ABSTRACT
White peach scale (Pseudaulacaspis pentagona) is a polyphagous pest of perennial horticulture in Asia and many other warm-temperate and tropical regions. In China, it is a serious pest of kiwifruit. The optimal time to control P. pentagona field populations is at the first instar stage of the first, spring generation. Three methods for predicting the hatching of eggs of the first generation from field populations were tested on kiwifruit in Sichuan, based upon the accumulation of heat units (day-degrees) above a developmental threshold, calculated as 10.75 °C. A method developed for P. pentagona control in tea in Japan gave excellent predictions of 50% hatching in 5 of the 6 years under study (2009–2014). The other two methods also gave acceptable predictions of dates of 50% egg hatching (within 5 days of field observed dates). These models may be used as a basis for forecasting spring insecticide application dates targeting the first instar stage (crawlers and settled ‘white caps’).
Introduction
White peach scale (WPS; Pseudaulacaspis pentagona [Targioniet et Tozzetti]) is a cosmopolitan polyphagous pest of perennial horticultural plants. It has a temperate to tropical distribution, with two or three generations a year (Mazzoni & Cravedi Citation1995; Erkilic & Uygun Citation1997; Mazzoni & Cravedi Citation1999; Takeda Citation2006). WPS can cause serious damage to a wide range of fruit and ornamental species and has been responsible for dieback and yield loss on kiwifruit, stonefruit and ornamentals in Europe, North America and China (Miller & Davidson Citation1990; Hanks & Denno Citation1993; Hill et al. Citation2008) and tea in Japan (Tatara Citation1999; Takeda Citation2006). Immature armoured scale insects are much easier to control than mature females when using contact (non-systemic) insecticides, and the timing of insecticide applications, targeting the first instar (mobile crawler or newly settled ‘white cap’), is important for achieving good control (Blank & Olson Citation1987; Tatara Citation1999). The emergence of crawlers (newly eclosed first instar nymphs) of the first (spring) generation of P. pentagona is more synchronised than that of later generations. Thus, the most effective time to apply a contact insecticide against a P. pentagona population is in spring at the end of egg hatching when most of the population is in the first instar stage (Hill et al. Citation2007). In Japan, it has been estimated that the optimal time to apply insecticide for control of the first generation of P. pentagona is 2–5 days after peak catches of WPS crawlers on sticky traps in tea bushes (Tatara Citation1999).
This research was carried out at the Sichuan Provincial Academy of Natural Resource Science (SPANRS) Research Centre, Shifang, Sichuan (662 m above sea level, 31°13.663′N, 101°01.161′E) and adjacent commercial orchards. In China, commercial kiwifruit cultivation has increased rapidly over the past decade, with the development and release of new varieties. There are now an estimated 106,000 ha under cultivation in China, including 33,000 ha in Sichuan. The red-fleshed cultivar Actinidia chinensis ‘Hongyang’, which is very susceptible to P. pentagona, has been planted on approximately 26,000 ha in Sichuan. Mineral oil and organophosphate sprays are widely used for P. pentagona control on kiwifruit in Sichuan (QG Zhuang, unpubl. data). The P. pentagona population at Shifang is biparental, with three generations a year. Overwintering occurs as mated females. The purpose of this study was to measure the phenology of first generation crawler emergence on kiwifruit vines in spring in Sichuan, and to determine which of three heat-unit (degree-day) models was best for predicting crawler emergence and forecasting spray timing.
Methods
Both field and laboratory observations of P. pentagona oviposition and hatching were conducted, along with detailed observations of egg development rate at a range of constant laboratory temperatures.
Field studies
In spring, observations of P. pentagona adult female scale insects were made on vines in the field at Shifang by lifting scale caps and looking for eggs. Once egg laying had commenced, a minimum of 100 females were examined every 2 days. When >50% of females examined on consecutive dates had begun to lay eggs, the second day was designated as the biofix date i.e. the day on which >50% of females had commenced oviposition. These data were collected from 2009 to 2014.
To determine the phenology of the first generation P. pentagona, crawlers of the spring generations of 2008–2014 trapped on sticky bands on canes of A. chinensis ‘Hongyang’ vines in the field were counted every 3–4 days. Bands (30–40, each 1.5 cm wide) were applied at the rate of 2–5 bands per vine (depending on insect abundance) at two sites 1 km apart. Data from each site were pooled.
Laboratory studies
In 2008 and 2009, A. chinensis ‘Hongyang’ canes infested with P. pentagona were taken from the field in spring, set up in water in a laboratory at 20–22 °C and observed daily for the onset of egg laying. As soon as >50% of females examined on consecutive days had begun to lay eggs, six to eight canes were placed at four constant temperatures in 2008 (17, 20, 23 and 25 °C) and 2009 (17, 20, 23 and 26 °C). Crawler emergence was measured by counting the numbers of crawlers trapped in sticky bands on the canes every 3–4 days (crawlers are mobile for <24 h, after which they settle on the cane and become sessile). The % crawler emergence was plotted against day-degree accumulations from the onset of the experiment above a developmental threshold of 10.75 °C and a logistic equation:
was fitted to predict % crawler emergence from accumulated day-degrees from the date on which 50% of females had begun to lay eggs (i.e. the biofix date).
Kiwifruit (A. chinensis ‘Hongyang’) canes (c. 60 cm long) infested with female P. pentagona were cut from vines between 24 February and 14 April 2009, transferred to a laboratory and held at 25 °C with the cut end of the cane inserted in a water bath. The female P. pentagona were observed every 1 or 2 days until egg laying was observed. Freshly laid eggs (0–6 h old) were taken from the females and put on to sprouting potato tubers (a commonly used host for rearing diaspidids [Rosen Citation1990]) held at six constant temperatures (17, 20, 23, 25, 26 and 29 °C) and an RH of 60%–70% in controlled environment chambers. Between 400 and 1000 eggs were placed on each potato and three or four potatoes were placed at each temperature. The potato tubers had double-sided sticky tape wrapped around them. This tape trapped the P. pentagona crawlers after their emergence from the eggs. The number of crawlers trapped by the tape was counted at 12 h intervals using a binocular microscope.
The development rate (1/time) of the egg stage from laying to 50% hatch on each potato was plotted against temperature, and the developmental threshold and heat units (day-degrees) were calculated using the standard linear regression equation T = C + KV; where T is the development rate (1/days) for eggs from laying to hatching, V is constant temperature and the developmental threshold is C/K.
Models
Three methods were used to predict crawler emergence and are described below. All three methods accumulate day-degrees (average of daily maximum and minimum temperatures) above a temperature threshold from a defined time point. Daily maximum and minimum temperatures were recorded from a weather station operating at the Shifang field station. Two of the methods (Takeda and empirical) start temperature accumulations from a pre-determined time point. Takeda’s starts from 1 January, while the empirical (data-mining) method starts from a date that minimises the error in estimating the crawler field emergence data over 4 years (2009–2012) (Ring & Harris Citation1983; Hill & McLaren Citation1988). The third, ‘biofix’ method relies on field observations each year to determine the date on which >50% of the population begins to lay eggs and uses that as the date from which day-degrees are accumulated. Day-degrees were summed until the date of 50% crawler emergence.
Takeda’s model (Takeda Citation2004; Figure 9) is:
where y is cumulative proportion of hatched eggs and x is day-degrees accumulated from 1 January above a threshold of 10.5 °C.
The empirical method used combinations of base temperature (0–12 °C in 1 °C increments) and starting dates (days from 1 January to 1 March) that minimised the root mean square error (RMSE) of deviations between the observed day-degrees accumulated per year for 10%, 50%, 75% and 90% crawler emergence and the means of these events over all 4 years (2009–2012) was determined with a MS Excel macro. Predictions of crawler emergence in future years (2013 and 2014) were made by summing day-degrees from the calculated optimal starting date and base temperature up to the mean day-degrees required for each crawler % emergence event.
In the third, biofix method, the logistic equation developed from laboratory data relating % crawler emergence to average daily temperatures above 10.75 °C was used to predict crawler emergence. Day-degrees were accumulated from the biofix date when 50% of insects were observed to have begun oviposition in the field.
Results
There was a linear relationship between egg development rate (1/days to 50% egg hatch) and constant rearing temperatures between 17 °C and 29 °C; egg development rate = 0.0099 T – 0.1062 (where T is temperature in °C; adjusted r2 = 0.962) (data summary in ). The development threshold was estimated as 10.75 ± 0.7 (SEM) °C and the mean degree-days required for 50% egg hatch (K) was 100.8 ± 5.0 (SEM).
Table 1. Data used to calculate the development rate–constant temperature relationship for P. pentagona egg hatching.
The relationship based on laboratory observations in 2008 and 2009 between cumulative % emergence of crawlers (% egg hatch) and cumulative day-degrees above 10.75 °C at four constant temperatures from the time when 50% of the females had begun to lay eggs is shown in . The fitted curve (thick solid line) describing the relationship between % crawler emergence and day-degrees above 10.75 °C is:
Figure 1. The relationship between cumulative % emergence of first generation Pseudaulacaspis pentagona crawlers on Actinidia chinensis ‘Hongyang’ canes and heat unit accumulations at four constant temperatures over 2 years.
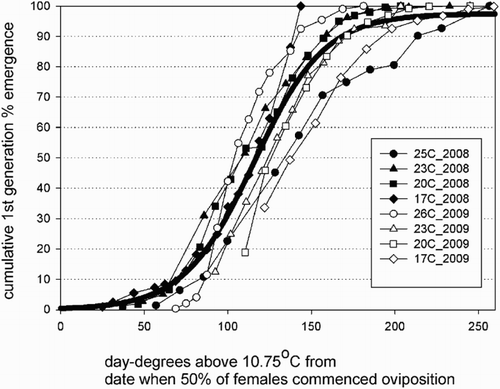
where DD is cumulative day-degrees above 10.75 °C from the biofix date when 50% of females have begun to lay eggs. Optimisation of base temperatures and start dates for estimating the 10th, 50th, 75th and 90th percentiles of crawler emergence () gave RMSE minima with threshold temperatures ranging from 0–5 °C and start dates from 17–25 February. Threshold temperatures were much lower than those calculated from laboratory-derived development data in this paper (10.75 °C) or by Takeda (Citation2004) (10.5 °C).
Table 2. Empirical model outputs.
The 50% crawler emergence date varied by 20 days over the 6 years of observations (), with 2012 having the latest and 2013 the earliest dates for crawler emergence.
Figure 2. Emergence curves for first generation Pseudaulacaspis pentagona crawlers on Actinidia chinensis ‘Hongyang’ at Shifang, Sichuan (●), and predictions from three models: (1) Takeda (Citation2004) (▪); (2) biofix (▴); and (3) data mining (○) for 6 years (data mining for 2 years only).
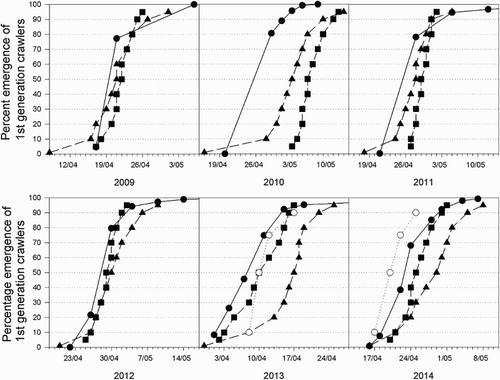
The predicted crawler emergence dates from the three models (Takeda, empirical and biofix) are shown in and . The model of Takeda (Citation2004) predicted the 50% emergence date within 2 days of the field-estimated date in all but 1 year (2010), when it was 10 days late. The biofix model prediction was within 3 days of the field-estimated 50% emergence date in 4 years (). However, it failed to predict crawler emergence accurately in 2013 when temperatures were very high (8 days late) and in 2010 (7 days late). The data-mining model was 3 days late in 2013 and 2 days early in 2014.
Table 3. Date of 50% crawler emergence of the first (spring) generation of Pseudaulacaspis pentagona on Actinidia chinensis ‘Hongyang’ and results of three model predictions for 50% crawler emergence in the field for 6 years at Shifang, Sichuan, China.
Discussion
The developmental threshold for P. pentagona eggs in this study (10.75 ± 0.7 °C) was not significantly different from that calculated by Takeda (10.5 °C) and is similar also to the P. pentagona first and second stage developmental thresholds of 10 °C calculated by Erkiliç and Uygun (Citation1997) in Turkey, but substantially higher than the figure of 6.3 °C calculated for nymphal and adult stage P. pentagona development in Italy (Mazzoni & Cravedi Citation1999).
This article is concerned with predicting 50% crawler hatching not with spray timing per se. Further research is needed to implement appropriate extension spray recommendations based on these findings and among other things, local conditions, weather forecasting, insecticide used and grower practices. However, for the purposes of this discussion, we assume that a forecast accuracy of 5 days for 50% crawler hatch is likely to be adequate in practice. On that basis, the biofix model was sufficiently accurate for spray prediction purposes (estimated 50% crawler emergence within 5 days of field observation) in 4 of the 6 years, but proved inaccurate (>1 week late) in the hottest (2013) and coldest (2010) years, suggesting that it is not very robust. An advantage of this model is that it is easy for growers to adopt. They can be easily trained to record the biofix date on their vines and accumulate day-degrees from daily maximum and minimum daily temperatures recorded by them in their orchards over the subsequent 2–3 week period. However, its relative inaccuracy may limit its usefulness.
The method of Takeda (Citation2004) was better than our biofix method (prediction within 5 days of actual in 5 years) and worked surprisingly well considering that it was developed on tea in Japan. Takeda (Citation2004) measured the proportional hatching of egg batches, whereas we monitored crawler emergence using sticky traps. These methods should produce similar phenologies as long as all or most eggs are laid before the commencement of 50% hatching (if this is the event to be predicted), and as long as it is possible to distinguish dead eggs from those that have not yet hatched. Our experience is that estimating the proportion of hatched eggs in an egg batch is difficult and time consuming compared with using sticky bands to monitor crawlers.
The empirical (data-mining) method was also accurate enough to forecast 50% crawler emergence within 5 days for 2013 and 2014, using the preceding 4 years’ data to build the model. A strength of this method is that it makes no prior assumptions regarding the relationship between base temperature for development, starting date for heat unit accumulation, and heat units accumulated. Thus is does not require the gathering of laboratory data on insect development at constant temperatures and the building of a temperature-development model from these data. Its disadvantage is that it requires a minimum of 3–4 years’ field data before the model can be developed. However, once sufficient data are available, the model can be updated every year. Its success in predicting crawler emergence in both 2013 and 2014 suggests that it will be useful for practical spray forecasting. However, its empirical nature means that it may not generalise to other districts well, and this will require further testing.
Both the biofix and Takeda model predictions were notably inaccurate for 2010 crawler emergence predictions. Plots of mean daily temperature and deviations in the mean daily temperature from the 6 year average during the period of crawler emergence (days 90–120 where 1 January is day 1) (Figures S1–S2) show that 2010 was unusually cold compared with the other years, leading up to and during crawler emergence, with 4 consecutive days just before crawler emergence being more than 4 °C colder than average (Figure S2). This inaccuracy may reflect the weakness of a linear developmental model when temperatures remain close to or below a linear developmental threshold for several days close to hatching. Given the excellent predictive ability of the Takeda model in 5 out of 6 years, this is not a fatal flaw, and it would be possible to develop and apply a correction to forecasts of 50% hatching dates in unusually cold years.
Of the three P. pentagona phenology prediction methods, Takeda’s is the most accurate, while the biofix method would be the most convenient for growers to use on their orchards. The empirical method, which was accurate enough for spray forecasting in the 2 years available for testing, should also be relatively easy for growers to use, as heat units are accumulated over a much shorter time than for the Takeda method. The models are sufficiently robust to be implemented in trial forecasts for spring insecticide applications in commercial orchards around Shifang, Sichuan and if successful, extended for testing at regions further away from these experimental sites.
Spraying of contact insecticides (organophosphates or mineral oils) should be timed to coincide with the period when most of the population is in the first instar stage, as this stage is the easiest to kill with insecticides (Blank & Olson Citation1987; Miller & Davidson Citation2005). Zhuang (Citation2012) calculated that the P. pentagona first instar stage on kiwifruit in Sichuan lasts for 141 day-degrees summed above the developmental threshold of 10.75 °C. This represents an average duration of 14.8 days (range 11–17 days; 2009–2014). The average period over which crawler emergence was observed was about 20 days (), but most of the emergence occurred within less than 1 week. Thus, if ≥50% crawler emergence occurs within 7–10 days of the onset of hatching, we estimate that optimal times for spraying are 5–10 days after the predicted date of 50% crawler emergence. These models for spray forecasting and P. pentagona control in kiwifruit orchards in Sichuan now require field validation by extension workers and growers. Given the important pest status of P. pentagona on New Zealand kiwifruit varieties grown in Europe (Hill et al. Citation2008) and elsewhere (New Zealand is the only kiwifruit-growing country to be free of P. pentagona [CABI Citation2015]), it would be worthwhile to test these models for insecticide spray timing in other locations where kiwifruit is grown commercially.
Supplementary data
Figure S1. Deviations of mean daily temperatures in 2009 and 2010 from average mean daily temperatures for the period 2009 to 2014 from 1 January to 10 May, at Shifang, Sichuan, China. Solid black bars represent the main period of Pseudaulacaspis pentagona crawler emergence in each year (see ).
Figure S2. Mean daily temperatures for the 6 years from 2009 to 2014 at Shifang, Sichuan, China, from 90 to 120 days since 1 January (1 April to 10 May), covering the main period of Pseudaulacaspis pentagona crawler emergence (see for crawler emergence plots for each year), and the deviations over the same time period of mean daily temperatures in 2010 compared with average mean daily temperatures for the period 2009–2014. Both graphs show how unusually cold 2010 was leading up to and during crawler release.
Acknowledgements
We are grateful to an anonymous reviewer and David Logan for very helpful comments on a draft.
Disclosure statement
No potential conflict of interest was reported by the authors.
Additional information
Funding
References
- Blank RH, Olson MH. 1987. Differential toxicological responses of greedy scale stages to diazinon. Proc New Zeal Weed Pest Control Conf. 40:161–164.
- CABI. 2015. Pseudaulacaspis pentagona. In: Crop protection compendium. Wallingford, UK: CAB International; [cited 2015 Dec 1]. Available from: www.cabi.org/cpc.
- Erkilic LB, Uygun N. 1997. Development time and fecundity of the white peach scale, Pseudaulacaspis pentagona, in Turkey. Phytoparasitica. 25:9–16. doi: 10.1007/BF02981474
- Hanks LM, Denno RF. 1993. The white peach scale, Pseudaulacaspis pentagona (Targioni-Tozzetti) (Hemiptera: Diaspididae): life history in Maryland, host plants, and natural enemies. P Entomol Soc Wash. 95:79–88.
- Hill MG, McLaren GF. 1988. Predicting European red might hatch on apricots 1. A day-degree model. Proc New Zeal Weed Pest Control Conf. 40:90–93.
- Hill MG, Pollini A, Rinaldi Ceroni M, Spinelli R, Dobson S. 2008. Strategie di difesa contro Pseudaulacaspis pentagona su Actinidia. ATTI Giornate Fitopatologiche. 1:159–166.
- Hill MG, Pollini A, Rinaldo-Ceroni M, Spinelli R, Dobson SJ. 2007. Controlling white peach scale on ‘Hort16A’ in Italy. Acta Hortic. 753:693–702. doi: 10.17660/ActaHortic.2007.753.91
- Mazzoni E, Cravedi P. 1995. Temperature and developmental rate of Pseudaulacaspis pentagona (Targioni-Tozzetti) (Homoptera: Diaspididae). In IOBC WPRS working group ‘Integrated plant protection in stone fruit’ held at Nimes, France; 1994 Sep 6–8, 18:24–27.
- Mazzoni E, Cravedi P. 1999. White peach scale phenology and climatic parameters. IOBC wprs Bulletin (Integrate Plant Protection in Stone Fruit). 22:101–106.
- Miller D, Davidson JA. 1990. A list of the armoured scale insect pests. In: Rosen D, editor. Armored scale insects, their biology, natural enemies and control. Amsterdam: Elsevier; p. 299–306.
- Miller DR, Davidson JA. 2005. Armoured scale insect pests of trees and shrubs. Ithaca: Cornell University Press; 442 p.
- Ring DR, Harris MK. 1983. Predicting pecan nut casebearer (Lepidoptera: Pyralidae) activity at College Station, Texas. Environ Entomol. 12:482–486. doi: 10.1093/ee/12.2.482
- Rosen D, editor. 1990. Armored scale insects: their biology, natural enemies, and control, volume A. Amsterdam: Elsevier.
- Takeda M. 2004. Effects of temperature on oviposition in overwintering females and hatch in first-generation larvae of Pseudaulacaspis pentagona (Hemiptera: Diaspididae). Appl Entomol Zool. 39:15–26. doi: 10.1303/aez.2004.15
- Takeda M. 2006. Effect of temperature on the maintenance and termination of diapause in overwintering females of Pseudaulacaspis pentagona (Hemiptera: Diaspididae). Appl Entomol Zool. 41:429–434. doi: 10.1303/aez.2006.429
- Tatara A. 1999. Determination of optimum spraying time for chemical control of mulberry scale, Pseudaulacaspis pentagona (Targioni) (Hemiptera: Diaspididae) in tea fields. JARQ-Jpn Agr Res Q. 33:155–161.
- Zhuang QG. 2012. Susceptibiltiy of kiwifruit germplasm to white peach scale Pseudaulacaspis pentagona (Homoptera: Coccoidea) and management of armoured scale insects [Unpublished PhD thesis]. Chengdu, Sichuan: University of Sichuan.