Abstract
All the data recently reported for the purported air/water stable PrIV complex, [(L)PrIV(L)4(OH0.94Cl0.06)2PrIV(L)]n (L = N-acetylanthranilate) [1], have been reinterpreted to show that it is, in fact, the PrIII complex [Pr2L6(H2O)2]n. Importantly, it is isomorphous with its reported EuIII analogue [Eu2L6(H2O)2]n [2], providing further evidence of the incorrect assignment in the literature.
1. Introduction
Among the many exciting developments in rare earth chemistry in recent decades are the preparations of molecular complexes in unusual oxidation states. A major achievement has been the extension of the divalent oxidation state from the traditional Sm, Eu, and Yb to all the elements except Pm [Citation3, Citation4]. More recently, notably in work by Mazzanti and La Pierre, molecular PrIV and TbIV complexes have been prepared [Citation5–15], these oxidation states having been previously accessed only in oxides and fluorides [Citation16]. There have been further elaborations of PrIV silyloxide in complexes by Zheng [Citation17, Citation18]. All these syntheses and handling of products required the use of inert atmosphere conditions because of the instability of the LnIV complexes to air and moisture. Consequently, we were surprised or even astonished to read a report of an air and water-stable PrIV coordination polymer, [(L)PrIV(L)4(OH0.94Cl0.06)2PrIV(L)] (L = N-acetylanthranilate), and its anti-proliferative activity against several cancer cell lines [Citation1]. This has led us to re-refine the reported data and we find that it can be satisfactorily fitted to a PrIII polymer with the composition [Pr2L6(H2O)2], which also is consistent with the reported microanalysis for the bulk product and accords with the air and water stability. Furthermore, there is a reported EuIII complex of the N-acetyl anthranilate ligand [Citation2] that is isomorphous with the Pr complex.
2. Discussion
compares the reported refinement as a PrIV complex [Citation1] with our refinement as [Pr2L6(H2O)2]n and the reported [Eu2L6(H2O)2]n [Citation2]. As is evident, the solution as a trivalent complex is at least as satisfactory as that reported with the additional advantage of explaining the air and water stability. Our structure based on the new refinement is shown in . The complex is a polymer of centrosymmetric dimeric units in which PrIII is eight-coordinate, with one chelating carboxylate, four oxygen atoms from four bridging bidentate carboxylates, one coordinated acetyl oxygen, and one water molecule. The acetyl oxygen is from a ligand separate from those of the dimeric unit and hence this linkage builds the dimers into a polymer.
Figure 1. Crystal structure of the PrIII complex [Pr2L6(H2O)2]n (symmetry code: *1 − X, 1 − Y, 1 − Z; #1 − X, −Y, 1 − Z; ^+X, 1 + Y, +Z). Hydrogen atoms were omitted for clarity.
![Figure 1. Crystal structure of the PrIII complex [Pr2L6(H2O)2]n (symmetry code: *1 − X, 1 − Y, 1 − Z; #1 − X, −Y, 1 − Z; ^+X, 1 + Y, +Z). Hydrogen atoms were omitted for clarity.](/cms/asset/6c4341d6-e2d8-49b6-bf6b-dbdf472097f2/gcoo_a_2384711_f0001_c.jpg)
Table 1. Crystal data and structural refinement for the reported PrIV complex, as a PrIII [Pr2L6(H2O)2]n complex and the reported EuIII complex.
A key difference between the PrIV and the PrIII structures is that the former has a disordered Pr-OH/Cl (94/6%), while the latter has a Pr-OH2 bond. The Pr-OH2 bond length (2.477(3) Å) lies within the range 2.44–2.62 Å for 18 examples of eight-coordinate complexes with PrIII-OH2 bonds listed in the CCDC [Citation19]. On the other hand, this distance (2.477(3) Å) is far too long for a terminal PrIV-OH bond. There are only two terminal PrIII-OH bonds in the CCDC, 2.257 Å [Citation20] and 2.373 Å [Citation21], and PrIV-OH bonds should be 0.16 Å shorter than PrIII-OH [Citation22], i.e. 2.21–2.09 Å. O10 from the terminal water forms hydrogen bonds to two neighboring O atoms, O6& (−x, 1 − y, 1 − z) and O3$(1/2 − x, y − 1/2, 1/2 − z), consistent with coordination of water and not OH ().
Figure 2. H-Bond formation from O10 of coordinated water to two neighboring oxygen atoms. H10B–O6& (−x, 1 − y, 1 − z) 1.895(4) Å; H10A–O3$ (1/2 − x, y − 1/2, 1/2 − z 1.851(4) Å. O10–H10B–O6& 171.7(3)°, O10–H10A–O3$ 158.3(3)°.
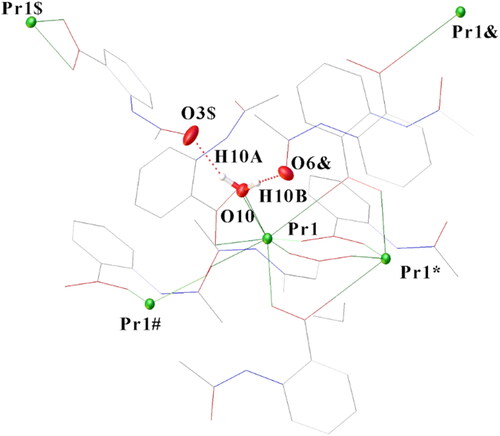
The Pr-O(carboxylate) bond lengths () are also appropriate for chelating and bridging bidentate carboxylatopraseodymium(III) complexes (e.g. [Pr(4-HOC6H4COO)3(H2O)(DMF)]2·(DMF)3 [Citation23], [Pr2(aip)3(H2O)2·3H2O]n (aip = 5-aminoisophthalate) [Citation24]), but are significantly longer than would be expected for PrIV analogues. Likewise binding of the acetyl group Pr-O9 () is also appropriate for a PrIII complex with a bond length close to that of PrIII-OH2. Comparing the bond lengths with those of the isomorphous EuIII complex () shows that the bond length difference for the chelating carboxylate and the coordinated water is near the 0.06 Å expected from ionic radius values [Citation22]. The differences for the Ln-O(bridging carboxylate) bond lengths are much more variable and the average is less than expected, perhaps pointing to increased steric strain with the smaller Eu. On the other hand, the coordinated carbonyl oxygen is more distant than expected in the Pr complex.
Table 2. Bond lengths (Å) for the reported PrIV complex, as a PrIII complex [Pr2L6(H2O)2]n and the reported EuIII complex.
About bulk composition, the observed microanalysis values [Citation1] C, 46.63; H, 3.71; N 5.99% fit [Pr2L6(H2O)2]n, C54H52N6O20Pr2 calc.: C 46.77; H, 3.78; N 6.06%, as well as for the PrIV formulation, calc. C, 46.76; H, 3.63; N, 6.06%. Regarding the reported 1H NMR assignments [Citation1], most apply equally well to the present structural proposal, except that 14.79 ppm, assigned to Pr-OH, was an unlikely attribution owing to exchange with water in the d6-dmso solvent. If 10.54 ppm is assigned to NH of the uncoordinated N-acetylamido group, 14.75 ppm may be assigned to NH of the coordinated-acetylamido group, paramagnetic shifted by PrIII. The pale green color of the complex [Citation1] is as expected for Pr3+, while a broad ligand absorption at 305 nm obscures any f-f transitions, which are of low intensity [Citation1]. The reported TGA [Citation1] shows a weight loss of 2.47% below 200 °C, which can be attributed to loss of coordinated water before more substantial decomposition above 200 °C. The broad features in the IR spectrum from 3250 to 2550 cm−1 can encompass OH stretching of coordinated water as well as extensive NH stretching. Carboxylate absorptions are similarly assigned for either structure, though the use of KBr disks raises the possibility of carboxylate exchange giving KL and Pr bromide species [Citation25]. The reported magnetic moment of 2.8 B.M. is low for PrIII [Citation26], but not unknown [Citation27]. Further, with errors in oxidation state and composition, the diamagnetic correction will be wrong. Accordingly, all the reported data for the reported PrIV polymeric complex can be accommodated with it being the PrIII complex [Pr2L6(H2O)2]n.
3. Conclusion
The reported air stable PrIV complex, [[(L)PrIV(L)4(OH0.94Cl0.06)2PrIV(L)]n (L = N-acetylanthranilate), is in reality a PrIII complex [Pr2L6(H2O)2]n.
4. Experimental
The X-ray crystal structure of the reported PrIV complex (CCDC 2075265) was downloaded from the CCDC database, and res/hkl files were extracted from the cif file in Olex2 [Citation28]. The structure was solved and refined by SHELXT and SHELX2015 [Citation29]. Crystal data and refinement details are given in . CCDC 2355887 for the PrIII compound reported here contains the supplementary crystallographic data for this paper. The data can be obtained free of charge from the Cambridge Crystallographic Data Centre via www.ccdc.cam.ac.uk/data_request/cif.
Disclosure statement
No potential conflict of interest was reported by the authors.
Additional information
Funding
References
- A.A.M. Aly, A.B.M. Ibrahim, A.S.A. Zidan, H.K. Mosbah, S.A. Atta, I. Schicht, A. Villinger. J. Mol. Struct., 1256, 132508 (2022).
- S. Zhang, C. Li, W. Li, Y. Yang. Chin. J. Inorg. Chem., 24, 1701 (2008).
- (a) W.J. Evans. Inorg. Chem., 46, 3435 (2007). (b) M.R. MacDonald, J.E. Bates, J.W. Ziller, F. Furche, W.J. Evans. J. Am. Chem. Soc., 135, 9857 (2013).
- (a) J.C. Wedal, W.J. Evans. J. Am. Chem. Soc., 143, 18354 (2021). (b) J.D. Queen, L.M. Anderson-Sanchez, C.R. Stennett, A. Rajabi, J.W. Ziller, F. Furche, W.J. Evans. J. Am. Chem. Soc., 146, 3279 (2024).
- C.T. Palumbo, I. Zivkovic, R. Scopelliti, M. Mazzanti. J. Am. Chem. Soc., 141, 9827 (2019).
- N.T. Rice, I.A. Popov, D.R. Russo, J. Bacsa, E.R. Batista, P. Yang, J. Telser, H.S. La Pierre. J. Am. Chem. Soc., 141, 13222 (2019).
- T.P. Gompa, A. Ramanathan, N.T. Rice, H.S. La Pierre. Dalton Trans., 49, 15945 (2020).
- A.R. Willauer, C.T. Palumbo, F. Fadaei-Tirani, I. Zivkovic, I. Douair, L. Maron, M. Mazzanti. J. Am. Chem. Soc., 142, 5538 (2020).
- A.R. Willauer, C.T. Palumbo, R. Scopelliti, I. Zivkovic, I. Douair, L. Maron, M. Mazzanti. Angew. Chem. Int. Ed., 59, 3549 (2020).
- N.T. Rice, I.A. Popov, D.R. Russo, T.P. Gompa, A. Ramanathan, J. Bacsa, E.R. Batista, P. Yang, H.S. La Pierre. Chem. Sci., 11, 6149 (2020).
- A.R. Willauer, I. Douair, A.-S. Chauvin, F. Fadaei-Tirani, J.-C.G. Bünzli, L. Maron, M. Mazzanti. Chem. Sci., 13, 681 (2022).
- N.T. Rice, I.A. Popov, R.K. Carlson, S.M. Greer, A.C. Boggiano, B.W. Stein, J. Bacsa, E.R. Batista, P. Yang, H.S. La Pierre. Dalton Trans., 51, 6696 (2022).
- A. Ramanathan, J. Kaplan, D.-C. Sergentu, J.A. Branson, M. Ozerov, A.I. Kolesnikov, S.G. Minasian, J. Autschbach, J.W. Freeland, Z. Jiang, M. Mourigal, H.S. La Pierre. Nat. Commun., 14, 3134 (2023).
- A. Ramanathan, E.D. Walter, M. Mourigal, H.S. La Pierre. J. Am. Chem. Soc., 145, 17603 (2023).
- T.P. Gompa, S.M. Greer, N.T. Rice, N. Jiang, J. Telser, A. Ozarowski, B.W. Stein, H.S. La Pierre. Inorg. Chem., 60, 9064 (2021).
- F.A. Cotton, G. Wilkinson. Advanced Inorganic Chemistry: A Comprehensive Text, 5th Edn. pp. 976–977 (1988).
- T. Xue, Y.-S. Ding, Z. Zheng. Dalton Trans., 53, 5779 (2024).
- T. Xue, Y.-S. Ding, X.-L. Jiang, L. Tao, J. Li, Z. Zheng. Precis. Chem., 1, 583 (2023).
- The survey was performed with CCDC: ConQuest Version 2024.1.0 (2024).
- Y. Song, B. Yan, L. Weng. Polyhedron, 26, 4591 (2007).
- H. Sheng, Y. Feng, Y. Zhang, Q. Shen. Eur J. Inorg. Chem., 2010, 5579 (2010).
- R.D. Shannon. Acta Crystallogr. Sect. A, 32, 751 (1976).
- X. Zhou, W.-T. Wong, S.C.K. Hau, P.A. Tanner. Polyhedron, 88, 138 (2015).
- Y. Qiu, H. Deng, S. Yang, J. Mou, C. Daiguebonne, N. Kerbellec, O. Guillou, S.R. Batten. Inorg. Chem., 48, 3976 (2009).
- G.B. Deacon, R.J. Phillips. Coord. Chem. Rev., 33, 227 (1980).
- W.J. Evans, M.A. Hozbor. J. Organomet. Chem., 326, 299 (1987).
- K.O. Hodgson, F. Mares, D.F. Starks, A. Streitwieser, Jr. J. Am. Chem. Soc., 95, 8650 (1973).
- O.V. Dolomanov, L.J. Bourhis, R.J. Gildea, J.A.K. Howard, H. Puschmann. J. Appl. Crystallogr., 42, 339 (2009).
- G.M. Sheldrick. Acta Cryst., C71, 3 (2015).