ABSTRACT
Washington navel orange, produces fruit of high nutritional value but is susceptible to chilling injury. It has previously been shown that nitric oxide (NO) can induce chilling tolerance in several types of fruit. Therefore, here, we examined the effect of using 0.25 and 0.5 mM sodium nitroprusside (SNP) as an NO donor on chilling injury in orange during storage at 3°C. We found that NO treatment reduced the incidence of chilling injury in orange fruit, with 0.5 mM SNP having the largest effect. NO treatment also reduced the lipid peroxidation levels and hydrogen peroxide contents of the peel and pulp during storage. Physiological analyses showed that NO induced the activity of several antioxidant enzymes, as well as 2,2-diphenyl-1-picrylhydrazyl (DPPH) radical scavenging activity. These findings indicate that NO successfully alleviates chilling injury in orange fruit, possibly through the elicitation of an antioxidant response, whilst maintaining the quality of the fruit by decreasing lipid peroxidation and the hydrogen peroxide content.
Introduction
Chilling injury (CI) is one of the main postharvest issues that occurs in orange (Citrus sinensis L.) and many other horticulture crops during storage (Wang Citation1993; Manners et al. Citation2003; Salcini and Massantini Citation2005; Lafuente and Zacarias Citation2006; Zhang and Tian Citation2010). Washington navel orange fruit are susceptible to CI during storage below 5°C (Syvertsen Citation1982), with the main symptoms being surface pitting, browning, discolouration and decay (Schirra et al. Citation2005; Gualanduzzi et al. Citation2009; Sevillano et al. Citation2009). Several promising methods have been developed to alleviate CI symptoms in orange fruit, including postharvest physical treatments with ultraviolet (UV-C) radiation (Odriozola-Serrano et al. Citation2007; Slaughter et al. Citation2008), modified atmosphere packaging (Manners et al. Citation2003), temperature conditioning (Porat et al. Citation2000; Bassal and El-Hamahmy Citation2011; Obenland et al. Citation2012) and chemical treatments with plant growth regulators (Lafuente et al. Citation1997; Schirra et al. Citation1998; Skog and Chu Citation2001; Schirra et al. Citation2005; Montesinos-Herrero and Palou Citation2010).
Oxidative stress from excess reactive oxygen species (ROS) has been associated with the appearance of chilling damage in fruits (Hodges et al. Citation2004). ROS react with numerous cell components causing a cascade of oxidative reactions that lead to the inactivation of enzymes, lipid peroxidation, protein degradation and DNA damage (Scondalios Citation1993). Plants have evolved a complex antioxidant system to protect them from the effects of ROS, and to fine tune the balance between ROS production and removal.
This system involves both lipid-soluble antioxidants (α-tocopherol and carotenoids), and water-soluble reductants (glutathion and ascorbate) and enzymes, such as catalas (CAT), glutathion reductase (GR), ascorbate peroxidase (APX), superoxide dismutase (SOD) and peroxidase (POD) (Apel and Hirt Citation2004). Thus, oxidative damage only occurs when this complex system fails to limit ROS accumulation. Previous studies have shown that there is a positive relationship between antioxidant enzyme activity and chilling tolerance in harvested fruits (Wang Citation1995; Sala Citation1998), suggesting that enhanced antioxidant enzyme systems and reduced peroxidation of membrane lipids may be involved in this process.
Nitric oxide (NO) is an important signalling molecule that is involved in many plant physiological processes (Leshem Citation2000; Crawford Citation2006; Wu et al. Citation2014) and also protects plant cells against oxidative stress by reducing ROS accumulation (Leshem et al. Citation1998; Xu et al. Citation2009; Zhang et al. Citation2009). Previous studies have shown that NO can counteract oxidative damage and has a protective effect under various stressful conditions (Song et al. Citation2006; Shi et al. Citation2007; Zhang et al. Citation2009; Hu et al. Citation2014); and the exogenous application of NO has been found to result in an improved chilling tolerance and reduced incidence of CI in several fruits (Pfeiffer et al. Citation1999; Singh et al. Citation2009; Zhu et al. Citation2010; Liu et al. Citation2016).
Therefore, the objective of this study was to evaluate the effects of NO on CI, lipid peroxidation levels, hydrogen peroxide (H2O2) contents, the activities of antioxidant enzymes, such as CAT, POD, SOD and APX, and DPPH radical scavenging activity in the fruit of Washington navel orange (C. sinensis L.) during storage at 3°C.
Materials and methods
Washington navel orange fruit and treatments
Washington navel orange fruit were harvested from a commercial orchard (growing conditions: temperature during fruit growth, 15–25°C; ripening period, 7 months; plant age, 23 years) in Kerman, Iran, at commercial maturity and were transported to the laboratory on the same day. Orange fruit (n = 450) were selected based on uniformity in size and the absence of physical injury or disease (i.e. wounds or rot). The harvested fruit were disinfected with 1% sodium hypochlorite (v/v) for 2 min, washed and dried in the air. They were then randomly divided into three groups. Two of these groups were immersed in aqueous solution containing 0.25 or 0.5 mM sodium nitroprusside (SNP) for 5 min SNP was used as an NO donor and concentrations were selected based on preliminary experiments; data not shown), while the third group was immersed in distilled water for 5 min and served as a control. All of the fruit were enclosed in plastic boxes containing polyethylene bags (70 cm long × 50 cm wide × 30 μm thick) to maintain a relative humidity of c. 95% and were stored at 3°C. The experiment was conducted in a completely randomised design. Fruit were randomly sampled and evaluated at 0, 30, 60, 90, 120 and 150 days after storage (3 replications with 10 fruit per replication). CI symptoms were then assessed 24 h after the fruit had been transferred to room temperature.
Evaluation of CI
CI (%) was calculated for each treatment using the following formula (Obenland et al. Citation2009):
Measurement of lipid peroxidation levels
Lipid peroxidation levels were determined and expressed as malondialdehyde (MDA) equivalents, according to the method of Rajinder et al. (Citation1981) with slight modifications. Pulp and peel tissues (4.0 g) were removed from the orange fruit, homogenised with 20 ml of 10% trichloroacetic acid and centrifuged for 10 min at 5000 g. The supernatant (1 ml) was then mixed with 3 ml of 0.5% thiobarbituric acid (TBA) that had previously been dissolved in 10% trichloroacetic acid. The reaction mixture was heat treated for 20 min at 95°C, quickly cooled and then centrifuged for 10 min at 10,000 g to clarify the precipitation. The absorbance at 532 nm was then measured and subtracted from the non-specific absorbance at 600 nm. The amount of MDA was calculated using an extinction coefficient of 155 mM−1 cm−1 and was expressed as mM g−1 fresh weight (FW).
Hydrogen peroxide assay
The H2O2 content of the fruit in each treatment was assessed using the procedure described by Prasad (Citation1996). Fresh tissues (2 g) were homogenised with 10 ml of acetone at 0°C and centrifuged for 15 min at 6000 g and 4°C. The supernatant (1 ml) was then mixed with 0.1 ml of 5% titanium sulphate and 0.2 ml of ammonia, and centrifuged for 10 min at 6000 g and 4°C. The pellets were dissolved in 3 ml of 10% (v/v) H2SO4 and centrifuged for 10 min at 5000 g. Absorbance of the supernatant phase was measured at 410 nm. The H2O2 content was calculated using H2O2 as a standard and then expressed as µg g−1 FW (Prasad Citation1996).
Antioxidant enzyme activity assay
Catalase and peroxidase
The CAT and POD activities were analysed according to Xing et al. (Citation2011) with slight modification. The reaction mixture consisted of 2 ml of sodium phosphate buffer (50 mM, pH 7.0), 0.5 ml of H2O2 (40 mM) and 0.5 ml of enzyme extract. The decomposition of H2O2 was measured by the decline in absorbance (A) at 240 nm. CAT-specific activity was expressed as U kg−1 FW, where U = Δ − A at 240 nm per second. For POD determination, 0.5 ml of enzyme extract was incubated in 2 ml of buffered substrate (100 mM sodium phosphate, pH 6.4 and 8 mM guaiacol) for 5 min at 30°C and the increased absorbance was measured at 460 nm every 30 s for 120 s after adding 1 ml of H2O2 (24 mM). POD and CAT activities were expressed as U mg−1 protein.
Superoxide dismutase
The SOD activity was assayed according to Misra and Fridovich (Citation1972). Fresh tissues (c. 200 mg) were homogenised in 5 ml of 100 mM potassium phosphate buffer (pH 7.8) containing 0.1 mM ethylenediaminetetraacetic acid (EDTA), 0.1% (v/v) Triton X-100 and 2% (w/v) polyvinyl pyrrolidone (PVP). The extract was then filtered through muslin cloth and centrifuged at 22,000 g for 10 min at 4–8°C. The supernatant was dialysed in cellophane membrane tubing against cold carbonate/bicarbonate extraction buffer for 4 h and was then used in the assay. The assay mixture was a total volume of 3 ml containing 50 mM sodium carbonate/bicarbonate buffer (pH 9.8), 0.1 mM EDTA, 0.6 mM epinephrine and enzyme, with epinephrine being the last component to be added. Adrenochrome formation over the subsequent 4 min was recorded at 475 nm in a UV–Vis spectrophotometer. One unit of SOD activity was expressed as the amount of enzyme that was required to cause 50% inhibition of epinephrine oxidation under the experimental conditions. The specific activity of SOD was expressed as U mg−1 protein.
Ascorbate peroxidase
The APX activity was assayed according to Nakano and Asada (Citation1981). The reaction mixture was a total volume of 1 ml containing 50 mM potassium phosphate buffer (pH 7.0), 0.2 mM ascorbic acid, 0.2 mM EDTA, 20 mM H2O2 and enzyme, with H2O2 being the last component to be added. The decrease in absorbance was recorded at 290 nm (extinction coefficient of 2.8 mM−1 cm−1) using a UV–Vis spectrophotometer. A correction was made for the low non enzymatic oxidation of ascorbic acid by H2O2. The specific activity of APX was expressed as U mg−1 protein.
DPPH radical scavenging activity
The DPPH radical scavenging activity was measured according to Oliveira et al. (Citation2008) with some modifications. Fresh tissue (0.5 g) from each sample was homogenised in 4 ml of absolute methanol at 4°C. Following centrifugation, 0.3 ml of the methanol extract was mixed with 2.7 ml of methanolic solution containing DPPH radicals (0.1 mMl−1), and the reaction mixture was shaken and incubated for 120 min at room temperature. The absorbance was then measured at 517 nm against a blank. The scavenging ability was calculated using the following equation:
α-tocopherol was analysed at the same time as a standard antioxidant. The final results were expressed as α-tocopherol equivalents per gram FW.
Data analysis
All statistical analyses were performed with SPSS version 13.0 (SPSS Inc., Chicago, IL, USA). The data from each time point were analysed by one-way analysis of variance (ANOVA), following which mean separations were performed with Duncan’s new multiple range test. P < .05 was considered statistically significant. Each treatment was sampled at six time points with three replicates per time point and 10 fruit per replicate.
Results
Effect of NO on CI in orange fruit
The effect of NO on CI in orange fruit during cold storage is shown in . The untreated control orange fruit exhibited CI symptoms (surface pitting and browning) 1 month after storage and had a high CI index (40.45%) at 5 months (). By contrast, orange fruit that had been treated with NO exhibited CI symptoms at 2 months after storage and had a significantly lower CI index than the control fruit. Treatment with 0.5 mM SNP was most effective in alleviating CI symptoms.
Figure 1. Effect of nitric oxide (NO) on chilling injury in Washington navel orange (C. sinensis) fruit during storage at 3°C. Means that are followed by different letters at each assessment time are significantly different at P < .05 (Duncan’s multiple range test). Vertical bars represent the standard deviation of the mean. C: control fruit; N1: 0.25 mM sodium nitroprusside (SNP); N2: 0.5 mM SNP.
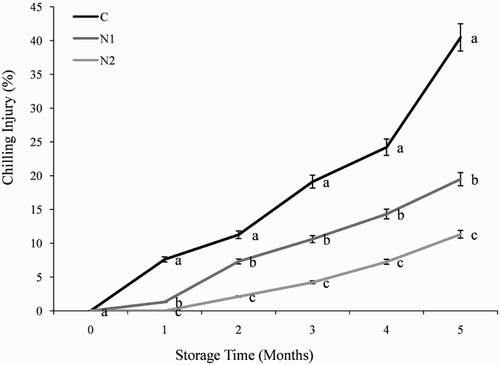
Lipid peroxidation and H2O2 contents
Lipid peroxidation levels have previously been used as a direct indicator of membrane injury, which is often associated with CI (Xu et al. Citation2009). A continuous increase in lipid peroxidation levels was observed in both the control and treated orange fruit during storage at 3°C, but the application of NO significantly delayed the increase in lipid peroxidation during storage (). At the end of the storage period (5 months), fruit that had been treated with 0.5 mM SNP had significantly lower lipid peroxidation levels than the control fruit. This pattern was observed in both the peel and pulp of the orange fruit ().
Figure 2. Effect of nitric oxide (NO) on lipid peroxidation levels in the peel and pulp of Washington navel orange (C. sinensis) fruit during storage at 3°C. Means that are followed by different letters at each assessment time are significantly different at P < .05 (Duncan’s multiple range test). Vertical bars represent the standard deviation of the mean. C: control fruit; N1: 0.25 mM sodium nitroprusside (SNP); N2: 0.5 mM SNP.
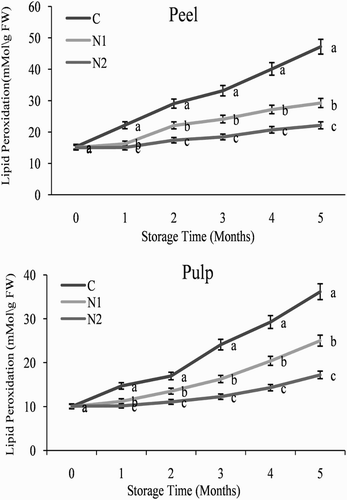
Changes in the H2O2 content of the orange fruit are presented in . The H2O2 contents of the peel and pulp of the orange fruit were initially low (10–12.11 µg g−1 FW) but generally increased with increasing storage time. At the end of the storage period (5 months), the H2O2 contents of NO-treated samples and control samples were 17.69 and 40.23 µg g−1 FW in the peel, and 16.26 and 36.27 µg g−1 FW in the pulp, respectively (), demonstrating that the control group experienced a much greater increase in H2O2 content than the NO-treated groups. Thus, since H2O2 content is as an indicator of membrane integrity (Xu et al. Citation2009), these findings demonstrate that membrane integrity was maintained as a result of NO treatment.
Figure 3. Effect of nitric oxide (NO) on the H2O2 content of the peel and pulp of Washington navel orange (C. sinensis) fruit during storage at 3°C. Means that are followed by different letters at each assessment time are significantly different at P < .05 (Duncan’s multiple range test). Vertical bars represent the standard deviation of the mean. C: control fruit; N1: 0.25 mM sodium nitroprusside (SNP); N2: 0.5 mM SNP.
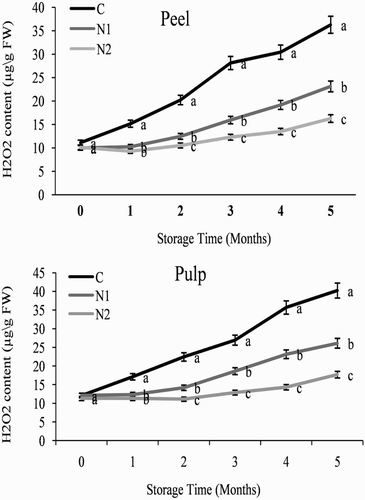
Effect of NO on antioxidant enzyme activity
As illustrated in and , treatment with 0.25 and particularly 0.5 mM SNP induced the activity of CAT and POD in orange fruit stored at 3°C. The activities of CAT, POD, SOD and PAX showed a similar pattern during storage in both the peel and pulp in all treatments () increasing at 120 days and then decreasing. Both of the NO treatments induced higher activities of CAT, POD, SOD and PAX than was observed in the control fruit (), with 0.5 mM SNP increasing the activity levels throughout the storage period.
Figure 4. Effect of nitric oxide (NO) on catalase activity in the peel and pulp of Washington navel orange (C. sinensis) fruit during storage at 3°C. Means that are followed by different letters at each assessment time are significantly different at P < .05 (Duncan’s multiple range test). Vertical bars represent the standard deviation of the mean. C: control fruit; N1: 0.25 mM sodium nitroprusside (SNP); N2: 0.5 mM SNP.
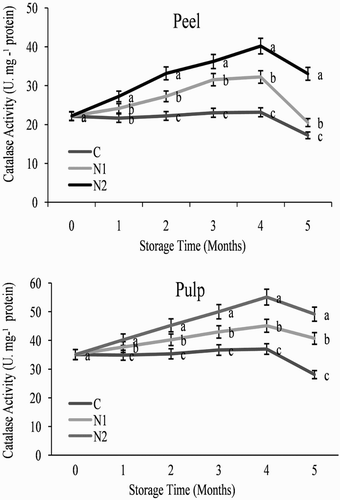
Figure 5. Effect of nitric oxide (NO) on peroxidise activity in the peel and pulp of Washington navel orange (C. sinensis) fruit during storage at 3°C. Means that are followed by different letters at each assessment time are significantly different at P < .05 (Duncan’s multiple range test). Vertical bars represent the standard deviation of the mean. C: control fruit; N1: 0.25 mM sodium nitroprusside (SNP); N2: 0.5 mM SNP.
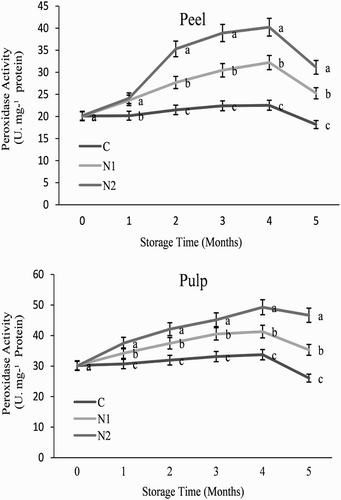
Figure 6. Effect of nitric oxide (NO) on ascorbate peroxidase activity in the peel and pulp of Washington navel orange (C. sinensis) fruit during storage at 3°C. Means that are followed by different letters at each assessment time are significantly different at P < .05(Duncan’s multiple range test). Vertical bars represent the standard deviation of the mean. C: control fruit; N1: 0.25 mM sodium nitroprusside (SNP); N2: 0.5 mM SNP.
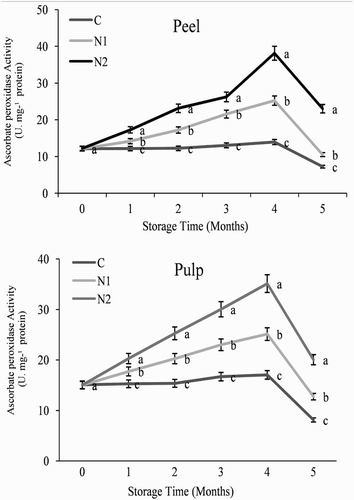
Figure 7. Effect of nitric oxide (NO) on superoxide dismutase activity in the peel and pulp of Washington navel orange (C. sinensis) fruit during storage at 3°C. Means that are followed by different letters at each assessment time are significantly different at P < .05 (Duncan’s multiple range test). Vertical bars represent the standard deviation of the mean. C: control fruit; N1: 0.25 mM sodium nitroprusside (SNP); N2: 0.5 mM SNP.
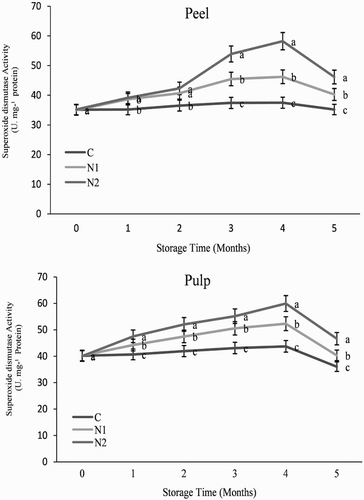
Effect of NO on DPPH radical scavenging activity
As illustrated in , treatment with 0.25 and particularly 0.5 mM SNP induced DPPH radical scavenging activity in orange fruit stored at 3°C. DPPH radical scavenging activity showed a similar pattern during storage in both the peel and pulp in all treatments (), increasing at 4 months and then remaining constant until the end of the storage period at 150 days. Both of the NO treatments induced a higher DPPH radical scavenging activity than was observed in the control fruit (), with 0.5 mM SNP increasing the activity level throughout the storage period.
Figure 8. Effect of nitric oxide (NO) on the 2,2-diphenyl-1-picrylhydrazyl (DPPH)-radical scavenging activity in the peel and pulp of Washington navel orange (C. sinensis) fruit during storage at 3°C. Means that are followed by different letters at each assessment time are significantly different at P < .05 (Duncan’s multiple range test). Vertical bars represent the standard deviation of the mean. C: control fruit; N1: 0.25 mM sodium nitroprusside (SNP); N2: 0.5 mM SNP.
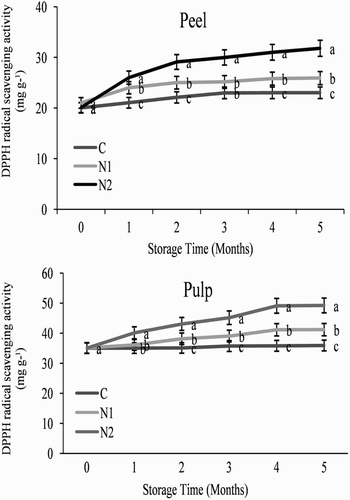
Discussion
CI is a major factor that reduces the quality and limits the storage time of subtropical fruits, including orange. When horticultural crops are exposed to severe abiotic stresses, including cold stress, large amounts of intracellular ROS are generated (Gualanduzzi et al. Citation2009; Wagstaff et al. Citation2010; Wu et al. Citation2014), the detoxification of which is dependent on antioxidant enzymes such as CAT and POD (Ding et al. Citation2007; Aghdam et al. Citation2012). However, low temperatures disrupt the metabolism of ROS, leading to their accumulation (Egley et al. Citation1983; Hung et al. Citation2007), and inactivate scavenging enzymes such as SOD, CAT, POD and APX (Kang and Saltveit Citation2002a, Citation2002b; Wang et al. Citation2015). The resulting accumulation of ROS induces lipid peroxidation, damages membrane structure and causes solute leaking (Soegiarto et al. Citation2003; Xi et al. Citation2008; Wu et al. Citation2014). A number of strategies have been evaluated to prevent CI development and extend the shelf life of fruits, including the use of physical and chemical treatments (Schirra and D’hallewin Citation1997; Obenland et al. Citation2008, Citation2009; Palou et al. Citation2010; Smilanick Citation2011). NO is considered to be involved in a network of interacting signal transduction pathways that regulate defence responses to abiotic stresses (Laspina et al. Citation2005; Shi et al. Citation2007; Zhang et al. Citation2009; Huque et al. Citation2013; Kang et al. Citation2016) and has previously been used to reduce the development of CI symptoms in a number of horticultural crops, including broccoli (Brassica oleracea var. italica), green bean (Phaseolus vulgaris), bokchoy (Brassica rapa subsp. chinensis) and cucumber (Cucumis sativus) (Soegiarto and Wills Citation2004; Liu et al. Citation2016); apple (Malus × domestica) (Huque et al. Citation2013); tomato (Solanum lycopersicum) (Lai et al. Citation2011); strawberry (Fragaria × ananassa) (Wills et al. Citation2000); peach (Prunus persica) and plum (Prunus domestica) (Singh et al. Citation2009; Zhu et al. Citation2010; Asbahi-Sis et al. Citation2012; Kang et al. Citation2016); and mango (Mangifera indica) (Vazquez-Celestino et al. Citation2016). Therefore, the aim of this study was to assess the effects of NO treatment on CI symptoms and the quality of Washington navel orange fruit during chilling stress, as well as on antioxidant systems, which may represent one mechanism for improving chilling tolerance.
We found that exogenous pre-treatment with 0.25 and 0.5 mM SNP effectively alleviated CI symptoms in orange fruit during cold storage (). The occurrence of CI is often accompanied by oxidative damage, including increased levels of lipid peroxidation (Xu et al. Citation2009; Wang et al. Citation2015). In the present study, we found that there was a continuous increase in lipid peroxidation levels in the peel and pulp in all treatments during storage, but the application of NO significantly delayed this increase (). Furthermore, the change in membrane permeability (as revealed by the H2O2 content) showed a similar trend, with the peel and pulp H2O2 contents increasing with storage duration, but NO markedly delaying this increase ().
It has previously been reported that the improvement of chilling tolerance in harvested horticultural crops is related to the enhanced activities of antioxidant enzymes. For example, Sala (Citation1998) found that antioxidant enzyme activity was higher in chilling-tolerant than chilling-sensitive mandarins (Citrus reticulata); and antioxidant enzyme activity has been shown to be enhanced following the use of a number of postharvest treatments that induce chilling tolerance and alleviate CI (Wang Citation1995; Sala and Lafuente Citation2000; Wendehenne et al. Citation2001; Zheng et al. Citation2008; Wang et al. Citation2015). Here, we found that treatment with NO significantly increased the activities of CAT, POD, SOD and APX under chilling stress (). Similarly, Yang et al. (Citation2011) and Liu et al. (Citation2016) demonstrated that exogenous NO was effective in reducing CI in cucumber by eliciting CAT, POD, APX and polyphenol oxidase activities; and Shi et al. (Citation2007) reported that NO enhanced the activity of CAT, APX and SOD in cucumber roots, and apoplastic H2O2 in NO-induced antioxidant defence. Increased SOD activity could enhance the ability of tissues to eliminate H2O2, explaining the lower level of H2O2 that was observed in NO-treated fruit (). Thus, it appears that the ability of NO to reduce the occurrence of CI is correlated with enhanced antioxidant enzyme activity, an increase which contributes to the adaptation of plants to cold stress and ameliorates oxidative damage such as lipid peroxidation and H2O2 contents (Huang and Guo Citation2005; Aghdam et al. Citation2012; Huque et al. Citation2013; Kang et al. Citation2016).
Higher levels of DPPH radical scavenging activity are correlated with increased chilling tolerance in rice (Oryza sativa) and cucumber seedlings, and cucumber fruits (Kang and Saltveit Citation2002a, Citation2002b; Yang et al. Citation2011). Similarly, we found that DPPH radical scavenging activity in orange fruit was improved following treatment with NO under low temperature stress (). Therefore, it may be considered that NO increases the chilling tolerance in orange fruit through the activation of DPPH radical scavenging activity.
Our findings are consistent with those of a previous study which showed that the exogenous application of NO is effective in protecting Arabidopsis suspension cells against cold stress (Huang et al. Citation2002). However, to the best of our knowledge, this is the first study to demonstrate that NO has beneficial effects against CI in postharvest orange fruit. Low concentrations of NO induce the expression or activation of antioxidant enzymes, as demonstrated in plants (Laspina et al. Citation2005; Shi et al. Citation2007; Zhang et al. Citation2009; Huque et al. Citation2013; Kang et al. Citation2016; Liu et al. Citation2016). Here, we suggest that the induction of antioxidant enzyme activity in orange fruit by NO may be a key factor in lowering the oxidative damage caused by cold stress, detoxifying ROS such as H2O2, O2−, and thus improving the cold tolerance and alleviating CI in orange fruit stored at 3°C.
Conclusion
In conclusion, the application of NO reduced CI in orange fruit stored at 3°C and maintained their quality. CI, lipid peroxidation levels and H2O2 contents were significantly reduced by NO treatment, particularly at a concentration of 0.5 mM SNP. This induction of cold resistance by NO treatment may result from the stimulation of antioxidant enzyme and DPPH radical scavenging activities, protection against membrane oxidative damage, decreased lipid peroxidation levels and reduced H2O2 contents in the orange fruit. These results may have implications for the use of NO in managing postharvest CI in other subtropical fruits that are stored at low temperatures.
Disclosure statement
No potential conflict of interest was reported by the authors.
References
- Aghdam MS, Asghari M, Farmani B, Mohayeji M, Moradbeygi H. 2012. Impact of postharvest brassinosteroids treatment on PAL activity in tomato fruit in response to chilling stress. Scientia Horticultureae. 144:116–120. doi: 10.1016/j.scienta.2012.07.008
- Apel K, Hirt H. 2004. Reactive oxygen species: metabolism oxidative stress, and signal transduction. Annual Review Plant Biology. 55:373–399. doi: 10.1146/annurev.arplant.55.031903.141701
- Asbahi-Sis S, Mostofi Y, Boojar MMA, Khalighi A. 2012. Effect of nitric oxide on ethylene biosynthesis and antioxidant enzymes on Iranian peach (Prunus persica cv. Anjiri). Journal of Food and Agriculture and Environment. 10:125–129.
- Bassal M, El-Hamahmy M. 2011. Hot water dip and preconditioning treatments to reduce chilling injury and maintain postharvest quality of Navel and Valencia oranges during cold quarantine. Postharvest Biology and Technology. 60:186–191. doi: 10.1016/j.postharvbio.2011.01.010
- Crawford NM. 2006. Mechanisms for nitric oxide synthesis in plants. Journal of Exponential Botany. 57:471–478. doi: 10.1093/jxb/erj050
- Ding Z, Tian S, Zheng X, Zhou Z, Xu Y. 2007. Responses of reactive oxygen metabolism and quality in mango fruit to exogenous oxalic acid or salicylic acid under chilling temperature stress. Physiology of Plant. 130:112–121. doi: 10.1111/j.1399-3054.2007.00893.x
- Egley GH, Paul RN, Vaughn KC, Duke SO. 1983. Role of peroxidase in the development of water impermeable seed coats in Sida spinosa L. Planta. 157:224–232. doi: 10.1007/BF00405186
- Gualanduzzi S, Baraldi E, Braschi I, Carnevali F, Gessa CE, De Santis A. 2009. Respiration, hydrogen peroxide levels and antioxidant enzyme activities during cold storage of zucchini squash fruit. Postharvest Biology and Technology. 52:16–23. doi: 10.1016/j.postharvbio.2008.09.010
- Hodges DM, Lester GE, Munro KD, Toivonen PM. 2004. Oxidative stress: importance for postharvest quality. HortScience. 39:924–929.
- Hu M, Yang D, Huber DJ, Jiang Y, Li M, Gao Z, Zhang Z. 2014. Reduction of postharvest anthracnose and enhancement of disease resistance in ripening mango fruit by nitric oxide treatment. Postharvest Biology and Technology. 97:115–122. doi: 10.1016/j.postharvbio.2014.06.013
- Huang M, Guo Z. 2005. Responses of antioxidative system to chilling stress in two rice cultivars differing in sensitivity. Biology of Plant. 49:81–84. doi: 10.1007/s00000-005-1084-3
- Huang X, Von Rad U, Durner J. 2002. Nitric oxide induce transcriptional activation of the nitric oxide-tolerant alternative oxidase in Arabidopsis suspension cell. Planta. 215:914–923. doi: 10.1007/s00425-002-0828-z
- Hung SH, Wang CC, Ivanov SV, Alexieve V, Yu CW. 2007. Repetition of hydrogen peroxide treatment induces a chilling tolerance comparable to cold acclimation in mung bean. Journal of American Society of Horticultural Science. 132:700–776.
- Huque R, Wills RBH, Pristijono P, Golding JB. 2013. Effect of nitric oxide (NO) and associated control treatments on the metabolism of fresh-cut apple slices in relation to development of surface browning. Postharvest Biology and Technology. 78:16–23. doi: 10.1016/j.postharvbio.2012.12.006
- Kang HM, Saltveit ME. 2002a. Antioxidant defense system and DPPH-radical scavenging activity in chilled and heat shocked rice (Oryza sativa L.) seedlings radicals. Journal of Agriculture and Food Chemistry. 50:513–518. doi: 10.1021/jf011124d
- Kang HM, Saltveit ME. 2002b. Effect of chilling on antioxidant enzymes and DPPH-radical scavenging activity of high and low vigor cucumber seedling radicals. Plant Cell and Environment. 25:1233–1238. doi: 10.1046/j.1365-3040.2002.00915.x
- Kang R, Zhang L, Jiang L, Yu M, Ma R, Yu Z. 2016. Effect of postharvest nitric oxide treatment on the proteome of peach fruit during ripening. Postharvest Biology and Technology. 112:277–289. doi: 10.1016/j.postharvbio.2015.08.017
- Lafuente MT, Martínez-Téllez MA, Zacarías L. 1997. Abscisic acid in the response of ‘fortune’ mandarins to chilling. Effect of maturity and high-temperature conditioning. Journal of Science of Food and Agriculture. 73:494–502. doi: 10.1002/(SICI)1097-0010(199704)73:4<494::AID-JSFA761>3.0.CO;2-B
- Lafuente MT, Zacarías L. 2006. Postharvest physiological disorders in citrus fruit. Stewart Postharvest Review. 2:1–9.
- Lai T, Wang Y, Li B, Qin G, Tian S. 2011. Defense responses of tomato fruit to exogenous nitric oxide during postharvest storage. Postharvest Biology and Technology. 62:127–132. doi: 10.1016/j.postharvbio.2011.05.011
- Laspina NV, Groppa MD, Tomaro ML, Benavides MP. 2005. Nitric oxide protects sunflower leaves against Cd-induced oxidative stress. Plant Science. 169:323–330. doi: 10.1016/j.plantsci.2005.02.007
- Leshem YY. 2000. Nitric oxide in plants. Occurrence, function and use. Dordrecht: Kluwer Academic; p. 23–48.
- Leshem YY, Wills RBH, Ku VVV. 1998. Evidence for the function of the free radical gas nitric oxide (NO) as an endogenous maturation and senescence regulating factor in higher plants. Plant Physiology and Biochemistry. 36:825–833. doi: 10.1016/S0981-9428(99)80020-5
- Liu Y, Yang X, Zhu S, Wang Y. 2016. Postharvest application of MeJA and NO reduced chilling injury in cucumber (Cucumis sativus) through inhibition of H2O2 accumulation. Postharvest Biology and Technology. 119:77–83. doi: 10.1016/j.postharvbio.2016.04.003
- Manners GD, Breksa AP, Schoch TK, Hidalgo MB. 2003. Analysis of bitter limonoids in Citrus juices by atmospheric pressure chemical ionization and electrospray ionization liquid chromatography-mass spectrometry. Journal of Agriculture and Food Chemistry. 51:3709–3714. doi: 10.1021/jf021124t
- Misra HP, Fridovich I. 1972. The role of superoxide anion in the auto-oxidation of epinephrine and a simple assay for superoxide dismutase. Journal of Biology and Chemistry. 247:3170–3175.
- Montesinos-Herrero C, Palou L. 2010. Combination of physical and low-toxicity chemical postharvest treatments for the management of Citrus fruit: a review. Stewart Postharvest Review. 6:1–11.
- Nakona Y, Asada K. 1981. Hydrogen peroxide scavenged by ascorbate-specific peroxidase in spinach chloroplast. Plant Cell Physiology. 22:867–880.
- Obenland D, Collin S, Sievert J, Arpaia ML. 2012. Impact of high-temperature forced-air heating of navel oranges on quality attributes, sensory parameters, and flavor volatiles. HortScience. 47:386–390.
- Obenland D, Collin S, Sievert J, Fjeld K, Doctor J, Arpaia ML. 2008. Commercial packing and storage of navel oranges alters aroma volatiles and reduces flavor quality. Postharvest Biology and Technology. 47:159–167. doi: 10.1016/j.postharvbio.2007.06.015
- Obenland D, Dennis M, Collin S, Sievert J, Fjeld K, Arpaia ML, Thompson J, Slaughter D. 2009. Peel fluorescence as a means to identify freeze-damaged navel oranges. HorTechnology. 19:379–384.
- Odriozola-Serrano I, Hernndez-Jover T, Martn-Belloso O. 2007. Comparative evaluation of UV–HPLC methods and reducing agents to determine vitamin C in fruits. Food Chemistry. 105:1151–1158. doi: 10.1016/j.foodchem.2007.02.037
- Oliveira I, Sousa A, Ferreira IC, Bento A, Estevinho L, Pereira JA. 2008. Total phenols, antioxidant potential and antimicrobial activity of walnut (Juglans regia L.) green husks. Food Chemical Toxicology. 46:2326–2331. doi: 10.1016/j.fct.2008.03.017
- Palou L, Smilanick JL, Montesinos-Herrero C, Valencia-Chamorro S, Perez-Gago MB. 2010. Novel approaches for postharvest preservation of fresh Citrus fruits. In: Slaker DA, editor. Citrus fruits: properties, consumption and nutrition. New York: Nova Science; p. 1–45.
- Pfeiffer S, Mayer B, Hemmens B. 1999. Nitric oxide: chemical puzzles posed by a biological messenger. A New Chemistry International Education. 38:1714–1731. doi: 10.1002/(SICI)1521-3773(19990614)38:12<1714::AID-ANIE1714>3.0.CO;2-3
- Porat R, Pavoncello D, Peretz J, Ben-Yehoshua S, Lurie S. 2000. Effects of various heat treatments on the induction of cold tolerance and on the postharvest qualities of ‘Star Ruby’ grapefruit. Postharvest Biology and Technology. 18:159–165. doi: 10.1016/S0925-5214(99)00075-7
- Prasad TK. 1996. Mechanisms of chilling-induced oxidative stress injury and tolerance in developing maize seedlings: changes in antioxidant system, oxidation of proteins and lipids, and protease activities. Plant Journal. 10:1017–1026. doi: 10.1046/j.1365-313X.1996.10061017.x
- Rajinder SD, Dhindsa PP, Thorpe TA. 1981. Leaf senescence: correlated with increased levels of membrane permeability and lipid peroxidation, and decreased levels of superoxide dismutase and catalase. Journal of Experimental Botany. 32:93–101. doi: 10.1093/jxb/32.1.93
- Sala JM. 1998. Involvement of oxidative stress in chilling injury in cold stored mandarin Fruits. Postharvest Biology and Technology. 13:255–261. doi: 10.1016/S0925-5214(98)00011-8
- Sala JM, Lafuente M. 2000. Catalase enzymes activity is related to tolerance of mandarin fruits to chilling. Postharvest Biology and Technology. 20:81–89. doi: 10.1016/S0925-5214(00)00115-0
- Salcini MC, Massantini R. 2005. Minimally processed fruits: an update on browning control. Stewart Postharvest Review. 1:7.
- Schirra M, Agabbio M, D'hallewin G. 1998. Chilling responses of grapefruit as affected by cultivar and harvest date. Advances Horticultural Sciences. 12:118–122.
- Schirra M, D’hallewin G. 1997. Storage performance of Fortune mandarins following hot water dips. Postharvest Biology and Technology. 10:229–238. doi: 10.1016/S0925-5214(96)01301-4
- Schirra M, Mulas M, Fadda A, Mignani I, Lurie S. 2005. Chemical and quality traits of ‘Olinda’ and ‘Campbell’ oranges after heat treatment at 44 or 46°C for fruit fly disinfestations. Lebenson Wiss Technology. 38:519–527. doi: 10.1016/j.lwt.2004.07.011
- Scondalios JG. 1993. Oxygen stress and superoxide dismutases. Plant Physiology. 101:7–12. doi: 10.1104/pp.101.1.7
- Sevillano L, Sanchez-Ballesta MT, Romojaro F, Flores FB. 2009. Physiological, hormonal and molecular mechanisms regulating chilling injury in horticultural species. Postharvest technologies applied to reduce its impact. Journal of Sciences of Food and Agriculture. 89:555–573. doi: 10.1002/jsfa.3468
- Shi QH, Ding F, Wang XF, Wei M. 2007. Exogenous nitric oxide protect cucumber roots against oxidative stress induced by salt stress. Plant Physiology and Biology and Chemistry. 45:542–550. doi: 10.1016/j.plaphy.2007.05.005
- Singh SP, Singh Z, Swinng EE. 2009. Postharvest nitric oxide fumigation delays fruit ripening and alleviates chilling injury during cold storage of Japanese plums (Prunus saliciana L.). Postharvest Biology and Technology. 53:101–108. doi: 10.1016/j.postharvbio.2009.04.007
- Skog LJ, Chu CL. 2001. Effect of ozone on qualities of fruits and vegetables in cold storage. Canadian Journal of Plant Sciences. 81:773–778. doi: 10.4141/P00-110
- Slaughter DC, Obenland DM, Thompson JF, Arpaia ML, Margosan DA. 2008. Non-destructive freeze damage detection in oranges using machine vision and ultraviolet fluorescence. Postharvest Biology and Technology. 48:341–346. doi: 10.1016/j.postharvbio.2007.09.012
- Smilanick JL. 2011. Integrated approaches to postharvest disease management in California Citrus packinghouses. Acta Horticultureae. 905:145–148. doi: 10.17660/ActaHortic.2011.905.14
- Soegiarto L, Wills RBH. 2004. Short-term fumigation with nitric oxide gas in air to extend the postharvest life of broccoli, green bean and bok choy. HortTechnology. 14:538–540.
- Soegiarto L, Wills RBH, Seberry JA, Leshem YY. 2003. Nitric oxide degradation in oxygen atmospheres and rate of uptake by horticultural produce. Postharvest Biology and Technology. 28:327–331. doi: 10.1016/S0925-5214(02)00199-0
- Song LL, Ding W, Zhao MG, Sun BT, Zhang LX. 2006. Nitric oxide protects against oxidative stress under heat stress in the calluses from two ecotypes of reed. Plant Sciences. 171:449–458. doi: 10.1016/j.plantsci.2006.05.002
- Syvertsen JP. 1982. Dehydration of freeze-damaged oranges. HortScience. 17:803–804.
- Vazquez-Celestino D, Ramos-Sotelo H, Rivera-Pastrana DM, Vazquez-Barrios E, Mercado-Silva EM. 2016. Effects of waxing, micro-perforated polyethylene bag, 1-methylcyclopropene and nitric oxide on firmness and shrivel and weight loss of ‘Manila’ mango fruit during ripening. Postharvest Biology and Technology. 111:398–405. doi: 10.1016/j.postharvbio.2015.09.030
- Wagstaff C, Bramke I, Breeze E, Thornber S, Harrison E, Thomas B, Buchanan-Wollaston V, Stead T, Rogers H. 2010. A specific group of genes respond to cold dehydration stress in cut Alstroemeria flowers whereas ambient dehydration stress accelerates developmental senescence expression patterns. Journal of Experimental Botany. 61:2905–2921. doi: 10.1093/jxb/erq113
- Wang CY. 1993. Approaches to reduce chilling injury of fruits and vegetables. Horticultural Review. 15:63–132.
- Wang CY. 1995. Effect of temperature preconditioning on catalase, peroxidase, and superoxide dismutase in chilled zucchini squash. Postharvest Biology and Technology. 5:67–76. doi: 10.1016/0925-5214(94)00020-S
- Wang Y, Luo Z, Khan ZU, Mao L, Ying T. 2015. Effect of nitric oxide on energy metabolism in postharvest banana fruit in response to chilling stress. Postharvest Biology and Technology. 108:21–27. doi: 10.1016/j.postharvbio.2015.05.007
- Wendehenne D, Pugin A, Klessig DF, Durner J. 2001. Nitric oxide: comparative synthesis and signaling in animal and plant cells. Trends Plant Sciences. 6:177–183. doi: 10.1016/S1360-1385(01)01893-3
- Wills RBH, Ku VVV, Leshem YY. 2000. Fumigation with nitric oxide to extend the postharvest life of strawberries. Postharvest Biology and Technology. 18:75–79. doi: 10.1016/S0925-5214(99)00061-7
- Wu B, Guo Q, Li Q, Ha Y, Li X, Chen W. 2014. Impact of postharvest nitric oxide treatment on antioxidant enzymes and related genes in banana fruit in response to chilling tolerance. Postharvest Biology and Technology. 92:157–163. doi: 10.1016/j.postharvbio.2014.01.017
- Xi ZX, Duan LS, Tian XL, Wang BM, Eheji AE, Li ZH. 2008. Coronatine alleviates salinity stress in cotton by improving the antioxidative defense system and radical- scavenging activity. Journal of Plant Physiology. 165:375–384. doi: 10.1016/j.jplph.2007.06.001
- Xing Y, Li X, Xu Q, Yun J, Lu Y, Tang Y. 2011. Effects of chitosan coating enriched with cinnamon oil on qualitative properties of sweet pepper (Capsicum annuum L.). Food Chemistry. 124:1443–1450. doi: 10.1016/j.foodchem.2010.07.105
- Xu W, Peng X, Luo Y, Wang J, Guo X, Huang K. 2009. Physiological and biochemical responses of grapefruit seed extract dip on ‘Redglobe’ grape. LWT –Food Sciences and Technology. 42:471–476. doi: 10.1016/j.lwt.2008.09.002
- Yang H, Wu F, Cheng J. 2011. Reduced chilling injury in cucumber by nitric oxide and the antioxidant response. Food Chemistry. 211–216.
- Zhang CF, Tian SP. 2010. Peach fruit acquired tolerance to low temperature stress by accumulation of linolenic acid and N-acylphosphatidylethanolamine in plasma membrane. Food Chemistry. 120:864–872. doi: 10.1016/j.foodchem.2009.11.029
- Zhang LG, Zhou S, Xuan Y, Sun M, Zhao L. 2009. Protective effect of nitric oxide against oxidative damage in Arabidopsis leaves under ultraviolet-B irradiation. Journal of Plant Biology. 52:135–140. doi: 10.1007/s12374-009-9013-2
- Zheng YH, Raymond WF, Wang SY, Wang CY. 2008. Transcript levels of antioxidative genes and oxygen radical scavenging enzyme activities in chilled zucchini squash in response to superutmospheric oxygen. Postharvest Biology and Technology. 47:151–158. doi: 10.1016/j.postharvbio.2007.06.016
- Zhu LQ, Zhou J, Zhu SH. 2010. Effect of a combination of nitric oxide treatment and intermittent warming on prevention of chilling injury of ‘Feicheng’ peach fruit during storage. Food Chemistry. 121:165–170. doi: 10.1016/j.foodchem.2009.12.025