ABSTRACT
Ethylene is a potent phytohormone that influences a number of physiological and morphological characters in plants, and its biosynthesis is regulated by several factors, including nutrient availability. This study investigated the effect of using paint-coated calcium carbide (PCC) as a source of ethylene on nitrogen metabolism, photosynthesis and yield in cucumber (Cucumis sativus L.). The application of PCC significantly enhanced plant ethylene production regardless of the addition of nitrogen and there was a concurrent improvement in the morphophysiological attributes of cucumber. PCC application (30 mg kg−1) in combination with N (60 mg kg−1) significantly improved the leaf area, number of primary branches, nitrate reductase activity, nitrogen contents, photosynthetic rate, stomatal conductance, carboxylation efficiency, water use efficiency and fruit yield in cucumber. Therefore, the addition of soil-applied PCC and N could potentially be used to achieve a higher fruit yield in cucumber by improving nitrogen assimilation and photosynthetic characteristics.
Introduction
Cucumber (Cucumis sativus L.) is an important vegetable crop that is cultivated all over the world for its economic and nutritional benefits. Mature cucumber fruits are used in salads while the immature fruits are used to produce pickles. The growth and development of cucumber are adversely affected in stressful environments, including under nutrient-deficient conditions (Yang et al. Citation2009). Nitrogen (N) is an important phytonutrient that is usually required in large amounts, with its deficiency generally limiting plant growth and development (Iqbal et al. Citation2015). Plants assimilate N by reducing nitrate to nitrite and then ammonium through the action of respective reductase enzymes. Thus, N functions as a signalling element that sustains plant growth and development, particularly in stressful conditions (Mi et al. Citation2008; Mohd-Radzman et al. Citation2013). It has previously been shown that both deficient and excessive amounts of N have a significant negative impact on mustard (Brassica juncea; Iqbal et al. Citation2015), demonstrating that the amount of N needs to match the optimum requirements of a particular crop.
Plants respond to different external factors and adjust their physiological processes and molecular mechanisms by producing regulatory molecules to improve their growth and productivity (Khan & Singh Citation2008). Ethylene is one such signalling molecule that interacts with nutrients to influence physiological processes (Iqbal et al. Citation2013). Exogenous ethylene affects ethylene biosynthesis in various plant parts through homeostasis. Since ethylene is a potent phytohormone, small changes in its production result in the re-regulation of a number of vital plant processes, such as photosynthesis, cell division, cell elongation, and the metabolism of mineral nutrients (Khan et al. Citation2008). Like other nutrients, the availability of N is known to regulate the biosynthesis of ethylene (Khan et al. Citation2015). For instance, Fiebig and Dodd (Citation2015) reported that tomato (Solanum lycopersicum) plants reduce ethylene synthesis back to the normal level following the addition of 10 mM N. Similarly, the application of N improves photosynthesis in mustard plants through the regulation of the phytohormones proline and ethylene (Iqbal et al. Citation2015), but excess N is related to a higher production of ethylene.
In addition to nutrients, various precursors of ethylene have also been used to induce ethylene production in plants, including ethephon, ethrel and 1-aminocyclopropane-1-carboxylase (ACC). Khan et al. (Citation2008) reported that the application of ethephon in the presence of N improved the growth, physiology and N status of mustard through the production of ethylene; and similarly, Iqbal et al. (Citation2011) showed that the use of ethephon and ethrel in combination with 80 mg N kg−1 soil increased ethylene production, and improved the photosynthetic and carboxylation efficiency in mustard. To date, most studies have investigated the role of these various ethylene sources on the morphophysiological characters of plants using a foliar application. However, the calcium carbide (CaC2)-based ethylene producer ‘Retprol’ was one of the first products to be used for ethylene production and was applied as a soil amendment (Muromtsev et al. Citation1990). Upon reacting with soil moisture, CaC2 produces acetylene (C2H2) gas, which is later reduced to ethylene through the action of the native soil microbial community (Yaseen et al. Citation2006; Kashif et al. Citation2008). Recently, the application of coated CaC2 has been reported to enhance the growth, photosynthetic activity and nitrogen uptake in tomato (Siddiq et al. Citation2012), sweet pepper (Capsicum annum; Ahmed et al. Citation2014) and cucumber (Shakar et al. Citation2016). However, despite these positive effects of CaC2, it has been reported that commercial grade CaC2 contains traces of arsenic (0.001–0.0001% by volume) and phosphorus hydride (Asif Citation2012). Therefore, it is advised that only pure grade CaC2 is added to the soil or coated with organic matter to avoid this risk.
It has been well documented that addition of the precursor of ethylene (ethephon) either alone (Öztürk et al. Citation2008) or in combination with N (Khan et al. Citation2008; Iqbal et al. Citation2011) has a significant effect on plant growth and development. However, little is known about the effects of CaC2 in combination with different levels of N fertilizers on physiological and growth indices of cucumber under field conditions. We hypothesised that soil-applied paint-coated calcium carbide (PCC) would induce ethylene production in cucumber, which would positively regulate the biochemical profile and increase fruit yield. Therefore, the specific objective of this research was to evaluate the effect of soil-applied PCC on endogenous ethylene production and concomitant changes in N assimilation, photosynthetic characteristics and fruit yield in cucumber.
Materials and methods
Site description
The experiment was conducted in a polyhouse at a temperature of 24 ± 4°C and a relative humidity of 60–80%. The soil in the polyhouse field was sandy clay loam (pH 8.0), which had an electrical conductivity of 2.41 dS m−1, and contained 0.04% total N, 5.06 ppm available P and 0.98% total organic matter.
Experimental setup
Cucumber plants (Bolan-F1 hybrid) were cultivated in a nursery on a compost and sand mix, following which 15-day-old seedlings were transplanted to the polyhouse. The polyhouse field was divided into total 36 plots of 4 m × 2 m wide. Six cucumber plants were planted in each plot at spacing of 100 cm between plants and 200 cm between rows. The plots were then treated with different rates of PCC and N fertilizer in a randomised complete block design (RCBD). Calcium carbide granules (2–4 mm diameter) were coated with paint following the same procedure as described by Mahmood et al. (Citation2010), and added to the soil at 0, 20 and 30 mg kg−1 soil at a depth of 6 cm three days after transplanting. Nitrogen was applied at 0, 30 and 60 mg kg−1 soil as urea, half as a basal dose and the remaining half at flowering. Phosphorus (36 mg kg−1 soil) as single super phosphate and potassium (36 mg kg−1 soil) as sulphate of potash were applied as a basal dose in all treatments. The plants were irrigated with canal water to keep the soil at or near field capacity. Uniform plant protection and weed control measures were adopted for all plots throughout the entire crop period.
Morphophysiological characters of plants
Eight weeks after sowing, the vine length, leaf area, number of primary branches, fruit yield, photosynthetic rate (PN), stomatal conductance (Gs), intercellular CO2 concentration (Ci) and nitrate reductase (NR) activity in the leaves were determined. PN, Gs and Ci were measured using an Infrared Gas Analyser (IRGA; model ADC; Bioscientific Ltd., England) between 11:00 am and 12:00 noon, when the amount of photosynthetically active radiation above the plant canopy was ≥1060 μmol (photons) m−2 s−1. The inside temperature of the leaf cuvette was set at 30 ± 2°C and light response curves were generated at ambient CO2 concentrations (300–350 μmol mol−1). Care was taken to select fully expanded leaves of uniform size and age when recording photosynthesis data from control and treated plants. Leaf area was measured with a leaf area metre (LI-3100; LI-COR Biosciences, Lincoln, NE, USA).
Nitrogen, carboxylation and photosynthetic water use efficiencies
The N content of plants was determined using the micro-Kjeldahl method (Jones et al. Citation1991). Nitrogen use efficiency was calculated as an increase in plant N at a particular level of soil N compared with the control. Carboxylation efficiency and photosynthetic water use efficiency (WUE) were calculated as the ratios of PN to Ci (Farquhar & Sharkey Citation1982) and PN to Gs (von Caemmerer & Farquhar Citation1981), respectively.
NR activity
To determine NR activity, 200 mg of shredded leaves were placed in 5 mL of incubation medium consisting of buffer containing 0.1 M phosphate (pH 7.5), 5% propanol and 0.02 M KNO3 with a few drops of chloramphenicol. The reaction mixture was kept in the dark at 25°C for 2 h, following which the reaction was stopped by adding 0.3 mL of sulphanilamide (1%) and 0.3 mL of 0.02% N-Napthyl ethylene diaminedihydrochloride (N-NEDD) to 0.4 mL of the reaction mixture. The amount of nitrite released was then determined spectrometrically by measuring the absorbance at 540 nm (T80 UV/VIS Spectrophotometer; PG Instruments Limited) and expressed as nmol NO2 g−1 fresh weight (FW) h−1 (Jaworski Citation1971).
Ethylene production
The concentration of ethylene in the developing leaves was determined 40 days after sowing following the same procedure as described by Atta-Aly (Citation1998). Briefly, the second emerging leaf from the top of each plant was sampled at 11 am and the leaf petiole was immediately dipped in 5 mL of water in a 150-mL glass tube. The tube was then sealed with a secured rubber cap and kept under light for 6 h, following which air from the tube head space was sampled with a hypodermic syringe. The ethylene concentration was then determined through gas chromatography (Kashif et al. Citation2008).
Statistical analysis
Data for all parameters were analysed using two-way analysis of variance (ANOVA) and the means were compared using the least significant difference (LSD) test at a 5% significance level in Statistix v8.1 (Steel et al. Citation1997).
Results
Growth and yield parameters
The leaf area, number of primary branches, number of fruit and fruit yield in the cucumber plants gradually increased with increasing rates of N and PCC application (). By contrast, the vine length increased with N application but decreased following the addition of PCC. Increasing rates of N and PCC application led to early flowering and fruit setting ().
Table 1. Main effects of the addition of various concentrations of nitrogen and paint-coated calcium carbide (PCC) on a range of morphophysiological characteristics in cucumber (Cucumis sativus).
The interaction effects between N and PCC on the growth and yield parameters of cucumber are presented in . It was found that PCC did not affect vine length or leaf area in the absence of N fertilizer. However, PCC at 30 mg kg−1 soil decreased the vine length by 14% at 30 and 13% at 60 mg N kg−1 soil compared with no PCC treatment at the same N levels (). By contrast, PCC application led to a 15% and 11% increase in leaf area when used in combination with 30 and 60 mg N kg−1 soil, respectively, compared with no PCC treatment at the same N levels. There was no significant difference in leaf area between plants treated with 20 and 30 mg CaC2 kg−1 soil (). At each level of N, increased application rates of PCC significantly increased the number of primary branches in cucumber. PCC applied at 30 mg kg−1 soil increased primary branches by 24%, 23% and 16% at 0, 30 and 60 mg N kg−1 soil, respectively; .
Table 2. Interactive effects of nitrogen and paint-coated calcium carbide (PCC) applications on the growth and fruit yield of cucumber (Cucumis sativus).
In the absence of PCC, an increased rate of N application did not significantly affect days to female flower initiation. However, the addition of PCC at 30 mg kg−1 soil induced flowering approximately 7 and 10 days earlier than observed in control (no PCC) plants in the absence and presence of N fertilizer, respectively (). Similarly, fruit setting was also accelerated by the addition of PCC at any of the three levels of N fertilizer. At 0 and 30 mg N kg−1 soil, PCC decreased the time from flowering to fruit setting by 4 days, whereas at 60 mg N kg−1 soil, this was only decreased by 2 days compared with control (no PCC) plants. The application of PCC in combination with N fertilizer significantly increased the number of fruit per plant and fruit yield. For both of these parameters, the application of PCC and N fertilizer had an additive effect. In the absence of N, the fruit number and yield increased by 18% and 33%, respectively, compared with the control. However, in treatments with 30 and 60 mg N kg−1 soil, the addition of PCC at 30 mg kg−1 soil increased the number of fruit by 31% and 33%, and fruit yield by 35% and 50%, respectively.
Physiological characteristics
Data regarding the impact of N and PCC (main effects) on the physiological characteristics of cucumber are presented in . It was found that an increasing rate of N application significantly improved all of the recorded physiological characteristics except N use efficiency, which remained the same at 30 and 60 mg N kg−1 soil of N fertilizer. By contrast, PCC application significantly increased N use efficiency, as well as PN, Gs, Ci, carboxylation efficiency, photosynthetic WUE, ethylene production and NR activity in the cucumber plants ().
There was a positive interaction between N and PCC applications, both improving most of the assessed physiological characters. For instance, PCC application increased PN by 46%, 56% and 61% at 0, 30 and 60 mg N kg−1 soil, respectively. A similar trend was noted for Ci, where the positive impact of PCC gradually increased from 19% to 33% at 0 and 60 mg N kg−1 soil, respectively. The carboxylation efficiency was calculated by linear regression between PN and Ci (). The application of PCC at 30 mg kg−1 soil significantly increased the carboxylation efficiency compared with the control at each level of N. However, the maximum carboxylation efficiency (22% higher than 0 mg PCC kg−1 soil) occurred when this was used in combination with 60 mg N (). The application of N and PCC also supported each other in enhancing PCC-induced stomatal conductance, which increased by 28% in plants treated with PCC alone and 40% in plants treated with PCC plus N ().
Figure 1. Linear regression between the photosynthetic rate (Pn) and intercellular CO2 concentration (Ci), which was used to calculate the carboxylation efficiency of cucumber (Cucumis sativus) plants.
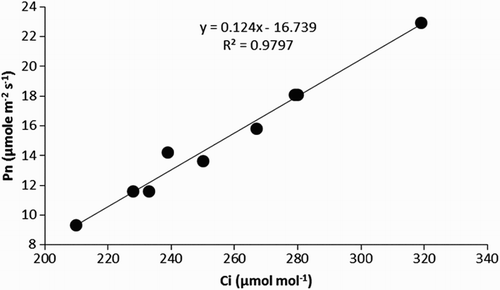
Table 3. Interactive effects of nitrogen and paint-coated calcium carbide (PCC) applications on the physiological parameters of cucumber (Cucumis sativus).
Ethylene production and NR activity
shows that each increment in N decreased ethylene production (from 0.58 to 0.33 nL g−1 h−1) and increased NR activity (from 336 to 555 nmol NO2·g FW −1·h−1), but had no significant impact on N use efficiency. By contrast, each increment in PCC had a significant impact on all of these indices.
In contrast to the findings for N application, the amount of PCC applied was positively related to the amount of ethylene produced (). The exogenous application of PCC increased ethylene production at each level of N, reaching a maximum (76% greater than the control) in plants treated with 30 mg PCC kg−1 soil in the absence of N application. By contrast, the same level of PCC (30 mg kg−1 soil) induced comparatively less ethylene production in treatments with N application, leading to 56% and 52% increases at 30 and 60 mg N kg−1 soil, respectively, compared with the application of these levels of N alone (). The application of PCC also increased the N fertilizer use efficiency of cucumber, with plants grown at a low level of N (30 mg kg−1 soil) in the presence of 30 mg PCC kg−1 soil using N more efficiently (14%) than those cultivated at an adequate level of N (60 mg kg−1 soil). PCC also enhanced the NR activity of cucumber plants, although this response varied with N fertilizer level. The application of PCC induced higher NR activity in the absence of N (18%) than at low (11%) and adequate (7%) levels of N compared with the respective no-PCC treatment at each N level ().
Table 4. Interactive effects of nitrogen and paint-coated calcium carbide (PCC) applications on ethylene production, nitrogen use efficiency (NUE), and nitrate reductase (NR) activity in cucumber (Cucumis sativus).
Discussion
Ethylene is a gaseous phytohormone that interacts with a number of signalling molecules to regulate numerous physiological and morphological plant responses under both optimal and stressful conditions (Iqbal et al. Citation2013). In the present study, the leaf ethylene concentration in cucumber was found to be downregulated following the application of N, with minimum levels being observed at the highest level of N fertilizer (60 mg N kg−1 soil). This response appears to be related to N signalling in the plant, the mechanism of which is not yet fully understood (Lea & Miflin Citation2011). One explanation may be that deficient or suboptimal levels of N impose a nutrient stress on the plant, which, in turn, causes an upregulation of ethylene production at the post-translational level (Iqbal et al. Citation2015). However, transcriptional regulation of the ethylene response to N application has also been well documented in the literature. For instance, Khan et al. (Citation2015) presented the hierarchical average of ethylene genes that were induced in response to different N perturbations, which showed that nitrate starvation and low/high N levels up- or downregulate more than 50 genes related to ethylene biosynthesis, signalling and response.
Owing to ethylene homeostasis, the application of PCC as a precursor of exogenous ethylene resulted in more ethylene being produced in cucumber leaves. This response was stronger in plants that were grown under N deficient or suboptimal conditions, indicating that N stress not only increases ethylene production but also amplifies the homeostasis. This type of response was also noted by Misyura et al. (Citation2014) in rice (Oryza sativa), where ethylene may act as a plant–plant communication signal under high density stress and homeostasis is regulated but the mineral N supply to the plants.
The application of CaC2 significantly improved the N use efficiency of cucumber plants, which can be attributed to the exploration of a greater soil volume allowing increased nutrient uptake by the plant roots, the growth of which has been reported to be stimulated by exogenous ethylene (Dolan Citation2001). In addition, this PCC-improved N use efficiency appears to be related to better N assimilation in plants owing to an enhanced NR activity and the inhibition of soil nitrification. Calcium carbide-released acetylene is a potent nitrification inhibitor that can effectively decrease N fertilizer losses through denitrification and nitrate leaching, assuring N availability and uptake over longer periods of time (Mahmood & Yaseen Citation2014). Consequently, the application of PCC reduced the optimum level of N fertilizer for cucumber, as shown by the increased N use efficiency of plants in the presence of PCC with 30 mg N kg−1 soil rather than 60 mg N kg−1 soil (). These findings indicate that in PCC-treated soil, the fertilizer requirement of cucumber lies between 30 and 60 mg N kg−1 soil, compared with the usual recommendation of 60 mg N kg−1 soil. The efficient use of N in the presence of CaC2-based soil amendments has also been reported in sweet pepper (Ahmed et al. Citation2014) and tomato (Siddiq et al. Citation2012).
The findings of this study showed that the application of PCC at each level of N improved photosynthesis in the cucumber plants. This appears to be associated with an increase in stomatal conductance and the diffusion rate of CO2, as evidenced from the higher Ci in PCC-treated plants (). Photosynthesis was also increased with an increasing rate of N. A number of studies have shown that N regulates ethylene production to remove any stress-induced inhibition of photosynthesis (Iqbal et al. Citation2015). Iqbal et al. (Citation2015) further clarified the effect of N on photosynthesis through ethylene when they demonstrated that excess N inhibited photosynthesis by causing excess ethylene to be produced in plants. In the present study, plant ethylene was also regulated following the addition of exogenous ethylene released by PCC to improve photosynthesis and other growth parameters. This indicates that every signal that can regulate the ethylene response in plants can only have a positive effect on photosynthesis at the optimum ethylene level (Iqbal et al. Citation2015).
The observed PCC-induced increase in photosynthesis may also be attributed to the increase in leaf area. Leaves with a greater surface area will be able to absorb more light and CO2 to input into the photosynthesis process. However, PCC application did not affect the leaf area in the absence of N fertilizer, indicating that N and ethylene have some complementary relationship in leaves to increase their area. These results are in line with the findings of Ren et al. (Citation2013), who concluded that N regulates ethylene in leaves to control leaf longevity; and of Tian et al. (Citation2009), who showed that the inhibition of ethylene biosynthesis with cobalt chloride decreased the leaf N concentration and longevity.
Vine length significantly increased by increasing the level of N but decreased following the application of PCC at both low (30 mg kg−1 soil) and high (60 mg kg−1 soil) levels of N (). The inhibition of apical growth by PCC appears to be caused by PCC-released ethylene, which inhibits elongation whilst promoting radial expansion and an increased number of branches per plant. This type of ethylene-related responses may be caused by an ethylene-induced dominance of cell enlargement over cell division (Smalle et al. Citation1997) and the regulation of plant polar auxin transport (Růžička et al. Citation2007). Early female flowering and fruit setting, and an increased fruit yield in PCC-treated plants were also attributed to the concentration of plant ethylene, which was regulated by N concentration and exogenous ethylene released from PCC. Therefore, it seems that PCC not only improved photosynthesis, as discussed earlier, but also regulated the allocation of photosynthates from vegetative growth to enhance early fruiting and the number of fruit. This type of ethylene-related increase in female flower count, fruit setting and accelerated fruit development has been reported previously (Kashif et al. Citation2007; Thappa et al. Citation2011).
Conclusion
The application of PCC improved photosynthesis, growth and yield parameters in cucumber under deficient and adequate N fertilizer conditions. Both the exogenous ethylene released from PCC and N fertilizer act as signalling molecules to regulate ethylene biosynthesis in the plant which, in turn, influence nearly all of the measured physiological and morphological characteristics of the plant. However, the plant responses to PCC application varied with the application rate of N fertilizer, with maximum fruit yield being observed when PCC was applied at 30 mg kg−1 soil in combination with an adequate level of N fertilizer (60 mg kg−1 soil). Thus, it appears that PCC could be used to improve the economical yield of cucumber cultivated at suboptimal or optimal levels of N fertilizer. Future studies need to investigate the impact of PCC on other vegetable crops optimal levels of N fertilizer to determine its wider applicability in vegetable production.
Disclosure statement
No potential conflict of interest was reported by the authors.
Additional information
Funding
References
- Ahmed W, Yaseen M, Arshad M, Shahid M. 2014. Effect of polyethylene coated calcium carbide on physiology, photosynthesis, growth and yield of sweet pepper. Pak J Agr Sci. 51:59–65.
- Asif M. 2012. Physico-chemical properties and toxic effect of fruit-ripening agent calcium carbide. Ann Trop Med Public Health. 5:150–156. doi: 10.4103/1755-6783.98602
- Atta-Aly MA. 1998. Soaking summer squash seeds in low concentrations of cobalt solution before sowing increased plant growth, femaleness, and fruit yield via increasing plant ethylene level. J Plant Growth Regul. 17:25–32. doi: 10.1007/PL00007008
- von Caemmerer S, Farquhar GD. 1981. Some relationships between the biochemistry of photosynthesis and the gas exchange of leaves. Planta. 153:376–387. doi: 10.1007/BF00384257
- Dolan L. 2001. The role of ethylene in root hair growth in Arabidopsis. J Plant Nutr Soil Sci. 164:141–145. doi: 10.1002/1522-2624(200104)164:2<141::AID-JPLN141>3.0.CO;2-Z
- Farquhar GD, Sharkey TD. 1982. Stomatal conductance and photosynthesis. Ann Rev Plant Physiol. 33:317–345. doi: 10.1146/annurev.pp.33.060182.001533
- Fiebig A, Dodd IC. 2015. Inhibition of tomato shoot growth by over-irrigation is linked to nitrogen deficiency and ethylene. Physiol Plant. doi: 10.1111/ppl.12343
- Iqbal N, Nazar R, Syeed S, Masood A, Khan NA. 2011. Exogenously-sourced ethylene increases stomatal conductance, photosynthesis, and growth under optimal and deficient nitrogen fertilization in mustard. J Exp Bot. 62:4955–4963. doi: 10.1093/jxb/err204
- Iqbal N, Trivellini A, Masood A, Ferrante A, Khan NA. 2013. Current understanding on ethylene signaling in plants: the influence of nutrient availability. Plant Physiol Biochem. 73:128–138. doi: 10.1016/j.plaphy.2013.09.011
- Iqbal N, Umar S, Khan NA. 2015. Nitrogen availability regulates proline and ethylene production and alleviates salinity stress in mustard (Brassica juncea). J Plant Physiol. 178:84–91. doi: 10.1016/j.jplph.2015.02.006
- Jaworski EG. 1971. Nitrate reductase assay in intact plant tissues. Biochem Biophysic Res Commun. 43:1274–1279. doi: 10.1016/S0006-291X(71)80010-4
- Jones JB Jr., Wolf B, Mills H. 1991. Plant analysis handbook. Athens (GA): Micro-Macro Publishing; p. 23–26.
- Kashif SR, Yaseen M, Arshad M, Abbas M. 2007. Evaluation of calcium carbide as a soil amendment to improve nitrogen economy of soil and yield of okra. Soil Environ. 26:69–74.
- Kashif SR, Yaseen M, Arshad M, Ayub M. 2008. Response of okra (Hibiscus esculentus L.) to soil given encapsulated calcium carbide. Pak J Bot. 40:175–181.
- Khan MIR, Trivellini A, Fatma M, Masood A, Francini A, Iqbal N, Ferrante A, Khan NA. 2015. Role of ethylene in responses of plants to nitrogen availability. Front Plant Sci. 6:927. doi: 10.3389/fpls.2015.00927
- Khan NA, Mir MR, Nazar R, Singh S. 2008. The application of ethephon (an ethylene releaser) increases growth, photosynthesis and nitrogen accumulation in mustard (Brassica juncea L.) under high nitrogen levels. Plant Biol. 10:534–538. doi: 10.1111/j.1438-8677.2008.00054.x
- Khan NA, Singh S. 2008. Abiotic stress and plant responses. New Delhi: I. K. International. ISBN: 978-81-89866-95-2.
- Lea PJ, Miflin BJ. 2011. Nitrogen assimilation and its relevance to crop improvement. In: Foyer C, Zhang H, editor. Nitrogen metabolism in plants in the post-genomic era. Ann Plant Rev. 42:1–40.
- Mahmood R, Yaseen M. 2014. Calcium carbide-based formulations cause slow release of acetylene and ethylene in soil and nitrification inhibition. Commun Soil Sci Plant Anal. 45:2277–2288. doi: 10.1080/00103624.2014.932370
- Mahmood R, Yaseen M, Arshad M, Tanvir A. 2010. Comparative effect of different calcium carbide based formulations on growth and yield of wheat. Soil Environ. 29:33–37.
- Mi G, Chen F, Zhang F. 2008. Multiple signaling pathways controls nitrogen-mediated root elongation in maize. Plant Signal Behav. 3:1030–1032. doi: 10.4161/psb.6800
- Misyura M, Guevara D, Subedi S, Hudson D, McNicholas PD, Colasanti J, Rothstein SJ. 2014. Nitrogen limitation and high density responses in rice suggest a role for ethylene under high density stress. BMC Genomics. 15:681. doi: 10.1186/1471-2164-15-681
- Mohd-Radzman NA, Djordjevic MA, Imin N. 2013. Nitrogen modulation of legume root architecture signaling pathways involves phytohormones and small regulatory molecules. Front Plant Sci. 4:385. doi: 10.3389/fpls.2013.00385
- Muromtsev GS, Letunova SV, Beresh IG, Alekseeva SA. 1990. Soil ethylene as a plant growth regulator and ways to intensify its formation in soils. Biol Bull Acad Sci USSR. 16:455–461.
- Öztürk L, Küfrevioğlu Öİ, Demir Y. 2008. In vivo and in vitro effects of ethephon on some oxidative enzymes in spinach leaves. Acta Physiol Plant. 30:105–110. doi: 10.1007/s11738-007-0096-4
- Ren H, Xu Z, Zhang W, Jiang L, Huang J, Chen S, Wang L, Han X. 2013. Linking ethylene to nitrogen-dependent leaf longevity of grass species in a temperate steppe. Ann Bot. doi: 10.1093/aob/mct223
- Růžička K, Ljung K, Vanneste S, Podhorská R, Beeckman T, Friml J, Benková E. 2007. Ethylene regulates root growth through effects on auxin biosynthesis and transport-dependent auxin distribution. The Plant Cell. 19:2197–2212. doi: 10.1105/tpc.107.052126
- Shakar M, Yaseen M, Mahmood R, Ahmad I. 2016. Calcium carbide induced ethylene modulate biochemical profile of Cucumis sativus at seed germination stage to alleviate salt stress. Sci Hort. 213:179–185. doi: 10.1016/j.scienta.2016.10.037
- Siddiq S, Yaseen M, Arshad M, Ahmed N. 2012. Effect of calcium carbide on photosynthetic characteristics, growth and yield of tomato cultivars. Pak J Agri Sci. 49:505–510.
- Smalle J, Haegman M, Kurepa J, Van Montagu M, Van Der Straeten D. 1997. Ethylene can stimulate Arabidopsis hypocotyl elongation in the light. Proc Natl Acad Sci USA. 94:2756–2761. doi: 10.1073/pnas.94.6.2756
- Steel RGD, Torrie JH, Dickey DA. 1997. Principles and procedures of statistics. A biometrical approach. New York (NY): McGraw-Hill Book Companies.
- Thappa M, Kumar S, Rafiq R. 2011. Influence of plant growth regulators on morphological, floral and yield traits of cucumber (Cucumis sativus L.). Kasetsart J Nat Sci. 188:177–188.
- Tian QY, Sun P, Zhang WH. 2009. Ethylene is involved in nitrate-dependent root growth and branching in Arabidopsis thaliana. The New Phytol. 184:918–931. doi: 10.1111/j.1469-8137.2009.03004.x
- Yang X, Wang X, Wei M, Hikosaka S, Goto E. 2009. Changes in growth and photosynthetic capacity of cucumber seedlings in response to nitrate stress. Braz J Plant Physiol. 21:309–317. doi: 10.1590/S1677-04202009000400007
- Yaseen M, Arshad M, Khalid A. 2006. Effect of acetylene and ethylene gases released from encapsulated calcium carbide on growth and yield of wheat and cotton. Pedobiologia. 50:405–411. doi: 10.1016/j.pedobi.2006.08.002