ABSTRACT
Aims
To compare the recovery of lambs, goats, and calves from head-only (HO) or high-frequency head-to-body stunning and evaluate the complementary use of behaviour and electroencephalography (EEG) to assess return to consciousness after electrical stunning in these species.
Methods
Six-month-old lambs, adult goats and calves (< 7 days old) were subjected to reversible head-only stunning (50 Hz, 1 A, 2 seconds) or reversible high-frequency head-to-body stunning (RHTB: HO followed by 2,000 Hz, 2 A, 4-second stun to body). Following stunning, behavioural recovery was assessed in 21 lambs, 22 goats, and 20 calves. Latencies to first perform behaviours (end of convulsions, head lift, attempt to right, successful righting, attempt to stand, successful standing) after stunning were scored from video recordings. Recovery of electrical brain activity indicative of consciousness was assessed using EEG in a separate cohort of minimally-anaesthetised lambs, goats and calves (n = 20 per species). EEG traces collected before and after stunning were classified as normal, epileptiform, isoelectric, or transitional activity. Following stunning, the duration of epileptiform and isoelectric activity combined (states of brain activity incompatible with conscious awareness) was calculated, as was latency to return of normal (pre-stun) EEG.
Results
The RHTB stun was reversible in all three species, although one sheep failed to recover and was euthanised. Both methods caused tonic and clonic convulsions in all species. Behavioural recovery of sheep and calves was similar for both methods while goats took longer to recover from RHTB than HO stunning. There was no evidence of differences between methods in the duration of EEG incompatible with consciousness or the latency to recovery of normal EEG.
Conclusions
Head-to-body stunning as applied here produced a reversible electrical stun in lambs, adult goats and young calves, although the benefits in terms of meat quality and operator safety are uncertain. Goats took longer to recover behaviourally from head-to-body stunning, possibly due to disrupted motor function, but there was no indication that post-stun unconsciousness lasted longer than following head-only stunning in any species. The normal behaviour for the animals’ developmental age should be considered when deciding on behavioural indicators of recovery. The minimal anaesthesia model provided excellent quality EEG data that was valuable for interpretation of the behavioural responses.
Clinical relevance
For the purposes of pre-slaughter stunning of sheep, goats and young calves, recovery appears comparable between the two methods, with all but 1/63 animals in the behaviour study recovering normal function.
Introduction
In New Zealand, livestock animals such as sheep, goats, and cows must be stunned to cause loss of consciousness before slaughter for both standard and halal meat production. Reversible methods of stunning are generally acceptable in halal meat production, on the basis that the animal is capable of recovering normal function following the stun (Farouk Citation2013).
Low-frequency (50 Hz) head-only (HO) electrical stunning is reversible (Blackmore and Newhook Citation1982) and is thus an acceptable method for halal meat production in New Zealand. However, head-only stunning can result in extensive seizure activity that presents a risk to worker safety and the need for further immobilisation as well as potentially causing carcass damage and inferior meat quality (Hillebrand et al. Citation1996; Gregory Citation2005; Simmons et al. Citation2006). To address these problems, the electrical waveform can be passed from the head through the body, inducing spinal inhibition of seizure movement as well as rendering the animal unconscious (Gregory et al. Citation1991).
However, the frequency of the waveform applied in head-to-body stunning influences reversibility and thus suitability for halal meat production. Low-frequency (50 Hz) head-to-body stunning has been shown to cause cardiac arrest and is not reversible (Gregory and Wotton Citation1984; Llonch et al. Citation2015). In contrast, application of a high-frequency waveform (> 300 Hz) from the head through the body does not affect cardiac function (Simmons et al. Citation2001). Thus, high-frequency head-to-body (RHTB) stunning may have acceptable outcomes in terms of reversibility, operator safety, and meat quality compared to head-only stunning. However, there is a need to demonstrate that animals do recover normal function before any new stunning system proposed for halal slaughter in New Zealand can be implemented.
The effects of stunning and subsequent recovery of consciousness can be evaluated using behaviour, neural reflexes, and measures of higher brain activity, and the use of multiple indicators is strongly recommended (Velarde et al. Citation2002; Verhoeven et al. Citation2015). Information about higher brain activity in the context of stunning and slaughter is often collected using the minimal anaesthesia model. Using this model, a light plane of anaesthesia is achieved to keep the animal unconscious but still allow it to demonstrate electroencephalographic (EEG) responses from the cerebral cortex (Murrell and Johnson Citation2006). This approach facilitates evaluation of the loss and/or recovery of normal brain function, reflecting the capacity for awareness, without the experimental animal consciously perceiving any negative experiences associated with that process (e.g. Gibson et al. Citation2009a, Citation2009b; Sabow et al. Citation2017). It also reduces the loss of EEG information due to displacement of recording electrodes or interference in the signal from muscle activity (Gibson et al. Citation2007). However, EEG alone does not provide information on the recovery of behavioural function, which requires stunning and observation of unanaesthetised animals (Verhoeven et al. Citation2015). Thus, the most complete picture of recovery of consciousness following electrical stunning can be gained from measurement of both EEG and behaviour.
A new high frequency head-to-body stunning system was developed in New Zealand by Carne Technologies (Cambridge, NZ) and Jarvis Engineering Technologies NZ (Auckland, NZ) to comply with halal meat production requirements. The system first produces a head-only stun, rendering the animal unconscious, followed by a high frequency head-to-body current. The aims of the present study were to compare the recovery of lambs, goats and calves from head-only or high-frequency head-to-body stunning, and to evaluate the complementary use of behaviour and EEG to assess return to consciousness after electrical stunning in these species.
Materials and methods
The study was carried out at a Massey University research farm (Palmerston North, NZ) in October and November 2014. All procedures were approved by the Massey University Animal Ethics Committee (protocol 14/12) and were conducted in accordance with the Massey University Code of Ethical Conduct for the Use of Live Animals in Research, Testing and Teaching.
Animals
Sheep
Forty-one female Romney-cross lambs (approximately 6 months old) were used, 21 for the behavioural evaluation and 20 for the evaluation of brain electrical activity by EEG. Lambs were delivered the day before the study began and were grazed as a single mob in a nearby paddock.
Goats
Forty-two adult goats of varied sex and breed were used, 22 for the behavioural study and 20 for the EEG study. Goats were delivered the day before the study began and were held overnight in pens inside the research shed and fed concentrated feed.
Calves
Forty bobby (unwanted dairy breed) calves of both sexes and all < 7 days of age were used, 20 in the behaviour study and 20 in the EEG study. Several calves were delivered in a small, covered trailer from a nearby farm (∼ 10 km away) on each morning of the study.
Electrical stunning system and stun application
A VF 2000 stunner (Jarvis Engineering Technologies NZ) was built and installed at Massey University (Supplementary Figure 1) by the manufacturer. This is a small stock stunner that has been developed to provide a head-to-back (immobilising) stun. It first produces a low frequency stun applied only to the head (50 Hz; 1 A, 2 seconds), rendering the animal unconscious (HO stun). When set to RHTB, a 2-second, head-only stun is immediately followed by a high-frequency head-to-body stun (2,000 Hz; 2 A; 4 seconds to the body).
The stunning system was connected to a custom-designed gun-grip applicator with stainless steel electrodes placed on either side of the animal’s head between the lateral canthus of the eye and the external auditory meatus. For animals receiving the RHTB stun, a lead was also clipped to a hind limb (Supplementary Figures 1–4). The applicator had an automatic wetting system for the electrodes, and the device measured the current applied to ensure that the stun delivered an appropriate current for an appropriate duration as indicated above.
Experimental procedures
Sheep and goats were randomly assigned to the behaviour or EEG study by drafting roughly half the animals from the main mob for each study. For calves, the first 20 calves delivered were used in the behaviour study and the rest were used in the EEG study. Both studies were undertaken in an indoor research facility. Within each study, animals were randomly assigned to receive either a low frequency head-only stun or reversible head-to-body high frequency stun, with roughly equal numbers of each treatment on any given day. Randomisation for stunning treatment was achieved by selecting a treatment out of a hat for each animal.
For the behaviour study, this resulted in 11 HO and 11 RHTB goats and 10 HO and 10 RHTB calves. For sheep, it was planned that there would be 10 HO and 11 RHTB animals. However, for three animals assigned to RHTB group the stun was misapplied; it appeared in all cases to be a failure of the body component of the stun. The decision was therefore made to reassign those three sheep to the HO group, and three fewer of the planned HO stuns were thus performed. Three additional sheep were then RHTB-stunned to bring the final number for that group to 11. Thus, there were 11 sheep in the RHTB group and 10 in the HO group (three of which were “failed RHTB” stuns).
For the EEG study, 10 sheep and 10 goats were subjected to each stunning treatment, while 12 calves were HO-stunned and 8 were RHTB-stunned. Animals in the behaviour groups were marked with a unique number using scourable stock spray on the dorsal and lateral aspects of the abdomen for identification on the video recordings.
Behaviour study
On the day of testing, each animal was taken from the holding pen individually and moved into an adjoining room for stun application. Goats and sheep were placed in a restraining cradle hung within an open-sided crate for stunning while calves stood in the crate without the cradle (Supplementary Figures 1–3). Video recording began once the animal was in the crate and continued until the end of the post-stun observation period. The stun was applied as described above, after which the animal was immediately lifted out of the cradle/crate, carried 5 m to an individual solid-sided room approximately 2 m × 2 m, and placed in lateral recumbency (Supplementary Figures 1–3). The animal was left undisturbed for up to 30 minutes while a researcher with a hand-held camera (Handycam Camcorder DCR-SX65; Sony, Tokyo, Japan) recorded from outside the room, and a second camera recorded an overhead view. The observation period was terminated after 30 minutes or after 20 minutes if the animal had stood before that time. The test animal was then moved to another room to fully recover before being returned the mob, which was managed on a Massey University farm thereafter.
EEG study
All stunning procedures and recording of EEG were carried out under general anaesthesia. On the day of testing, each animal was taken from the holding pen individually, weighed and anaesthetised using a mixture of 3.4 mg/kg ketamine (Phoenix Pharm Distributors Ltd., Auckland, NZ) and 4.1 mg/kg propofol (Norbrook NZ Ltd., Tullamarine, VIC, Australia) administered to effect by rapid injection into a jugular vein (Gibson et al. Citation2007). Animals were intubated using a cuffed endotracheal tube, carried into an adjoining room and placed in right lateral recumbency on a stretcher (Supplementary Figure 4) and video recording started. Anaesthesia was maintained using inhalation of halothane in oxygen, delivered via a precision vapouriser and circle breathing system. Animals were allowed to breathe spontaneously throughout the experiment. End-tidal halothane tension was monitored and maintained at 0.9% ± 0.05%. End-tidal carbon dioxide tension, heart rate and respiratory rate were monitored using an anaesthetic agent monitor (Carescape B650; General Electric Healthcare, Chicago, IL, USA).
To collect EEG data, subdermal, 27-gauge, stainless-steel needle electrodes were placed in a three-electrode montage. The non-inverting electrode was placed in the midline between the medial canthi of the eyes, the inverting electrode over the left mastoid process, and the ground electrode caudal to the poll. A base-apex electrode configuration was used to record the electrocardiogram (ECG). Thirty minutes was allowed for equilibration of general anaesthesia and to ensure that the ketamine/propofol did not influence EEG activity (Rault et al. Citation2014), after which a 5-minute pre-treatment EEG trace was recorded. At time zero one of the stunning methods was applied as for the behaviour study. Data were recorded for 30 minutes after stun application.
There was a 60-day withholding period before animals treated with these drugs could enter the food chain; after the recording period, some animals were recovered and rehomed on the understanding that they be kept on-farm for the withholding period. Goats and some calves were rehomed, and sheep were retained on a Massey University farm following recovery. Where this was not possible (some calves), animals were euthanised without recovery from anaesthesia by overdose of pentobarbital (Pentobarb 500; Provet NZ Pty Ltd., Auckland, NZ).
Data collection and statistical analysis
Behaviour
For each species, an ethogram of key behaviours reflecting recovery from electrical stunning was developed following definitions from Velarde et al. (Citation2002) and Verhoeven et al. (Citation2015). The latencies to first perform the following behaviours after stun application were scored from the video recordings: end of convulsions (both tonic and clonic); head lift; attempt to right; successful righting; attempt to stand; successful standing (). Sometimes several behaviours would occur together, e.g. the animal righted or stood on its first attempt. In such cases, both behaviours were assigned the same latency. The scorer was not blinded to the stunning treatment as behaviours were scored from the end of stun application, the method of which could be observed from the videos.
Table 1. Ethogram of key behavioural indicators of recovery of sheep, goats and calves from head-only or reversible head-to-body electrical stunning (definitions taken from Velarde et al. Citation2002; Verhoeven et al. Citation2015).
Behaviour data for each species were analysed separately (Genstat, 18th edition, 2015; VSN International Ltd, Hemel Hempstead, UK). Behaviours that were performed by all animals within the 30-minute observation period were analysed using one-way ANOVA to compare the effect of treatment on latency. Residuals were evaluated for normal distribution by visually inspecting the shape of the Q-Q plots and checking homogeneity of variance for the treatment groups; data were log transformed before analysis when necessary. For these analyses raw means and SE of the differences are presented. Some behaviours were not performed by all animals within the observation period (i.e. their latencies were right-censored) and treatment effects were evaluated using Kaplan–Meier survival analysis. For these behaviours, median survival times and 95% CI are presented. Kaplan–Meier curves were constructed by plotting the survival function for each treatment group (proportion of animals that have not shown the variable of interest at each time point) against time.
EEG measures
Separate amplifiers (Iso-Dam Isolated Biological Amplifier; World Precision Instruments Inc., Sarasota, FL, USA) were used to record EEG and ECG. Signals were amplified with a gain of 1,000 and a bandpass of 1.0–500 Hz and digitised at a rate of 1 kHz (Powerlab 4/20; ADInstruments Ltd., Colorado Springs, CO, USA). The digitised signals were recorded on a personal computer for off-line analysis.
Only EEG data from the right cerebral cortex were analysed. EEG traces were visually inspected and classified into one of four categories: normal, epileptiform, isoelectric and transitional. Normal EEG represented the normal cerebrocortical activity of anaesthetised animals in terms of waveform and amplitude. Epileptiform EEG was classified as a waveform with rhythmic activity of high amplitude and low frequency. Isoelectric EEG was classified as a stable trace consisting of background noise with an amplitude less than 1/8th of the normal pre-stunning EEG with little to no frequency component. Transitional EEG was classified as having amplitude of less than half of the normal pre-stunning EEG with a significant frequency change (Gibson et al. Citation2009a).
For each species, the duration of stunning artefact, epileptiform, and isoelectric activity combined (i.e. states of brain activity incompatible with conscious awareness) after application of the stun was calculated, as was the latency to return of normal EEG. The latency of return to normal EEG was calculated as the period between the start of the stunning artefact and the end of the last period of isoelectric, epileptiform or transitional EEG. Normal EEG returned within 30 minutes of stun application in all sheep and goats, thus the effect of treatment was evaluated using one-way ANOVA. For these analyses, duration data were log transformed to equalise the variance to better meet the normality assumptions of the analysis. Raw means and SED are presented. One calf did not return to normal EEG within 30 minutes; Kaplan–Meier survival analysis was used to evaluate the effect of stunning treatment on this variable, and median (95% CI) survival times are presented along with the Kaplan–Meier curve.
Results
Behaviour
Sheep
The video file for one RHTB sheep was lost, leaving only 20 behaviour datasets for analysis. For three other RHTB sheep, stun characteristics and their immediate behavioural response suggested that the stun was misapplied; these sheep were reassigned to the HO group (10 RHTB, 10 HO including 3 “failed RHTB” stuns). Initial analysis showed the data from the three reassigned sheep were not the same as the other HO sheep, so they were excluded from analysis, leaving 10 RHTB and 7 HO in the final analysis. All sheep showed convulsive activity, both tonic and clonic, after stun application and were observed to perform head-lifting. There was no evidence of a difference in either the duration of convulsions (HO 106 seconds, RHTB 139 seconds (SED 28.7); F = 0.98, p = 0.3) or the latency to head-lift (HO 161 seconds, RHTB 187 seconds (SED 42); F = 0.38, p = 0.55) between stun treatments.
One RHTB sheep did not attempt to right or perform any other behaviours during the 30-minute observation period and was unable to right with assistance shortly afterwards. It displayed dysrhythmic, laboured breathing throughout, remained laterally recumbent for several hours of post-study monitoring and was then euthanised. All HO sheep righted and all but one attempted to stand (15% of data censored for attempt to stand). Five RHTB sheep (50% of data censored) and three HO sheep (43% censored) did not stand within the observation period. There were no statistically significant differences in HO and RHTB sheep’s latencies to attempt to right, right, attempt to stand, or to stand (; ).
Figure 1. Kaplan–Meier graphs of time (seconds) taken for 6-month-old sheep to right (A) or stand (B) following reversible head-only (HO; n = 7) or head-to-body (RHTB; n = 10) stunning with a VF 2000 stunner (Jarvis Engineering Technologies NZ, Auckland, NZ).
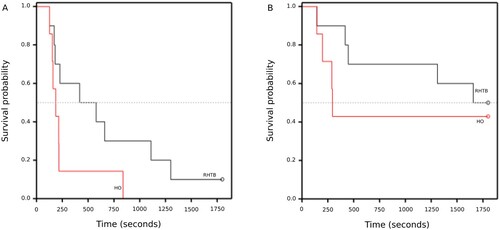
Table 2. Median (95% CI) survival time (seconds) for sheep (approximately 6 months old) to perform behaviours after head-onlyTable Footnotea (HO; n = 7) and reversible head-to-body (RHTB; n = 10) electrical stunningTable Footnotea.
Goats
Three goats were incompletely stunned, so 19 datasets were available for analysis (9 HO, 10 RHTB). All goats showed convulsive activity, both tonic and clonic, after stun application and were observed to perform head-lifting and righting. Goats stunned with RHTB took significantly longer to lift their heads than goats stunned with HO (). Numerically, RHTB goats were also slower to attempt to right and to right but there was large variation within the groups and differences were not significant.
Table 3. Mean latency in seconds for adult goats to perform behaviours after head-only (HO; n = 9) and reversible head-to-body (RHTB; n = 10) electrical stunningTable Footnotea with the SED.
While all HO goats stood within the 30-minute observation period, 3/10 RHTB goats failed to stand and two of these failed even to attempt to stand. Accounting for censored data, goats stunned with HO attempted to stand sooner than did RHTB goats (median survival time: HO 101 (95% CI = 87–184) seconds, RHTB 365 (95% CI = 151–1,280) seconds; log rank statistic = 7.22, p = 0.007; ). Likewise, HO goats stood sooner than those RHTB goats that stood (HO 129 (95% CI = 103–209) seconds, RHTB 1,094 (95% CI = 365 to indeterminate); log rank statistic = 15.77, p < 0.001; ).
Calves
One calf was incompletely stunned, so 19 datasets were available for analysis (9 HO, 10 RHTB). All calves showed convulsive activity, both tonic and clonic, after stun application and were observed to perform head-lifting. There was no evidence of a difference in either the calves’ duration of convulsions (HO 42.8 seconds, RHTB 51 seconds (SED 7.2 seconds); F = 1.29, p = 0.27) or latency to head-lift (HO 101 seconds, RHTB 307 seconds (SED 168 seconds); F = 1.51, p = 0.24) between stun treatments.
Two RHTB calves and one HO calf did not right or even attempt to right during the observation period. There was no evidence of a difference between treatments in the latency to attempt to right (HO 505 (95% CI = 314–1,201) seconds, RHTB 708 (95% CI = 446–1,605) seconds; log rank statistic = 0.29, p = 0.59; ) or to successfully right. The values for attempt to right and right were almost identical so the results and graph are presented only for attempt to right. Most calves achieved sternal recumbence and remained in that posture for the remainder of the observation period. Only two RHTB calves stood, one at 144 seconds after an unsuccessful attempt (119 seconds) and one on its first attempt at 690 seconds. One HO calf attempted to stand at 59 seconds but did not successfully stand during the observation period. No statistical analysis was performed on these variables.
EEG and ECG measures
For all animals of all species, ECG data confirmed that the heart remained in sinus rhythm following the electrical stun (data not shown).
Sheep
Useable EEG datasets were collected from 18 animals: 10 RHTB and 8 HO. There was no evidence of a difference between stunning methods in the duration of isoelectric/epileptiform EEG following stunning or the latency to return to normal EEG ().
Table 4. Mean duration of isoelectric/epileptiform electroencephalogram (EEG) activity and latency to return to normal (pre-stun) EEG of sheep, goats and calvesTable Footnotea following head-only (HO) or head-to-body (RHTB) electrical stunningTable Footnoteb, with the SED.
Goats
All 20 EEG datasets were useable: 10 HO and 10 RHTB. There was no evidence of differences in the duration of isoelectric/epileptiform activity for goats stunned by the two methods or the latency to return to normal EEG ().
Calves
One calf (HO) was excluded because of incorrect placement of EEG electrodes, thus eight RHTB and 11 HO-stunned calves were included in the analyses. There was no evidence of a difference in the duration of epileptiform/isoelectric EEG for calves stunned by the two methods (). One HO calf had not returned to normal EEG by 30 minutes. Accounting for censored data, there was no evidence of a difference between treatments in the latency of calves to return to normal EEG activity (median: HO 554 (95% CI = 521–663) seconds, RHTB 607 (95% CI = 452–681) seconds; log rank statistic = 0.07, p = 0.80; ).
Figure 4. Kaplan–Meier graph of time (seconds) taken for calves (≤ 7 days old) to return to normal electroencephalogram (EEG) activity following reversible head-only (HO; n = 11) or head-to-body (RHTB; n = 8) stunning with a VF 2000 stunner (Jarvis Engineering Technologies NZ, Auckland, NZ).
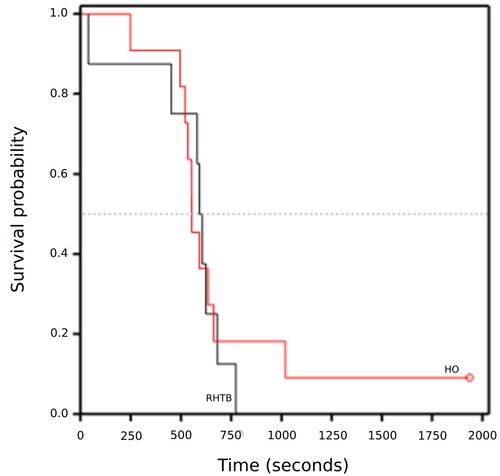
provides a summary of the general progression of recovery behaviours and EEG activity following stunning, generated using data from the two separate groups of animals per species.
Discussion
The main aim of this study was to compare the recovery of slaughter-age sheep, goats and young calves from two methods of electrical stunning: HO stunning and RHTB stunning. All but one of the 123 animals recovered normal brain electrical activity or behaviour indicative of some level of consciousness following stunning, regardless of method. There was some indication that goats recovered more quickly from HO stunning while sheep and calves showed similar recovery from both methods.
One sheep stunned using RHTB failed to show any recovery behaviours following the end of convulsive activity and was unable to maintain sternal recumbence even with assistance at the end of the 30-minute observation period. This animal displayed an abnormal pattern of laboured breathing until it was euthanised several hours after stunning; noisy, laboured breathing was characteristic in sheep recovering from RHTB stunning in this study. It is possible that the electrical current down the spinal cord disrupted motor neurone function in this sheep, leading to impaired diaphragm activity and the inability to control locomotor muscles. While this animal was unable to demonstrate gross behavioural recovery, whether it recovered brain function compatible with awareness is unknown.
In the remaining sheep, the time course of recovery was broadly similar for HO and RHTB stunning. However, while there was no statistically significant difference in the duration of epileptiform and/or isoelectric EEG between the treatment groups, this period ended < 45 seconds after stun application in 5/8 (62.5%) HO-stunned sheep compared with only 2/10 (20%) RHTB-stunned sheep. This interval is consistent with the duration of definite unconsciousness (average 36 seconds) reported by Velarde et al. (Citation2002) in HO stunned lambs, also inferred from EEG data though using different analytical techniques. In the current study, the next shortest duration in either group was nearly 2 minutes after stun application. As these EEG patterns indicate the post-stunning period during which the animal is unequivocally incapable of consciousness (Devine et al. Citation1986), HO-stunned sheep may have been more likely than RHTB-stunned sheep to recover some level of awareness rapidly in the minute following stunning.
Later in the post-stun period, the median times to attempt to stand and to successfully stand, for those sheep that did, were not statistically different. However, the time taken to show these behaviours by RHTB sheep varied widely, ranging from around 2 minutes to more than 21 minutes for sheep that stood spontaneously. In contrast, all HO sheep that stood during the observation period did so in less than 5 minutes, supporting the notion that HO-stunned sheep recovered more quickly. Similarly, while HO sheep appeared to return to normal EEG earlier than RHTB sheep (average 5 vs. 9 minutes), there was wide variability within the groups and the difference was not statistically significant.
In goats, the delay in behavioural recovery following RHTB stunning was pronounced. HO-stunned goats lifted their heads, attempted to stand and stood sooner than did RHTB-stunned goats. However, their EEG recovery was similar. While there was an average of 1.4 minutes of epileptiform/isoelectric EEG following HO-stunning compared to nearly 4 minutes for RHTB goats, the difference was not significant, nor was the time to recovery of normal EEG. Taken together, the evidence suggests that the period of unconsciousness may have been similar for both treatments, while the recovery of behavioural function in RHTB-stunned goats was delayed. The more variable and slower behavioural recovery of RHTB-stunned goats might be explained by different degrees of inhibition of motor function by electrical current travelling down the spinal cord.
Despite the differences in the time course of recovery, all goats and all but one sheep were considered to have recovered normal function by 30 minutes after stunning and cardiac function was preserved in all RHTB goats and sheep. Three of seven (43%) HO-stunned sheep and 5/10 (50%) RHTB-stunned sheep failed to stand spontaneously during the observation period. Likewise, 3/10 (30%) RHTB-stunned goats did not stand. However, all except the one sheep that never recovered could stand when prompted. Likewise, all goats and sheep returned to normal EEG within 30 minutes. Thus, for the purposes of pre-slaughter stunning of sheep, recovery was comparable for the two methods and all but one animal recovered normal function.
Similar to adolescent sheep, calves showed similar recovery from the two methods of stunning, although the behavioural indicators of recovery had to be modified. The duration of convulsions, time to lift the head, attempt to right and right did not differ between methods though there was wide variability within groups in the latencies to display these behaviours. On average, calves convulsed for < 1 minute and finished epileptiform/isoelectric EEG around 2 minutes, indicating the period of unequivocal unconsciousness. In contrast to the sheep and goats, most calves failed to progress through the full suite of recovery behaviours during the 30-minute observation period. Three of 19 calves did not even attempt to right (two RHTB and one HO) and most, once righted, remained in sternal recumbence for the remainder of the time. Only two calves stood, one within minutes of stunning and one much later. This finding likely reflects the difference in the age of the animals and the normal behaviour of the species at this age. Calves < 1 week of age spend most of their time resting in sternal recumbence (Whalin et al. Citation2021) so it may be most appropriate to use righting as the reference point for return to normal behaviour following stunning for these animals.
With the exception of one calf, all animals recovered normal EEG within 30 minutes of stun application, and this calf returned to normal EEG shortly after the end of the official recording period. Thus, while most calves failed to stand, the combined evidence suggests that all calves recovered normal function and that recovery was similar for both methods of stunning.
It is interesting to note that all animals of all species showed periods of both tonic and clonic convulsions following stunning with both methods. It would be useful in future to characterise the intensity of tonic convulsions in particular, to determine whether RHTB stunning using this system provides any advantage over HO stunning in terms of operator safety.
The stun appeared to be misapplied in 5% (calves) to 15% (sheep) of animals conscious at the point of stun application (behaviour study). These rates of mis-stunning are higher than is accepted in commercial slaughter of large mammals in New Zealand (MPI Citation2018) and thus likely represent the method used to restrain the animals and the inexperience of the operator applying the stun. Incomplete or misapplied stuns occurred in both treatments, suggesting that they related to placement of the electrodes on the animals’ heads. Sheep and goats were more often mis-stunned than calves, probably because they were older and more behaviourally active and because they were restrained in a sling that allowed a degree of movement.
The second aim of this study was to evaluate the complementary use of behaviour and EEG for assessing animals’ return to consciousness after electrical stunning. As noted by Verhoeven et al. (Citation2015) in their review of indicators used to assess unconsciousness in livestock: “Studies performed in an experimental set-up have often looked at either the EEG or reflexes and behavioural indicators and there is a scarcity of studies that correlate these different readout parameters”.
In making comparisons between behaviour and electrical brain activity, it is important to acknowledge that these data were collected from different groups of animals. Thus only central tendencies at group level can be compared, and the measures of central tendencies from the appropriate statistical analyses were not the same for all variables (mean vs. median; ). This means that comparison is necessarily qualitative in nature. Nonetheless, some general trends can be discussed.
EEG data were most valuable for determining the period for which animals were unequivocally unconscious following stunning, indicated by the duration of electrical brain activity incompatible with awareness, i.e. epileptiform and isoelectric EEG (Blackmore and Newhook Citation1982). In this early post-stunning period, seizure activity dominated in all species, making it difficult to use behaviour to confidently identify the start of returning consciousness. Previous studies have used behaviours such as focused eye movement, rhythmic breathing and purposeful head-lifting, the first component of righting, to assess return to consciousness in various species (reviewed by Verhoeven et al. Citation2015). In this study, recognising eye focus and differentiating purposeful head lifting from convulsions was difficult, and EEG provided a more reliable indicator of the earliest possible time that consciousness could begin to return.
In addition, the method of stunning was found to influence expression of some behaviours. For example, in sheep, RHTB stunning apparently interfered with the normal rhythm of breathing for quite some time, meaning that this was not a useful indicator of returning consciousness in this species. Likewise, the congruence of behavioural and EEG recovery in the early post-stun period was influenced by stunning method. In HO-stunned goats and sheep, the average time to the end of all “unconscious EEG” was similar to the end of convulsive activity and generally preceded the first attempts to right, meaning that these behaviours could be used to indicate the start of recovery. In contrast, in RHTB-stunned animals, bouts of epileptiform and/or isoelectric EEG activity persisted on average for several minutes after the end of convulsive behaviour and even after apparently purposeful head-lifting. It is possible that behavioural seizure activity was suppressed by neuromotor inhibition due to the head-to-body component of the stun and that what was taken to be purposeful head-lifting was actually a continuation of seizure activity during unconsciousness. As these early behaviours apparently occurred during the period of epileptiform/isoelectric EEG, they are unlikely to be reliable indicators of returning consciousness following this form of electrical stunning.
In young calves, the end of convulsive behaviour tended to precede the end of “unconscious EEG” following both stunning methods. HO-stunned calves lifted their heads around the end of epileptiform/isoelectric EEG while RHTB-stunned calves lay unmoving for several minutes longer on average. The latter likely reflects the neuromotor effects of the head-to-body stun, reinforcing the low value of early behaviour for predicting return to consciousness in RHTB-stunned animals.
Following the end of epileptiform/isoelectric EEG is a period of “transitional” EEG activity, reflecting the progressive return of the pre-stunning amplitude and frequency of cerebrocortical activity (Gibson et al. Citation2009a). The point during this period of transitional EEG at which there is capacity for some level of consciousness is unclear (Johnson et al. Citation2015), making behavioural indicators more useful for assessing recovery during this intermediate period following stunning.
In mature sheep and goats, the first attempts to right occurred around the end of epileptiform/isoelectric EEG or early in the period that transitional EEG was recorded in the anaesthetised cohort. Successful righting and sternal recumbence generally occurred shortly afterwards. HO-stunned goats demonstrated the whole sequence of recovery behaviours through to standing very early in the period during which transitional EEG was likely to have been occurring, while HO-stunned sheep progressed through the behaviours more gradually with some attempting to stand. RHTB-stunned goats also righted and attempted to stand early in the period of transitional EEG but were not likely to have been able to achieve a standing posture until after normal EEG had returned. These observations suggest either that some level of consciousness and thus the capacity for welfare compromise was recovered early in the period of transitional EEG or that “normal” cerebrocortical function, as indicated by EEG activity, is not required for the expression of behaviours such as attempting to right and stand. Alternatively, these behaviours are reflexes generated at lower brain levels and are not reliable indicators of recovery of consciousness in this context. It has long been known that cerebrocortical input is not required for basic postural and locomotor function (Hinsey et al. Citation1930), but recent studies have also demonstrated that the cerebral cortex can influence posture, supporting the value of postural behaviours in determinations of return to consciousness in some circumstances (Takakusaki Citation2017).
Finally, it is important to note that failure to demonstrate behaviours such as righting and standing does not preclude the return of consciousness. In the context of head-to-body stunning, failure to attempt or successfully perform these behaviours is likely to reflect neuromotor impairment rather than delayed return of consciousness. To illustrate, while the median time to first attempt to right in RHTB-stunned sheep occurred around or even before the end of epileptiform/isoelectric EEG, they only achieved righting after the time that normal EEG resumed and didn’t attempt to stand until later. Similarly, RHTB-stunned goats were unable to successfully stand until after the time at which normal EEG returned in the anaesthetised cohort, although they attempted to stand much earlier.
Likewise, the failure of young RHTB-stunned calves to lift their heads until well into the period of transitional EEG and failure to attempt to right until after the return of normal EEG may reflect the interaction between motor inhibition and their developmental immaturity rather than delayed recovery of consciousness. In support, HO-stunned calves lifted their heads around the time that both anaesthetised groups ended epileptform/isoelectric EEG. In addition, most calves failed to progress past sternal recumbence, probably due to the natural behaviour of the species at this young age, rather than failure to recover consciousness. This again highlights the value of complementary measurement of behaviour and EEG in the context of electrical stunning.
Towards the end of the recovery process, EEG was useful for indicating the return of normal (pre-stun) EEG function, the point at which animals would inarguably be fully conscious again. As noted, these EEG data are particularly important when motor inhibition may impair the animal’s ability to express the desired behaviour and particularly in developmentally immature animals. To illustrate, those sheep that stood during the observation period did so around the time full consciousness would have resumed, and all HO-stunned goats were standing already before that point. In contrast, RHTB-stunned sheep only attempted to stand and goats only stood successfully several minutes after resumption of normal EEG, when they would already have been fully conscious. Likewise, HO-stunned calves attempted to right during transitional EEG while RHTB-stunned calves did not even attempt this behaviour until the time they would have been fully conscious. Thus, EEG data are needed to facilitate interpretation of behaviour performed in the early and late stages of recovery, while behaviour is useful to help understand the progressive recovery of consciousness in the transitional phase.
Overall, the findings of this study indicate that the head-to-body stun employed here for sheep, goats and young calves was reversible, with the exception of one sheep that was euthanised for ethical reasons. Stunning with both methods caused both tonic and clonic convulsive behaviour in all species. Thus, the benefits in terms of meat quality and operator safety are uncertain. Behaviourally, RHTB stun delayed recovery of goats relative to HO stunning. However, all animals (except the one noted above) were able to stand when encouraged or assisted (calves) and thus were considered to have recovered fully. In support, all anaesthetised animals recovered normal EEG within or very shortly after the 30-minute recording period. It is important to consider the normal behaviour for the developmental age of the animals when deciding on behavioural indicators of recovery.
As noted above, the use of separate cohorts to measure EEG and our ability to compare the timeline of recovery only at the group level limits interpretation of these data. Future studies could employ intra-cranial electrodes to capture EEG data from behaving animals but the quality of EEG during convulsive activity would likely be compromised. In addition, such studies are invasive and require surgery and recovery for several days before data collection. The use of the minimal anaesthesia model, while presenting some limitations, provided excellent-quality data that was valuable for interpretation of the behavioural responses observed.
Supplemental Material
Download PDF (4.4 MB)Acknowledgements
The Meat Industry Association of New Zealand (MIA, Contract # A20960) funded this project. Frankie Huddart of AgResearch Ltd. and Neil Ward of Massey University provided technical support. Thank you also to Peter Johnstone for statistical support.
Disclosure statement
No potential conflict of interest was reported by the author(s).
References
- Blackmore DK, Newhook JC. Electroencephalographic studies of stunning and slaughter of sheep and calves – Part 3: The duration of insensibility induced by electrical stunning in sheep and calves. Meat Science 7, 19–28, 1982. https://doi.org/10.1016/0309-1740(82)90094-8
- Devine CE, Gilbert KV, Graafhuis AE, Tavener A, Reed H, Leigh P. The effect of electrical stunning and slaughter on the electroencephalogram of sheep. Meat Science 17, 267–81, 1986. https://doi.org/10.1016/0309-1740(86)90045-8
- Farouk MM. Advances in the industrial production of halal and kosher red meat. Meat Science 95, 805–20, 2013. https://doi.org/10.1016/j.meatsci.2013.04.028
- Gibson TJ, Johnson CB, Stafford KJ, Mitchinson SL, Mellor DJ. Validation of the acute electroencephalographic responses of calves to noxious stimulus with scoop dehorning. New Zealand Veterinary Journal 55, 152–7, 2007. https://doi.org/10.1080/00480169.2007.36760
- Gibson TJ, Johnson CB, Murrell JC, Mitchinson SL, Stafford KJ, Mellor DJ. Electroencephalographic responses to concussive non-penetrative captive-bolt stunning in halothane-anaesthetised calves. New Zealand Veterinary Journal 57, 90–5, 2009a. https://doi.org/10.1080/00480169.2009.36884
- Gibson TJ, Johnson CB, Murrell JC, Hulls CM, Mitchinson SL, Stafford KJ, Johnstone A, Mellor DJ. Electroencephalographic responses of halothane-anaesthetised calves to slaughter by ventral-neck incision without prior stunning. New Zealand Veterinary Journal 57, 77–83, 2009b. https://doi.org/10.1080/00480169.2009.36882
- Gregory NG. Recent concerns about stunning and slaughter. Meat Science 70, 481–91, 2005. https://doi.org/10.1016/j.meatsci.2004.06.026
- Gregory NG, Wotton SB. Sheep slaughtering procedures. III. Head-to-back electrical stunning. British Veterinary Journal 140, 570–5, 1984. https://doi.org/10.1016/0007-1935(84)90008-3
- Gregory N, Wilkins L, Wotton S. Effect of electrical stunning frequency on ventricular fibrillation, downgrading and broken bones in broilers, hens and quails. British Veterinary Journal 147, 71–7, 1991. https://doi.org/10.1016/0007-1935(91)90069-Y
- Hillebrand SJW, Lambooy E, Veerkamp CH. The effects of alternative electrical and mechanical stunning methods on hemorrhaging and meat quality of broiler breast and thigh muscles. Poultry Science 75, 664–71, 1996. https://doi.org/10.3382/ps.0750664
- Hinsey JC, Ranson SW, McNattin RF. The role of the hypothalamus and mesencephalon in locomotion. Archives of Neurological Psychiatry 23, 1–43, 1930. https://doi.org/10.1001/archneurpsyc.1930.02220070004001
- Johnson CB, Mellor DJ, Hemsworth PH, Fisher AD. A scientific comment on the welfare of domesticated ruminants slaughtered without stunning. New Zealand Veterinary Journal 63, 58–65, 2015. https://doi.org/10.1080/00480169.2014.964345
- Llonch P, Rodríguez P, Casal N, Carreras R, Muñoz I, Dalmau A, Velarde A. Electrical stunning effectiveness with current levels lower than 1 A in lambs and kid goats. Research in Veterinary Science 98, 154–61, 2015. https://doi.org/10.1016/j.rvsc.2014.12.009
- *MPI. Code of Welfare: Commercial Slaughter. https://www.mpi.govt.nz/dmsdocument/46018-Code-of-Welfare-Commercial-slaughter (accessed 20 May 2024). Ministry for Primary Industries, Wellington, NZ, 2018
- Murrell JC, Johnson CB. Neurophysiological techniques to assess pain in animals. Journal of Veterinary Pharmacology and Therapeutics 29, 325–35, 2006. https://doi.org/10.1111/j.1365-2885.2006.00758.x
- Rault JL, Hemsworth P, Cakebread P, Mellor DJ, Johnson CB. Evaluation of microwave energy as a humane stunning technique based on electroencephalography (EEG) of anesthetized cattle. Animal Welfare 23, 391–400, 2014. https://doi.org/10.7120/09627286.23.4.391
- Sabow AB, Goh YM, Zulkifli I, Sazili AQ, Ab Kadir MZA, Kaka U, Khadija N, Adeyemi KD, Ebrahimi M. Electroencephalographic responses to neck cut and exsanguination in minimally anaesthetized goats. South African Journal of Animal Science 47, 124–34, 2017. https://doi.org/10.4314/sajas.v47i1.6
- *Simmons NJ, Daly CC, Mudford CR, Collinson MM. Modification of Pig Stunning Procedures to Improve Meat Quality. AgResearch, Hamilton, NZ, 2001
- Simmons NJ, Daly CC, Mudford CR, Richards I, Jarvis G, Pleiter H. Integrated technologies to enhance meat quality – an Australasian perspective. Meat Science 74, 172–9, 2006. https://doi.org/10.1016/j.meatsci.2006.05.007
- Takakusaki K. Functional neuroanatomy for posture and gait control. Journal of Movement Disorders 10, 1–17, 2017. https://doi.org/10.14802/jmd.16062
- Velarde A, Ruiz-de-la-Torre JL, Roselló C, Fàbrega E, Diestre A, Manteca X. Assessment of return to consciousness after electrical stunning in lambs. Animal Welfare 11, 333–41, 2002. https://doi.org/10.1017/S096272860002491X
- Verhoeven M, Gerritzen M, Hellebrekers L, Kemp B. Indicators used in livestock to assess unconsciousness after stunning: a review. Animal 9, 320–30, 2015. https://doi.org/10.1017/S1751731114002596
- Whalin L, Weary DM, von Keyserlingk MAG. Understanding behavioural development of calves in natural settings to inform calf management. Animals 11, 2446, 2021. https://doi.org/10.3390/ani11082446
- *Non-peer-reviewed