Abstract
A glasshouse pot experiment was conducted to investigate the effect of two species of arbuscular mycorrhizal fungi (Glomus intraradices and Acaulospora laevis) and Pseudomonas fluorescens under three levels of superphosphate fertilizer (half dose, 40 kg/ha; recommended dose, 80 kg/ha; and double dose, 160 g/ha) on the growth and yield of broccoli (Brassica oleracea var. italica). There was negligible colonization of broccoli roots by the mycorrhizal fungi, demonstrating that they have no role in the growth of this plant. However, the strain of P. fluorescens used (MTCC No. 103) was found to have a potential role in enhancing the growth, phosphatase activity, chlorophyll content, nutrient uptake and yield of broccoli when combined with the recommended dose of fertilizer. By contrast, P. fluorescens combined with a half or double dose of fertilizer led to poor growth and delayed head formation. This suggests that root inoculation with P. fluorescens (MTCC No. 103) will improve plant growth, nutrient uptake and yield of broccoli when combined with the recommended dose of superphosphate fertilizer.
Introduction
Broccoli (Brassica oleracea L. var. italica Plenck), which is also known as ‘the crown jewel of nutrition’, is a highly priced vegetable (Watt Citation1983; American Dietetics Association Citation1992). Consumers recognize this vegetable as a functional food that confers health benefits, as it contains important antioxidant phytochemicals such as polyphenols, β-carotene, α-tocopherol, indoles and isothiocyanates (Singh et al. Citation2004, Citation2006; Farnham et al. Citation2005; Podsedek Citation2007). However, there is still little quality production of this crop in India, partly due to limited knowledge about the appropriate method of production among growers and partly due to low phosphorus (P) levels in the soil, which is a key element for increasing the yield of broccoli (Ouda & Mahadeen Citation2008; Islam et al. Citation2010).
Phosphorus is an essential inorganic nutrient for plants as it plays an important role in the formation of nucleic acids and phospholipids, the metabolism of carbon, and the activation of a wide range of enzymes (Lambers et al. Citation2006). However, 70%–90% of applied P fertilizer soon becomes unavailable to plants as it is converted into inorganic P fractions that are fixed by chemical adsorption and precipitation in the soil (Sharma et al. Citation2012). Similarly, organic P fractions are immobilized in the soil organic matter (Sanyal & De Datta Citation1999). Consequently, broccoli production relies on growing suitable varieties that are able to unlock and absorb P from the bound soil resource, and on improving farming practices through the balanced and judicious use of P fertilizers. To date, there has been very little research into methods to enhance the growth and production of broccoli. However, recently it has been recommended that organic management could be used to partially or totally substitute for the use of costly synthetic fertilizers (Singh et al. Citation2011; Tanwar et al. Citation2012; Citation2013a).
Microorganisms play a fundamental role in the cycling of inorganic and organic P in the rhizosphere (Richardson & Simpson Citation2011). Arbuscular mycorrhizal (AM) symbiosis is known to bring a wide range of benefits to the host plant in both agricultural production systems and natural ecosystems. The main effect of AM fungi is their capacity to supply mineral nutrients that are relatively immobile in the soil, particularly P and trace elements (copper [Cu] and zinc [Zn]); they achieve this by exuding organic anions and phosphatase enzymes, and by modifying the pH around their hyphae (Giri et al. Citation2005). Many plant growth-promoting rhizobacteria (PGPR) are also known to stimulate plant growth through direct or indirect interactions with plant roots. In soil with low P availability, PGPR (mainly pseudomonads) are known to help with the release of phosphate ions from both sparingly soluble inorganic sources of P (via the secretion of organic acids) and organic sources (via the secretion of phosphatase enzymes) (Artursson et al. Citation2006).
Members of the family Brassicaceae are generally considered to be non-mycorrhizal, possibly due to locally induced changes in endogenous concentrations of glucosinolates (Tong et al. Citation2011). However, despite this, there have been numerous reports of mycorrhizal colonization in some members of Brassicaceae, including broccoli (Purakayastha et al. Citation1998), radish (Kamalakannan & Manivannan Citation2002), Biscutella laevigata (Orlowska et al. Citation2002) and Thlaspi spp. (Regvar et al. Citation2003; Pongrac et al. Citation2007), suggesting that colonization (or lack of) is related to environmental or edaphic factors rather than phylogenetic constraints (Miller & Bever Citation1999).
Therefore, this study investigated (1) whether two species of AM fungi (Glomus intraradices Schenck and Smith and Acaulospora laevis Gerd. and Trappe) are able to colonize broccoli roots; and (2) what effect the PGPR Pseudomonas fluorescens and the AM fungi have on broccoli growth and yield under different doses of P fertilizer, so that the most appropriate bioinoculant and concentration of P fertilizer can be recommended to farmers.
Materials and methods
Experimental design
Broccoli requires a P fertilizer concentration of 80 kg/ha when applied as P2O5 (Malik & Kumar Citation2007). This experiment was conducted using a 3 × 6 factorial study design that was completely randomized in which three concentrations of superphosphate fertilizer, i.e. half the recommended dose (F1), 0.3 g/pot; the recommended dose (F2), 0.6 g/pot; and double the recommended dose (F3), 1.2 g/pot, and six bioinoculant combinations, i.e. control (C), G. intraradices (G), A. laevis (A), G. intraradices + P. fluorescens (G + Pf), A. laevis + P. fluorescens (A + Pf) and G. intraradices + A. laevis + P. fluorescens (G + A + Pf) were used, with five replicates of each.
Growth conditions
The experiment was carried out at a temperature of 20 ± 5 °C and a humidity of 50%–70% in a glasshouse at the Botany Department of Kurukshetra University, Kurukshetra, Haryana, from October 2011 to February 2012. Light was provided by cool white fluorescent lamps (8000 lux) under a 16 h photoperiod and natural sunlight was also available in the glasshouse. The physico-chemical parameters of the soil samples were analysed by the Directorate of Agriculture, Krishi Bhawan, Sector 21, Panchkula, Haryana, India. The soil characteristics were as follows: sand, 64.2%; silt, 21.81%; clay, 3.9%; pH 6.8; electrical conductivity 0.25 dS/m; organic carbon 0.4%; total nitrogen (N), 55 mg/kg soil; P, 9.13 mg/kg soil; potassium (K), 110 mg/kg soil; and sulphur (S), 14.8 mg/kg soil.
Isolation, quantification and identification of AM fungi
Rhizosphere soil samples of broccoli were collected from the fields of Kurukshetra District, Haryana, India and assessed for the presence of AM fungi by isolating AM spores using the wet sieving and decanting technique of Gerdemann & Nicolson (Citation1963). In brief, rhizosphere soil samples were air dried, powdered and passed through a 2 mm sieve. Fifty grams of this soil was dispersed in 500 mL water, stirred on a magnetic stirrer for about 30 min and left undisturbed overnight. This suspension was decanted through a series of sieves (150 µm, 120 µm, 90 µm, 63 µm, 45 µm) placed in descending order. The residue in the sieves was filtered by repeated washing with water through gridded Whatman's filter paper number 1, which was divided into many small compartments.
Quantification of the isolated spores was carried out using the grid line intersect method proposed by Adholeya & Gaur (Citation1994). The filter paper containing spores was spread on an inverted Petri dish and scanned under a stereo binocular microscope at 60× magnification. All intact spores were counted in each column and summed to determine the total number of spores. These spores were transferred on to a glass slide with polyvinyl lactic acid (PVLA) using a hypodermic needle.
Slides were examined under a high-power research microscope for identification. Spores were identified to species level on the basis of their morphology and wall ornamentation characteristics, which include colour, shape, size, wall structure, spore ornamentation, nature and size of subtending hyphae, and number and arrangement of spores in the sporocarp, according to the manuals of Walker (Citation1986), Morton & Benny (Citation1990), Schenck & Perez (Citation1990), Mukerji (Citation1996) and Morton & Redecker (Citation2001). It was found that G. intraradices and A. laevis were the dominant AM fungal strains.
Bioinoculant preparation
The starter inoculum of each selected species of AM fungus was raised following the funnel technique of Menge & Timmer (Citation1982), using maize as a host for 3 months. With this technique, a sterilized Borosil funnel (250 mL) was filled with a 3:1 mixture of sterilized soil and sand that was inoculated with the selected AM fungal spores, and five disinfected seeds of maize were sown in each funnel. After 60 days of growth, the maize plant roots were inspected for mycorrhizal colonization and the rhizosphere soil was analysed to determine the number of AM spores. This inoculum was further multiplied first in earthen funnels and then in larger earthen pots (Tanwar et al. Citation2013c). The resulting inoculum was used in the present experiment.
The inoculum of P. fluorescens (Microbial Type Culture Collection No. 103) was obtained from the Institute of Microbial Technology, Chandigarh, India, which received it from the National Collection of Industrial and Marine Bacteria in the UK under the accession number NCIB9046. P. fluorescens was cultured in a nutrient broth medium (beef extract, 3 g/L; peptone, 5 g/L; NaCl, 5 g/L) which was incubated at 32 °C for 48 h to obtain a concentration of 1 × 109 colony forming units (cfu) mL−1. This strain of P. fluorescens had previously been used for plant growth promotion experiments because of its ability to solubilize P (Tanwar et al. Citation2012, Citation2013a, Citation2013b).
Experimental setup
The experiment had 18 treatments, with five replicates of each. This included six combinations of inoculum (see ‘Experimental design’ above) each at three levels of P fertilizer. Soil from the experimental site was sieved through a 2 mm sieve and mixed with sand at a ratio of 3:1 soil:sand. It was autoclaved at 121 °C for 2 h for 2 consecutive days. Earthen pots (25.4 cm length × 25 cm diameter) with a capacity of 2 kg soil were used and a basal dressing of one of three levels of P (as superphosphate) fertilizer (F1, 0.3 g/pot; F2, 0.6 g/pot; and F3, 1.2 g/pot) was applied to each pot. A 10% (w/w) inoculum of AM fungi was added to each pot and mixed with the soil, which consisted of chopped up pieces of AM colonized roots of maize plants plus the soil containing AM spores (400–450 per 100 g inoculum). P. fluorescens was inoculated using the root dip method. In the control set, no inoculum was added.
Plant material
Broccoli seeds (Fiesta-F) (Bejo Sheetal Seeds, Maharashtra, India) were surface sterilized using 0.5% (v/v) sodium hypochlorite for 10 min and then washed with sterilized deionized water. Seeds were germinated in a shallow tray containing a 3:1 ratio of sterilized soil and sand. Thirty days after emergence, healthy seedlings were uprooted and their roots washed with running water to remove adhering soil particles. Plants were either replanted (control and treatments without P. fluorescens) or dipped in a cell suspension of P. fluorescens for 5 min and replanted (treatments with P. fluorescens), with a single seedling planted in each pot. Plants were watered daily to maintain the moisture content of the soil at approximately 60% water holding capacity and fertilized every 15 days with 100 mL/pot Hoagland nutrient solution (without KH2PO4). Each treatment was replicated five times to give a total of 90 pots.
Plant harvest and analysis
Plants were harvested 120 days after transplantation (DAT) and the effect of bioinoculants on various growth parameters was recorded. The change in plant height (cm) and chlorophyll content (mg g−1 FM) (Arnon Citation1949) were recorded. At 120 DAT, flower heads were harvested and weighed to obtain the fresh apical head weight (g) for each plant. Similarly, head diameter (cm), lateral head fresh weight (g) and petiole length (cm) were measured. Plants were harvested, and roots and shoots were weighed separately to obtain their fresh weight (g) and dry weight (g) (following oven drying at 70°C until a constant weight was obtained).
Roots were stained and assessed using Phillips & Hayman's (Citation1970) rapid clearing and staining technique. With this technique, roots were washed with tap water and cut into 1 cm pieces. The roots were cleared with 10% KOH overnight, followed by 5–10 min treatment with 1% HCl. Finally, they were stained overnight with 0.5% Trypan blue followed by overnight destaining with lactophenol to remove any excess stain.
Root colonization was evaluated using the root slide technique (Giovannetti & Mosse Citation1980). Stained roots were mounted under two glass slides with a 1:1 ratio of lactic acid:glycerol. Each root fragment was thoroughly checked along its entire length at 100× and 400× magnification to record mycorrhizal structures, such as the presence of arbuscules, vesicles and hyphal coils. Root colonization was calculated using the following formula:
Mycorrhizal dependency was calculated by using the dry weight of mycorrhizal and non-mycorrhizal plants, according to the method of Plenchette et al. (Citation1983):
Fresh roots were used for the extraction of alkaline phosphatase (ALP) and acid phosphatase (ACP), following the procedure of Tabatabai & Bremner (Citation1969). With this method, p-nitrophenyl phosphate (PNPP) was used as a substrate that was later hydrolysed to p-nitrophenol (PNP) by the enzyme. One gram of fresh roots were homogenized in 5 mL of ice cold sodium acetate buffer (0.1 M at pH 4.8) to extract acid phosphatase and in 5 mL of sodium carbonate-bicarbonate buffer (0.05 M at pH 10) to extract alkaline phosphatase. The resulting homogenate was centrifuged at 10,000 rpm for 15 min and the supernatant thus obtained (which is referred to as crude enzyme extract) was used for the assay of acid phosphatase activity.
The phosphorus content of broccoli shoots, roots and heads was determined using the vanadomolybdate phosphoric yellow colour method (Jackson Citation1973); and total nitrogen was calculated using the Kjeldahl method (Kelplus nitrogen estimation system, supra-LX, Pelican Equipments, Chennai, India).
Statistical analysis
Data were analysed using analysis of variance and means were compared using Fisher's least significant difference test in the Statistical Package for Social Sciences (ver. 11.5, Chicago, USA).
Results
Mycorrhization
Mycorrhization was determined in terms of AM root colonization, AM spore number and mycorrhizal dependency of the host plant (). Neither of the AM fungi (G. intraradices or A. laevis) colonized broccoli roots. The intraradical hyphae colonized the tip portion only, rarely being found in cortical cells. Extraradical hyphae were also detected, whereas arbuscules and vesicles were not observed.
Table 1 Efficacy of bioinoculants and superphosphate doses on mycorrhization of broccoli.
The data presented in show that the number of AM spores in the soil and the percentage root colonization varied between treatments under all levels of applied fertilizer, and that there was no correlation between AM spore number and root colonization. The maximum number of spores was recorded in G + A + Pf at F1, followed by the single inoculations of A and G and the combination of G + A + Pf at F2. Similarly, the highest proportion of colonized roots was detected in the single inoculation of A. While G + A + Pf at F1 showed maximum mycorrhizal dependency followed by G at F1.
Plant photosynthetic activity
Increasing the concentration of P fertilizer from a half dose to the recommended dose (F1 to F2) increased plant chlorophyll content; however, the highest P fertilizer dose (F3) led to a reduction in chlorophyll (). The presence of P. fluorescens generally resulted in increased chlorophyll content (a and b). The application of G + A + Pf increased the concentration of chlorophyll a at F1 and F2 whereas G + A + Pf at F2 increased the concentration of chlorophyll b in broccoli leaves compared with F1 and F3 ().
Table 2 Efficacy of bioinoculants and superphosphate doses on leaf chlorophyll content and root phosphatase activity of broccoli.
Plant phosphatases
The activity of phosphatases (ACP and ALP) was higher in the roots of all of the inoculated plants compared with the control (), with ACP being more activated than ALP. Plant phosphatase activity increased as the concentration of P fertilizer was increased from F1 to F2, but decreased at F3. Maximum ALP activity was noted in G + A + Pf at F2 and maximum ACP activity was found in G + Pf at F2.
Growth performance
Increasing the level of P fertilizer from F1 to F2 increased most of the morphological characteristics studied (, ). Within each treatment, the rate of promotion usually increased as the concentration of fertilizer increased from F1 to F2. But further increase in fertilizer input to F3 adversely affected plant growth except for G + A + Pf, which showed a gradual increase in root length. The greatest effect on plant height was seen in the sole application of A, whereas the maximum increase in root length was observed under the sole application of G. The application of A + Pf led to the greatest shoot and root fresh weight and dry weight, followed by sole application of G.
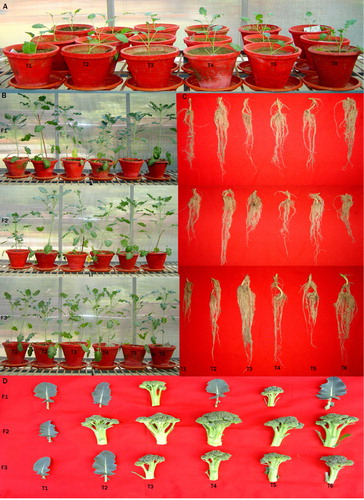
Table 3 Efficacy of bioinoculants and superphosphate doses on growth parameters of broccoli.
Plant nutrient uptake
At 120 DAT, plant nutrient content (P and N) of the shoots and roots was higher in all of the inoculated plants than in the controls (). Maximum nutrient content was found in plants that had been supplemented with the recommended dose of P fertilizer (F2), with F1 leading to the second highest nutrient content and F3 the lowest. Both shoot and root P levels were highest in G + A + Pf followed by A + Pf, G + Pf, A and G, respectively, at F2. The same treatment (G + A + Pf) also exhibited the highest N content (shoot and root).
Table 4 Efficacy of bioinoculants and superphosphate doses on phosphorus and nitrogen content of broccoli.
Fruit yield and quality
Broccoli yield was greatly influenced by the applied P fertilizer, and there was a positive interaction between the recommended fertilizer (F2) dose and applied bioinoculants ( and ). For most treatments, the yield parameters were more pronounced in plants that had been supplied with the recommended dose of fertilizer (F2), followed by F1 and then F3. The number of days of head initiation decreased with increasing levels of phosphorus from F1 to F2, with head formation first starting at 62 DAT in plants inoculated with G + Pf at F2. This treatment also resulted in maximum apical and lateral head fresh weight, whereas A + Pf treated plants experienced maximum head diameter and petiole length. A + Pf treated plants also had maximum fruit P content, while G + A + Pf had maximum N and protein content. By contrast, over-fertilization (F3) resulted in delayed head formation, extending it to 114 DAT in some cases, and even no head formation after 120 DAT for other treatments.
Table 5 Efficacy of bioinoculants and superphosphate doses on broccoli fruit yield and quality.
Discussion
In this study, inoculated broccoli roots showed the presence of AM spores, intraradical hyphae and extraradical hyphae. However, no arbuscules and vesicles were detected. The absence of arbuscules was also reported by Demars & Boerner (Citation1994) in Capsella bursa-pastoris, a member of the Brassicaceae family, but in their study, internal hyphae, vesicles and chlamydospores were present. AM root colonization of broccoli ranged from 1.24% ± 2.77% to 9.99% ± 3.42%, whereas colonization was totally absent from the uninoculated control. This colonization rate was very low, however, and neither one of the AM fungal species was able to effectively colonize broccoli roots, confirming the low dependency of broccoli plants on AM fungi (Tanwar et al. Citation2013b). These results contrast with the earlier finding of Purakayastha et al. (Citation1998), who reported high levels of root colonization (60%) by G. macrocarpum and G. fasciculatum. This difference may be due to the different strains of Glomus that were investigated. However, even when colonization does occur, it is doubtful whether an effective symbiosis is formed between both partners with the mutual exchange of nutrients (Regvar et al. Citation2003). The non-susceptibility of broccoli roots to AM infection is due to the presence of aromatic glucosinolate 2-phenylglucosinolate, which has antifungal properties (Vierheilig et al. Citation2000).
Interestingly, it was found that inoculation with P. fluorescens did not have an inhibitory effect on AM fungi, which is why P. fluorescens are known as mycorrhiza helper bacteria (Azćon-Aguilar & Barea Citation1992). Increased mycorrhizal colonization in the presence of P. fluorescens has also been documented by others (Krey et al. Citation2011). However, it was found that when A. laevis and P. fluorescens were inoculated at the recommended fertilizer level (F2), spore numbers were 50% lower than in plants that had been treated with A. laevis alone. This could be ascribed to a lower compatibility of P. fluorescens with A. laevis than G. intraradices. High levels of P fertilizer also appear to be inhibitory to AM fungi, as root colonization was negligible at double the recommended dose (F3). A reduction in AM fungal growth and spore numbers at high soil P availability has also been recorded by various other researchers (Prasad et al. Citation2012; Tanwar et al. Citation2012), which may lead to the beneficial effect of AM fungi on the plants being less pronounced (Zubek et al. Citation2012).
The interactive effect of AM fungi, P. fluorescens and P fertilizer proved more effective than the sole application of AM fungi in terms of increasing plant chlorophyll content at F2 and F1, which may be due to higher nutrient absorption. However, there was no such positive effect on chlorophyll content in F3 fertilized plants due to the antagonistic effect of high amounts of P fertilizer on AM fungi and bacteria (Tanwar et al. Citation2012, Citation2013a). Similarly, Mehrvarz et al. (Citation2008) found that barley plants had higher levels of leaf chlorophyll pigment (a and b) following the co-inoculation of AM fungi and bacteria, and that high levels of P fertilizer had negative effects on bacteria survival.
Phosphatases are believed to be important for the scavenging and remobilization of phosphorus in plants (Rodríguez & Fraga Citation1999). Increased phosphatase activity at F1 and F2 confirms that these enzymes are only active at low soil P levels and that their activity is inversely proportional to the applied P rate. Alkaline phosphatases hydrolyse soil P, which is normally present in the form of polyphosphate, and translocate it to the plant via AM hyphae. However, the lack of effective colonization of broccoli roots by AM fungi eliminates their role in enzyme production. Therefore, these enzymes must be produced either by the plant roots themselves or by the colonized bacteria, i.e. P. fluorescens. Enhanced phosphatase activity through the application of P. fluorescens may help the plant to acquire the required amount of phosphate from the rhizosphere by catalysing the hydrolysis of several organic phosphate monoesters, which will liberate any available phosphate in the soil, allowing it to be absorbed by the plant roots.
The greatest increase in plant height and root length was observed in AM treated plants. These growth enhancing effects occurred when G. intraradices and A. laevis were applied on their own and when combined with P. fluorescens; however, there was much less of an effect when all three were applied together. These results are comparable to those of Pearson et al. (Citation1994), who found that the response of one fungal strain can vary with the presence of other AM strains as well as with the availability of P in the soil. However, the lack of effective AM colonization of broccoli roots suggests that AM fungi were not responsible for this stimulation, and so the exact reason for this is not known. The application of P. fluorescens also increased all other growth parameters in broccoli plants. This may be due to the production of a wide range of secondary metabolites, including siderophore, hydrogen cyanide and indole acetic acid, which have been found to have antagonistic effects on pathogenic fungi and the ability to promote plant growth (Park et al. Citation2009). Pseudomonas fluorescens has been shown to be highly efficient in solubilizing insoluble P and increasing the uptake of nutrients (especially phosphorus), resulting in better plant growth (Khan et al. Citation2009). Furthermore, Pseudomonas spp. are known to secrete ACC (1-aminocyclopropane-1-carboxylic acid) deaminase, which has been reported to be associated with increased root elongation due to its inhibitory effect on ethylene production (Gravel et al. Citation2007); and better root system development leads to increased absorption of nutrients from the soil, which, in turn, results in increased plant growth. In the present study, the application of bioinoculants had the greatest influence on plant growth promotion at F2 followed by F1, whereas plant growth was lowest at the highest fertilizer dose (F3). Similarly, Adesemoye et al. (Citation2009) found that the addition of PGPR in combination with 75% of the recommended dose of fertilizer resulted in increased plant height, shoot dry weight and root dry weight of tomato.
Plants that were treated with microbial inoculant had greater nutrient content than uninoculated control plants. Since the inoculants used (namely, AM fungi and P. fluorescens) are known to play a significant role in solubilizing inorganic phosphate and mineralizing organic phosphate, the combined inoculation of both can strongly enhance P uptake. However, the AM fungi used in the present study were not able to colonize broccoli roots, showing that the principle mechanism for the solubilization of P was via the production of organic acids and secretion of phosphatases by P. fluorescens. The inoculation of P. fluorescens in the soil at F2 led to greater plant nutrient content (P and N) than at F1. It is possible that at the lower soil P level, the P requirement of broccoli plants was partially satisfied by the P reserve of the soil, whereas when there was a greater supply of P in the soil, P was absorbed from the soil solution via the added bioagents. The application of high levels of chemical P fertilizer had an antagonistic effect on the bacteria and AM fungi (Mehrvarz et al. Citation2008). This effect was also observed with the A + Pf treatment, where the P content of shoots at F3 decreased to almost one quarter of the level at F2. It is possible that an excess of this nutrient might interfere with the assimilation of other nutrients (e.g. Cu, Zn), leading to reduced plant growth. Kohler et al. (Citation2008) demonstrated an enhanced uptake of P, N, iron (Fe), calcium (Ca) and manganese (Mn) in lettuce following inoculation with Pseudomonas mendocina along with AM fungi (G. mosseae and G. intraradices). An increase in the uptake of N could be attributed to the enhanced uptake of P, as there is thought to be a positive correlation between the two (Sharma et al. Citation2012).
The quality and yield of broccoli floret heads were greatly increased for all F1 and F2 fertilized plants, regardless of treatment. However, a further increase in fertilizer application dose (F3) had no significant effect on yield and inhibited head initiation in some of the treatments, possibly due to the poor structure of the plants as a result of excessive fertilizer uptake. Inoculation with P. fluorescens also proved highly effective in increasing most of the yield characteristics, and early head initiation was recorded in P. fluorescens inoculated plants at the recommended fertilizer application rate (F2). This indicates that phosphorus might have stimulated the plants to reach reproductive maturity earlier, which supports the previous findings of Islam et al. (Citation2010). These results are also in accordance with the findings of Shaharoona et al. (Citation2008), who found that PGPR increased root growth of wheat in a pot trial, although their efficacy decreased with increasing levels of NPK input.
Conclusions
Our results suggest that P. fluorescens has a potential role in P solubilization and plant growth improvement in broccoli. This bioagent increased broccoli yield and quality when applied with the recommended dose of phosphorus fertilizer, whereas higher concentrations of fertilizer led to lower growth. It should be noted, however, that results obtained under pot conditions might not always be reproducible under field conditions, and so field experiments are needed to confirm the efficacy of these bioinoculants for commercial use, and to determine the effect of intercropping with highly mycorrhizal plants on biotization, growth and yield.
Acknowledgements
The financial assistance in the form of a university research scholarship provided by Kurukshetra University, Kurukshetra, to AT is highly acknowledged. The authors also wish to thank Professor C.R. Darolia and Dr Hardeep Joshi, Department of Psychology, Kurukshetra University, Kurukshetra, for giving valuable suggestions regarding statistical analysis.
References
- Adesemoye AO, Torbert HA, Kloepper JW 2009. Plant growth-promoting rhizobacteria allow reduced application rates of chemical fertilizers. Microbial Ecology 58: 921–929. 10.1007/s00248-009-9531-y
- Adholeya A, Gaur A. 1994. Estimation of VAM fungal spores in soil. Mycorrhiza News 6: 10–11.
- American Dietetics Association 1992. Handbook of clinical dietetics. New Haven, CT, Yale University Press.
- Arnon DL 1949. C copper enzyme is isolated chloroplast polyphenol oxidase in Beta vulgaris. Plant Physiology 24: 1–15. 10.1104/pp.24.1.1
- Artursson V, Finlay R, Jansson JK 2006. Interactions between arbuscular mycorrhizal fungi and bacteria and their potential for stimulating plant growth. Environmental Microbiology 8(1): 1–10. 10.1111/j.1462-2920.2005.00942.x
- Azćon-Aguilar R, Barea JM 1992. Nodulation nitrogen fixation (15N) and N-nutrition relationship in mycorrhizal or phosphate amended alfalfa plants. Symbiosis 12: 33–41.
- Demars BG, Boerner REJ 1994. Vesicular-arbuscular mycorrhizal fungi colonization in Capsella bursa-pastoris (Brassicaceae). American Midland Naturalist 132: 377–380. 10.2307/2426593
- Farnham MW, Stephenson KK, Fahey JW 2005. Glucoraphanin levels in broccoli seed is largely determined by genotype. HortScience 40: 50–53.
- Gerdemann JW, Nicolson TH 1963. Spores of mycorrhiza Endogone species extracted from soil by wet sieving and decanting. Transactions of the British Mycological Society 46: 235–244. 10.1016/S0007-1536(63)80079-0
- Giovannetti M, Mosse B 1980. An evaluation of techniques for measuring vesicular–arbuscular infection in roots. New Phytologist 84: 489–500. 10.1111/j.1469-8137.1980.tb04556.x
- Giri B, Kapoor R, Mukerji KG 2005. Effect of arbuscular mycorrhizae Glomus fasciculatum and G. macrocarpum on the growth and nutrient content of Cassia siamea in a semi-arid Indian wetland soil. New Forests 29: 63–73. 10.1007/s11056-004-4689-0
- Gravel V, Antoun H, Tweddell RJ 2007. Growth stimulation and fruit yield improvement of greenhouse tomato plants by inoculation with Pseudomonas putida or Trichoderma atroviride: Possible role of indole acetic acid (IAA). Soil Biology and Biochemistry 39: 1968–1977. 10.1016/j.soilbio.2007.02.015
- Islam MH, Shaheb MR, Rahman S, Ahmed B, Islam ATMT, Sarker PC 2010. Curd yield and profitability of broccoli as affected by phosphorus and potassium. International Journal of Sustainable Crop Production 5: 1–7.
- Jackson ML 1973. Soil chemical analysis. New Delhi, Prentice Hall of India Pvt. Ltd.
- Kamalakannan S, Manivannan K 2002. Influence of Azospirillum, phosphobacteria and vesicular arbuscular mycorrhizae on growth parameters on radish (Raphanus sativus L.). Research on Crops 3: 138–141.
- Khan AA, Jilani G, Akhtar MS, Naqvi MS, Rasheed M 2009. Phosphorus solubilizing bacteria: occurrence, mechanisms and their role in crop production. Journal of Agricultural and Biological Sciences 1: 48–58.
- Kohler J, Hernández JA, Caravaca F, Roldán A 2008. Plant-growth-promoting rhizobacteria and arbuscular mycorrhizal fungi modify alleviation biochemical mechanisms in water-stressed plants. Functional Plant Biology 35: 141–151. 10.1071/FP07218
- Krey T, Caus M, Baum C, Ruppel S, Eichler-Löbermann B 2011. Interactive effects of plant growth–promoting rhizobacteria and organic fertilization on P nutrition of Zea mays L. and Brassica napus L. Journal of Plant Nutrition and Soil Science 174: 602–613. 10.1002/jpln.200900349
- Lambers H, Shane MW, Cramer MD, Pearse SJ, Veneklaas EJ 2006. Root structure and functioning for efficient acquisition of phosphorus: matching morphological and physiological traits. Annals of Botany (London) 98: 693–713. 10.1093/aob/mcl114
- Malik ME, Kumar V 2007. Effect of nitrogen and phosphorus on biological yield of broccoli (Brassica oleracea var. italica Plenck.). Haryana Journal of Horticultural Science 36: 133–134.
- Mehrvarz S, Chaichi MR, Alikhani HA 2008. Effects of phosphate solubilizing microorganisms and phosphorus chemical fertilizer on yield and yield components of barely (Hordeum vulgare L.). American-Eurasian Journal of Agriculture and Environmental Science 3: 822–828.
- Menge JA, Timmer LW 1982. Procedure for inoculation of plants with VAM in the laboratory, greenhouse and field. In: Schenck NC ed. Methods and principles of mycorrhizal research. St. Pauls, American Phyto-Pathological Society, Pp. 59–67.
- Miller SP, Bever JD 1999. Distribution of arbuscular mycorrhizal fungi in stands of the wetland grass Panicum hemitomon along a wide hydrologic gradient. Oecologia 119: 586–592. 10.1007/s004420050823
- Morton JB, Benny GL 1990. Revised classification of arbuscular mycorrhizal fungi (Zygomycetes): a new order, Glomales, two new suborders, Glomineae and Giagasporineae, and two new families, Acaulosporaceae and Gigasporaceae, with an emendation of Glomaceae. Mycotaxon 37: 471–491.
- Morton JB, Redecker D 2001. Two new families of Glomales, Archaeosporaceae and Paraglomaceae, with two new genera Archaeospora and Paraglomus based on concordant molecular morphological characters. Mycologia 93: 181–195. 10.2307/3761615
- Mukerji KG 1996. Taxonomy of endomycorrhizal fungi. In: Mukerji KG, Mathur B, Chamola B, Chitralekha P eds. Advances in botany. New Delhi, A.P.H. Publishing Corporation. Pp. 211–221.
- Orlowska E, Zubek SZ, Jurkiewicz A, Szarek-Àukaszewska G, Turnau K 2002. Influence of restoration on arbuscular mycorrhiza of Biscutella laevigata L. (Brassicaceae) and Plantago lanceolata L. (Plantaginaceae) from calamine spoil mounds. Mycorrhiza 12(3): 153–160. 10.1007/s00572-001-0155-4
- Ouda BA, Mahadeen BA 2008. Effect of fertilizers on growth, yield and yield components, quality and certain nutrient contents in broccoli (Brassica oleracea). International Journal of Agricultural and Biology 10: 627–32.
- Park KH, Lee CN, Son HJ 2009. Mechanism of insoluble phosphate solubilization by Pseudomonas fluorescens RAF15 isolated from ginseng rhizosphere and its plant growth-promoting activities. Letters in Applied Microbiology 49: 222–228. 10.1111/j.1472-765X.2009.02642.x
- Pearson JN, Abbott LK, Jasper DA 1994. Phosphorus, soluble carbohydrates and the competition between two arbuscular mycorrhizal fungi colonizing subterranean clover. New Phytologists 127: 101–106. 10.1111/j.1469-8137.1994.tb04263.x
- Phillips JM, Hayman DS 1970. Improved produces for clearing roots and staining parasitic and VAM fungi for rapid assessment of infection. Transactions of the British Mycological Society 55: 158–161. 10.1016/S0007-1536(70)80110-3
- Plenchette C, Fortin JA, Furlan V 1983. Growth responses of several plant species to mycorrhizae in a soil of moderate P-fertility. I. Mycorrhizal dependency under field conditions. Plant and Soil 70: 199–209. 10.1007/BF02374780
- Podsedek A 2007. Natural antioxidants and antioxidant capacity of Brassica vegetables: a review. LWT- Food Science and Technology 40: 1–11. 10.1016/j.lwt.2005.07.023
- Pongrac P, Vogel-Mikus K, Kump P, Necemer M, Tolrá Poschenrieder C, Barceló J, Regvar M 2007. Changes in elemental uptake and arbuscular mycorrhizal colonisation during the life cycle of Thlaspi praecox Wulfen. Chemosphere 69: 1602–1609. 10.1016/j.chemosphere.2007.05.046
- Prasad K, Aggarwal A, Yadav K, Tanwar A 2012. Impact of different levels of superphosphate using arbuscular mycorrhizal fungi and Pseudomonas fluorescens on Chrysanthemum indicum L. Journal of Soil Science and Plant Nutrition 12: 451–462.
- Purakayastha TJ, Singh CS, Chhonkar PK 1998. Growth and iron nutrition of broccoli (Brassica oleracea L. var. Italica Plenck), grown in a Typic Ustochrept, as influenced by vesicular-arbuscular mycorrhizal fungi in the presence of pyrite and farmyard manure. Biology and Fertility of Soils 27: 35–38. 10.1007/s003740050396
- Regvar M, Vogel K, Irgel N, Wraber T, Hildebrandt U, Wilde P, Bothe H 2003. Colonization of pennycresses (Thalaspi spp.) of the Brassicaceae by arbuscular mycorrhizal fungi. Journal of Plant Physiology 160: 615–626. 10.1078/0176-1617-00988
- Richardson AE, Simpson RJ 2011. Soil microorganisms mediating phosphorus availability. Plant Physiology 156: 989–996. 10.1104/pp.111.175448
- Rodríguez H, Fraga R 1999. Phosphate solubilizing bacteria and their role in plant growth promotion. Biotechnology Advances 17: 319–339. 10.1016/S0734-9750(99)00014-2
- Sanyal SK, De Datta SK 1999. Chemistry of phosphorus transformation in soil. Advances in Soil Sciences 16: 1–120.
- Schenck NC, Perez Y 1990. Manual for the identification of VA mycorrhizal (VAM) fungi. Florida, USA, University of Florida Press.
- Shaharoona B, Naveed M, Arshad M, Zahir ZA 2008. Fertilizer dependent efficiency of Pseudomonads for improving growth, yield, and nutrient use efficiency of wheat (Triticum aestivum L.). Applied Microbiology and Biotechnology 79: 147–155. 10.1007/s00253-008-1419-0
- Sharma A, Rawat US, Yadav BK 2012. Influence of phosphorus levels and phosphorus solubilizing fungi on yield and nutrient uptake by wheat under sub-humid region of Rajasthan, India. ISRN Agronomy.
- Singh J, Rai M, Upadhyay AK, Bahadur A, Chaurasia SNS, Singh KP 2006. Antioxidant phytochemicals in broccoli (Brassica oleracea L. var. italica Plenck) cultivars. Journal of Food Science and Technology 43: 391–393.
- Singh M, Rana DK, Rawat JMS, Rawat SS 2011. Effect of GA3 and kinetin on growth, yield and quality of sprouting broccoli (Brassica oleracea var. italica). Journal of Horticulture and Forestry 3: 282–285.
- Singh J, Upadhyay AK, Bahadur A, Singh KP 2004. Dietary antioxidant and minerals in Crucifers. Journal of Vegetable Crop Production 10: 33–41.
- Tabatabai MA, Bremner JM 1969. Use of p-nitrophenol for assay of soil phosphatase activity. Soil Biology and Biochemistry 1: 301–307. 10.1016/0038-0717(69)90012-1
- Tanwar A, Aggarwal A, Kadiyan N, Gupta A 2013a. Arbuscular mycorrhizal inoculation and rock phosphate application influence plant growth and yield of Capsicum annuum L. Journal of Soil Science and Plant Nutrition 13: 55–66.
- Tanwar A, Aggarwal A, Karishma, Neetu 2012. Effectiveness of endomycorrhizal fungi and Pseudomonas fluorescens under different phosphorus levels on Capsicum annuum L. Kasetsart Journal (Natural Sciences) 46: 769–782.
- Tanwar A, Aggarwal A, Kaushish S, Chauhan S 2013b. Interactive effect of AM fungi with Trichoderma viride and Pseudomonas fluorescens on growth and yield of broccoli. Plant Protection Science 49: 140–148.
- Tanwar A, Aggarwal A, Yadav A, Parkash V 2013c. Screening and selection of efficient host and sugarcane bagasse as substrate for mass multiplication of Funneliformis mosseae. Biological Agriculture and Horticulture: An International Journal for Sustainable Production Systems 29: 107–117. 10.1080/01448765.2013.771955
- Tong Y, Scheiner M, George E, Gabriel-Neumann E 2011. Mycorrhizal effect on the interaction between sesame and broccoli roots in a model system. Second annual joint meeting, 6 September 2011, Embassy of the French Republic, Berlin, Pariser Platz 5.
- Vierheilig H, Bennett R, Kiddle G, Kaldorf M, Ludwig-Müller J 2000. Differences in glucosinolate patterns and arbuscular mycorrhizal status of glucosinolate-containing plant species. New Phytology 146: 343–352. 10.1046/j.1469-8137.2000.00642.x
- Walker C 1986. Taxonomic concepts in the Endogonaceae. II. A fifth morphological wall type in endogonaceous spores. Mycotaxon 25: 95–105.
- Watt BK 1983. Nutritive value of fruits and vegetables. USAID handbook No. 8. Cited in Nonnecke IL 1989. Vegetable production. New York, Van Nostrand Reinhold. Pp. 369–414.
- Zubek S, Mielcarek S, Turnau K 2012. Hypericin and pseudohypericin concentrations of a valuable medicinal plant Hypericum perforatum L. are enhanced by arbuscular mycorrhizal fungi. Mycorrhiza 22: 149–156. 10.1007/s00572-011-0391-1