial loss of bodyweight and condition (Cross et al. Citation2000a, Citationb). Progression of the disease can be prolonged in ferrets and it may eventually contribute to mortality in the wild (Lugton et al. Citation1996). However, because of their inherent high annual mortality rate, which can average 1.1 per year for females (Caley and Morriss Citation2001), there remains uncertainty over whether tuberculous ferrets suffer increased mortality due to disease advancement (Caley and Hone Citation2002).
In common with the other major wildlife hosts in New Zealand and elsewhere, protective vaccination against M. bovis has been investigated in ferrets as a potential means of controlling TB. Vaccination using M. bovis bacille Calmette-Guérin has been shown experimentally to confer some protection to captive ferrets against pathogenic strains of the bacillus (Qureshi et al. Citation1999; Cross et al. Citation2000b); however, lethal population control currently remains the tool used for control of TB in feral ferrets. As with the other mammalian wildlife hosts of TB in New Zealand, the wide-scale use of lethal control is facilitated because ferrets are an introduced invasive pest species.
Ferret population demographics and ecology
Ferrets prefer pasture, rough grasses and scrubland (Clapperton and Byrom Citation2005) and there are good correlations between ferret density and the abundance of rabbits, their primary prey (Mills Citation1994; Ragg and Walker Citation1996; Norbury et al. Citation2002). As a result, ferret densities are highest in semi-arid rabbit-prone grasslands and braided river areas of the eastern South Island, where estimated population densities range from 2.9 to 10.1 per km2, with densities typically lower at North Island sites (). These habitats therefore hold the greatest risk of persistence of TB in ferrets independent of other wildlife hosts. An extensive investigation of field techniques to assess ferret relative abundance, and calibrating these to absolute densities, was undertaken by Moller et al. (Citation2002) and concluded that, while no relative abundance measurement technique was particularly precise, a trap-catch index (TCI) offered the most reliable measure. The authors suggested that standardised levels of residual trap catch indices could be set to guide wildlife management decisions around ferret populations, similar to the way these are used by possum TB managers (Anonymous Citation2011b).
Table 1. Estimates of feral ferret (Mustela furo) densities (per km2) for different habitats in New Zealand.
Life expectancy of juvenile ferrets once they emerge from their natal den typically ranges from 1 to 2 years (Caley and Morriss Citation2001; Byrom et al. Citation2008), although survival up to 3.5 years has been recorded (Nugent and Whitford Citation2008). Caley et al. (Citation2002) estimated a survival probability of 25% during the first year of life, rising to 55% thereafter, resulting in a skewed age distribution towards young individuals in any population. In the Mackenzie Basin, survival of juveniles varied from 19% to 100% in the 4 months after emergence from the natal den, decreasing to 0–60% by the end of the first winter, depending inversely on ferret density (Byrom Citation2002). Annual survival rates of 15–65% have been reported for feral ferrets in New Zealand (Norbury and Heyward Citation1997; Morley Citation2002; Byrom et al. Citation2008), similar to reported rates of 32–67% for the polecat in Europe (Kristiansen et al. Citation2007). Due to the high annual mortality rate among ferrets, they are regarded as less likely to contribute to risk of TB persistence than longer-lived wildlife hosts of TB in New Zealand, such as wild deer (Barron et al. Citation2013; Nugent et al. Citation2015b).
Home ranges and movement patterns
Movements and home ranges of wildlife hosts provide an indication of the potential for both disease spread (Byrom Citation2004) and for surveillance utility (Anderson et al. Citation2013; Yockney et al. Citation2013). Home range size of ferrets varies between habitats, but is typically around 140 hectares for males and 100 hectares for females (). Male and female home ranges overlap, both within and between the sexes, especially in areas with high densities of ferrets resulting in high levels of social contact (Ragg Citation1997; Norbury et al. Citation1998a; Moller and Alterio Citation1999). Ferrets increase the size of their home ranges at times of low food availability, for example after rabbit population control (Norbury et al. Citation1998b).
Table 2. Home range sizes (hectares) of male and female ferrets (Mustela furo) in the South Island of New Zealand.
Male and female ferrets are equally likely to disperse, and 50% of juvenile ferrets move more than 2–5 km from their place of birth (Caley and Morriss Citation2001; Byrom Citation2002). Most dispersal occurs during late summer (late February and early March), with no sex difference in mean dispersal distance (Caley and Morriss Citation2001; Byrom Citation2002; Byrom et al. Citation2008). Three straight-line dispersal distances were recorded of juvenile female ferrets of 12, 20 and 27 km in the Maniototo district of the Otago region by Ragg (Citation2002).
Caley and Morriss (Citation2001) reported that ferrets dispersed non-directionally across pasture habitat in North Canterbury, with a mean dispersal distance of 2.1 (SE 1.0) km; whereas in the Mackenzie Basin, dispersal has been reported to be directional, following braided riverbed channels. Mean dispersal distances were longer and were recorded as 6.7 (SE 1.6) km for males and 11.8 (SE 3.4) km for females (Byrom Citation2002). In a mix of pasture, scrub and riverbed habitat in inland Marlborough, maximum distances travelled by ferrets of both sexes were 10–27 km (Byrom Citation2004; Byrom et al. Citation2008).
Control, capture and survey methods
Control of ferrets is conducted primarily by trapping (Spurr et al. Citation2001), usually using traps baited with carrion, e.g. rabbit meat, chicken necks (Norbury et al. Citation2004). Poisoning is rarely used, although sometimes fish-based bait containing diphacinone can be deployed (Spurr et al. Citation2001). Ferret control is done mostly in late summer/autumn and early winter, because control at that time will have the greatest impact on the population (Barlow and Norbury Citation2001), not least because juvenile dispersal and low adult trap-shyness at these times makes ferrets easier to target. Most trapping for surveillance purposes is also conducted at that time, mostly to maximise the numbers of ferrets obtained by avoiding times of low trappability.
Ferrets can also be controlled through secondary poisoning, particularly of rabbit populations, although kill rate is highly variable depending on the toxin used. Heyward and Norbury (Citation1999) recorded approximately 10% incidental ferret mortality as a result of rabbit poisoning using carrots coated with 0.02% w/w sodium fluoroacetate (1080). In contrast, Alterio (Citation1996) recorded a 100% mortality in five ferrets following a rabbit poisoning operation using broadifacoum. The recent emphasis on ferrets as sentinels means that trapping is still the preferred method of control as it enables carcass recovery.
As alluded to above, capture rates and bait-take by ferrets decline during mid- to late-winter and into spring, coincident with the breeding season (Clapperton and Byrom Citation2005). Several hypotheses have been proposed to explain this (Spurr et al. Citation2001), including a preference for live prey as juvenile rabbits become more common at this time (Byrom et al. Citation2003), reduced home range sizes and reduced food consumption, and/or seasonal neophobia (i.e. a transient aversion to novel objects in the environment). However, home ranges appear to change little during the breeding season and ferrets may actually increase their activity levels within their range during that time (Ragg Citation2002), while the hypothesis of reduced food intake has been only weakly supported in empirical studies (Spurr et al. Citation2001). Of the above theories, neophobia appears the most plausible explanation to explain changes in seasonal trappability (Hammond Citation1996; Byrom et al. Citation2002). Attempts to increase the trappability of ferrets during late winter and spring (e.g. using scented lures) have met with only limited success (Clapperton Citation1996; Spurr et al. Citation2001).
Risk factors posed by ferrets to TB management
Inter-specific transmission of M. bovis to ferrets
Quantifying the relative risk of infected wildlife such as pigs, deer and possums causing new TB outbreaks in ferrets is important in characterising potential routes and rates of transmission within the New Zealand wildlife host complex (Caley and Hone Citation2004). As noted above, experimentally reducing possum (but not ferret) abundance can reduce prevalence of TB in ferrets (Caley et al. Citation2001). That research identified that possum-to-ferret transmission was common, and indicated that most M. bovis infection observed in ferrets had resulted from transmission from infected possums (Caley and Hone Citation2005). In support of this, Ragg et al. (Citation2000) and McAuliffe (Citation2001) used night-time video surveillance of freshly killed possum carcasses to characterise visits by ferrets to carrion in South Island farmland settings. The two studies found that 77% and >20%, respectively, of ferret encounters with possum carcasses resulted in feeding.
Ferrets also scavenge on the carcasses of other hosts of TB. Yockney and Nugent (Citation2003) investigated the rate of scavenging of potentially infectious pig carcasses by ferrets in a mosaic of scrub, river flats, and farmland in the Marlborough region, also using detailed video surveillance. Ferrets visited 10/13 (77%) pig offal piles in summer, but none in winter. Probable family groups of ferrets were observed feeding together, often spending long periods of time at a carcass and clambering over each other and the carcass to gain access to soft visceral tissues (Yockney and Nugent Citation2003). In the four instances in which a whole pig carcass was scavenged, ferrets were responsible for the initial opening of the carcass, leading the authors to conclude that the remains of just one infected pig could theoretically amplify the numbers of tuberculous wild animals locally. Ferrets have also been observed feeding on the carcasses of deer (Yates et al. Citation1995).
When coupled with the predominance of alimentary tract lesions, these studies and observations strongly implicate scavenging on the lesioned carcasses of other hosts of TB (particularly possums) as a likely mechanism of infection for ferrets, suggesting that they might be amplifier hosts of infection, i.e. one tuberculous carcass producing many new infected ferret cases. Through amplification of the total burden of infection in the environment, ferrets therefore have the potential to deepen and broaden the risk pathways that could generate new outbreaks of TB. What is not known with any accuracy or precision is the rate at which ferrets become infected from other infected wildlife. Nevertheless, to enable quantitative use of ferrets as sentinels in predictive epidemiological modelling frameworks, Anderson et al. (Citation2013) proposed and used a probability value of 0.08 to describe the possible transmission rate to ferrets arising from a single infected possum in cases involving home range overlap.
Intra-specific transmission; infection of ferrets by ferrets
Ferrets have the potential not only to amplify M. bovis infection but to independently sustain it. There has been considerable debate over the route and extent of intra-specific transmission (Caley and Hone Citation2004, Citation2005).
Several possible routes by which intra-specific M. bovis transmission could occur among ferrets in the wild have been postulated, including cannibalism (Ragg et al. Citation2000; McAuliffe Citation2001; Caley and Hone Citation2005), pseudo-vertical transmission between mothers and their dependent offspring, routine social contact such as mating or fighting (Caley and Hone Citation2002), or other environmental factors such as animals examining latrine sites. Qureshi et al. (Citation2000) demonstrated horizontal transmission of three strains of M. bovis among captive ferrets housed at close quarters in a pen trial, although by an undefined mechanism. Other observed risk behaviours included den sharing, sniffing of orifices and faeces, cannibalism and aggressive mating behaviour. Infection through cannibalism is possible as McAuliffe (Citation2001) observed that ferrets were more than twice as likely to visit ferret carcasses than hedgehog or possum carcasses in a field study in North Canterbury farmland in autumn-winter, and >10% of these visits resulted in scavenging. Other studies have reported scavenging rates of ferrets by ferrets ranging from 31% to >60% (Ragg et al. Citation2000; Byrom Citation2004; Caley and Hone Citation2005).
Table 3. Percentage of ferret (Mustela furo) carcasses visited by each of five wildlife species and two livestock species in summer and winter, with average time to first discovery for carcasses visited, and the percentage of carcasses touched or sniffed, licked or fed upon. There were 13 ferret carcasses in summer and 20 in winter (from Byrom Citation2004).
Acquisition of infection by non-oral routes has also been inferred. Approximately 20% of visible TB lesions in ferrets occur at peripheral lymph node sites in surveys reporting gross pathology (Ragg et al. Citation1995a), suggesting that infection might sometimes occur via skin breakage, possibly through abrasions incurred during aggressive social contacts with other ferrets. This has also been reported among tuberculous cases in Eurasian badgers (Meles meles) and African meerkats (Gallagher and Clifton-Hadley Citation2000, Drewe et al. Citation2009). Peripheral site infections are more common among males, possibly reflecting the higher rate at which males interact with other ferrets (Ragg et al. Citation1995b; Lugton et al. Citation1997b), which could also explain the greater force of infection (the rate at which susceptible individuals acquire infection) observed in male ferrets (Caley and Hone Citation2002). Overall, however, non-oral acquisition of infection in feral ferrets in New Zealand is thought to be less important than oral acquisition (Caley and Hone Citation2002).
More crucially important than the mechanism(s) of intra-specific transmission between ferrets is the purported rate at which this occurs. If each infected ferret could infect (on average) one or more other ferrets during its life-span, elimination of TB from wildlife would necessarily require ferret management. In reality, this could occur wherever ferret populations surpassed the threshold density theoretically required for independent disease maintenance (Caley and Hone Citation2005). Caley (Citation2002) demonstrated that, among high-density populations, reducing ferret abundance alone could reduce the consequent force of infection in ferrets, indicating some level of intra-specific transmission was occurring. But such transmission in isolation was not sufficient to signify sustained independent maintenance of TB in the ferret population. In order for ferrets to maintain TB independently of other wildlife sources of infection, it was postulated that ferret populations would need to reach a peak density of ≥5.3 ferrets per km2, and a year-round density of ≥2.9 ferrets per km2 although these figures have wide confidence limits (Caley Citation2002). There has been no further research on this, so more precise empirical data, quantifying the rate at which intra-specific transmission naturally occurs in ferrets, are still needed to unequivocally clarify the host status of ferrets in different habitats (Caley and Hone Citation2005) and for epidemiological models of infection in multi-host complexes (Craft et al. Citation2008).
Thus there seems to be little doubt that ferret-ferret transmission occurs (probably by a variety of mechanisms), and occurs frequently enough to temporarily amplify infection within the species at high population densities, but it appears to only rarely, if ever, occur frequently enough for ferrets to be regarded as true maintenance hosts. Accordingly, ferrets are generally classed as being spillover hosts in New Zealand's multi-host wildlife TB complex (Nugent Citation2011).
The risk of spillback infection to other wildlife hosts from ferrets
As ferrets are seen as spillover hosts in most areas, determining the risk of spillback is crucial in assessing whether ferrets should be regarded as an end-host, or whether M. bovis-infected ferrets have the potential to cause new TB outbreaks in the other wildlife species from which they, directly or indirectly, originally acquired TB. From the perspective of TB management, the principal risk is that ferrets could re-establish infection in populations of the primary maintenance host (possums) among which M bovis had been eradicated, and after possum control had been discontinued. Ferrets could potentially do this by carrying TB into the area from some distant source, or by acting as a ‘go-between’ host, transmitting residual infection from long-lived hosts (such as deer) to possums (Nugent Citation2011). This could occur by interactions between the other wildlife species and live M. bovis-infected ferrets, or through interaction with ferret carcasses. The former seems unlikely, as observations of livestock interactions with simulated terminally ill wildlife have shown that deer are far less likely to make contact with ferrets than with possums (Sauter and Morriss Citation1995). Further, we are unaware of data or observations showing interaction between live ferrets and possums, and suggest that healthy ferrets would avoid pigs.
Compared to pigs and deer, the often highly liquefactive lesions observed in ferrets probably contain greater numbers of live bacilli per gram of tissue and potentially present more of an infection risk (Lugton et al. Citation1996), thus tuberculous ferret carcasses may represent a greater risk of spillback infection of M. bovis per unit size than do those of pigs and deer (Nugent et al. Citation2003). Accordingly, the risk to other wildlife species of infection from tuberculous ferrets is mostly the risk posed by ferret carcasses, and this has been explored in monitoring studies. Ferret carcasses (13 in summer and autumn, 20 in winter and spring) were monitored using infrared-triggered cameras in the presence of a full complement of potential wildlife hosts (including pigs and deer) and free-ranging livestock (Byrom Citation2004), in conjunction with a similar study monitoring pig carcasses (Yockney and Nugent Citation2003). Of the 15 different species visiting the ferret carcass sites, sheep and cattle, Australasian harriers (Circus approximans), ferrets, possums, cats, and hedgehogs (Erinaceus europaeus) approached to within 1 m of, and interacted with, the carcasses in some way. Many encounters resulted in behaviours that could be considered risky in terms of TB transmission (i.e. rolling on the carcass, sniffing, licking, or scavenging), and the average duration of each interaction was about 30 seconds. Interactions by different species in summer and winter are presented in . Pigs and deer did not visit the ferret carcasses in either season despite being present in the area. By comparison, the concurrent study on pig carcasses indicated somewhat higher scavenging rates by wildlife, including ferrets (although only during the summer months) (Yockney and Nugent Citation2003). Based on recorded interactions with ferret carcasses in these studies, the authors concluded that the overall risk of transmission of M. bovis infection from dead ferrets to pigs and deer was low, particularly compared with the likelihood of transmission from wild pigs to ferrets.
In the monitoring study of Byrom (Citation2004), possums themselves were not observed directly consuming tissue from ferret carcasses, although other close-contact behaviour, specifically sniffing and licking of carcasses, was noted. In contrast, Ragg et al. (Citation2000) documented an instance (from two encounters) of a possum actively scavenging at a ferret carcass, showing at least the feasibility of direct M. bovis transmission to possums by scavenging from ferret carcasses. Transmission to possums from ferret carcasses could also occur indirectly, as McAuliffe (Citation2001), Ragg et al. (Citation2000) and Byrom (Citation2004) all observed other wildlife species (such as harriers) scavenging from ferret carcasses, sufficient to open up the carcass and scatter potentially infectious material over a wide area. Potentially therefore, any possum subsequently visiting the site (even if it was not actually scavenging on the carcass) could come into contact with infectious material now dispersed beyond the immediate vicinity of the carcass.
Transmission of M. bovis infection to livestock from ferrets
There is little empirical evidence with which to quantify the potential risk of transmission of infection from ferrets to livestock. Ferrets must play some role in livestock infection, because one study has shown that the incidence of TB in cattle can be reduced following ferret control in the area (Caley et al. Citation1998). Potential routes of transmission of M. bovis from ferrets to domestic stock are similar to those postulated for possum-to-livestock routes, i.e. via the mouth or nasopharynx by licking or sniffing carcasses (Sauter and Morris Citation1995), although it is possible that oral-route infection of livestock could also occur by contact with live bacilli shed onto the ground by tuberculous ferrets (Ragg et al. Citation1995a, Citationb; Lugton et al. Citation1997a, Citationb) or by bacilli dispersed from a tuberculous carcass by the action of scavenging ferrets. Shedding of potentially infectious bacilli through faeces and urine, or in maternal milk, has been shown for ferrets. About a quarter of tuberculous ferrets in the study by Lugton et al. (Citation1997b) provided evidence of oral shedding of viable M. bovis bacilli through coughing or sneezing, apparently in ferrets where disease was well advanced.
As noted above, live drugged ferrets exhibiting behaviour similar to late-stage tuberculous animals were nosed and sniffed by cattle and deer in an experimental study, although to a lesser degree than similarly drugged possums (Sauter and Morris Citation1995). Byrom (Citation2004) also observed that cattle investigated ferret carcasses, sniffing and making nose contact on several occasions, often after environmental exposure of opened lesions could have occurred through scavenging by harriers. Whether such behaviours result in transmission of infection to livestock under natural circumstances, and the frequency with which that might occur, are not known under New Zealand conditions, making it difficult to quantify the management significance of this transmission pathway.
The potential for long-distance spread of TB by ferrets
Information on the movements and dispersal patterns of wildlife TB hosts is critical in assessing the role they play not only in disease persistence but also in the geographic spread of the disease, and therefore in determining how much emphasis should be placed on population control as part of vector management programmes (Pech et al. Citation2010). Ferrets are capable of acquiring M. bovis infection soon after emerging from the natal den (Caley and Hone Citation2002; Yockney and Nugent Citation2003). However, dispersal does not occur until about 57 days on average after emergence (Byrom Citation2002), so there is a window in which juveniles could acquire infection and then transport it elsewhere.
The probability of long-distance dispersal of TB by infected ferrets has long been inferred from their general movement behaviour (Livingstone Citation1996), and such dispersal was implicated as the likely underlying cause of the rapid southward spread of TB in North Canterbury in the early 1990s (O'Hara Citation1993). Probable cases of such dispersal were recorded in a study in the North Canterbury high country (Byrom Citation2004) in which 10 juvenile (seven male, three female) ferrets were trapped, radio-collared, and successfully tracked from mid-summer, a time when they were likely to still be in family groups and close to their mothers’ home ranges (Byrom Citation2002), until they were recaptured and killed in autumn, during which time they had moved 0–10.7 (median 2.5) km. By autumn, three of the males were M. bovis-infected, with these three having moved straight-line distances of 1.5, 3.5, and 10.7 km. The carcasses of four of the ferrets were video-monitored and two were confirmed as being scavenged by other ferrets.
The geographical scale of the risk of TB spread as a result of host dispersal is critical in determining the boundaries of Vector Risk Areas (VRA), the areas in which there is a risk of TB being persistent in wildlife. To help TB managers delineate boundaries that could minimise the risk of disease spread by wide-ranging spillover hosts, Nugent et al. (Citation2003) modelled the relative risk of persistence and spread of TB posed by ferrets, assuming that possum densities within VRA boundaries were kept very low and that TB would therefore eventually disappear from both possum and ferret populations (model input parameters as outlined in Supplementary Information 1Footnote1). The modelling predicted that, if ferret densities were close to the theoretical disease maintenance threshold (Caley and Hone Citation2005), TB could persist in the ferret population within a VRA for >20 years, at least when TB-induced mortality in ferrets was set to zero, although it would be <8 years if TB infection significantly increased ferret mortality. Persistence was predicted to reduce substantially if ferret density was reduced (i.e. if ferret control was imposed).
Empirical dispersal data was used from two contrasting case study sites, a typical pastoral habitat, Scargill Valley in North Canterbury (Caley and Morriss Citation2001) and a sparsely vegetated riverbed habitat, the Mackenzie Basin (Byrom Citation2002). Modelling predicted that few juvenile ferrets would disperse long distances in the pastoral habitat but would disperse further in the riverbed habitat, and relatively few ferrets were predicted to disperse more than 10 km in either habitat (Nugent et al. Citation2003). Importantly, for both habitat types, the density of M. bovis-infected juvenile ferrets settling outside the area containing infected possums was high when the possum population inside the VRA was not controlled. Under some scenarios, more than one M. bovis-infected juvenile ferret was predicted to settle beyond the VRA for each kilometre of VRA boundary in the simulation, at a proximity of <1 km to the VRA boundary. Possum control within the VRA was predicted to quickly reduce that figure to one ferret per 15 km of VRA boundary. The density of infected juvenile ferrets settling further outside the VRA boundary was predicted to decline to near zero over just 4 km from the boundary under the Scargill Valley dispersal profile, or 8 km under the McKenzie Basin profile. Overall, the width of a precautionary buffer around the known or suspected locations of TB in possums was therefore predicted be <10 km. Thus, while it is plausible that ferrets could occasionally carry TB up to 27 km from a VRA (Ragg Citation2002), the probability of that is extremely low, excluding any area where ferret densities are high enough to be maintenance hosts.
Use of ferrets for TB surveillance
Over the last two decades prevalence of TB has fallen dramatically in livestock in New Zealand (Livingstone et al. Citation2015) and in possum populations (Nugent et al. Citation2015a), and the focus of research and management efforts has shifted from predominantly control towards a combination of both control and surveillance of TB in wildlife, particularly with the purpose of confirming absence of TB in response to wildlife control (Anderson et al. Citation2015). This has seen increased use of spillover hosts (in this case ferrets) as a sentinel for detecting persistence of M. bovis infection in sympatric possum populations (Nugent et al. Citation2002; Yockney et al. Citation2013; Anderson et al. Citation2013). This concept has now been formally integrated into a national proof of freedom of TB surveillance framework for New Zealand (Nugent et al. Citation2006; Hutchings et al. Citation2011), and also underpins an equivalence-based TB-testing approach developed for game estates (Byrom et al. Citation2004).
The most in-depth comparative analysis of the sensitivity (as sentinels for persistent wildlife TB) of all of the main non-possum hosts of TB in New Zealand was conducted in semi-arid mountain land in the Marlborough region (Molesworth Station 42.2°S, 173.1°E) by Byrom et al. (Citation2008), and Nugent and Whitford (Citation2008) (input data parameters are outlined in Supplementary Information 2Footnote1). The results indicated that 21.7% of 407 ferrets surveyed were infected with M. bovis, with infection prevalence increasing with age (). However, there were no clear changes in patterns of the apparent annual incidence (Thrusfeld Citation1995) of infection with age, indicating that acquisition of new infection probably occurred steadily throughout life for these ferrets. It was estimated that the ferret population accumulated new M. bovis infections steadily at a rate of 0.6 occasions per year of ferret exposure. Ferret densities were crudely estimated to span 0.9–1.5 per km2 which, combined with an apparent annual incidence of 0.6, suggested 0.5–0.9 cases of new ferret M. bovis infection occurring per km2 per year (Nugent and Whitford Citation2008). Using matching data and estimates of possum population density, average home range and prevalence of TB, and assuming a ferret home range size of 1.2 km2 (Byrom et al. Citation2008), Nugent and Whitford (Citation2008) calculated that there would be 1.7–3.4 infected possum carcasses available annually to be encountered within each ferret range. If the bulk of the observed infection in ferrets was acquired from possums, individual ferrets would have become infected on >30% of occasions during which an infected possum carcass occurred within their range. However, that figure, which is effectively the marginal probability of ferrets detecting TB in possums, ignores the possibility of ferrets being infected from pigs or deer carcasses. Therefore a much lower figure (8%) has been adopted conservatively in the proof of freedom model (Anderson et al. Citation2013) that is now widely used to quantify the probability of true absence of TB in possums.
By comparison with the surveillance sensitivity calculated for ferrets, the probability of detection for pigs on Molesworth was estimated by Nugent and Whitford (Citation2008) to be higher still, whereas those for cattle and deer were predicted to be much lower (as shown in Supplementary Table 1Footnote1). Taking into account differences in the mean exposure (life span) of each sentinel species and home range size, and the cost of obtaining and testing each sentinel, ferrets on Molesworth Station were predicted to be only about half as cost-effective (per hectare) as pigs, but twice as cost-effective as using tuberculin testing of cattle, and many times more cost-effective than surveying wild deer, or possums directly (Supplementary Table 1Footnote1). As a result of that moderately high utility, ferrets are now widely used, usually in conjunction with direct surveys of possums, for proving freedom from TB of wildlife during surveillance operations in farmland and unforested semi-arid areas, particularly those where feral pigs and wild deer are scarce (Anderson et al. Citation2015).
In practical terms, the importance of ferrets to effective monitoring and management of TB in New Zealand is borne out in surveillance statistics (Supplementary Information 3 and Supplementary Table 2Footnote1). Over the 2010/11 period, TBfree New Zealand reported capture and necropsy of 17,397 ferrets for TB surveillance (data sourced from unpublished metadata in the VectorNet database, TBfree Ltd, Wellington, NZ). Most of the ferrets were trapped from management units that had been subject to intensive possum control for many years. Some of these surveys were conducted primarily to help assess the probability of freedom from TB, but others aimed to support preventive control, either to investigate the potential role of wildlife in a recent TB outbreak among livestock, or to confirm reduction of TB within VRA or disease containment in areas close to the perimeter of VRA. These latter surveys yielded 4,447 ferrets, including 3,782 captured inside VRA zones, of which 33 animals were confirmed M. bovis-culture-positive. All 33 infected ferrets were located within the southern South Island VRA, representing an overall prevalence of TB in ferrets of 0.89% within VRA and 0% in perimeter areas (Anonymous Citation2011a), suggesting that containment of disease in ferrets to the VRA was effective.
Conclusions: Management of ferrets for TB control in New Zealand
Ferrets are consummate scavengers of potentially infectious material, with a demonstrated propensity for pig (and presumably possum and deer) remains. They are also cannibalistic. Where scavenging occurs within TB-endemic areas, ferrets are likely to amplify the disease. In the habitats most favourable to ferrets, and in the absence of ferret control, amplification by cannibalism (and/or other means) may be sufficient for ferrets to independently maintain TB. Although feasible (Caley Citation2002; Caley and Hone Citation2005), there is, as yet, no confirmation that ferrets do actually achieve true maintenance host status in New Zealand. Rather than maintenance hosts, ferrets might therefore best be characterised as extended spillover hosts in which M. bovis infection originally acquired from possums could then occasionally cycle within a ferret population before eventually disappearing. Thus, even when possum control has been sufficient to reduce inter-specific M. bovis transmission to ferrets to near zero, ferrets may sometimes provide a persistent reservoir of TB after possum control has been undertaken, particularly if there is no additional mortality attributable to the disease burden (Nugent et al. Citation2003).
The probability of M. bovis infection being transmitted from tuberculous ferrets on to, or back to, other species of wildlife through scavenging, particularly by key host species such as pigs and possums, appears to be low (Ragg et al. Citation2000; McAuliffe Citation2001). Likewise, the risk of transmission from live infectious ferrets to other hosts such as cattle and deer appears to be lower than it would be for possums (Sauter and Morris Citation1995). Nonetheless, there are indications that some transmission to livestock does occur (Caley et al. Citation1998), so this risk must also be considered and managed as part of the overall TB problem.
As pigs and deer are spillover hosts for TB in New Zealand (Nugent et al. Citation2015b), and because TB in livestock can be prevented from spreading to other livestock by standard diagnostic testing and movement control means (Buddle et al. Citation2015), it is the risk of occasional transmission of M. bovis infection from ferrets to possums that is of greatest management significance. This is firstly because it could increase the time required to achieve eradication of TB from possums within a VRA. At densities below the theoretical TB maintenance threshold for ferrets, complementing possum control with ferret control should help to reduce the prevalence TB in ferrets, and therefore risk of transmission of infection from ferrets to livestock, more quickly than possum control alone. The geographical areas where this might apply need to be better defined, which would require field validation of ferret-ferret transmission rates and, ideally, the TB-persistence threshold densities proposed by Caley (Citation2002) and Caley and Hone (Citation2005) for key habitat types containing ferrets.
The second main risk from occasional ferret-possum transmission arises because they are more likely, on average, to move further than possums, dispersing on a scale equivalent to that of deer and pigs (Yockney et al. Citation2013). Infected ferrets could, therefore, cause new outbreaks of TB in areas previously free of tuberculous wildlife. However, both the empirical dispersal data available and the modelling summarised above suggest that movement of TB by ferrets is restricted to the immediate periphery of a VRA, as the frequency with which dispersing M. bovis-infected ferrets might transport TB well beyond VRA (>10 km) appears to be low. The probability of an infected ferret dispersing a long distance and the probability of ferret-possum transmission are both low, so the joint risk of both occurring appears negligible, especially once intensive possum control has been initiated. Adding to that, the prevalence of TB is lowest in the juvenile age class most likely to disperse. For older age classes, ferrets pose little risk of moving outside a VRA unless there is a sudden environmental perturbation, such as a rapid reduction in rabbit numbers (Norbury et al. Citation1998b). There is also a smaller likelihood of ferrets being moved by humans compared to species such as deer and pigs (which are hunted for recreation), so the risk of TB spread by ferrets is likely to be low overall (Nugent et al. Citation2003).
As sentinels for assessing the likelihood of freedom from TB in possums, ferrets are cost-effective largely because, like pigs, they cover much larger areas than possums and appear to have a moderately high chance of finding and feeding on any carcasses of tuberculous possums that occur within their home range. Ferrets rank second in terms of wildlife TB-detection sensitivity behind pigs (Yockney et al. Citation2013), but nonetheless they are now frequently surveyed (and concurrently controlled) in many of the less-developed farmland areas within VRA in order to provide data needed to estimate the probability that that area is free of TB. For this purpose, the number of years of active surveillance of ferrets without any detection of TB becomes a crucial consideration. The other purpose of using ferrets as a survey tool is to determine the prevalence and distribution of residual TB in wildlife in areas where it is known (or suspected) to persist even after possum control. Nugent and Whitford (Citation2008) concluded that where TB is common, both pig and ferret necropsy and culture, as well as diagnostic testing in cattle, would all provide equally inexpensive ways of monitoring trends in prevalence of TB. Overall, ferrets have been an important part of the National Pest Management Plan for bovine TB in New Zealand (Anonymous Citation2009) and will remain so.
NVL | = | No visible (TB) lesions |
TB | = | Tuberculosis due to Mycobacterium bovis |
TCI | = | Trap-catch index |
VRA | = | Vector risk area |
14-0021_Byrom_Supplementary_information
Download PDF (129 KB)Acknowledgements
Partial funding for the personnel hours involved in drafting this review was provided by TBfree New Zealand (Project R10735-01), with co-funding from the Ministry of Business, Innovation and employment (Contract C09X0803); neither party exerted influence over the content or significance of the research reviewed here, and the views expressed are solely those of the contributing authors. We thank Frank Cross (Landcare Research, Lincoln) for editorial assistance with the manuscript. Phil Cowan (Landcare Research, Lincoln) provided valuable comments on earlier drafts of the manuscript. Studies reported here involving experimental manipulation of live animals all obtained animal ethics approval from the Landcare Research Animal Ethics Committee, with approval details contained in the original reports. We finally thank Kirsten Vryenhoek of TBfree New Zealand/OSPRI for the compilation of the industry contract reports cited in this manuscript and for making them available online.
Notes
*Non-peer-reviewed
References
- Alterio N. Secondary poisoning of stoats (Mustela erminea), feral ferrets (Mustela furo), and feral house cats (Felis catus) by the anticoagulant poison, brodifacoum. New Zealand Journal of Zoology 23, 331–8, 1996
- Anderson DP, Ramsey DSL, Nugent G, Bosson M, Livingstone P, Martin PAJ, Sergeant E, Gormley AM, Warburton B. A novel approach to assess the probability of disease eradication from a wild-animal reservoir host. Epidemiology and Infection 141, 1509–21, 2013
- Anderson DP, Ramsey DSL, de Lisle GW, Bosson M, Cross ML, Nugent G. Development of integrated surveillance systems for the management of tuberculosis in New Zealand wildlife. New Zealand Veterinary Journal 63 (Suppl. 1), 89–97, 2015
- *Anonymous. National Bovine Tuberculosis Pest Management Strategy: An amendment proposal prepared by the Animal Health Board Incorporated. http://www.tbfree.org.nz/Portals/0/AHB%20NPMS%20Proposal%2009.pdf (accessed 20 September 2014) Animal Health Board, Wellington, New Zealand, 2009
- *Anonymous. TBfree Annual Report 2010/2011. http://www.tbfree.org.nz/Portals/0/AHB%20Annual%20Report_web.pdf (accessed 08 October 2014). TBfree New Zealand, Wellington, NZ, 2011a
- *Anonymous. Best Practice Standard A1 Possum Population Monitoring Using the Trap-Catch Method. http://www.npca.org.nz/images/stories/NPCA/PDF/a1_monittrapc_201110_web.pdf (accessed 08 October 2014). National Pest Control Agencies, Wellington, NZ, 2011b
- *Baker G. Aspects of mammalian predator ecology co-inhabiting giant skink habitat. MSc Thesis, University of Otago, Dunedin, New Zealand, 1989
- Barlow ND, Norbury GL. A simple model for ferret population dynamics and control in semi-arid New Zealand habitats. Wildlife Research 28, 87–94, 2001
- Barron MC, Nugent G, Cross ML. Importance and mitigation of the risk of spillback transmission of Mycobacterium bovis infection for eradication of bovine tuberculosis from wildlife in New Zealand. Epidemiology and Infection 141, 1394–406, 2013
- Buddle B, de Lisle G, Griffin J, Hutchings S. Epidemiology, diagnostics, and management of tuberculosis in domestic cattle and deer in New Zealand in the face of a wildlife reservoir. New Zealand Veterinary Journal 63 (Suppl. 1), 19–27, 2015
- Byrom AE. Ferrets as vectors of bovine TB in New Zealand: a review. Proceedings of the New Zealand Society of Animal Production 61, 60–3, 2001
- Byrom AE. Dispersal and survival of juvenile ferrets Mustela furo in New Zealand. Journal of Applied Ecology 39, 67–78, 2002
- *Byrom AE. R-10618 Spread of Tb by Ferrets in the Northern South Island High Country. http://www.tbfree.org.nz/Portals/0/2014AugResearchPapers/Byrom%20AE.%20Spread%20of%20Tb%20by%20ferrets%20in%20the%20northern%20South%20Island%20high%20country.%20Landcare%20Research%20contract%20report%20LC0304146.%20Landcare%20Research,%20Lincoln,%20New%20Zealand,%202004.pdf (accessed 08 October 2014). TBfree New Zealand, Wellington, NZ, 2004
- *Byrom AE, Spurr EB, O'Connor CE. Ferret Neophobia to Traps (R-10508). http://www.tbfree.org.nz/Portals/0/2014AugResearchPapers/Byrom%20AE,%20Spurr%20EB,%20O'Connor%20CE.%20Ferret%20neophobia%20to%20traps.pdf (accessed 08 October 2014). TBfree New Zealand, Wellington, NZ, 2002
- *Byrom AE, Spurr EB, Morriss GA, Morse CW, O'Connor CE. R-10520 Trap Catch of Ferrets, Using Live and Dead Prey as Lureso. http://www.tbfree.org.nz/Portals/0/2014AugResearchPapers/Byrom%20AE,%20Spurr%20EB,%20Morriss%20GA,%20Morse%20CW,%20O'Connor%20CE.%20Trap%20catch%20of%20ferrets,%20using%20live%20and%20dead%20prey%20as%20lures.pdf (accessed 08 October 2014) TBfree New Zealand, Wellington, NZ, 2003
- *Byrom AE, Nugent G, Ramsey DSL, Yockney IJ. R-10615 l A New Tb Surveillance Paradigm and Model for Game Estates. http://www.tbfree.org.nz/Portals/0/2014AugResearchPapers/Byrom%20AE,%20Nugent%20G,%20Ramsey%20DSL,%20Yockney%20IJ.%20A%20new%20Tb%20surveillance%20paradigm%20and%20model%20for%20game%20estates.pdf (accessed 08 October 2014). TBfree New Zealand, Wellington, NZ, 2004
- *Byrom A, Nugent G, McKenzie J, Porphyre T, Pouto N, Shepherd J, Whitford J, Yockney I. Animal Health Board Project No. R-80629 Cost-effective Control of Tb in the Northern South Island High Country: Identifying the habitats and vector species requiring control. http://www.tbfree.org.nz/Portals/0/2014AugResearchPapers/Byrom%20A,%20Nugent%20G,%20Yockney%20I,%20Poutu%20N,%20Whitford%20J,%20McKenzie%20J,%20Shepherd%20J,%20Porphye%20T.%20Cost-effective%20control%20of%20Tb%20in%20the%20Northern%20South%20Island%20High%20Country%20(NSIHC).pdf (accessed 08 October 2014). TBfree New Zealand, Wellington, NZ, 2008
- Caley P. Broad-scale possum and ferret correlates of macroscopic Mycobacterium bovis infection in feral ferret populations. New Zealand Veterinary Journal 46, 157–62, 1998
- *Caley P. R-10481 Assessing the Host Status of Feral Ferrets for Mycobacterium bovis in New Zealand. http://www.tbfree.org.nz/Portals/0/2014AugResearchPapers/Caley,%20P.%20Assessing%20the%20host%20status%20of%20feral%20ferrets%20for%20Mycobacterium%20bovis%20in%20New%20Zealand.pdf (accessed 08 October 2014). TBfree New Zealand, Wellington, NZ, 2002
- Caley P, Hone J. Estimating the force of infection; Mycobacterium bovis infection in feral ferrets Mustela furo in New Zealand. Journal of Animal Ecology 71, 44–54, 2002
- Caley P, Hone J. Disease transmission between and within species, and the implications for disease control. Journal of Applied Ecology 41, 94–104, 2004
- Caley P, Hone J. Assessing the host disease status of wildlife and the implications for disease control: Mycobacterium bovis infection in feral ferrets. Journal of Applied Ecology 42, 708–19, 2005
- Caley P, Morriss G. Summer/autumn movements and mortality rates of feral ferrets (Mustela furo) at a farmland site in North Canterbury, New Zealand. New Zealand Journal of Ecology 25, 53–60, 2001
- *Caley P, Morley C, Thomas MD. Effects of Ferret Control on Cattle Reactor Incidence, Ferret TB Prevalence, and Rabbit Numbers- the North Canterbury ferret control trials Final report. http://www.tbfree.org.nz/Portals/0/2014AugResearchPapers/Caley%20P,%20Morley%20C,%20Thomas%20MD.%20Effects%20of%20ferret%20control%20on%20cattle%20reactor%20incidence,%20ferret%20TB%20prevalence,%20and%20rabbit%20numbers.pdf (accessed 08 October 2014). TBfree Zealand, Wellington, NZ, 1998
- Caley P, Hone J, Cowan PE. The relationship between prevalence of Mycobacterium bovis infection in feral ferrets and possum abundance. New Zealand Veterinary Journal 49, 195–200, 2001
- Caley P, McElrea LM, Hone J. Mortality rates of feral ferrets (Mustela furo) in New Zealand. Wildlife Research 29, 323–8, 2002
- Clapperton, BK. Scent lures for trapping ferrets. The Royal Society of New Zealand Miscellaneous Series 36, 74–77, 1996
- *Clapperton BK, Byrom AE. Feral ferret. In: King CM (ed). The Handbook of New Zealand Mammals. 2nd Edtn. Pp 294–307. Melbourne, Oxford University Press, 2005
- Craft ME, Hawthorne PL, Packer C, Dobson AP. Dynamics of a multihost pathogen in a carnivore community. Journal of Animal Ecology 77, 1257–64, 2008
- Cross ML, Smale A, Bettany S, Numata M, Nelson D, Keedwell R, Ragg J. Trap catch as a relative index of ferret abundance in New Zealand pastoral habitat. New Zealand Journal of Zoology 25, 69–71, 1998
- Cross ML, Labes RE, Mackintosh CG. Oral infection of ferrets with virulent Mycobacterium bovis or Mycobacterium avium: susceptibility, pathogenesis and immune response. Journal of Comparative Pathology 123, 15–21, 2000a
- Cross ML, Labes RE, Griffin JF, Mackintosh CG. Systemic but not intra-intestinal vaccination with BCG reduces the severity of tuberculosis infection in ferrets (Mustela furo). International Journal of Tuberculosis and Lung Disease 4, 473–80, 2000b
- Delahay RJ, Smith GC, Barlow AM, Walker N, Harris A, Clifton-Hadley RS, Cheeseman CL. Bovine tuberculosis infection in wild mammals in the South-West region of England: A survey of prevalence and a semi-quantitative assessment of the relative risks to cattle. The Veterinary Journal 173, 287–301, 2007
- de Lisle GW, Crews K, de Zwart J, Jackson R, Knowles GJ, Paterson KD, MacKenzie RW, Waldrup KA, Walker R. Mycobacterium bovis infection in feral ferrets. New Zealand Veterinary Journal 41, 148–9, 1993
- de Lisle GW, Yates GF, Caley P, Corboy RJ. Surveillance of wildlife for Mycobacterium bovis infection using culture of pooled tissue samples from ferrets (Mustela furo). New Zealand Veterinary Journal 53, 14–8, 2005a
- *de Lisle GW, Cannon M, Collins DM. Preliminary investigation on the use of a polymerase chain reaction (PCR) test to detect Mycobacterium bovis in pools of lymph nodes from ferrets without demonstrable evidence of macroscopic tuberculosis. http://www.tbfree.org.nz/Portals/0/R-30654.pdf (accessed 08 October 2014). TBfree New Zealand, Wellington, NZ, 2005b
- de Lisle GW, Kawakami RP, Yates GF, Collins DM. Isolation of Mycobacterium bovis and other mycobacterial species from ferrets and stoats. Veterinary Microbiology 132, 402–7, 2008
- Drewe JA, Foote AK, Sutcliffe RL, Pearce GP. Pathology of Mycobacterium bovis infection in wild meerkats (Suricata suricatta). Journal of Comparative Pathology 140, 12–24, 2009
- *Dymond SJ. Winter use by ferrets (Mustela furo) of vegetation buffer zones surrounding yellow-eyed penguin (Megadyptes antipodes) breeding areas. Diploma of Wildlife Management Research Report, University of Otago, Dunedin, NZ, 1991
- Gallagher J, Clifton-Hadley RS. Tuberculosis in badgers; a review of the disease and its significance for other animals. Research in Veterinary Science 69, 203–17, 2000
- Gavier-Widen, D, Cooke MM, Gallagher J, Chambers MA, Gortazar C. A review of infection of wildlife hosts with Mycobacterium bovis and the diagnostic difficulties of the ‘no visible lesion’ presentation. New Zealand Veterinary Journal 57, 122–31, 2009
- Hammond B. Ferret control on Mandamus Downs. The Royal Society of New Zealand Miscellaneous Series 36, 69–70, 1996
- Heyward RP, Norbury GL. Secondary poisoning of ferrets and cats after 1080 rabbit poisoning. Wildlife Research 26, 75–80, 1999
- *Hutchings S, Hancox N, Livingstone P. Approaches to eradication of tuberculosis from wildlife in New Zealand: a revised pest management strategy. Vetscript 24 (8), 8–12, 2011
- *Krebs CJ. Ecological methodology. 2nd Edtn. Addison-Wesley Publishers, Menlo Park, CA, USA, 1998
- Kristiansen LV, Sunde P, Nachman G, Madsen AB. Mortality and reproductive patterns of wild European polecats Mustela putorius in Denmark. Acta Theriologica 52, 371–8, 2007
- Livingstone P. Overview of the ferret problem. The Royal Society of New Zealand Miscellaneous Series 36, 2–6, 1996
- Livingstone P, Nugent G, de Lisle GW, Hancox N. Toward eradication: the history and impacts of Mycobacterium bovis infection in wildlife on the development of bovine tuberculosis management in New Zealand. New Zealand Veterinary Journal 63 (Suppl. 1), 4–18, 2015
- Lugton IW, Wobeser G, Morris RS, Caley P. A cultural and histopathological study of Mycobacterium bovis infection in feral ferrets in New Zealand. The Royal Society of New Zealand Miscellaneous Series 36, 20–4, 1996
- Lugton IW, Wobeser G, Morris RS, Caley P. Epidemiology of Mycobacterium bovis infection in feral ferrets (Mustela furo) in New Zealand: I. Pathology and diagnosis. New Zealand Veterinary Journal 45, 140–50, 1997a
- Lugton IW, Wobeser G, Morris RS, Caley P. Epidemiology of Mycobacterium bovis infection in feral ferrets (Mustela furo) in New Zealand: II. Routes of infection and excretion. New Zealand Veterinary Journal 45, 151–7, 1997b
- *McAuliffe RJ. The role of scavenging in the spread of bovine tuberculosis in free-living ferret populations in New Zealand. MSc thesis, Lincoln University, Lincoln, NZ, 2001
- *Middlemiss A. Predation of lizards by feral house cats (Felis catus) and ferrets (Mustela furo) in the tussock grassland of Otago. MSc thesis, University of Otago, Dunedin, NZ, 1995
- *Mills RG. Rabbit predators in the semi-arid high country of the South Island of New Zealand. MSc Thesis, Lincoln University, Lincoln, NZ, 1994
- Moller H, Alterio N. Home range and spatial organisation of stoats (Mustela erminea), ferrets (Mustela furo) and feral house cats (Felis catus) on coastal grasslands, Otago Peninsula, New Zealand: Implications for yellow-eyed penguin (Megadyptes antipodes) conservation. New Zealand Journal of Zoology 26, 165–74, 1999
- Moller H, Norbury G, King CM. Ecological and behavioural constraints to effective control of ferrets (Mustela furo). The Royal Society of New Zealand Miscellaneous Series 36, 54–68, 1996
- Moller H, Clapperton BK, Fletcher DJ. Density of rabbits (Oryctolagus cuniculus L.) in the Mackenzie basin, South Island, New Zealand. New Zealand Journal of Ecology 21, 161–7, 1997
- *Moller H, Ragg JR, Bowman R, Hunter M, Reed C. Improved Monitoring and Control of Ferret Abundance for Efficient Bovine Tuberculosis Mitigation. http://www.tbfree.org.nz/Portals/0/2014AugResearchPapers/Moller%20H,%20Ragg%20JR,%20Bowman%20R,%20Hunter%20M,%20Reed%20C.%20%20Improved%20monitoring%20and%20control%20of%20ferret%20abundance%20for%20efficient%20bovine%20tuberculosis%20mitigation.pdf (accessed 08 October 2014). TBfree New Zealand, Wellington, NZ, 2002
- *Morley CG. The ecology and behaviour of feral ferrets (Mustela furo) in Canterbury farmland, New Zealand. PhD thesis, University of Canterbury, Christchurch, NZ, 1999
- Morley CG. Ferret (Mustela furo) abundance, survival and recruitment on farmland in North Canterbury, New Zealand. New Zealand Journal of Zoology 29, 23–31, 2002
- Morris RS, Pfeiffer DU. Directions and issues in bovine tuberculosis epidemiology and control in New Zealand. New Zealand Veterinary Journal 43, 256–65, 1995
- Morris RS, Pfeiffer DU, Jackson R. The epidemiology of Mycobacterium bovis infections. Veterinary Microbiology 40, 153–77, 1994
- *Norbury G, Heyward R. Impact of Rabbit Control on Predator Ecology. http://www.landcareresearch.co.nz/__data/assets/pdf_file/0017/77021/rabbit-control-predator-ecology.pdf (accessed 08 October 2014). Manaaki Whenua - Landcare Research, Alexandra, NZ, 1997
- Norbury GL, Norbury DC, Heyward RP. Space use and denning behaviour of feral ferrets (Mustela furo) and cats (Felis catus). New Zealand Journal of Ecology 22, 149–60, 1998a
- Norbury GL, Norbury DC, Heyward RP. Behavioural responses of two predator species to sudden declines in primary prey. Journal of Wildlife Management 62, 45–58, 1998b
- *Norbury GL, Spencer N, Webster R, Bailey J, Walker R, Wilson R, Hunter M, Reed C. Best-Practice Trapping of Ferret Populations. http://www.tbfree.org.nz/Portals/0/2014AugResearchPapers/Norbury%20GL,%20Spencer%20N,%20Webster%20R,%20Bailey%20J.%20%20Best-practice%20trapping%20of%20ferret%20populations%20.pdf (accessed 08 October). TBfree New Zealand, Wellington, NZ, 2002
- Norbury G, Heyward R, Parkes J. Short-term ecological effects of rabbit haemorrhagic disease in the short-tussock grasslands of the South Island, New Zealand. Wildlife Research 29, 599–604, 2003
- *Norbury GL, Heyward R, McElrea GJ, McElrea LM, Peach R, Byrom AE. Project No. R-10591 Cost-effectiveness of Alternative Ferret Control Practices. http://www.tbfree.org.nz/Portals/0/2014AugResearchPapers/Norbury%20GL,%20Heyward%20R,%20McElrea%20GJ,%20McElrea%20LM,%20Peach%20R,%20Byrom%20AE.%20Cost-effectiveness%20of%20alternative%20ferret%20control%20practices.pdf (accessed 08 October 2014). TBfree New Zealand, Wellington, NZ, 2004
- Nugent G. Maintenance, spillover and spillback transmission of bovine tuberculosis in multi-host wildlife complexes: A New Zealand case study. Veterinary Microbiology 151, 34–42, 2011
- *Nugent G, Whitford J. Animal Health Board Project No. R-10652 Relative Utility of TB Hosts as Sentinels for Detecting TB. http://www.tbfree.org.nz/Portals/0/2014AugResearchPapers/Nugent%20G,%20Whitford%20J.%20Relative%20utility%20of%20Tb%20hosts%20as%20sentinels%20for%20detecting%20Tb.pdf (accessed 08 October 2014). TBfree New Zealand, Wellington, NZ, 2008
- Nugent G, Whitford J, Young N. Use of released pigs as sentinels for Mycobacterium bovis. Journal of Wildlife Diseases 38, 665–77, 2002
- *Nugent G, Byrom A, Arthur T, Whitford J, Yockney I. Project No. R-10576 Risk of TB Persistence and Spread: The Role of Deer, Pigs, and Ferrets. http://www.tbfree.org.nz/Portals/0/2014AugResearchPapers/Nugent%20G,%20Byrom%20A,%20Arthur%20T,%20Whitford%20J,%20Yockney%20I.%20Risk%20of%20Tb%20persistence%20and%20spread%20The%20role%20of%20deer,%20pigs,%20and%20ferrets.pdf (accessed 08 October 2014). Tbfree New Zealand, Wellington, NZ, 2003
- *Nugent G, Ramsey DSL, Caley P. Animal Health Board Project No. R-10627 Enhanced Early Detection of Tb Through Use and Integration of Wildlife Data into the National Surveillance Model. http://www.tbfree.org.nz/Portals/0/2014AugResearchPapers/Nugent%20G,%20Ramsey%20D,%20Caley%20P.%20Enhanced%20early%20detection%20of%20Tb%20through%20use%20and%20integration%20of%20wildlife%20data%20into%20the%20national%20surveillance%20model.pdf (accessed 08 October 2014). TBfree New Zealand, Wellington, NZ, 2006
- Nugent G, Buddle BM, Knowles GJE. Epidemiology and control of Mycobacterium bovis infection in brushtail possums (Trichosurus vulpecula), the primary wildlife host of bovine tuberculosis in New Zealand. New Zealand Veterinary Journal 63 (Suppl. 1), 28–41, 2015a
- Nugent G, Gortazar C, Knowles G. The epidemiology of Mycobacterium bovis in wild deer and feral pigs and their roles in the establishment and spread of bovine tuberculosis in New Zealand wildlife. New Zealand Veterinary Journal 63 (Suppl. 1), 54–67, 2015b
- *O'Hara PJ. Chief Veterinary Officer's Annual Report 1993 - Disease and pest control. Surveillance 21 (3), 10–6, 1993
- Palmer MV, Thacker TC, Waters WR, Gortazar C, Corner LAL. Mycobacterium bovis: a model pathogen at the interface of livestock, wildlife, and humans. Veterinary Medicine International e236205, 2012
- Pech R, Byrom AE, Anderson DP, Thomson C, Coleman M. The effect of poisoned and notional vaccinated buffers on possum (Trichosurus vulpecula) movements: minimising the risk of bovine tuberculosis spread from forest to farmland. Wildlife Research 37, 283–92, 2010
- *Pierce RI. Predators in the MacKenzie Basin: Their diet, population dynamics, impacts in relation to the abundance and availability of their main prey (rabbits). Report for the Wildlife Service, Department of Internal Affairs, Wellington, NZ, 1987
- *Qureshi T, Labes R, Mackintosh CG. Experimental infections of Mycobacterium bovis in ferrets. In: Vaccination as a Means for Control of Bovine Tuberculosis. Royal Society National Science Strategy Publication 80, Wellington, NZ, 1997
- Qureshi T, Labes RE, Cross ML, Griffin JF, Mackintosh CG. Partial protection against oral challenge with Mycobacterium bovis in ferrets (Mustela furo) following oral vaccination with BCG. International Journal of Tuberculosis and Lung Disease 3, 1025–33, 1999
- Qureshi T, Labes RE, Lambeth M, Montgomery H, Griffin JFT, Mackintosh CG. Transmission of Mycobacterium bovis from experimentally infected ferrets to non-infected ferrets (Mustela furo). New Zealand Veterinary Journal 48, 99–104, 2000
- *Ragg JR. Tuberculosis (Mycobacterium bovis) epidemiology and the ecology of ferrets (Mustela furo) on New Zealand farmland. PhD thesis, University of Otago, Dunedin, NZ, 1997
- Ragg JR. Intra-specific and seasonal difference in the diet of feral ferrets (Mustela furo) in a pastoral habitat, East Otago, New Zealand. New Zealand Journal of Ecology 22, 113–9, 1998
- *Ragg JR. Home Range, Movements and Activity Patterns of Female Ferrets: Testing Hypotheses Relating to Low Trappability in Winter and Spring. http://www.tbfree.org.nz/Portals/0/2014AugResearchPapers/Ragg%20JR.%20Home%20range,%20movements%20and%20activity%20patterns%20of%20female%20ferrets%20testing%20hypotheses%20relating%20to%20low%20trappability%20in%20winter%20and%20spring.pdf (accessed 08 October 2014). TBfree New Zealand, Wellington, NZ, 2002
- Ragg JR, Walker R. The association of tuberculous ferrets with infected cattle and deer herds in the South Island. The Royal Society of New Zealand Miscellaneous Series 36, 7–14, 1996
- Ragg JR, Waldrup KA, Moller H. The distribution of gross lesions of tuberculosis caused by Mycobacterium bovis in feral ferrets (Mustela furo) from Otago, New Zealand. New Zealand Veterinary Journal 43, 338–41, 1995a
- Ragg JR, Moller H, Waldrup KA. The prevalence of bovine tuberculosis (Mycobacterium bovis) infections in feral populations of cats (Felis catus), ferrets (Mustela furo) and stoats (Mustela erminea) in Otago and Southland, New Zealand. New Zealand Veterinary Journal 43, 333–7, 1995b
- Ragg JR, Mackintosh CG, Moller H. The scavenging behaviour of ferrets (Mustela furo), feral cats (Felis domesticus), possums (Trichosurus vulpecula), hedgehogs (Erinaceus europaeus) and harrier hawks (Circus approximans) on pastoral farmland in New Zealand: implications for bovine tuberculosis transmission. New Zealand Veterinary Journal 48, 166–75, 2000
- Renwick AR, White PC, Bengis RG. Bovine tuberculosis in southern African wildlife: a multi-species host-pathogen system. Epidemiology and Infection 135, 529–40, 2007
- Sauter CM, Morris RS. Behavioural studies on the potential for direct transmission of tuberculosis from feral ferrets (Mustela furo) and possums (Trichosurus vulpecula) to farmed livestock. New Zealand Veterinary Journal 43, 294–300, 1995
- *Seber GAF. The Estimation of Animal Abundance and Related Parameters. Hafner Press, New York, USA, 1973
- Smith, GP, Ragg JR, Moller H, Waldrup KA. Diet of feral ferrets (Mustela furo) from pastoral habitat in Otago and Southland, New Zealand. New Zealand Journal of Zoology 22, 363–9, 1995
- *Spurr EB, Ogilvie SC, Eason CT, Morse CW, Young J. A Poison-Baiting Strategy for Effective Ferret Control. http://www.tbfree.org.nz/Portals/0/2014AugResearchPapers/Spurr%20EB,%20Ogilvie%20SC,%20Eason%20CT,%20Morse%20CW.%20A%20poison-baiting%20strategy%20for%20effective%20ferret%20control.pdf (accessed 08 October 2014). TBfree New Zealand, Wellington, NZ, 1997
- *Spurr EB, O'Connor CE, Byrom AE. Review of research on improving the effectiveness of ferret control in winter and spring. Proceedings of 12th Australasian Vertebrate Pest Conference. Pp 353–7, Australian Department of Natural Resources and Environment, Melbourne, Victoria, Australia, 2001
- *Thrusfield M. Veterinary Epidemiology. 2nd Edtn. Blackwell Science, Oxford, 1995
- *Walker R, Reid B, Crews K. Bovine tuberculosis in predators in the Mackenzie Basin. Surveillance 20 (2), 11–4, 1993
- Yates GF, Collins DM, MacKenzie RW, Crews KB, Walker R. A study of bovine tuberculosis in domestic animals and wildlife in the MacKenzie Basin and surrounding areas using DNA fingerprinting. New Zealand Veterinary Journal 43, 266–71, 1995
- *Yockney I, Nugent G. Project No. R-10577 Scavenging of Potentially Tuberculosis Feral Pig Carcasses in the Northern South Island High Country. http://www.tbfree.org.nz/Portals/0/2014AugResearchPapers/Yockney%20I,%20Nugent%20G.%20Scavenging%20of%20potentially%20tuberculous%20feral%20pig%20carcasses%20in%20the%20northern%20South%20Island%20high%20country.pdf (accessed 08 October 2014). TBfree New Zealand, Wellington, NZ, 2003
- Yockney IJ, Nugent G, Latham MC, Perry M, Cross ML, Byrom AE. Comparison of ranging behaviour in a multi-species complex of free-ranging hosts of bovine tuberculosis in relation to their use as disease sentinels. Epidemiology and Infection 141, 1407–16, 2013
- *Young JB. Movement patterns of two New Zealand mustelids: Implications for predator pest management. MApplSc thesis, Lincoln University, Canterbury, NZ, 1998