ABSTRACT
Anthocyanins present in the skin of full red and blush cultivars of European pear (Pyrus communis L.) generally make this fruit more attractive to consumers than traditional green–yellow pears. Crop value depends on achieving peak redness at harvest and this can be heavily influenced by growing conditions. Anthocyanin synthesis is promoted by ultraviolet light and components of white light that up-regulate transcription factor complexes to promote increased transcript levels of regulatory genes that up-regulate key structural genes. Various orchard management practices promote sunlight exposure of fruit to aid blush development but in warm-to-hot climates anthocyanin synthesis can be supressed by high light–high temperature conditions that induce pigment fading and degradation. Unlike apples, some coloured pear cultivars attain peak colouration well before fruit are ripe and pre-harvest colour loss reduces crop quality. This paper reviews the genetics and biochemistry of anthocyanin development, considers solar radiation as the main environmental influencing factor and presents options for orchard practices that promote blush.
Introduction
The mature fruit of some European pear (Pyrus communis L.) cultivars have skins that are fully or partially red, or blushed, depending on genetics, environment and horticultural management. Red colouration is attributable to the presence of anthocyanins which are important for human health as a source of antioxidant compounds (Oancea and Oprean Citation2011). Blush (B) cultivars of P. communis that show a minimum 50% bright red skin colour are regarded in many markets as novel and command a price premium over more traditional green–yellow types (Human Citation2013) and full red (FR) fruit (Steyn et al. Citation2004a). Insufficient red colour can limit the profitability of growing bi-coloured pears (Roberts et al. Citation2008).
For many kinds of fruit, the past 10 years have seen important advances in knowledge of the molecular and biochemical basis for red colour development. In apples (Malus domestica Borkh.) colour development is comparatively well understood (Thomson and Goodwin Citation2016), while for pears and more specifically P. communis, less is known because there has been comparatively little research on this species. Nonetheless, it has been established that the regulatory molecular mechanism for red colour trait in FR and B pears is complex (Yang et al. Citation2015a). Different cultivars of red pear do not behave as a homogeneous group and the presence, and extent, of red blush varies considerably between them (Dussi Dehais Citation1993; Steyn et al. Citation2005; Yang et al. Citation2015a, Citation2015b).
Coloured pear breeding programmes around the world are actively working to understand the genetics of blush development (Dondini et al. Citation2008; Human Citation2013). For these programmes to achieve invigoration of local pear industries (McClymont et al. Citation2016) by generating improved, value-added fruit containing optimised flavonoid content, better knowledge of the regulatory mechanisms of anthocyanin biosynthesis is required (Hichri et al. Citation2011; Zoratti et al. Citation2014a).
Most FR and B cultivars in commercial Australian orchards have been planted and managed based on principles for anthocyanin development in apple crops without consideration of any genetics and physiology that could be more characteristic of P. communis. Blushed pear cultivars in existing Australian orchards may not be ideally suited to their growing conditions and this could compromise growers’ capacity to maximise production of perfectly coloured fruit. Limited knowledge means that rigorous advice on pear orchard establishment and management strategies cannot be offered to Australian growers seeking to promote and maximise red colour development in existing orchards. Nonetheless, solar radiation is known to play a key role.
In South Africa, accelerated loss of red colour during periods of high temperature prior to harvest has been reported in some cultivars (Huysamer Citation1998), and in Australia which shares a similar climate, the negative impact of elevated temperatures on ‘Cripps Pink’ apple has also lead to reduced fruit blush and lower commercial profits (Whale and Singh Citation2007). The problem is likely to be amplified by rising temperatures associated with global warming. Some FR and B pear cultivars accumulate anthocyanin with lesser dependence on climatic conditions. As these types are less susceptible to fluctuation in colour, they are likely to be easier to manage in commercial orchards where environmental factors might negatively impact colouration (Steyn et al. Citation2004b). However, research related to this issue needs to be expanded to include more cultivars and additional growing regions.
This review considers endogenous and environmental regulation of red colour development in FR and B cultivars of European pear. Colour maximising options for orchard practices and future investigations are recommended with a focus on production in warm-to-hot regions where daytime maximum summer temperatures exceed 35°C.
Genetic control of anthocyanin biosynthesis
Anthocyanins are secondary metabolites and a class of plant flavonoids synthesised through the flavonoid biosynthetic pathway. Red colour of FR and B pear fruit is due to presence of the anthocyanins cyanidin3-galactoside and cyanidin3-arabinoside in hypodermal layers of the skin (Dussi et al. Citation1995). The cyanidin3-galactoside contained in both Pomoideae genera, Malus and Pyrus, is absent from other subfamilies of Rosaceae (Dussi et al. Citation1995). In apples, cyanidin3-galactoside is the main anthocyanin (Liu et al. Citation2013).
Anthocyanin formation and hence the development of red colour during growth and ripening of fruit cultivars is regulated by an interaction between internal and external stimuli. Genetic background is the main determinant of phenolic content, including flavonoids and derived anthocyanins, but levels are modulated by external environmental factors that may cause qualitative or quantitative changes in the composition of these compounds (Zoratti et al. Citation2014a). Anthocyanin synthesis depends primarily on light intensity with temperature, carbohydrates, water stress and hormones all playing a lesser role. These environmental factors influence anthocyanin formation by affecting the synthesis of substrate for metabolic processes that are under endogenous control, or by interfering with endogenous control itself (Saure Citation1990).
The flavonoid biosynthetic pathway and production of anthocyanins is catalysed by structural genes encoding an array of key enzymes that have been characterised in several plant species (Hichri et al. Citation2011) including apple (Kim et al. Citation2003; Xu et al. Citation2012) and pear (Yang et al. Citation2015a). Enzymes in this pathway include chalcone synthase (CHS), chalcone isomerase (CHI), flavanone 3-hydroxylase (F3H), dihydroflavonol 4-reductase (DFR), anthocyanidin synthase (ANS), phenylalanine ammonia-lyase (PAL) and UDP-glucose-flavonoid 3-O-glucosyltransferase (UFGT) (Kayesh et al. Citation2013). Many of the genes encoding these enzymes share high sequence similarity across species (Stommel et al. Citation2009). Disruptions to the sequential functioning of enzymes by regulatory genes and environmental factors can impact negatively on anthocyanin production.
Expression or suppression of structural genes contributes to a variety of changes that make fruits visually attractive, and edible, based on blush development (Kayesh et al. Citation2013). Over the past decade several studies have identified genes encoding transcription factors that regulate expression of the structural genes. Key regulatory genes controlling the anthocyanin biosynthetic pathway have now been isolated in many plant species (Ban et al. Citation2007; Jaakola Citation2013; Kayesh et al. Citation2013; Wang et al. Citation2015). Expression of structural genes encoding enzymes in the flavonoid biosynthetic pathway is regulated at the transcription level by regulatory proteins belonging to the myeloblastosis (MYB), basic helix-loop-helix (bHLH) and WD40 classes (Koes et al. Citation2005; Allan et al. Citation2008; Lin-Wang et al. Citation2010; Hichri et al. Citation2011; Xu et al. Citation2012; Albert et al. Citation2014; Yang et al. Citation2015a). Details of how MYB-bHLH-WD40 transcriptional complexes operate are beginning to be elucidated (Petroni and Tonelli Citation2011; Li et al. Citation2014; Xu et al. Citation2015). Transcription factor MYB10 plays an important role in anthocyanin biosynthesis of apples (Espley et al. Citation2007) and pears (Pierantoni et al. Citation2010; Yang et al. Citation2013; Li et al. Citation2014).
Structural genes for PAL, CHS, CHI, F3H, DFR, ANS/LDOX (Leucoanthocyanidin dioxygenase), UFGT and transcription factors MYB10, bHLH and WD40 have been successfully isolated in pears (Yang et al. Citation2015a). Wang et al. (Citation2013) investigated pigmentation of red pear ‘Max Red Bartlett’ (‘Max RB’; FR), and its green-skinned sport (‘Max RB-G’). Transcript levels of structural gene PcUFGT paralleled a change in anthocyanin concentration in both ‘Max RB’ and ‘Max RB-G’ fruit.
Similarly, Pierantoni et al. (Citation2010) examined the red mutation ‘Max RB’ and its parent yellow pear P. communis ‘Bartlett’ (syn. ‘Williams’ Bon Chrétien’, WBC). Gene PcMYB10 which encodes an R2R3-MYB transcription factor involved in anthocyanin biosynthetic pathway regulation, was isolated from both ‘WBC’ and ‘Max RB’. Analysis of the deduced amino acid sequence suggested that this gene is an ortholog of anthocyanin regulators known in apple. Its expression level was significantly higher in ‘Max RB’ compared with the original yellow ‘Bartlett’. However, PcMYB10 is not directly responsible for red versus yellow colour in these two pear cultivars, as the mutation underlying this difference maps to a different region of the pear genome (Pierantoni et al. Citation2010).
Yang et al. (Citation2013) established that for P. communis ‘Early Red Doyenne du Comice’ (B) and its green skin mutant ‘Green Doyenne du Comice’ there were no sequence differences between the two colour types for seven key structural genes encoding enzymes in the flavonoid biosynthetic pathway. This means that skin colour change was not caused by their mutation. Expression levels of these structural genes showed that most were up-regulated in the red-skinned cultivar during fruit development but CHI and UFGT genes were highly expressed only at an early stage. Investigation of the expression levels of transcription factors MYB10, bHLH and WD40 showed that MYB10 was expressed at significantly higher levels in the red cultivar than in the green at the early stage, while expression of bHLH and WD40 were higher at a later stage. However, like Pierantoni et al. (Citation2010), Yang et al. (Citation2013, Citation2015a) concluded that MYB10 does not appear to be the key transcription factor regulating biosynthesis of anthocyanin and determining the red/green colour mutation. Nonetheless, this work shows that temporal expression of structural genes encoding enzymes and their transcription factors occurs within the flavonoid biosynthetic pathway, and their expression is also likely to be cultivar dependent.
Wu et al. (Citation2013) also investigated ‘Early Red Doyenne du Comice’ and ‘Green Doyenne du Comice’ for differentially expressed genes. A gene was found and named as PyMADS18. When the expression of PyMADS18 was up-regulated, anthocyanin accumulation increased; when its expression was down-regulated, anthocyanin accumulation decreased. Results indicated that PyMADS18 is mainly involved in regulation of anthocyanin synthesis in early stages of fruit development while other genes might be involved in regulation later in development. Other MADS-box genes take part in pigmentation in plants (Causier et al. Citation2002) and IbMADS10 gene is involved in anthocyanin accumulation in sweet potato (Ipomoea batatas) (Lalusin et al. Citation2006).
Li et al. (Citation2012) examined transcriptional levels of anthocyanin biosynthetic genes in P. communis ‘Wujiuxiang’. Expression of genes that encode F3H, DFR, ANS, UFGT and PcMYB10 was strongly positively correlated with anthocyanin accumulation in harvested fruit stored at low temperature (4°C). They concluded that transcription factor PcMYB10 may be responsible for both developmental and environmental control of anthocyanin biosynthesis in ‘Wujiuxiang’ pears.
Many gaps remain in our knowledge of the regulatory network of MYB, bHLH and WD40 transcription factors in fruits (Wang et al. Citation2015). It is likely that interaction between regulatory networks and the environment are important in the control of anthocyanin biosynthesis, and that further research will require an integrated approach to determine temporal and cultivar-specific effects of anthocyanin development in pear.
Solar radiation
One of the natural roles of red pigmentation in plants is protection from environmental adversity. In extreme conditions of high light, anthocyanins play an important role in mitigating photoinhibition and photodamage (Smillie and Hetherington Citation1999). This has been demonstrated in ‘D’Anjou’ where in high light and high temperature conditions, photosynthetic capacity was better maintained in ‘Red D’Anjou’ than ‘Green D’Anjou’ (Li and Cheng Citation2009). Also, different pear cultivars have been found to increase resistance to light stress with increasing skin redness (Steyn Citation2003). Given this protective function of anthocyanins, it is not surprising that blush formation on commercially grown pear fruit is heavily influenced by solar radiation. The capacity of solar radiation to both stimulate pear anthocyanin synthesis (Dussi et al. Citation1995) and promote anthocyanin degradation may occur simultaneously (Steyn et al. Citation2005). Whether radiation exposure results in a colour gain or colour loss depends on other favourable environmental and endogenous conditions for anthocyanin synthesis.
Light
Biosynthesis of anthocyanins in plant tissues either requires, or is enhanced by light, and intensity (quantity) and quality (wavelength) are key elements (Zoratti et al. Citation2014a). In general, greater exposure to light tends to increase anthocyanin content in most fruit types (Zoratti et al. Citation2014a) including pear (Dussi et al. Citation1995) (). Light’s positive influence extends beyond the sugar accumulation attributable to photosynthetically active radiation (PAR, 400–700 nm). For fruit producing plants in general, variation in the response of flavonoid biosynthesis to surrounding light conditions can be high even within a species, with synthesis influenced by light in a cultivar-specific manner (Zoratti et al. Citation2014a). Anthocyanin synthesis of the blush pear P. communis ‘Forelle’ and two fully red P. communis cultivars, ‘Bon Rouge’ and ‘Red D’Anjou’, was not stimulated by 72 h of post-harvest irradiation whereas similarly treated ‘Cripps Pink’ apples developed better blush (Marais et al. Citation2001). Differing sensitivities of pear cultivars to light-induced flavonoid biosynthesis have been reported (Qian et al. Citation2013).
Figure 1. Diagrammatic representation of solar radiation’s impact on anthocyanin accumulation in coloured pear fruit. Light’s positive influence on sugar accumulation attributable to photosynthetically active radiation (PAR, 400–700 nm) is assumed.
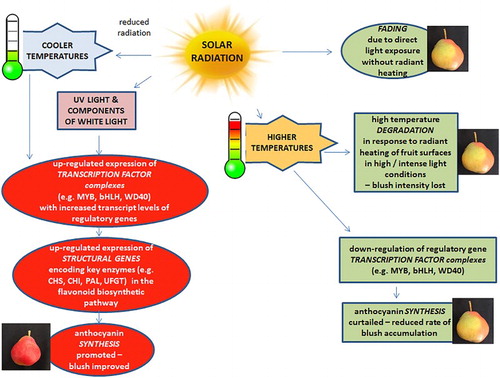
Recently, a considerable amount of new information has been gathered on environmental regulation of light-controlled anthocyanin biosynthesis in fruits (Jaakola Citation2013; Zoratti et al. Citation2014a) but many gaps remain in our understanding of relevant mechanisms. Critical wavelengths of solar radiation appear to be ultraviolet (UV, 280–400 nm) and some components of white light, however, synergism between different wavelengths may occur. Many flowers and fruit types produce flavonols, flavones and anthocyanins in response to UV radiation (Zoratti et al. Citation2014a). For apple fruit, the pathways by which light promotes colour development are increasingly understood. Various experiments with apples have demonstrated that simultaneous irradiation with white and UV light synergistically stimulates anthocyanin production (Bastías and Corelli-Grappadelli Citation2012).
Dussi et al. (Citation1995) examined the effect of light wavelength on anthocyanin levels in the skin of blush pear P. communis ‘Sensation Red Bartlett’. Gelatin filters of 1.5 cm2 with spectral-transmittance wavelengths from 400–500 nm, 500–600 nm and above 700 nm were attached over the sun-exposed sides of pears one month before harvest. Fruit from all filter treatments yielded higher anthocyanin content than control fruit exposed to natural sunlight. This finding is paralleled by observations made by Zoratti et al. (Citation2014b) on bilberries (Vaccinium myrtillus) exposed to light treatments for 48 h at approximately two weeks after pollination. They found that total anthocyanin content post harvest in ripe bilberries was increased by monochromatic lights of blue (400–500 nm), red (600–700 nm) and far-red (700–800 nm), in comparison to white light (400–800 nm) or darkness. The better performance of monochromatic light compared to white light is of interest here.
Plant MYB transcription factors are a crucial regulator of light-induced biosynthesis since they co-ordinate expression of structural genes for enzymes in the anthocyanin biosynthetic pathway. While specific information on the expression response of anthocyanin biosynthesis genes to different light wavelengths is still scarce (Zoratti et al. Citation2014a), it appears that UV plays a role (Guo et al. Citation2008). Li et al. (Citation2014) compared the shaded peel of ‘Green D’Anjou’, with its bud mutation ‘Red D’Anjou’. Red fruit had higher anthocyanin concentrations, higher transcript levels of MYB10 and higher activity of UFGT, suggesting that MYB10 regulates UFGT to control anthocyanin synthesis in ‘Red D’Anjou’ skin. In sun-exposed skin, activities of PAL, DFR, flavonol synthase, ANS and UFGT were higher in ‘Red D’Anjou’ than in Green (Li et al. Citation2014).
Light-mediated accumulation of anthocyanins is controlled by cryptochrome photosensors (Folta and Carvalho Citation2015). The UV-B photoreceptor UV-RESISTANCE LOCUS 8 has only recently been described at the molecular level (Heijde and Ulm Citation2012) and may also be involved in anthocyanin development. Tree crop research on cryptochromes in anthocyanin synthesis is limited to apples. Li et al. (Citation2013) concluded that the blue light receptor gene MdCRY2 acts as a promoter for anthocyanin accumulation since expression levels of MdCRY2 were low in apple fruit. It regulates anthocyanin expression at the translational or post-translational level, and likely interacts with the MdCOP1 gene directly or indirectly to mediate the stability of MdMYB1 regulatory proteins.
Given the dependency of other plant developmental processes such as shoot and leaf growth on the red/far-red ratio and incidence of blue light (Hogewoning et al. Citation2010), a systems approach is required to determine the optimum light environment for both tree and fruit growth in conjunction with development of FR and B skin finishes.
While beneficial light exposure is critical for promoting blush, there are two ways in which light might increase colour loss. Radiant heating can increase the temperature of fruits thereby accelerating anthocyanin degradation (Steyn et al. Citation2005), see section on Temperature, below. Direct light exposure without radiant heating is also believed to play a part in direct fading of existing, accumulated anthocyanins. Light is an important contributor to anthocyanin degradation and discolouration of food products (Cortez et al. Citation2017). Anthocyanin extracts from Cocculus hirsutus were slowly degraded in dark and highly degraded under light (Rakkimuthu et al. Citation2016). Once synthesised, anthocyanin molecules are transported to the vacuolar lumen where they are stabilised and stored. While, the mechanism that brings about fading remains unclear, it is believed to link to instability during the cellular storage phase (Hu et al. Citation2016; Passeri et al. Citation2016). How light might contribute also remains unresolved. Light can directly increase anthocyanin loss in detached apple and pear peel (Marais et al. Citation2001; Steyn et al. Citation2004b).
Temperature
Temperature of the fruit surface can strongly influence the process of anthocyanin accumulation. For plants in general, lower temperatures tend to promote skin blush while higher temperatures do not (). Historical research, contemporary industry reports and anecdotal information all attest to a negative role of high temperature in blush development of apple fruit (Thomson and Goodwin Citation2016). In general, red colour declines in apple cultivars when the temperature exceeds approximately 25°C (Curry Citation1997; Gouws Citation2010).
Fruit are at greatest risk of over-heating through radiant heating caused by direct exposure to high levels of solar radiation during mid to late afternoon. High temperature causes a decrease, and low temperature, an increase, in gene transcript levels in the anthocyanin biosynthesis pathway (Ubi Citation2004; Ubi et al. Citation2006). For apples, Palmer et al. (Citation2012) and Wang et al. (Citation2011) concluded that high temperature-induced down-regulation of biosynthesis is primarily due to down-regulation of the anthocyanin regulatory complex with reduced transcript levels of regulatory genes. Heating fruit rapidly reduces expression of the MYB transcription factors (MYB10/MYB1) responsible for elevating colour. In contrast, a single night of low temperatures can be sufficient to elicit a large increase in transcription of MdMYB10 and thereby promote anthocyanin biosynthesis (Wang et al. Citation2011).
As well as directly inhibiting anthocyanin synthesis, elevated temperatures can also potentially reduce stability of existing anthocyanins (Mori et al. Citation2007). In addition to light and temperature, this stability is influenced by tissue pH, presence of enzymes, oxygen and co-pigmentation with other natural co-existing components and metal ions in vacuoles (Luo et al. Citation2017). Consequently, a decrease in overall anthocyanin accumulation and associated blush at elevated temperatures could be attributable to a decrease in synthesis and increase in degradation (Shaked-Sachray et al. Citation2002). To date, few reports for fruit on trees have directly demonstrated accelerated anthocyanin degradation in the field due to high temperature (Goulas et al. Citation2012).
Changes in the vacuoles that store anthocyanins can decrease stability of the pigments and cause either chemical degradation or increased vulnerability to degrading enzymes (e.g. b-glucosidases, peroxidases) present in the vacuoles (Oren-Shamir Citation2009). For example, catabolism of anthocyanins in Cabernet Sauvignon grape (Vitis vinifera) skin exposed to high temperatures may be due to chemical degradation but could also be a product of peroxidase enzyme activity induced due to thermal stress (Mori et al. Citation2007).
In research on detached fruit, degradation of anthocyanins occurred at temperatures above 30°C in apple (Marais et al. Citation2001; Kevany et al. Citation2003) and anthocyanin degradation and colour loss were found to increase linearly between 10°C and 30°C in harvested apples and pears (Steyn et al. Citation2004b). Pre-harvest, field degradation of anthocyanins in FR and B pear skin is likely to increase as temperature rises but the effect may be cultivar specific and more research is required to confirm this and identify potential threshold temperatures. In the Goulburn Valley (36.44°S 145.27°E Victoria, Australia), the minimum air temperature at times when pear fruit surface temperature exceeded 45°C was 28.5°C (McClymont et al. Citation2016). However, given that high fruit temperatures are attributable to direct exposure to high levels of solar radiation, separating effects attributable to either light or temperature under natural conditions of exposure in the field requires well designed trials that still remain to be conducted for FR and B pears.
Compared to apples, low temperatures may play a lesser role in anthocyanin development of FR and B pears. Most apple cultivars either require or benefit from low temperatures but not all pear cultivars are as responsive. Steyn et al. (Citation2004a) found that of four pear cultivars studied, only ‘Rosemarie’ demonstrated an increase in red colour in response to low temperature. Compared to ‘Rosemarie’, colour of the red cultivar ‘Bon Rouge’ and blush pears ‘Flamingo’ and ‘Forelle’ was more stable and less responsive to temperature (Steyn et al. Citation2004a). However, it is not clear whether this stability is caused by less degradation or as suggested by Steyn et al. (Citation2004b), a continuation of anthocyanin synthesis regardless of high temperature. In support, analysis of enzyme activity indicated that anthocyanin synthesis in ‘Bon Rouge’ did not require low temperatures (Steyn et al. Citation2004a).
Alternating cycles of high and low temperatures can give rise to fluctuations in ‘Rosemarie’ colour during fruit development (Steyn et al. Citation2004b). For this blush cultivar, anthocyanin synthesis requires low temperatures and fruit gain red colour during conducive weather events such as the passing of cold fronts (Steyn et al. Citation2004a). Effect of cold temperature on enzyme activity in ‘Rosemarie’ was much greater during early fruit development than in the week before harvest. In response to a cold front that occurred earlier in development, PAL and UFGT activity in ‘Rosemarie’ skin increased by 130% and 200%, respectively, and hue angle of the skin’s colour decreased by 19° thereby denoting an improvement in redness (Steyn et al. Citation2004a). However, a second cold front closer to harvest brought about little response in enzyme activity. ‘Rosemarie’ pears rapidly lost red colour during intermittent warmer periods in South African orchards due to reduced anthocyanin synthesis and increased anthocyanin degradation during pre-harvest fruit development (Steyn et al. Citation2004b). Hue angle of ‘Rosemarie’ fruit skin was found to increase (i.e. less redness) by up to 5° during a single hot day (Steyn et al. Citation2005). Additional South African work by Wand et al. (Citation2005) also ascribed poor ‘Rosemarie’ colour to pre-harvest anthocyanin losses during periods of high temperature. Of all the FR and B pear cultivars grown in South Africa ten years ago, ‘Rosemarie’ had the lowest capacity for anthocyanin accumulation (Steyn et al. Citation2004a, Citation2004b).
From a few weeks before harvest, cool night temperatures of less than 18°C can enhance apple colour, especially on areas of the skin that have been poorly exposed to light (Reay Citation1999). Cold temperature thresholds affecting anthocyanin synthesis in FR and B pear cultivars have not been developed and their effect on skin colour is not well understood.
Developmental patterns of anthocyanin accumulation
Ability to accumulate anthocyanins is in part a function of a fruit’s developmental stage and for many fruit types, changes in skin colour and accumulation of anthocyanins coincide with ripening and physiological maturity (Zoratti et al. Citation2014a). In most flowering plants, maximum pigmentation and colour occur in ripe fruit with highest levels of colour attained during a ripening-associated peak (Saure Citation1990). It remains to be fully established whether some cultivars of coloured pear may deviate from this pattern (). Steyn et al. (Citation2004a) found that some cultivars (e.g. ‘Bon Rouge’ [FR], ‘Flamingo’ [B], ‘Rosemarie’ [B]) display an atypical anthocyanin accumulation pattern with maximum skin anthocyanin concentrations occurring approximately midway between anthesis and harvest, and a slow decrease in anthocyanin concentration and colour thereafter. However, it is unclear whether a decrease in synthesis of anthocyanins is involved. FR and B fruit colour at harvest is highly influenced by the maximum anthocyanin concentration reached and severity of colour loss (Steyn et al. Citation2004b). Steyn et al. (Citation2004a) proposed that loss of red colour towards harvest could relate to diminishing activity of PAL in ‘Rosemarie’ and ‘Bon Rouge’.
Table 1. Characteristics of blush development for selected full red and blush cultivars of European pear.
Steyn et al. (Citation2004b, Citation2005) suggest that an exponential relationship exists between anthocyanin concentration and hue angle of the skin’s colour at high pigment levels, while the relationship is linear at lower pigment levels. Consequently, much larger reductions in anthocyanin concentration are required to induce a similar extent of visible colour change in well-coloured fruit with high anthocyanin concentrations compared to poorly coloured fruit containing little anthocyanin (Steyn et al. Citation2005). This means that despite reductions in anthocyanin concentration attributable to fading and degradation, fruit containing a high anthocyanin concentration change little in redness but fruit with little content rapidly lose redness and become yellow/green under conditions favouring fading/degradation (Steyn et al. Citation2004b). The high pigment concentrations in a red cultivar, as compared to a blush type, may mean that even considerable anthocyanin synthesis or fading/degradation might have little effect on red colour (Steyn et al. Citation2004a). Consequently, fruit of blushed cultivars with a relatively low capacity for anthocyanin accumulation are much more prone to significant red colour loss prior to harvest. These cultivars experience greater fluctuation in redness between seasons due to the poor buffering of red colour by lower anthocyanin content (Steyn et al. Citation2004b).
Aside from high temperature and associated high light exposure, a third mechanism may reduce levels of accumulated anthocyanin molecules. Transient anthocyanin disappearances during plant development, or changes in environmental conditions, suggest that anthocyanin molecules may have limited stability and that turnover occurs with catabolism or degradation controlled and induced when beneficial to the plant (Oren-Shamir Citation2009). Several enzymes that degrade anthocyanins in harvested fruit may be candidates for in vivo degradation (Oren-Shamir Citation2009).
Orchard management practices for blush enhancement
Several orchard management practices are known to influence colouration in fruit (Senthilkumar and Vijayakumar Citation2014). With progressive increases in our understanding of factors that optimise anthocyanin synthesis, more effective orchard management practices can be developed and adopted on-farm to help improve FR and B pear colour. In Australia and other growing areas with warm-to-hot climates, mitigating the negative impacts of higher temperatures in increasingly variable climates is likely to consume more orchard management time and costs in the future.
Canopy growth
A range of canopy management options increase fruit blush of red apple cultivars like ‘Cripps Pink’ (Thomson and Goodwin Citation2016) and most should be relevant to crops of FR and B pears. Direct manipulation of shoot systems by training and correct pruning can reduce vegetative growth, open up the canopy and increase colouration by allowing better penetration of light through to fruit, especially in the lower canopy (Willaume et al. Citation2004). High density plantings combined with dwarfing rootstocks tend to deploy tree training systems that create good light penetration into canopies as long as shoot growth during spring does not shade the fruit. Fruit shading is more likely to cause poor colour in standard, pyramid or vase trained trees but these traditional shapes are unlikely to be favoured in modern pear orchards.
Techniques for shoot growth reduction to curtail shading in pears are less widely practised than for apples but include foliage thinning, use of regulated deficit irrigation (RDI), chemical growth regulators, changed fertiliser inputs, root pruning and trunk girdling. RDI supplies less water than plants require for maximum transpiration and growth, thereby inducing a low level of water stress. RDI reduces vegetative growth and lowers leaf densities to allow more light penetration through the canopy. For apples, anecdotal observations suggest that root pruning 50–60 cm out from the trunk between late dormancy and full bloom, or four to six weeks after fruit set, can produce good results where excess vigour and low colour are problems (Wilton and Hornblow Citation2003). Most probably, effects of root pruning are related to reduced vegetative vigour allowing greater fruit exposure to solar radiation. While these techniques can be beneficial for ‘Cripps Pink’ and other apple cultivars, new research needs to examine their effects on FR and B pears. In Australia, research must focus on the timing of foliage thinning to maximise colour development and minimise degradation from excessive exposure.
Advice for Australian orchardists suggests that ‘Forelle’ fruit which are not exposed to the sun do not develop the distinctive deep-red blush colour required in a premium product (van den Ende Citation2011). In the orchard, dappled sunlight must reach the ground at all times as an indicator of good light penetration through the canopy. Good light penetration allows fruitlets to continue synthesising and accumulating anthocyanin, so that they remain red throughout development and obtain attractive blush at harvest (Steyn et al. Citation2005; van den Ende Citation2011).
In warm-to-hot production areas encouraging exposure has the counter-effect of potentially exposing fruit surfaces to excess radiation, thereby accelerating blush loss due to light-induced fading and/or radiant temperature heating. Current research on tree density and training to increase precocity of pears recommends a shift to planting systems in which there is greater exposure of fruit to direct solar radiation (McClymont et al. Citation2016). Another consequence of greater light exposure can be increased risk of fruit sunburn but this can be managed with appropriate strategies. Most commercial sunscreen and sun-blocker products for spray application to foliage and fruit are formulated with the intention of allowing normal fruit development and colouration (Thomson et al. Citation2015). Threshold fruit surface temperature for the occurrence of sunburn damage on blush pear ‘ANP-0131’ (Deliza®) has been estimated at 47.1°C (McClymont et al. Citation2016).
Rootstocks
Roberts et al. (Citation2008) conducted a trial to establish whether red colour could be improved by choice of rootstock. ‘Forelle’ pears were planted on three clonal pear (P. communis) rootstocks (BP1, BP3 and OHF97) and three clonal quince (Cydonia oblonga) rootstocks (QA, QC51, BA29). Fruit from quince rootstocks showed better red colour than fruit from pear rootstocks. Skin chlorophyll and carotenoid concentrations were significantly lower in ‘Forelle’ fruit grown on quince. Differences in anthocyanin concentrations between the treatments were non-significant which suggests that fruit from quince rootstocks probably appeared redder due to intrinsically lower chlorophyll concentrations in the peel. Roberts et al. (Citation2008) concluded that quince rootstocks may have a direct effect on red colour that is not related to differences in light interception or maturity.
Whilst quince is the preferred pear rootstock in Europe, Pyrus species rootstocks are still heavily used elsewhere, including Australia. Current unpublished research in Australia is investigating rootstock effects on yield, vegetative growth and colour in blush pear cultivars ‘ANP-0118’ (Lanya®) and ‘ANP-0131’ (Deliza®). Rootstocks and interstem combinations include: C. oblonga ‘Quince A’ and ‘Quince C’ rootstocks with P. communis ‘Beurre Hardy’ interstem; P. communis ‘PB1’ rootstocks; and Pyrus calleryana ‘D6’ rootstocks with and without Pyrus pyrifolia ‘Nijisseiki’ interstem. Results so far have shown that ‘Quince A’ rootstocks had higher yield at the first harvest, three years after planting (). No impacts on colour or other fruit quality characteristics (soluble solids concentration, firmness, shape and sunburn) have been observed to date. Both scion cultivars on ‘Quince A’ rootstock and the cultivar ‘ANP-0131’ on ‘Quince C’ rootstock tended to have less vegetative growth (). Dwarfing rootstocks can play an important role in restricting pear canopy growth to help maximise light exposure.
Table 2. Comparison of fruit yield, red colour, and annual pruning weights for blush cultivars ‘ANP-0118’ (Lanya®) and ‘ANP-0131’ (Deliza®) grafted to five rootstock/interstem combinations.
Crop load
Red colour intensity in apple skin is partly a function of the energy requirement for pigment synthesis (Lister Citation1994; Veens Citation2009). Anecdotally, it appears that excessive crop load, with apple clusters of two to three fruits, can reduce fruit colouration through both competition for assimilates needed for increased blush, and by direct shading of neighbouring fruit (Gurnsey and Lawes Citation2014). A high fruit-to-leaf ratio may mean that leaves cannot produce enough carbohydrates to colour or size all fruit to their full potential. While this knowledge might be extrapolated to FR and B pears, it requires verification.
Interestingly, growers that have planted RB cultivars in high density orchards in Australia are hand thinning early in the season mainly to remove misshapen and poorly coloured fruit. This has dramatically improved packout.
Nitrogen
High levels of nitrogen (N) fertiliser have generally been found to inhibit colouration of red apple varieties but effect of N on pear blush is largely uninvestigated. The most obvious way that N can reduce red colouration is by increasing density of foliage, leading to shading and less light penetration into canopies (Gurnsey and Lawes Citation2014). However, decrease in red colouration with increasing N application has also be linked to direct inhibition of anthocyanin synthesis and delayed chlorophyll degradation (Wang and Cheng Citation2011). Nitrogen may inhibit flavonoid synthesis by enhancing the channelling of PAL toward protein synthesis, or alternatively, may negatively influence the enzyme system involved in the biosynthesis of phenolics (Senthilkumar and Vijayakumar Citation2014). In work on young apple leaves, Strissel et al. (Citation2005) observed that PAL activity seemed to be down-regulated by high N levels.
Evaporative cooling
In hot conditions, over-tree irrigation used for evaporative cooling can help lower fruit temperatures and reduce anthocyanin degradation. This is important for those blush cultivars where anthocyanin synthesis declines in the weeks immediately prior to harvest when temperatures have increased. For example, it is established that anthocyanin synthesis in ‘Rosemarie’ responds well to lower temperatures but is inhibited under hotter conditions (Steyn et al. Citation2004b). Evaporative cooling starting two weeks before harvest usually improved ‘Rosemarie’ fruit blush, however, its effect was relatively small compared to colour change in response to fluctuating temperature in warm production areas (Wand et al. Citation2005). Steyn et al. (Citation2004b) found that evaporative cooling reduced blush loss of ‘Rosemarie’ pear. Evaporative cooling had no significant effects on ‘Forelle’ fruit colour or weight but reduced firmness and soluble solids concentration when started early in the season (Wand et al. Citation2005). As discussed previously, ‘Forelle’ and ‘Flamingo’ tend to retain colour stability under hot conditions (Steyn et al. Citation2004b), and thus their cooling is likely to be less necessary.
Dussi et al. (Citation1997b) applied cooling with over-tree sprinkler irrigation to ‘Sensation RB’ pear trees during the final 30 days of fruit development when orchard air temperatures were greater than 29°C. Fruit from cooled trees had more red and less yellow than fruit from non-cooled trees, resulting in lower hue values by the middle of the harvestable maturity period. However, fruit firmness between 100 and 150 days after full bloom decreased more rapidly in fruit from cooled trees. To compensate, Dussi et al. (Citation1997b) recommended that cooled fruit should be harvested earlier than non-cooled fruit to maintain post-harvest quality. Differences between cooled and non-cooled fruit with respect to hue, surface blush and rate of firmness loss were also more pronounced in a warm season requiring frequent cooling (Dussi et al. Citation1997b).
For vulnerable cultivars, timing of cooling may be critical with applications of greatest benefit likely to be those closer to harvest, or when blush has reached appropriate commercial levels. Newer management options to deliver water to crops more efficiently can significantly improve the benefits of evaporative sprinkling (Evans and Van der Gulik Citation2011).
Over-tree netting
Shade created by netting used for hail and pest protection helps to reduce fruit temperatures during hot weather and this provides protection from some types of sunburn (Racsko and Schrader Citation2012) and can offer protection against degradation of pear anthocyanin content (Dussi et al. Citation1995). However, the impact may be cultivar specific. ‘Rosemarie’ could benefit from net shading because its red colour development slows in response to higher temperatures whereas potential netting benefits may be less for ‘Forelle’ and ‘Flamingo’ which may have some capacity to maintain anthocyanin synthesis in hot conditions prior to harvest (Steyn et al. Citation2004b).
Netting lowers light levels and in apple crops this can impair colour development by blocking both photosynthetically active radiation (PAR, 400–700 nm) that aids sugar development, and additional wavelengths (e.g. UV-B) believed to promote anthocyanin biosynthesis (Dussi et al. Citation2005; Solomakhin and Blanke Citation2007, Citation2010; Blanke Citation2009). The extent to which under-net light levels are reduced is predominantly determined by weave-density, a measure of the ratio of fabric strands to open gaps between strands. However, unlike weave-density that simply restricts the intensity of unfiltered radiation, reflectance and transmittance optical properties of the strand material itself create filtered, modified light quality (wavelength). Solomakhin and Blanke (Citation2010) suggested that different net colours could have practical implications in fruit colouration but the physiological mechanisms involved in plant responses to wavelength quality under coloured nets are still not totally understood (Bastías and Corelli-Grappadelli Citation2012). Coloured nets could be used to increase the proportion of short wavelength light reaching fruit to promote anthocyanin biosynthesis (Bastías et al. Citation2012). However, most research to date on the effects of net colour has been based on apples and focussed on PAR (Bastías and Corelli-Grappadelli Citation2012); limited work describes effects of net colour on UV transmission. In a typical netted apple orchard in the Goulburn Valley, total summer, mid-afternoon solar radiation was reduced by 15% and UV-B radiation by 32% under grey netting (McCaskill et al. Citation2016).
There is a limited knowledge base for making recommendations to pear orchardists about netting colour and its relationship to anthocyanin accumulation. More information on the response of fruit to specific wavelengths of light needs to be generated before field solutions can be developed with confidence. More precise orchard management can be achieved with retractable over-tree nets that restrict use of the covering to times of critical impact.
Reflective mulch
When over-tree nets are deployed, loss of light can be compensated for by using reflective cloths and films, sometimes called ‘mulches’, placed on the ground surface between tree rows. These reflect light back into the tree canopy and thereby improve colour (Privé et al. Citation2008, Citation2011). Different reports have shown that reflection of PAR and UV from grassed inter-rows is no more than 10% of light incoming to the ground, whereas up to 40% and 80% of PAR and UV respectively can by reflected by ground films (Bastías and Corelli-Grappadelli Citation2012). In apple orchards, these reflective films are increasingly used to alter fruit light interception for promotion of red colouration prior to harvest (Blanke Citation2015). Although work on pears remains at an experimental stage, deploying reflective mulch at critical periods increased flower bud formation of green cultivar ‘Clara Frijs’ (Bertelsen Citation2005) and yield of ‘Green D’Anjou’ (Einhorn et al. Citation2012).
Conclusion
To ensure that FR and B pear cultivars develop maximum fruit colour, there is a need to conduct future research to better separate direct light effects on promotion of anthocyanin synthesis from the negative influences of temperature, or radiant heating. The most critical wavelengths of light for anthocyanin synthesis also require further elucidation. Improved understanding of these fundamental mechanisms could make strategies for tree training, row orientation and timing of shoot thinning more efficient. Deployment of over-tree netting and evaporative cooling could also be improved by separating light and temperature effects on pigment accumulation; cooling lowers fruit surface temperatures but not irradiation levels, while netting also lowers fruit surface temperature but can change light quality reaching fruit. With rises in global temperatures, orchards need additional practical approaches to mitigate negative impacts of increased temperature. High and low temperature thresholds for anthocyanin synthesis should be established for widely planted cultivars, especially in warm-to-hot growing regions. Prediction of fruit surface temperature from prevailing weather conditions can be further developed and used to maximise beneficial light exposure but protect fruit from sunburn. For pears, little research has examined anthocyanin degradation caused by high fruit surface temperature, however, genes that increase stability of pigment molecules have been identified and may facilitate genetic manipulation to enable decreased degradation under broader environmental conditions (Luo et al. Citation2007; Oren-Shamir Citation2009).
Disclosure statement
No potential conflict of interest was reported by the authors.
References
- Albert N, Davies K, Lewis D, Zhang H, Montefiori M, Brendolise C, Boase M, Ngo H, Jameson P, Schwinn K. 2014. A conserved network of transcriptional activators and repressors regulates anthocyanin pigmentation in Eudicots. The Plant Cell. 26:962–980. doi: 10.1105/tpc.113.122069
- Allan AC, Hellens RP, Laing WA. 2008. MYB transcription factors that colour our fruit. Trends in Plant Science. 13(3):99–102. doi: 10.1016/j.tplants.2007.11.012
- Ban Y, Honda C, Hatsuyama Y, Igarashi M, Bessho H, Moriguchi T. 2007. Isolation and functional analysis of a MYB transcription factor gene that is a key regulator for the development of red coloration in apple skin. Plant Cell Physiol. 48:958–970. doi: 10.1093/pcp/pcm066
- Bastías RM, Corelli-Grappadelli L. 2012. Light quality management in fruit orchards: physiological and technological aspects. Chilean Journal of Agricultural Research. 72(4):574–581. doi: 10.4067/S0718-58392012000400018
- Bastías RM, Ruíz K, Manfrini L, Pierpaoli E, Zibordi M, Morandi B, Losciale P, Torrigiani P, Corelli-Grappadelli L. 2012. Effects of photoselective nets on phenolic composition in apple fruits. Acta Horticulturae. 939:77–83. doi: 10.17660/ActaHortic.2012.939.9
- Bertelsen M. 2005. Reflective mulch improves fruit size and flower bud formation of pear cv. ‘Clara Frijs’. Acta Horticulturae. 671:87–95. doi: 10.17660/ActaHortic.2005.671.10
- Blanke MM. 2009. The structure of coloured hail nets affects light transmission, light spectrum, phytochrome and apple fruit colouration. Acta Horticulturae. 817:177–184. doi: 10.17660/ActaHortic.2009.817.17
- Blanke M. 2015. Improving red colouration of apple fruit. Erwerbs-Obstbau. 57(2):47–62. doi: 10.1007/s10341-015-0234-x
- Causier B, Kieffer M, Davies B. 2002. MADS-box genes reach maturity. Science. 296:275–276. doi: 10.1126/science.1071401
- Cortez R, Luna-Vital DA, Margulis D, Gonzalez de Mejia E. 2017. Natural pigments: stabilization methods of anthocyanins for food applications. Comprehensive Reviews in Food Science and Food Safety. 16:180–198. doi: 10.1111/1541-4337.12244
- Curry EA. 1997. Temperatures for optimum anthocyanin accumulation in apple tissue. Journal of Horticultural Science. 72(5):723–729. doi: 10.1080/14620316.1997.11515564
- Dondini L, Pierantoni L, Ancarani V, D’Angelo M, Cho K-H, Shin I-S, Musacchi S, Kang S-J, Sansavini S. 2008. The inheritance of the red colour character in European pear (Pyrus communis) and its map position in the mutated cultivar ‘Max Red Bartlett’. Plant Breeding. 127:524–526. doi: 10.1111/j.1439-0523.2008.01500.x
- Dussi Dehais MC. 1993. Fruit color development in red pears [MSc thesis]. Oregon State University. 162 p.
- Dussi MC, Sugar D, Azarenko AN, Righetti TL. 1997a. Colometric characterization of red pear cultivars. Fruit Varieties Journal. 51(1):39–43.
- Dussi MC, Sugar D, Azarenko AN, Righetti TL. 1997b. Effects of cooling by over-tree sprinkler irrigation on fruit color and firmness in ‘Sensation Red Bartlett’ pear. HortTechnology. 7(1):55–57.
- Dussi MC, Giardina G, Reeb P. 2005. Shade nets effect on canopy light distribution and quality of fruit and spur leaf on apple cv. Fuji. Spanish Journal of Agricultural Research. 3(2):253–260. doi: 10.5424/sjar/2005032-144
- Dussi MC, Sugar D, Wrolstad RE. 1995. Characterizing and quantifying anthocyanins in red pears and the effect of light quality on fruit color. Journal of the American Society for Horticultural Science. 120(5):785–789.
- Einhorn T, Turner J, Laraway D. 2012. Effect of reflective fabric on yield of mature ‘d’Anjou’ pear trees. HortScience. 47(11):1580–1585.
- van den Ende B. 2011. Grow perfect Forelle pears on Tatura. Good fruit Grower Sept 1, 2011. http://www.goodfruit.com/grow-perfect-forelle-pears-on-tatura/.
- Espley RV, Hellens RP, Putterill J, Stevenson DE, Kutty-Amma S, Allan AC. 2007. Red colouration in apple fruit is due to the activity of the MYB transcription factor, MdMYB10. Plant Journal. 49:414–427. doi: 10.1111/j.1365-313X.2006.02964.x
- Evans RG, Van der Gulik TW. 2011. Irrigation for microclimate control – chapter 29. In: Stetson L, Dedrick A, editors. Irrigation. 6th ed. Falls, Church, VA: The Irrigation Association; p. 1015–1036.
- Folta KM, Carvalho SD. 2015. Photoreceptors and control of horticultural plant traits. HortScience. 50(9):1274–1280.
- Goulas V, Vicente AR, Manganaris GA. 2012. Chapter 10 – structural diversity of anthocyanins in fruits. In: Motohashi N, editor. Anthocyanins: structure, biosynthesis and health benefits. Pub. 2012. New York: Nova Science; p. 225–250.
- Gouws A. 2010. Optimum temperatures for colour development in apples. Masters of Agricultural Science, University of Stellenbosch, South Africa 94 p.
- Guo J, Han W, Wang M-H. 2008. Ultraviolet and environmental stresses involved in the induction and regulation of anthocyanin biosynthesis: a review. African Journal of Biotechnology. 7(25):4966–4972.
- Gurnsey S, Lawes GS. 2014. Improving Apple Color WSU Tree Fruit Research and Extension Center, Postharvest Information Network, Originally published in The Orchardist of NZ 1999. [accessed 2014 June 6]. http://postharvest.tfrec.wsu.edu/pages/RP2000A.
- Heijde M, Ulm R. 2012. UV-B photoreceptor-mediated signalling in plants. Trends in Plant Science. 17(4):230–237. doi: 10.1016/j.tplants.2012.01.007
- Hichri I, Barrieu F, Bogs J, Kappel C, Delrot S, Lauvergeat V. 2011. Recent advances in the transcriptional regulation of the flavonoid biosynthetic pathway. Journal of Experimental Botany. 19. doi:10.1093/jxb/erq442.
- Hogewoning SW, Douwstra P, Trouwborst G, Van Ieperen W, Harbinson J. 2010. An artificial solar spectrum substantially alters plant development compared with usual climate room irradiance spectra. Journal of Experimental Botany. 61(5):1267–1276. doi: 10.1093/jxb/erq005
- Hu DG, Sun CH, Ma QJ, You CX, Cheng LHY. 2016. MdMYB1 regulates anthocyanin and malate accumulation by directly facilitating their transport into vacuoles in apples. Plant Physiology. 170(3):1315–1330.
- Human JP. 2013. Breeding blush pears (Pyrus communis L.) in South Africa. Acta Horticulturae. 976:383–388. doi: 10.17660/ActaHortic.2013.976.52
- Huysamer M. 1998. Report of the blushed pear workgroup: Perceptions, facts and questions, p. 187–192. Proceedings Cape Pomology Association Technical Symposium, Cape Town, South Africa, 2–3 June.
- Jaakola L. 2013. New insights into the regulation of anthocyanin biosynthesis in fruits. Trends in Plant Science. 18(9):477–483. doi: 10.1016/j.tplants.2013.06.003
- Kayesh E, Shangguan L, Korir N, Sun X, Bilkish N, Zhang Y, Han J, Song C, Cheng Z-M, Fang J. 2013. Fruit skin color and the role of anthocyanin. Acta Physiologiae Plantarum. 35:2879–2890. doi: 10.1007/s11738-013-1332-8
- Kevany BM, Van Agtmael R, Dilley DR, Golding JB. 2003. Postharvest temperature affects colour development in ‘Rome’ apples. Acta Horticulturae. 628:623–625. doi: 10.17660/ActaHortic.2003.628.79
- Kim SH, Lee JR, Hong ST, Yoo YK, An G, Kim SR. 2003. Molecular cloning and analysis of anthocyanin biosynthesis genes preferentially expressed in apple skin. Plant Science. 165: 403–413. doi: 10.1016/S0168-9452(03)00201-2
- Koes R, Verweij W, Quattrocchio F. 2005. Flavonoids: a colorful model for the regulation and evolution of biochemical pathways. Trends in Plant Science. 10:236–242. doi: 10.1016/j.tplants.2005.03.002
- Lalusin AG, Nishita K, Kim S, Ohta M, Fujimura T. 2006. A new MADS-box gene (IbMADS10) from sweet potato (Ipomoea batatas (L.) Lam) is involved in the accumulation of anthocyanin. Molecular Genetics and Genomics. 275:44–54. doi: 10.1007/s00438-005-0080-x
- Li L, Ban ZJ, Li XH, Wu MY, Wang AL, Jiang YQ, Jiang YH. 2012. Differential expression of anthocyanin biosynthetic genes and transcription factor PcMYB10 in pears (Pyrus communis L.). PLoS One. 7(9):e46070. doi:10.1371/journal.pone.0046070. Epub 2012 Sep 28.
- Li P, Cheng L. 2009. The elevated anthocyanin level in the shaded peel of ‘Anjou’ pear enhances its tolerance to high temperature under high light. Plant Science. 177(5):418–426. doi: 10.1016/j.plantsci.2009.07.005
- Li P, Zhang Y, Einhorn TC, Cheng L. 2014. Comparison of phenolic metabolism and primary metabolism between green ‘Anjou’ pear and its bud mutation, red ‘Anjou’. Physiologia Plantarum. 150(3):339–354. doi: 10.1111/ppl.12105
- Li YY, Mao K, Zhao C, Zhao XY, Zhang RF, Zhang HL, Shu HR, Hao YJ. 2013. Molecular cloning and functional analysis of a blue light receptor gene MdCRY2 from apple (Malus domestica). Plant Cell Reports. 32(4):555–566. doi: 10.1007/s00299-013-1387-4
- Lin-Wang K, Bolitho K, Grafton K, Kortstee A, Karunairetnam S, McGhie TK, Espley RV, Hellens RP, Allan AC. 2010. An R2R3 MYB transcription factor associated with regulation of the anthocyanin biosynthetic pathway in Rosaceae. BMC Plant Biology. 10:50, 17 p. doi: 10.1186/1471-2229-10-50
- Lister CE. 1994. Biochemistry of fruit colour in apples (Malus pumila Mill.) [PhD thesis]. New Zealand: University of Canterbury. 256 p.
- Liu Y, Che F, Wang L, Meng R, Zhang X, Zhao Z. 2013. Fruit coloration and anthocyanin biosynthesis after bag removal in non-red and red apples (Malus × domestica Borkh.). Molecules. 18:1549–1563. doi: 10.3390/molecules18021549
- Luo H, Deng S, Fu W, Zhang X, Zhang X, Zhang Z, Pang X. 2017. Characterization of active anthocyanin degradation in the petals of Rosa chinensis and Brunfelsia calycina reveals the effect of gallated catechins on pigment maintenance. International Journal of Molecular Sciences. 18:699–716. doi: 10.3390/ijms18040699
- Luo J, Nishiyama Y, Fuell C, Taguchi G, Elliott K, Hill L, Tanaka Y, Kitayama M, Yamazaki M, Bailey P, et al. 2007. Convergent evolution in the BAHD family of acyl transferases: identification and characterization of anthocyanin acyl transferases from Arabidopsis thaliana. Plant Journal. 50:678–695. doi: 10.1111/j.1365-313X.2007.03079.x
- Marais E, Jacobs G, Holcroft DM. 2001. Postharvest irradiation enhances anthocyanin synthesis in apples but not in pears. HortScience. 36(4):738–740.
- McCaskill MR, McClymont L, Goodwin I, Green S, Partington DL. 2016. How hail netting reduces apple fruit surface temperature: a microclimate and modelling study. Agricultural and Forest Meteorology. 226–227:148–160. doi: 10.1016/j.agrformet.2016.05.017
- McClymont L, Goodwin I, Turpin S, Darbyshire R. 2016. Fruit surface temperature of red-blushed pear: threshold for sunburn damage. New Zealand Journal of Crop and Horticultural Science. 44(4):262–273. doi: 10.1080/01140671.2016.1216867
- Mori K, Goto-Yamamoto N, Kitayama M, Hashizume K. 2007. Loss of anthocyanins in red-wine grape under high temperature. Journal of Experimental Botany. 58(8):1935–1945. doi: 10.1093/jxb/erm055
- Oancea S, Oprean L. 2011. Anthocyanins, from biosynthesis in plants to human health benefits – review. Acta Universitatis Cibiniensis Series E: Food Technology. 15(1):3–16.
- Oren-Shamir M. 2009. Does anthocyanin degradation play a significant role in determining pigment concentration in plants? Plant Science. 177(4):310–316. doi: 10.1016/j.plantsci.2009.06.015
- Palmer J, Lozano L, Chagné D, Volz R, Lin-Wang K, Bonany J, Micheletti D, Troggio M, White A, Kumar S, et al. 2012. Physiological, molecular and genetic control of apple skin colouration under hot temperature environments. Acta Horticulturae. 929:81–87. doi: 10.17660/ActaHortic.2012.929.10
- Passeri V, Koes R, Quattrocchio FM. 2016. New challenges for the design of high value plant products: stabilization of anthocyanins in plant vacuoles. Frontiers in Plant Science. 7:153. doi:10.3389/fpls.2016.00153.
- Petroni K, Tonelli C. 2011. Recent advances on the regulation of anthocyanin synthesis in reproductive organs. Plant Science. 181(3):219–229. doi: 10.1016/j.plantsci.2011.05.009
- Pierantoni L, Dondini L, De Franceschi P, Musacchi S, Winkel BS, Sansavini S. 2010. Mapping of an anthocyanin-regulating MYB transcription factor and its expression in red and green pear, Pyrus communis. Plant Physiology and Biochemistry. 48(12):1020–1026. doi: 10.1016/j.plaphy.2010.09.002
- Privé JP, Russell L, LeBlanc A. 2008. Use of extenday reflective groundcover in production of ‘Gala’ apples (Malus domestica) in New Brunswick, Canada: 1. Impact on canopy microclimate and leaf gas exchange. New Zealand Journal of Crop and Horticultural Science. 36:221–231. doi: 10.1080/01140670809510238
- Privé JP, Russell L, LeBlanc A. 2011. Impact of reflective groundcover on growth, flowering, yield and fruit quality in Gala apples in New Brunswick. Canadian Journal of Plant Science. 91:765–772. doi: 10.4141/cjps10199
- Qian M, Zhang D, Yue X, Wang S, Li X, Teng Y. 2013. Analysis of different pigmentation patterns in ‘Mantianhong’ (Pyrus pyrifolia Nakai) and ‘Cascade’ (Pyrus communis L.) under bagging treatment and postharvest UV-B/visible irradiation conditions. Scientia Horticulturae. 151:75–82. doi: 10.1016/j.scienta.2012.12.020
- Racsko J, Schrader E. 2012. Sunburn of apple fruit: historical background, recent advances and future perspectives. Critical Reviews in Plant Sciences. 31:455–504. doi: 10.1080/07352689.2012.696453
- Rakkimuthu R, Palmurugan S, Shanmugapriya A. 2016. Effect of temperature, light, pH on the stability of anthocyanin pigments in Cocculus hirsutus fruits. International Journal of Multidisciplinary Research and Modern Education. 2(2):91–96.
- Reay PF. 1999. The role of low temperatures in the development of the red blush on apple fruit (‘Granny Smith’). Scientia Horticulturae. 79:113–119. doi: 10.1016/S0304-4238(98)00197-6
- Roberts SC, Steyn WJ, North MS. 2008. Effect of rootstock on red colour of bi-coloured ‘Forelle’ pears. Acta Horticulturae. 800:625–631. doi: 10.17660/ActaHortic.2008.800.83
- Saure MC. 1990. External control of anthocyanin formation in apple. Scientia Horticulturae. 42:181–218. doi: 10.1016/0304-4238(90)90082-P
- Senthilkumar S, Vijayakumar RM. 2014. Biochemical, physiological and horticultural perspectives of fruit colour pigmentation: a review. Research and Reviews: Journal of Agriculture and Allied Sciences. 3(1):9–16.
- Shaked-Sachray L, Weiss D, Reuveni M, Nissim-Levi A, Oren-Shamir M. 2002. Increased anthocyanin accumulation in aster flowers at elevated temperatures due to magnesium treatment. Physiologia Plantarum. 114:559–565. doi: 10.1034/j.1399-3054.2002.1140408.x
- Smillie RM, Hetherington SE. 1999. Photoabatement by anthocyanins shields photosynthetic systems from light stress. Photosynthetica. 36:451–463. doi: 10.1023/A:1007084321859
- Solomakhin AA, Blanke MM. 2007. Overcoming adverse effects of hailnets on fruit quality and microclimate in an apple orchard. Journal of the Science of Food and Agriculture. 87(14):2625–2637. doi: 10.1002/jsfa.3022
- Solomakhin AA, Blanke MM. 2010. The microclimate under coloured hailnets affects leaf and fruit temperature, leaf anatomy, vegetative and reproductive growth as well as fruit colouration in apple. Annals of Applied Biology. 156(1):121–136. doi: 10.1111/j.1744-7348.2009.00372.x
- Steyn WJ. 2003. Red colour development and loss in pear fruit [PhD-thesis]. South Africa: University of Stellenbosch.
- Steyn WJ, Holcroft DM, Wand JE, Jacobs G. 2004a. Regulation of pear color development in relation to activity of flavonoid enzymes. Journal of the American Society for Horticultural Science. 129(1):6–12.
- Steyn WJ, Holcroft DM, Wand JE, Jacobs G. 2004b. Anthocyanin degradation in detached pome fruit with reference to preharvest red color loss and pigmentation patterns of blushed and fully red pears. Journal of the American Society for Horticultural Science. 129(1):13–19.
- Steyn WJ, Wand SJE, Holcroft DM, Jacobs G. 2005. Red colour development and loss in pears. Acta Horticulturae. 671:70–85.
- Stommel JR, Lightbourn GJ, Winkel BS, Griesbach RJ. 2009. Transcription factor families regulate the anthocyanin biosynthetic pathway in Capsicum annuum. Journal of the American Society for Horticultural Science. 134(2):244–251.
- Strissel T, Halbwirth H, Hoyer U, Zistler C, Stich K, Treutter D. 2005. Growth promoting nitrogen nutrition affects flavonoid biosynthesis in young apple (Malus domestica Borkh.) leaves. Plant Biology. 7:677–685. doi: 10.1055/s-2005-872989
- Thomson G, Goodwin I. 2016. Review of pre-harvest determinants of blush in ‘Cripps Pink’ and other red apple varieties. Report for Agriculture Victoria – Department of Economic Development, Jobs, Transport & Resources. 16 p.
- Thomson G, McCaskill M, McClymont L, Goodwin I. 2015. Sunburn protection Part 3. Fruit protection with spray-on products, shade-netting or evaporative cooling can help reduce sunburn during heatwaves. Tree Fruit Magazine pages 3–5, January 2015.
- Ubi BE. 2004. External stimulation of anthocyanin biosynthesis in apple fruit. Food, Agriculture and Environment. 2(2):65–70.
- Ubi BW, Honda C, Bessho H, Kondo S, Wada M, Kobayashi S, Moriguchi T. 2006. Expression analysis of anthocyanin biosynthetic genes in apple skin: effect of UV-B and temperature. Plant Science. 170:571–578. doi: 10.1016/j.plantsci.2005.10.009
- Veens M. 2009. Fruit colour. Future Orchards 2012, 5 p.
- Wand SJE, Steyn WJ, Holcroft DM, Mdluli MJ, van den Dool K, Jacobs G. 2005. Use of evaporative cooling to improve ‘Rosemarie’ and ‘Forelle’ pear fruit blush colour and quality. Acta Horticulturae. 671:103–111. doi: 10.17660/ActaHortic.2005.671.12
- Wang H, Cheng L. 2011. Differential effects of nitrogen supply on skin pigmentation and flesh starch breakdown of ‘Gala’ apple. HortScience. 46(8):1116–1120.
- Wang H, Li M-F, Yang Y, Jin W-M. 2015. Recent advances on the molecular mechanisms of anthocyanin synthesis in fruits. Plant Physiology Journal. 51(1):29–43.
- Wang KL, Micheletti D, Palmer J, Volz R, Lozano L, Espley R, Hellens RP, Chagnè D, Rowan DD, Troggio M, et al. 2011. High temperature reduces apple fruit color via modulation of the anthocyanin regulatory complex. Plant, Cell and Environment. 34:1176–1190. doi: 10.1111/j.1365-3040.2011.02316.x
- Wang Z, Meng D, Wang A, Li T, Jiang S, Cong P, Li T. 2013. The methylation of the PcMYB10 promoter is associated with green-skinned sport in Max Red Bartlett pear. Plant Physiology. 162(2):885–896. doi: 10.1104/pp.113.214700
- Whale SK, Singh Z. 2007. Endogenous ethylene and colour development in the skin of ‘Pink Lady’ apple. Journal of the Amercian Society for Horticultural Science. 132:20–28.
- Willaume M, Lauri P-E, Sinoquet H. 2004. Light interception in apple trees influenced by canopy architecture manipulation. Trees. 18:705–713. doi: 10.1007/s00468-004-0357-4
- Wilton J, Hornblow C. 2003. Thinning, crop load and quality management of Pink Lady. Report for the New Zealand Pink Lady Growers Association. 5 p.
- Wu J, Zhao G, Yang YN, Le WQ, Khan MA, Zhang SL, Gu C, Huang W-J. 2013. Identification of differentially expressed genes related to coloration in red/green mutant pear (Pyrus communis L.). Tree Genetics and Genomes. 9(1):75–83. doi: 10.1007/s11295-012-0534-3
- Xu W, Dubos C, Lepiniec L. 2015. Transcriptional control of flavonoid biosynthesis by MYB-bHLH-WDR complexes. Trends in Plant Science. 20(3):176–185. doi: 10.1016/j.tplants.2014.12.001
- Xu X, Feng S, Jiao Q, Liu C, Zhang W, Chen W, Chen X. 2012. Comparison of MdMYB1 sequences and expression of anthocyanin biosynthetic and regulatory genes between Malus domestica Borkh. cultivar ‘Ralls’ and its blushed sport. Euphytica. 185:157–170. doi: 10.1007/s10681-011-0494-y
- Yang Y, Yao G, Yue W, Zhang S, Wu J. 2015a. Transcriptome profiling reveals differential gene expression in proanthocyanidin biosynthesis associated with red/green skin color mutant of pear (Pyrus communis L.). Frontiers in Plant Science 6: article 795, 14 p.
- Yang Y, Yao G, Zheng D, Zhang SL, Wang C, Zhang M, Wu J. 2015b. Expression differences of anthocyanin biosynthesis genes reveal regulation patterns for red pear coloration. Plant Cell Reports. 34(2):189–198. doi: 10.1007/s00299-014-1698-0
- Yang Y, Zhao G, Yue W-Q, Zhang S-L, Gu C, Wu J. 2013. Molecular cloning and gene expression differences of the anthocyanin biosynthesis-related genes in the red/green skin color mutant of pear (Pyrus communis L.). Tree Genetics and Genomes. 9(5):1351–1360. doi: 10.1007/s11295-013-0644-6
- Zoratti L, Karppinen K, Luengo Escobar A, Häggman H, Jaakola L. 2014a. Light-controlled flavonoid biosynthesis in fruits. Frontiers in Plant Science 5: article 534, 16 p.
- Zoratti L, Sarala M, Carvalho E, Karppinen K, Martens S, Giongo L, Häggman H, Jaakola L. 2014b. Monochromatic light increases anthocyanin content during fruit development in bilberry. BMC Plant Biology. 14:377, 10 p. doi: 10.1186/s12870-014-0377-1