Abstract
Vatiquinone is a potent inhibitor of 15-lipoxygenase and is in clinical development for the treatment of mitochondrial diseases and other disorders characterised by high levels of oxidative stress and dysregulation of energy metabolism.
In rats, 14C-vatiquinone-derived radioactivity was quickly and widely distributed throughout the body and cleared from most tissues by 24 h post-dose following a single oral dose of 14C-vatiquinone.
Following oral administration, 94% of dose was recovered within seven days in rats, approximately 61% of dose was recovered within seven days in dogs and approximately 93% of dose was recovered within nine days in human subjects (IND 119220). Faecal excretion was the major route (>56% dose) in all species; urinary excretion was minimal in rats and dogs (<3% dose) but was higher in humans (∼ 22% dose).
Following oral administration, vatiquinone was the dominant circulating component in rats and dogs but was minor in human subjects. There were no plasma metabolites that were more than 10% of total drug related exposures in all species.
Following oral administration, vatiquinone was not detectable in urine but was the most prominent component in faeces in rats, dogs, and humans.
Introduction
Vatiquinone, also known as PTC743 or EPI-743 (), is an orally bioavailable small molecule being developed for the treatment of mitochondrial diseases and other disorders characterised by high levels of oxidative stress and dysregulation of energy metabolism. Vatiquinone is the quinone oxidation product of alpha-tocotrienol, one of the eight naturally occurring forms of vitamin E, and is a member of the para-benzoquinone class of drugs (Hinman et al. Citation2018). Vatiquinone is a potent inhibitor of 15-lipoxygenase (15-LO), an oxidoreductase enzyme that governs the process of inflammation, oxidative stress, glutathione (GSH) depletion and cell death, also known as ferroptosis. The activity of 15-LO is particularly enhanced in patients with mitochondrial diseases and oxidative stress, leading to depletion of endogenous antioxidants such as GSH (Atkuri et al. Citation2009; Pastore et al. Citation2013). In clinical studies, vatiquinone was found to increase GSH levels and decrease the ratio of oxidised GSH to reduced GSH (i.e. improve redox charge ratio); these changes in GSH levels correlated with clinical improvement in disease symptoms, and arrest or reversal of disease progression (Blankenberg et al. Citation2012; Enns et al. Citation2012; Martinelli et al. Citation2012; Pastore et al. Citation2013). Vatiquinone has been studied extensively in clinical settings (Shrader et al. Citation2011; Martinelli et al. Citation2012; Sadun et al. Citation2012; Pastore et al. Citation2013; Zesiewicz et al. Citation2018). More than 600 patients have received vatiquinone in doses ranging from 50 mg twice a day to 400 mg three times a day (TID). Clinical data support a favourable safety profile of vatiquinone with no dose-limiting toxicities reported to date.
Figure 1. Structure of vatiquinone and metabolic pathways following oral dose administration in rats, dogs, and human subjects.
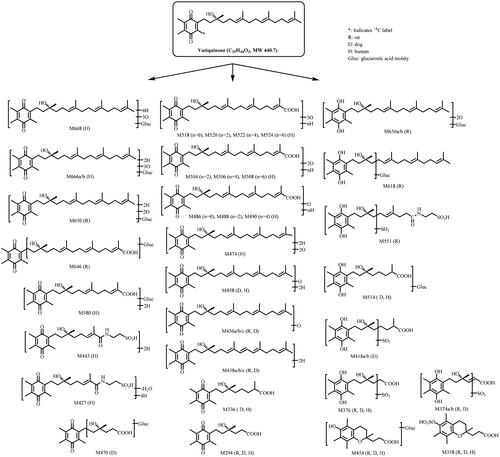
In vitro and in vivo drug metabolism and pharmacokinetic studies have been conducted in support of vatiquinone clinical development. Plasma protein binding of vatiquinone was high (>96%) in mouse, rat, rabbit, dog, monkey, and human plasma; metabolism of vatiquinone was more extensive in rabbit, monkey, and human hepatocytes than in dog, rat, and mouse hepatocytes; in vitro and clinical studies have indicated that CYP3A4 is the major enzyme involved in vatiquinone metabolism in human (PTC internal data). Although vatiquinone inhibited CYP2C9, CYP2C19 and drug transporter breast cancer resistance protein (BCRP) activity in vitro, clinical studies demonstrated that vatiquinone has no clinically meaningful effect on the pharmacokinetics of tolbutamide, omeprazole, and rosuvastatin, substrates of CYP2C9, CYP2C19, and BCRP, respectively (Murase et al. Citation2022; Lee et al. Citation2023).
The objectives of this study were to evaluate absorption, distribution, metabolism, and excretion (ADME) of vatiquinone in rats, dogs, and human healthy subjects following a single oral dose of 14C-vatiquinone. Rats and dogs were used for such studies because these species were also used for vatiquinone toxicology evaluation. Studies using live animals are essential for ADME property evaluation and are required by regulatory agencies worldwide for investigational drugs as no acceptable non-live animal models are available. The number of animal and human subjects used in these studies is the minimum required to obtain scientifically valid results. The results from these studies are essential to understand ADME properties of vatiquinone in animals and humans and are required prior to the conduct of other clinical studies.
Materials and methods
Materials
Non-GMP 14C-vatiquinone (52 µCi/mg, radiochemical purity 99.6% and chemical purity 98%) used for nonclinical studies and GMP 14C-vatiquinone (20 µCi/mg, radiochemical purity 99.4% and chemical purity 95%) used for clinical study were synthesised by Pharmaron (Cardiff, UK). Non-labelled vatiquinone (chemical purity 93.6%) was manufactured by Siegfried (Evionnaz, Switzerland). Vatiquinone is light sensitive, therefore vatiquinone and all related samples were handled and processed under yellow lights or reduced lighting.
Methods
ADME studies in laboratory animals and human subjects
All animal study protocols (including objectives, study design, and analysis plan etc.) employed were ethically reviewed and approved by PTC Therapeutics, Inc. before the study conducts. All animal facilities used were fully accredited by the Association for Assessment and Accreditation of Laboratory Animal Care International, and all animal studies were conducted after approval by the Institutional Animal Care and Use Committee and were in compliance with the US National Research Council’s Guide for the Care and Use of Laboratory Animals, the US Public Health Service’s Policy on Humane Care and Use of Laboratory Animals, and Guide for the Care and Use of Laboratory Animals.
The human study has been conducted in accordance with the Declaration of Helsinki and the International Council for Harmonisation Good Clinical Practice (ICH GCP). The Institutional Review Board reviewed and approved the clinical study protocol, any clinical study protocol amendments, subject information sheets, written informed consent forms, and other relevant documentation. Prior to participation in the study, each subject was apprised of the nature and purpose of the study, and the informed consent form was obtained.
Tissue distribution study in rats following a single oral dose of 14C-vatiquinone
Eleven intact male Long-Evans rats, approximately six weeks old, 205 to 225 g of body weights, were used in this study. Each animal was uniquely identified by a tail tattoo displaying a permanent animal number on their tails. A cage card was affixed to each cage displaying the permanent animal numbers. All animals were housed ≤5 per cage in polycarbonate shoebox cage containing hardwood shavings and were acclimated for approximately five days in an environmentally monitored room. Environmental conditions such as temperature, humidity, and lighting etc. for the animal room were monitored, and the 12 h (h) dark cycle was maintained at extent possible throughout the study. One day prior to and during the in-life testing period animals were housed individually in polycarbonate shoebox cages with stainless steel wire flooring inserts. Animals received Certified Rodent Diet #5002 (PMI Nutrition International, Inc., St. Louis, MO, USA) ad libitum. Fresh tap water was available ad libitum via bottles attached to the cages throughout the study. The animals were examined daily for any signs of abnormalities indicative of health problems. Only animals observed to be healthy were placed on the study. On the day of dosing, each animal was administered a single oral gavage dose of 14C-vatiquinone at 30 mg/kg (100 μCi/kg). At 1, 2, 4, 8, 24, 48, 72, 168, and 504 h post-dose, one animal per time point was anaesthetised by inhalation of isoflurane at designated time points post-dose, followed by blood collection via cardiac puncture and euthanasia by isoflurane overdose. Each carcase was then frozen in a dry ice/hexane bath. Blood and plasma samples were assayed for total radioactivity (TRA) concentrations by liquid scintillation counting (LSC). The tissue concentrations of TRA were determined using quantitative whole-body autoradiography (QWBA) techniques. Briefly, sagittal 30 µm thick sections of the carboxymethylcellulose (CMC)-embedded rat carcases, including quality control (QC) standards, and the CMC-embedded calibration standards, were sectioned using a Leica 9800 Cryomicrotome at −20 °C. The sections were dehydrated for at least 24 h in the Cryomicrotome chamber at approximately −20 °C and then transferred to a desiccant storage box for at least 1 h. The sections were removed from their frames, mounted on a support cardboard backing, labelled with radioactive ink, and the sections were wrapped with plastic wrap. Whole body sections, containing their QC standards, were placed in phosphor imaging plate (IP) cassettes along with a section containing blood calibration standards. The IPs were exposed to the sections for approximately four days at room temperature in a copper-lined lead shielded box. Autoradioluminograms were generated in a darkroom using a GE Typhoon FLA 9500 Phosphor Imager and analysed using AIDA software. The radioactivity concentrations in selected tissues were determined by digital analysis of the photo-stimulated light/unit area on each autoradioluminogram. A calibration curve was established for each IP and the concentrations of radioactivity in tissues were back calculated and expressed in µg eq/g tissue.
Absorption, metabolism, and excretion (AME) in rats following a single oral dose of 14C-vatiquinone
Intact male Sprague Dawley (SD) rats and bile-duct cannulated (BDC) male SD rats, approximately seven weeks old, 245 to 292 g of body weight, were used in this study. Each animal was uniquely identified by a permanent tail tattoo number. All animals were acclimated for five days. The intact animals were housed ≤5 per cage in solid bottom polycarbonate holding cages with bedding. BDC rats were individually housed in solid bottom polycarbonate holding cages with bedding. Animals were placed in the testing cages one day prior to dosing and during the in-life testing period. Intact rats for mass balance were housed individually in stainless steel metabolism cages equipped for the separate collection of urine and faeces. BDC rats were housed individually in Nalgene-type metabolism cages, equipped for the separate collection of urine, faeces, and bile. Animals for blood/plasma PK were housed ≤3 per cage in stainless steel wire mesh bottom holding cages. All animals received Certified Rodent Diet #5002 (PMI Nutrition International, Inc., St. Louis, MO, USA) ad libitum. Fresh tap water was available ad libitum via bottles attached to the cages throughout the study. Environmental conditions such as temperature, humidity, and lighting etc. for the animal room were monitored, and the 12 h dark cycle was maintained at extent possible throughout the study. The animals were examined daily for any signs of abnormalities indicative of health problems. Only animals observed to be healthy were placed on the study. On the day of dosing, each animal was administered a single oral gavage dose of 14C-vatiquinone at 300 mg/kg (100 μCi/kg). For mass balance in intact rats (n = 3), urine and faeces were collected at 0–8, 8–24 (0–24 for faeces), and every 24 h up to 168 h post-dose. For mass balance in BDC rats (n = 3), urine and faeces were collected at 0–8, 8–24, (0–24 for faeces), 24–48, 48–72 h post-dose, and bile was collected at 0–4, 4–8, 8–24, 24–48, and 48–72 h post-dose. Bile, urine and faecal samples were stored at approximately −70 °C before analysis. For plasma pharmacokinetics in intact rats, blood samples were collected at 0, 1, 2, 4, 6, 8, 12, 24, 48, 96, and 168 h post-dose (n = 3/timepoint) into syringes containing K2EDTA. Blood samples were centrifuged at approximately 4 °C for 10 min at 1372 g to isolate plasma. All samples were stored at approximately −70 °C before analysis.
AME in dogs following a single oral dose of 14C-vatiquinone
Three male non-naive beagle dogs, approximately 10 to 11 months of age and 10.2 to 11.1 kg of body weights, were used in this study. Each dog was identified by a distinct ear tattoo and a correlated permanent animal number. All animals were housed individually in clean, stainless-steel cages in a study-dedicated, environmentally controlled room. Individual cage cards were affixed to each cage and displayed at least the animal number, tattoo number, group number, and study number. All dogs were given regular opportunity for exercise and socialisation in accordance with the Animal Welfare Act, except during the course of the study. Approximately 300 grams of Certified Canine LabDiet 5007 (PMI Nutrition International, Inc., St. Louis, MO, USA) was offered once daily. Reverse osmosis-treated water was available ad libitum. Chew toys were also given during the study. Environmental conditions such as temperature, humidity, and lighting etc. for the animal room were monitored, and the 12 h dark cycle was maintained at extent possible throughout the study. One day prior to dosing, animals were weighed, and animals judged to be suitable for testing were assigned to the treatment group at random using a computer program based on the most recent animal weights. Only dogs observed to be healthy were placed on the study. Veterinary care was available throughout the course of the study and animals were examined by the veterinary staff as warranted by clinical signs or other changes. On the day of dosing, three dogs were each administered a single oral dose of 14C-vatiquinone at 100 mg/kg (100 μCi/kg). Blood samples were collected from the cephalic vein into K2EDTA tubes at 0, 1, 2, 4, 6, 8, 12, 24, 48, 96, and 168 h post-dose. Blood samples were centrifuged at 3000 g and 4 °C for 15 min to obtain plasma. Blood subsamples were stored at −20 °C and plasma samples were stored at −70 °C until analysis. Urine, faeces, cage rinse and cage wash were collected at 0–8, 8–24 (0–24 for faeces), and every 24 h up to 168 h post-dose. All urine, faeces and cage rinse samples were stored at −20 °C until analysis.
Following oral dose administration, animals were observed for general health and mortality. At the completion of the study, the dogs were healthy and returned to the colony.
AME in human subjects following a single oral dose of 14C-vatiquinone
A phase I, open-label study to investigate the absorption, metabolism, and excretion of 14C-vatiquinone (PTC743-NEU-004-FA, IND 119220) was conducted at Labcorp Early Development Laboratories Inc. (Madison, WI, USA). A total of eight healthy male subjects, three Black or African Americans, three whites, one American Indian or Alaska native, and one Asian, participated in the study. The mean ± SD age was 41.3 ± 11.32 years, body weight was 81.18 ± 11.632 kg, and body mass index was 26.36 ± 2.834 kg/m2.
On Days 1 to 6, each subject received 200 mg or 400 mg TID oral dose of vatiquinone based on a 6–6–12h interval that corresponded to the timing of breakfast, lunch, and dinner. All subjects consumed a medium-fat meal (approximately 25% to 50% of calories from fat) prior to each dosing. Plasma was collected on Day 1 and Day 6 for vatiquinone pharmacokinetics evaluation and the study details will be reported elsewhere. On Day 7, each subject received a single 400 mg (100 µCi) oral dose of 14C-vatiquinone with approximately 240 mL of water after a medium-fat meal (approximately 25% to 50% of calories from fat) prior to dosing. Blood samples were collected at 0, 1, 2, 3, 4, 6, 7, 8, 10, 12, 14, 16, 24, 36, 48, and at 24 h intervals thereafter, and urine and faecal samples were collected continuously over 24 h intervals until the subjects meet the discharge criteria (≥90% of the dose recovery or ≤1% of the dose in combined urine and faeces in 2 consecutive 24 h collection intervals). All plasma, urine and faecal samples were stored at −70 °C before analysis.
Human dosimetry was calculated based on rat QWBA study. The overall whole-body radiation dose in a male human subject after administration of a single 100 μCi oral dose of 14C-vatiquinone was projected to be 2.76 millirem. This value is well below the FDA exposure limit of 3000 millirem after a single dose for human radioisotope studies.
Sample preparation for TRA analysis
Aliquots of plasma, urine, bile, and cage rinse/wash samples were taken for direct LSC. Faecal samples were homogenised with isopropyl alcohol/purified water; aliquots of faecal homogenates and blood samples were airdried at room temperature and combusted in a biological sample oxidiser followed by direct LSC.
Sample preparation for metabolite profiling and identification
Rat AME study samples
Plasma samples at 1, 2, 4, 6, 8, 12, and 24 h were selected for radio-profiling. An equal volume (approximately 1 to 1.5 mL) of each selected plasma sample was pooled by time-points across animals. The pooled plasma samples were extracted with acetonitrile (3×, v/v) for three times. The acetonitrile extracts were combined and evaporated to dryness under the nitrogen stream. The residues were reconstituted in tetrahydrofuran for radio-profiling. An equal percentage by volume of individual bile samples at 0–48 h from male BDC rats were pooled by time intervals across animals. A portion of the pooled bile sample was centrifuged at approximately 10 000 g at 4 °C for 10 min. The supernatants were used for radio-profiling. An equal percentage by volume of individual urine samples at 0–48 h from intact rats and 0–72 h from BDC rats was pooled by group and time intervals across animals, respectively. A portion of the pooled urine sample was centrifuged at 10 000 g at 4 °C for 10 min. The supernatants were used for radio-profiling. An equal percentage by weight of individual faecal samples at 0–48 h from intact rats and BDC rats was pooled by groups and time intervals across animals, respectively. An aliquot of the pooled faecal homogenate was extracted three times with acetonitrile (3×, v/w). The acetonitrile extracts were combined and evaporated to dryness under the nitrogen stream. The residues were reconstituted with methanol:water (1:1) (v/v) for radio-profiling.
Dog AME study samples
Plasma from each animal at 1, 2, 4, 6, 12, 24, 48, and 96 h were selected and pooled with the same volume by the time points across animals. Each pooled plasma was extracted with acetonitrile (3×, v/v) for three times. The acetonitrile extracts were combined, evaporated into dryness under nitrogen stream and the residues were reconstituted with methanol:water (1:1, v/v) for radio-profiling. An equal percentage sample volume of individual urine samples at 0–144 h were pooled across time intervals and animals to obtain a pooled 0–144 h urine sample for metabolite radio-profiling. The pooled urine was centrifuged at 10 000 g at 4 °C for 10 min and the supernatant was used for radio-profiling. An equal percentage sample weight of individual faecal samples at 0–48 h were pooled across time intervals and animals. The pooled faecal homogenate was extracted three times with acetonitrile (3×, v/w), the acetonitrile extracts were combined and evaporated to dryness under the nitrogen stream. The residues were reconstituted with tetrahydrofuran for radio-profiling.
Human study samples
Following 400 mg (100 µCi) 14C-vatiquinone dose, plasma from each subject at 2, 3, 4, 5, 6, 8, 10, 24, 48, and 96 h were selected and pooled with the same volume by the time points across subjects. Each pooled plasma was extracted with acetonitrile (3×, v/v) for three times. The acetonitrile extracts were combined, evaporated into dryness under nitrogen stream and the residues were reconstituted with methanol:water (1:1, v/v) for radio-profiling. An equal percentage sample volume of individual 0–96 h urine from all 8 subjects were combined to prepare 0–24 and 24–96 h pooled urine samples. The pooled urine was loaded to a Waters Oasis 35CC HLB cartridge conditioned with 12 mL of ethanol, followed by 36 mL of water. The cartridge was washed with 8 mL of water followed by 24 mL of ethanol. The ethanol elute fractions were evaporated to dryness under the nitrogen stream and the residues were reconstituted with ethanol:water (1:1, v/v) for radio-profiling. An equal percentage sample weight of individual 0–168 h faecal samples were pooled across subjects to prepare 0–72 and 72–168 h pooled faecal samples. The pooled faecal homogenate was extracted three times with acetonitrile (3×, v/w). The acetonitrile extracts were combined and evaporated to dryness under the nitrogen stream. The residues were reconstituted with ethanol:water (1:1) (v/v) for radio-profiling.
Metabolite radio-profiling and identification
The metabolite profiles were determined by high performance liquid chromatography (HPLC) radio-chromatography using a Waters 2695 or Shimadzu Nexera LC-30AD system. An ACE Excel 3 C18 column, 3 μm, 150 × 4.6 mm, maintained at 40 °C, and two solvent systems of 10 mM ammonium acetate in water (A) and acetonitrile (B) were used. The flow rate was 0.7 mL/min and the linear gradients were: 0% B for 2 min; 0 to 5% B in 0.1 min; 5 to 37% B in 57.9 min; 37 to 70% B in 12 min; 70 to 95% B in 3 min; 95 to 100% B in 12 min; hold 100% B for 3 min; 100 to 0% B in 1 min and hold 0% B for 15 min. HPLC fractions were collected by time (15 s/fraction) into Deepwell LumaPlateTM 96 plates. The plates were subsequently dried by a SpeedVac concentrator for up to 8 h. The radioactivity in each fraction was determined by Packard TopCount NXTTM Microplate Scintillation and Luminescence Counter technology. HPLC radio-chromatograms were reconstructed, and the radioactivity peaks were integrated to determine the percentage distribution of individual radioactivity peaks or regions in each sample. Plasma, bile, urine and faecal metabolites were analysed by LC-MS/MS using a Thermo Q Extractive mass spectrometer (Thermo Fisher Scientific, Waltham, MA, USA). The Q Extractive was equipped with an electrospray ionisation source operated in positive or negative ion mode with a capillary temperature of 300 °C and spray voltage of 3.5 kV. The sheath gas, auxiliary gas, and sweep gas pressure was 50, 40, and 10 units, respectively.
Pharmacokinetic analysis
Pharmacokinetic (PK) data for TRA, vatiquinone and its metabolites were generated by non-compartmental PK analysis using Phoenix WinNonlin (Version 8.1 or higher. Certara, Princeton, NJ, USA). The following parameters were calculated to the extent possible: maximum concentration (Cmax), time of Cmax (Tmax), terminal half-life (T1/2) and area under the concentration versus time curve (AUC).
Results
Tissue distribution in rats following a single oral 14C-vatiquinone dose
A single 30 mg/kg (100 μCi/kg) oral dose of 14C-vatiquinone in male Long-Evans rats was well tolerated. The animals appeared to be normal throughout the study and demonstrated no clinical signs from the dose administration.
Following a single 30 mg/kg oral dose of 14C-vatiquinone, both blood and plasma TRA peaked at 2 h. Plasma TRA decreased steadily after Tmax and was below the quantifiable limit (BQL or <0.0441 µg eq/g) by 48 h post-dose. Blood TRA was slightly lower than plasma TRA for all time points post-dose and was BQL (<0.0901 µg eq/g) by 24 h post-dose. 14C-vatiquinone-derived radioactivity was widely distributed throughout the body with most of tissues showing the highest concentrations at 4 h after the dosing, and quickly cleared from most tissues. At 24 h post-dose, most tissues had concentrations BQL (<0.179 µg eq/g). The endocrine, metabolic/excretory, and fatty tissues, as well as the tissues of the gastrointestinal tract contained the highest distribution of 14C-vatiquinone-derived radioactivity. There was no significant retention of radioactivity in certain melanin containing tissues, such as the pigmented skin and uveal tract of the eye in the Long-Evans rats through 504 h post-dose. The concentration in the non-pigmented skin was similar when compared with the pigmented skin. PK parameters of 14C-vatiquinone-derived radioactivity in plasma and tissues are summarised in .
Table 1. Pharmacokinetic parameters of 14C-vatiquinone-derived radioactivity in plasma and tissues following a 30 mg/kg 14C-vatiquinone oral dose in male Long-Evans rats.
AME in rats following a single oral 14C-vatiquinone dose
A single 14C-vatiquinone oral dose at 300 mg/kg (100 μCi/kg) in SD rats was well tolerated. The animals appeared to be normal throughout the study and demonstrated no clinical signs from the dose administration.
Following a single 14C-vatiquinone oral dose at 300 mg/kg in rats, the mass balance data are summarised in . Plasma PK parameters and distribution data of vatiquinone and its metabolites are listed in and , respectively. The representative metabolite profiles are shown in and . The plasma concentration-time curves of TRA, vatiquinone and its prominent metabolites are shown in . Plasma and faeces extraction efficiency and reconstitution recovery ranged from 88 to 101% and the column recovery ranged from 91 to 117%.
Figure 2. Representative HPLC radio-chromatograms of 2 h (A), 4 h (B), 8 h (C), 12 h (D), and 24 h (E) plasma from intact rats following a single 300 mg/kg oral dose of 14C-vatiquinone.
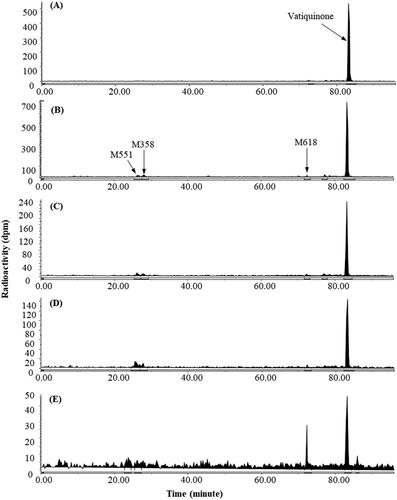
Figure 3. Representative HPLC radio-chromatograms 0–48 h bile (A), 0–72 h urine (B), and 0–48 h faeces (C) from bile-duct cannulated rats, and 0–48 h urine (D) and 0–48 h faeces (E) from intact rats following a single 300 mg/kg oral dose of 14C-vatiquinone.
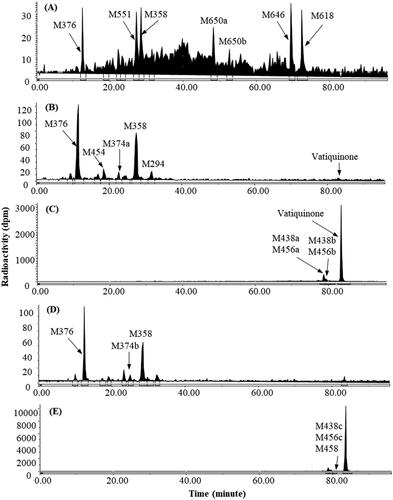
Table 2. Percentage of the administered dose recovered following a single oral dose of 14C-vatiquinone in male Sprague Dawley rats, male Beagle dogs, and male human subjects.
Table 3. Pharmacokinetic parameters for plasma metabolites following a single oral dose of 14C-vatiquinone in male Sprague Dawley rats, male Beagle dogs, and male human subjects.
Table 4. Percentage of dose excreted as vatiquinone and metabolites in pooled bile, urine, and faeces following a single oral dose of 14C-vatiquinone in male Sprague Dawley rats, male Beagle dogs, and male human subjects.
In intact male rats, approximately 95% of the 14C-vatiquinone-derived radioactivity was excreted by 168 h with close to 89% excreted within 24 h post-dose, and the main route of excretion was through faeces with less than 3% in urine. For the male BDC rats, approximately 94% of the 14C-vatiquinone-derived radioactivity was excreted by 72 h with more than 89% excreted within 48 h post-dose, and the main route of excretion was through faeces with minor excretion in bile and urine. The mean Cmax values of 10.2 µg eq/mL in plasma and 6.40 µg eq/mL in blood of 14C-vatiquinone-derived radioactivity were both observed at 4 h post-dose. Plasma TRA decreased quickly after Tmax and was above BQL (0.529 µg eq/mL) by 48 h post dose. Blood concentrations were lower than plasma concentrations at all time points post-dose and were above BQL (0.951 µg eq/mL) by 24 h post-dose. The blood:plasma concentration ratios from 1 h to 12 h post-dose ranged from 0.59 to 0.69.
Vatiquinone plasma concentration peaked at 4 h, was the dominant circulating radioactive component in intact rats. A few minor metabolites, each accounting for less than 8% of 0–24 h plasma TRA, were detected as circulating metabolites. Vatiquinone was not detectable or negligible in urine and bile but was the most abundant radioactive component in faeces from both intact and BDC rats following oral dose.
AME in dogs following a single oral 14C-vatiquinone dose
A single oral dose of 14C-vatiquinone at 100 mg/kg to male beagle dogs was well tolerated. The animals appeared to be normal throughout the study and demonstrated no clinical signs from the dose administration.
Following a single oral dose of 14C-vatiquinone in dogs, the radioactivities recovered in urine, faeces, and cage rinse/wash are summarised in , plasma PK parameters and distribution data of vatiquinone and its metabolites are listed in and , respectively. The representative metabolite profiles are shown in , and the plasma concentration-time curves of TRA, vatiquinone and its prominent metabolites are shown in . Plasma and faeces extraction efficiency and reconstitution recovery ranged from 91 to 103% and the column recovery ranged from 83 to 110%.
Figure 4. Representative HPLC radio-chromatograms of 1 h (A), 2 h (B), 4 h (C), 6 h (D), 12 h (E), 24 h (F), 48 h (G), and 96 h (H) plasma, 0–144 h urine (I) and 0–48 h faeces (J) from dogs following a single 100 mg/kg oral dose of 14C-vatiquinone.
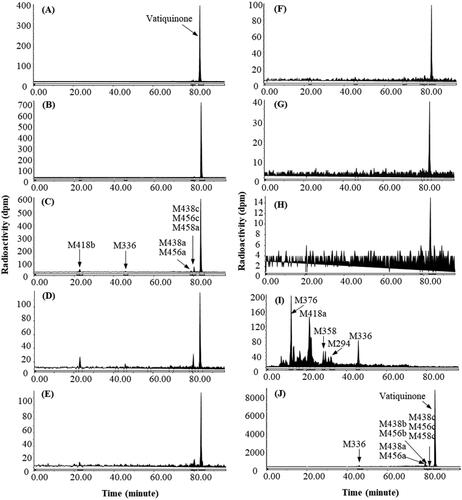
A total of 60.8% of the dose was recovered by 0–168 h post-dose and the main route of excretion was through faeces with less than 3% in urine. The mean Cmax values of 10.4 µg eq/mL in plasma and 5.10 µg eq/mL in blood of 14C-vatiquinone-derived radioactivity were both observed at 2 h post-dose. Plasma TRA decreased quickly after Tmax and was above BQL (0.119 µg eq/mL) by 168 h post-dose. Blood concentrations were lower than plasma concentrations at all time points post-dose and were above BQL (0.141 µg eq/mL) by 48 h post-dose. The mean blood:plasma concentration ratio was 0.5.
Vatiquinone plasma concentration peaked at 2 h, was the dominant circulating radioactive component in dog plasma. A few minor metabolites, each accounting for less than 9% of 0–96 h plasma TRA, were detected as circulating metabolites. Vatiquinone was not detectable in urine but was the most abundant radioactive component in faeces following a single oral dose in dogs.
AME in healthy male subjects following a single oral dose of 14C-vatiquinone
Following a single oral 400 mg 14C-vatiquinone dose on Day 7 which was preceded by TID oral dose of 200 mg or 400 mg for six days, subjects were eligible for discharge starting from 120 h and all were discharged by 216 h post Day 7 dose. There were no serious treatment-emergent adverse events (TEAEs) or TEAEs leading to discontinuation or death in this study. Only 1 subject reported individual adverse events of diarrhoea, pruritus, and rash. Individual TEAEs of pruritus and rash were assessed to be treatment-related. All TEAEs were mild in severity.
Following a single oral 400 mg 14C-vatiquinone dose on Day 7 which was preceded by TID oral dose of 200 mg or 400 mg for six days, the recoveries of dosed radioactivity in urine and faeces are summarised in , plasma PK parameters and distribution data of vatiquinone and its metabolites are listed in and , respectively. The representative metabolite profiles are shown in , and the plasma concentration-time curves of TRA, vatiquinone and its prominent metabolites are shown in . Plasma and faeces extraction efficiency and reconstitution recovery ranged from 79 to 121% and the column recovery ranged from 83 to 110%.
Figure 5. Representative HPLC radio-chromatograms of 2 h (A), 4 h (B), 6 h (C), 8 h (D), 24 h (E), and 48 h (F) plasma, 0–24 h urine (G), and 0–72 h faeces (H) from human subjects following a single 400 mg oral dose of 14C-vatiquinone.
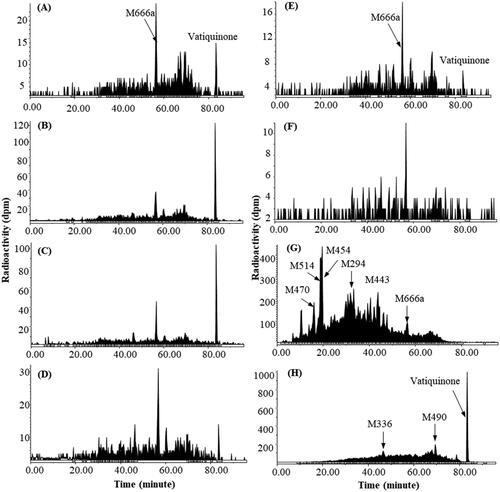
Figure 6. Plasma concentration–time curves of total radioactivity (TRA), vatiquinone, and its metabolites following oral dose administration in rats (A), dogs (B), and human subjects (C).
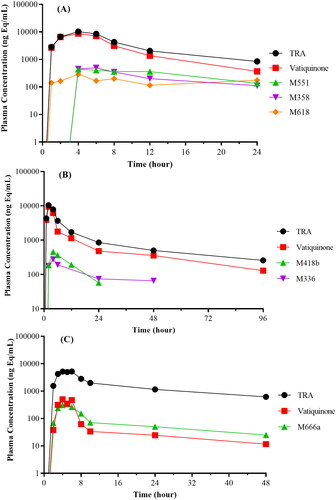
A total of 92.9% of the dose was recovered by 0–216 h post-dose with 70.6% of dose in faeces and 22.3% in urine. Most of the radioactivity was excreted by 144 h post-dose (87.7%). The mean Tmax values of 14C-vatiquinone-derived radioactivity in blood and plasma were observed at 4 and 6 h post-dose, with the mean Cmax values of approximately 2.6 and 5.3 µg eq/mL, respectively. Levels of radioactivity were quantifiable through at least 36 h post-dose in blood and were quantifiable through at least 72 h post-dose in plasma. Mean blood:plasma concentration ratios ranged from 0.47 to 0.62 through 96 h post-dose.
Vatiquinone, peaked at 6 h, was a minor circulating radioactive component in human plasma. A few minor metabolites, each accounting for less than 5% of 0–48 h plasma TRA, were detected as circulating metabolites. Vatiquinone was not detectable in urine but was the most abundant radioactive component in faeces.
Metabolite identification
In addition to unchanged vatiquinone, more than 30 metabolites were identified or characterised in plasma, bile, urine or faeces after oral administration of 14C-vatiquinone in rats, dogs, and human subjects.
Vatiquinone was identified by direct comparison of HPLC retention time and high-resolution mass spectral data with reference standard. Structures of other metabolites were proposed based on their high-resolution mass spectral data and fragmentation ions relative to vatiquinone.
Proposed metabolite assignment, molecular ion, molecular formula, and characteristic product ions of these major metabolites are shown in . Proposed structures and metabolic pathways for the formation of the detected metabolites are represented in .
Table 5. Mass spectral analysis of major metabolites of vatiquinone in plasma, bile, urine, and faeces from rats, dogs, and human subjects.
Discussion
Vatiquinone is considered a chroman-ring-opened metabolite of α-tocotrienol (Hinman et al. Citation2018) and should possess some similar physiochemical and ADME properties with vitamin E (tocopherols and tocotrienols). Vitamin E is absorbed along with other lipids (bile acids, free fatty acids, triglycerides and cholesterol, etc.) as micelle in intestine, and several receptors and transporters are involved in its absorption into lymph system and eventually into blood circulation and liver for metabolism and disposition (Schmölz et al. Citation2016). Following a 2 mg oral dose of SRR- or RRR α-14C-tocopherol in Fisher rats, the mass balance recovery was high (>89%) for both diastereomers but they showed different distribution and elimination patterns: RRR α-14C-tocopherol showed statistically higher exposures in plasma, brain, heart, liver, lung, kidney and adrenal gland, but less urinary excretion than SRR α-14C-tocopherol (Kaneko et al. Citation2000). Following a 10–15 mg dose of RRR α-14C-tocopherol succinate in young rabbits, oral absorption seemed limited and saturable, and the excretion of the radioactivity was much faster after oral dose than after subcutaneous or intravenous dose with higher dose being recovered in faeces than in urine irrespective of the dose route (Simon, Gross, et al. Citation1956). Following a single 0.18 nmol or 0.78 µg of 5-14CH3-(2R, 4’R, 8’R)-α-tocopherol to 6 male and 6 female healthy human subjects, 4.26% and 23.2% of dose were recovered in urine and faeces, respectively through 21 days, while the radioactivity in blood was detectable up to 460 days post-dose (Chuang et al. Citation2011).
In our study, metabolism and disposition of vatiquinone was investigated in rats, dogs, and human volunteers following a single oral dose of 14C-vatiquinone. Similar to SRR- and RRR α-14C-tocopherol in Fisher rats, following a single oral dose of 14C-vatiquinone in intact male SD rats, approximately 95% of the 14C-vatiquinone-derived radioactivity was excreted by 168 h with close to 89% excreted within 24 h post-dose, and the main route of excretion was through faeces with less than 3% in urine. In male BDC rats, approximately 94% of the 14C-vatiquinone-derived radioactivity was excreted by 72 h with more than 89% excreted within 48 h post-dose, and the main route of excretion was through faeces. The fast excretion in faeces and low recovery in combined bile and urine (approximately 16%) indicated that oral absorption in rats is relatively low (). However, following a single oral dose in dogs, only about 2.5% of dose in urine, 56.8% of dose in faeces, or 60.8% of dose in total was recovered over seven-day period post-dose (). The reason of the low mass balance recovery in dogs is unknown, and the possible reasons could be the retention of vatiquinone and its metabolites in the body as observed in rabbits following subcutaneous or intravenous dose of RRR α-[14C]-tocopherol succinate, and/or loss of radioactivity tracer due to volatile metabolite(s) formation in dogs. However, in contrast to slow excretion that resulted in less than 28% of dose recovery through 21 days following a single oral dose of 14CH3-α-tocopherol in human subjects (Chuang et al. Citation2011), excretion of 14C-vatiquinone and its metabolites seemed much faster: a total of 92.9% of dose was recovered thought 9 days post-dose with 70.6% of dose recovered in faeces and 22.3% of dose recovered in urine, and most of the radioactivity was excreted by 7 days post-dose (87.7%).
Metabolism of vitamin E starts with CYP4F2/CYP3A4 mediated ω-hydroxylation followed by subsequent β-oxidation on the side chain resulting in various carbon length ω-carboxylic acid metabolites (Wechter et al. Citation1996; Kiyose and Ueda Citation2004); and these phase I metabolites can be conjugated (such as glucuronides, sulphates or taurine conjugates) and be excreted, depending on the length of their side chain, either via urine or faeces (Simon, Eisengart, et al. Citation1956; Schmölz et al. Citation2016). For vatiquinone, in vitro and in vivo studies have indicated that CYP3A4 is the major enzyme involved in vatiquinone metabolism in human (PTC internal data).
Like vitamin E metabolism (Schmölz et al. Citation2016), the major metabolic pathways for vatiquinone involved reduction (quinone reduction and hydrogenation/saturation of double bonds), oxidation (including oxidation on the side chain and possibly on quinone methyl groups) and oxidative cleavage of the side chain (β-oxidation) leading to the formation of 12, 10, 8, and 6-carbon length carboxylic acid derivatives. Sulphation, glucuronidation and taurine conjugation are the common phase II metabolites (). For vitamin E metabolism, chroman ring opening followed by quinone reduction to form hydroquinone derivatives have been reported (Simon, Eisengart, et al. Citation1956; Schmölz et al. Citation2016; Hinman et al. Citation2018). Interestingly, a new chroman ring can be formed (e.g. M358 and M454), possibly from six-carbon length carboxylic acid metabolites after β-oxidation of vatiquinone in rats, dogs, and humans after oral dose ().
Species dependent metabolism of vatiquinone is observed in rats, dogs, and humans after oral dose administration. In rats, vatiquinone was the dominant circulating radioactive component, accounting for 78.7% of plasma radioactivity. M551 (a sulphate and taurine conjugate of 2,5-dihydroxyphenyldecanoic acid derivative), M358 (a dehydrated 2,5- dihydroxyphenylhexanoic acid derivative), and M618 (a glucuronide of vatiquinone hydroquinone), each accounting for less than 8% of plasma radioactivity, were the most prominent metabolites. Vatiquinone was not detected in rat urine and bile. Most metabolites in urine and bile were glucuronide or sulphate conjugates of β-oxidation products, and none of them was more than 1% of dose, while vatiquinone was the most abundant component in faeces (≥49% of the dose) following a 300 mg/kg oral dose of vatiquinone in rats ( and ). In dogs, vatiquinone was the dominant circulating radioactive component, accounting for 68.8% of plasma radioactivity. M438c (a dehydrogenated product of vatiquinone), M456c (a mono-oxidation product of vatiquinone), and M458 (a mono-oxidation and one double bound saturated product of vatiquinone) were the most abundant metabolites, together accounting for 8.54% of plasma radioactivity. In urine, vatiquinone was not detectable, and most metabolites in urine were β-oxidation products and their glucuronide or sulphate conjugates, and none of them was more than 0.25% of the dose. In faeces, vatiquinone, accounting for 36.8% of the dose, was the most abundant drug-related entity and no individual metabolite was greater than 3% of the dose ( and ). In human subjects, metabolism of vatiquinone seems more extensive following oral dose administration, and numerous vatiquinone metabolites and their isomers were detected in plasma, urine, and faeces (). Every effort was made to chromatographically separate radioactivity peaks as extensively as possible, complete separation and quantification of these metabolites/isomers was not feasible due to large numbers of coeluting metabolites/isomers. In plasma, vatiquinone was a minor radioactive component, and most of the plasma metabolites were the side chain oxidation products and their glucuronides, among which M666a (a glucuronide conjugate of vatiquinone tri-oxidation products) was the most abundant metabolite, accounting for 4.64% of plasma total radioactivity. In urine, vatiquinone was not detectable, and most metabolites were side chain oxidation and β-oxidation products and their glucuronide or sulphate conjugates, and none of them was more than 2% of the dose. In faeces, vatiquinone, accounting for 6.8% of the dose, was the most abundant drug-related component. Most metabolites were side chain oxidation products, and no individual metabolite was greater than 5% of the dose in faeces ( and ).
Conclusion
In summary, vatiquinone is a potent inhibitor of 15-lipoxygenase and is in clinical development for the treatment of mitochondrial diseases and other disorders characterised by high levels of oxidative stress and dysregulation of energy metabolism. Following a single oral dose of 14C-vatiquinone in rats, 14C-vatiquinone-derived radioactivity was quickly and widely distributed throughout the body and cleared from most tissues by 24 h post-dose. Vatiquinone showed different metabolism and disposition pattern in rats, dogs, and humans. Following oral dose administration, 94% of dose was recovered within seven days in rats, close to 93% of dose was recovered within nine days in human subjects, and about 61% of dose was recovered within seven days in dogs. Faecal excretion was the major route (>56% dose) in all species, while urinary excretion was minimal in rats and dogs (<3% dose) but was higher in humans (∼ 22% dose). Following oral dose administration, vatiquinone was the dominant circulating component in rats and dogs but was minor in human subjects. There were no plasma metabolites that were more than 10% of total drug related exposures in all species. Following oral dose administration, vatiquinone was not detectable in urine but was the most prominent component in faeces in all three species.
Author contributions
Ma J., Lee L., Yao B., Giannousis P., Thoolen M., Golden L., Klein M., and Kong R. participated in the research design. Ma J., Lee L., Ye Q., and Kong R. conducted experiments, performed data analysis and wrote or contributed to the writing of the manuscript.
Disclosure statement
All authors are current employees except that Thoolen M. is a former employee of PTC Therapeutics, Inc. Parts of this work were previously presented at a poster session of the American Epilepsy Society Annual Meeting; December 2–6. Nashville, Tennessee (Lee, et al. Citation2022).
Additional information
Funding
References
- Atkuri KR, Cowan TM, Kwan T, Ng A, Herzenberg LA, Herzenberg LA, Enns GM. 2009. Inherited disorders affecting mitochondrial function are associated with glutathione deficiency and hypocitrullinemia. Proc Natl Acad Sci U S A. 106(10):3941–3945. doi: 10.1073/pnas.0813409106.
- Blankenberg FG, Kinsman SL, Cohen BH, Goris ML, Spicer KM, Perlman SL, Krane EJ, Kheifets V, Thoolen M, Miller G, et al. 2012. Brain uptake of Tc99m-HMPAO correlates with clinical response to the novel redox modulating agent EPI-743 in patients with mitochondrial disease. Mol Genet Metab. 107(4):690–699. doi: 10.1016/j.ymgme.2012.09.023.
- Chuang JC, Matel HD, Nambiar KP, Kim S-H, Fadel JG, Holstege DM, Clifford AJ. 2011. Quantitation of [5-CH3]-(2R, 4'R, 8'R)-α-tocopherol in humans. J Nutr. 141(8):1482–1488. doi: 10.3945/jn.111.138925.
- Enns GM, Kinsman SL, Perlman SL, Spicer KM, Abdenur JE, Cohen BH, Amagata A, Barnes A, Kheifets V, Shrader WD, et al. 2012. Initial experience in the treatment of inherited mitochondrial disease with EPI-743. Mol Genet Metab. 105(1):91–102. doi: 10.1016/j.ymgme.2011.10.009.
- Hinman A, Holst CR, Latham JC, Bruegger JJ, Ulas G, McCusker KP, Amagata A, Davis D, Hoff KG, Kahn-Kirby AH, et al. 2018. Vitamin E hydroquinone is an endogenous regulator of ferroptosis via redox control of 15-lipoxygenase. PLoS One. 13(8):e0201369. doi: 10.1371/journal.pone.0201369.
- Kaneko K, Kiyose C, Ueda T, Ichikawa H, Igarashi O. 2000. Studies of the metabolism of α-tocopherol stereoisomers in rats using [5-methyl-14C]SRR- and RRR-α-tocopherol. J Lipid Res. 41(3):357–367. doi: 10.1016/S0022-2275(20)34474-6.
- Kiyose C, Ueda T. 2004. Distribution and metabolism of tocopherols and tocotrienols in vivo. J Clin Biochem Nutr. 35(1):47–52. doi: 10.3164/jcbn.35.47.
- Lee L, Ma M, Murase K, Thoolen M, Kong R. 2022. The clinical pharmacology of vatiquinone, a 15-lipoxygenase inhibitor targeting inflammation and oxidative stress. Poster at Behind the Exhibit Hall. American Epilepsy Society; Dec 2022; Nashville, TN.
- Lee L, Murase K, Ma J, Thoolen M. 2023. Clinical drug-drug interaction between vatiquinone, a 15-lipoxygenase inhibitor, and rosuvastatin, a breast cancer resistance protein substrate. Clin Pharmacol Drug Dev. 12(3):279–286. doi: 10.1002/cpdd.1199.
- Martinelli D, Catteruccia M, Piemonte F, Pastore A, Tozzi G, Dionisi-Vici C, Pontrelli G, Corsetti T, Livadiotti S, Kheifets V, et al. 2012. EPI-743 reverses the progression of the pediatric mitochondrial disease-genetically defined Leigh Syndrome. Mol Genet Metab. 107(3):383–388. doi: 10.1016/j.ymgme.2012.09.007.
- Murase K, Lee L, Ma J, Barrett R, Thoolen M. 2022. Evaluation of vatiquinone drug-drug interaction potential in vitro and in a phase 1 clinical study with tolbutamide, a CYP2C9 substrate, and omeprazole, a CYP2C19 substrate, in healthy subjects. Eur J Clin Pharmacol. 78(11):1823–1831. doi: 10.1007/s00228-022-03393-0.
- Pastore A, Petrillo S, Tozzi G, Carrozzo R, Martinelli D, Dionisi-Vici C, Giovamberardino GD, Ceravolo F, Klein MB, Miller G, et al. 2013. Glutathione: a redox signature in monitoring EPI-743 therapy in children with mitochondrial encephalomyopathies. Mol Genet Metab. 109(2):208–214. doi: 10.1016/j.ymgme.2013.03.011.
- Sadun AA, Chicani CF, Ross-Cisneros FN, Barboni P, Thoolen M, Shrader WD, Kubis K, Carelli V, Miller G. 2012. Effect of EPI-743 on the clinical course of the mitochondrial disease Leber hereditary optic neuropathy. Arch Neurol. 69(3):331–338. doi: 10.1001/archneurol.2011.2972.
- Schmölz L, Birringer M, Lorkowski S, Wallert M. 2016. Complexity of vitamin E metabolism. World J Biol Chem. 7(1):14–43. doi: 10.4331/wjbc.v7.i1.14.
- Shrader WD, Amagata A, Barnes A, Enns GM, Hinman A, Jankowski O, Kheifets V, Komatsuzaki R, Lee E, Mollard P, et al. 2011. α-Tocotrienol quinone modulates oxidative stress response and the biochemistry of aging. Bioorg Med Chem Lett. 21(12):3693–3698. doi: 10.1016/j.bmcl.2011.04.085.
- Simon EJ, Eisengart A, Sundheim L, Milhorat AT. 1956. The metabolism of vitamin E. II. purification and characterization of urinary metabolites of α-tocopherol. J Biol Chem. 221(2):807–817. doi: 10.1016/S0021-9258(18)65194-4.
- Simon EJ, Gross CS, Milhorat AT. 1956. The metabolism of vitamin E. 1. The absorption and excretion of d-α-tocopheroI-5-methyl-C14-succinate. J Biol Chem. 221(2):797–805. doi: 10.1016/S0021-9258(18)65193-2.
- Wechter WJ, Kantoci D, Murray ED, D'Amico DC, Jung ME, Wang WH. 1996. A new endogenous natriuretic factor: LLU-α. Proc Natl Acad Sci U S A. 93(12):6002–6007. doi: 10.1073/pnas.93.12.6002.
- Zesiewicz T, Salemi JL, Perlman S, Sullivan KL, Shaw JD, Huang Y, Isaacs C, Gooch C, Lynch DR, Klein MB. 2018. Double-blind, randomized and controlled trial of EPI-743 in Friedreich’s ataxia. Eurodegener Dis Manag. 8(4):233–242. doi: 10.2217/nmt-2018-0013.