ABSTRACT
A pot experiment evaluated the effect of seed vigour and biopriming with Trichoderma harzianum on growth of soybean seedlings under NaCl stress. The Trichoderma isolate was more effective in improving the emergence rate of seedlings produced from high-vigour seeds under saline conditions. Seedlings obtained from bioprimed vigorous seeds had a significantly higher root and shoot length as well as leaf greenness than the control group at all salinity concentrations. The treatment, non-aged high-vigour seeds showed a greater accumulation of proline in the roots and leaves of the pre-treated seedlings under saline conditions. The highest catalase activity was observed in the root and the leaf of the bioprimed low-vigour seed lot at a salt stress level of 6 dS/m. A similar pattern of guaiacol peroxidase activity was induced in root and leaf of seedlings obtained from pre-treated high-vigour seeds in response to NaCl stress. It can be deduced that seed invigoration with Trichoderma harzianum diminished the deleterious impacts of salinity, although the amelioration was better in bioprimed high-vigour seeds under the present experimental conditions.
Introduction
Soybean is the leading globally produced oilseed crop. Many biotic and abiotic stresses limit soybean production in different parts of the world (Singh and Shivakumar Citation2010). Of these, soil salinity is a major factor limiting soybean performance, especially in early seedling development. Soybean is considered a moderately salt-tolerant crop and the reported salinity threshold of soybean is about 5 dS/m (Rao and Reddy Citation2010). Salinity, as an abiotic hazard, induces numerous disorders in seeds during germination and subsequent seedling emergence. High levels of soil salinity can impair both seed and seedling vigour as a consequence of the combined effects of high osmotic potential and specific ion toxicity (Mahajan and Tuteja Citation2005). Salt-induced poor stand establishment adversely affects the growth of field crops, thereby resulting in low productivity (Wahid et al. Citation1999).
Salt stress effects the initial seedling development of soybean ranging from the loss of seed germinability and seedling growth to the induction of physiological and biochemical changes (Manchanda and Garg Citation2008). Plant root and shoot growth were retarded due to the inhibition of cell elongation mediated by salinity (Bandeoglu et al. Citation2004). Salinity involves an osmotic stress induced by the salts in the growing medium, thereby resulting in the disturbance of ion balance and the distribution of plant cells (Hameed et al. Citation2010). As pointed out by other researchers, salt-stressed plants show impaired levels of leaf chlorophyll (Manchanda and Garg Citation2008) and proline content (Garg Citation2004). A secondary aspect of salinity stress in plants is the stress-induced production of reactive oxygen species (ROS). The ROS are products of altered chloroplast and mitochondrial metabolism during stress. These species cause oxidative damage to different cellular components including membrane lipids, proteins, and nucleic acids (Bailly Citation2004). As a consequence, salinity stress often triggers cell signalling pathways including those that lead to a synthesis of compatible osmolytes, such as proline, and free radical scavenging enzymes, which adjust water relations and launch the scavenging of the ROS. ROS detoxification through the promotion of antioxidative enzymes, including catalase, peroxidise, and superoxide dismutase, constitute an important defence system against salt stress (Gomes and Garcia Citation2013).
Since the seed vigour status is the determining factor that influences field establishment and the subsequent performance of plants (Javadi et al. Citation2016), it seems that the negative impact of salinity on crop growth and yield could be mainly related to the initially poor seed vigour. On the other hand, physiological invigoration of seeds and improved seedling performance, especially under stressful conditions, has been necessitated to ensure promising plant productivity (Ghassemi-Golezani et al. Citation2008b). The introduction of a high-vigour seed lot (Khajeh-Hosseini et al. Citation2003; Javadi et al. Citation2016) and the incorporation of Trichoderma as a potential seed biopriming agent (Rawat et al. Citation2011; Shukla et al. Citation2015) in some crops have been regarded as ‘seed enhancement’ approaches in recent studies.
Oilseeds are very sensitive to lipid peroxidation caused by a ROS attack on unsaturated fatty acids; this accelerates deteriorative events in seeds. Seed vigour is an important quality that is lost due to seed deterioration, reducing the germination capacity and seedling vigour (McDonald Citation1999; Basra et al. Citation2003). High-vigour seeds rapidly germinate and produce robust seedlings, especially under abiotic stresses (Ellis and Roberts Citation1981; Rehman et al. Citation1999; Ghassemi-Golezani et al. Citation2008b). Seed aging can be caused by deteriorative events occurring with time, increasing seed vulnerability to external challenges and decreasing the ability of the seed to survive. Nearly all the subcellular components are affected by the deterioration process, leading to the syndrome of ‘low vigour’ (Roberts and Osei-Bonsu Citation1988; Ghassemi-Golezani et al. Citation2008b).
Biopriming, as one of the cost-effective seed pre-treatment techniques, refers to a combination of seed moistening and inoculation with biological materials for seed protection (Reddy Citation2013). Seeds evenly germinate under stressful conditions through the application of biopriming. Bio-control agents, such as Trichoderma, produce a variety of compounds that induce tolerance responses to biotic and abiotic stress factors (Harman et al. Citation2004). For example, biopriming with T. Harzianum offers an effective biological seed pre-treatment system and an alternative to fungicide for controlling the damping off of soybean caused by Colletotrichum truncatum (Begum et al. Citation2010). Some compatible osmolytes have been detected under saline conditions and proposed as part of a mechanism that provokes salinity tolerance in plants (Ashraf and Foolad Citation2007). The role of proline in salinity response has long been known in published literature. Free proline is one of the best known solutes, and its key role in salt tolerance induction and protective function during stress was demonstrated in a recent work by Rawat et al. (Citation2011). Some experiments have indicated that root inoculation by Trichoderma harzianum results in an elevated level of antioxidative enzymes, including various peroxidises to provide substantial stress tolerance (Harman Citation2006; Shoresh et al. Citation2010; Hamilton et al. Citation2012). Together, these strategies constitute a ‘priming memory’ in seeds, which can be drawn on during subsequent stress exposure and mediates a greater stress tolerance of germinating primed seeds. Seed priming has been successfully shown to improve seed germination and emergence in many crops, particularly under stressful conditions (Chen and Arora Citation2013).
Earlier, some efforts had been made to alleviate the injurious impacts of salinity on seed and seedling vigour by employing certain chemical and biochemical agents. The aim of the present research was to evaluate the influences of the biopriming of soybean seeds with the salinity-tolerant isolate of Trichoderma harzianum upon germinability, subsequent seedling growth, and some biochemical aspects under normal and saline conditions. In the present research, we have determined and assessed antioxidative enzyme activities and proline accumulation in the seedlings of bioprimed and unprimed seeds in order to utilise salt-tolerant Trichoderma isolate in relation to salt stress tolerance in soybean. This work is important as it demonstrates the collaborative role of seed vigour and Trichoderma harzianum in providing stress tolerance and creating awareness of the potential for soybean plants to be cultivated in salt-affected soils.
Materials and methods
Experiment and treatment combinations
The present experiment was conducted during 2014–2015 in the Department of Agronomy and Plant Breeding, Faculty of Agricultural Science at the University of Mohaghegh Ardabili, Ardabil, Iran. The seed lot of soybean (Glycine max L.) cv. Williams was obtained from SPCRI (Seed and Plant Certification and Registration Institute, Karaj), and then divided into two subsamples. A subsample was kept as control or a non-deteriorated seed lot. By applying the rapid-aging test (Ellis and Roberts Citation1981), the other subsample with about 16% moisture content was artificially deteriorated at 40°C for three days. As a result, the two seed lots had seed viabilities of 98% (high vigour) and 88% (low vigour), respectively. Before inoculation, the seeds were surface sterilised in 1% sodium hypochlorite for 5 min, and then thoroughly rinsed with ddH2O and air-dried.
Twelve Trichoderma isolates, which were collected from different salt-affected soils located in the Ardabil plains in north-west Iran and prepared at the Plant Pathology Laboratory, were considered for this research. The brief selection method of salt-tolerant Trichoderma isolate was as follows: the isolation was made by the Dilution Plate Technique using samples from the rhizosphere soil of soybean fields. Two millilitres of 10−4 dilution was spread on sterilised PDA petri dishes after autoclaving the medium and incubated at 25 ± 1°C under 12 h of both light and dark periods. The plates were rotated manually for uniform distribution and the suspension in the medium was allowed to solidify. The growing colonies of mycoflora were observed under the microscope, characterised by Rifai (Citation1969). The identified Trichoderma harzianum was purified by a hyphal segment culture and maintained under refrigeration at 4°C for more application. The purified isolates were incubated on an 80 g PDA medium in 13 cm Petri dishes at different NaCl (0, 40, 80, and 120 mM) concentrations and were assessed for their growth performance and population (CFU g−1 of air-dried substrate). Two mediums for each salinity level were kept and autoclaved at 121°C for 30 min. The mediums were inoculated with 2 ml Trichoderma suspension (107 spores ml−1) of individual isolate and incubated at 25 ± 1°C for 10 days. The best isolate (T-22) was selected as the salinity-tolerant Trichoderma harzianum on the basis of growth performance at different NaCl concentrations for further investigations to test its capability to gain salt stress tolerance in soybean. After the pre-soaking of seeds in water for 12 h, a soybean seed lot was mixed with the salt-tolerant isolate of T. harzianum @ 10 g/kg of seeds. The seed lot was then incubated under warm (28–30°C) and moist conditions until just before radicle protrusion. The heap contained soybean seeds and the Trichoderma hyphae were covered with a moist jute sack to maintain high humidity (Reddy Citation2013).
Non- and bioprimed seeds were sown in 25-cm diameter plastic pots containing perlite (previously tested to not release any salt) and placed in an open-air vegetation yard in four blocks. Such conditions secured natural temperature and light conditions. The maximum and minimum temperatures in early spring (month of June) were 14°C and 29°C, respectively. The mean radiation on top of the seedlings reached 19 MJ/m2/day during the period of the experiment (28 days). Each pot was filled with 1 kg of perlite. The plants were exposed to non-saline condition (control) and two levels of salinity viz. 3 and 6 dS/m. For salinity treatments, NaCl was added to the Hoagland nutrient solution to set the different preferred ECs. The pH was always checked and adjusted around 6.7. Hoagland solutions with varying salinities were added to the pots in accordance with the treatments to achieve 100% FC. During the growth period, the pots were weighed and the losses were made up with Hoagland solution (EC = 0.54 dS/m). The nutrient solutions were renewed every week.
The experiment was carried out in a randomised complete block (RCB) design with four replications. The present factorial experiment included three factors of salinity (0, 3, and 6 dS/m), seed vigour (98 and 88% viability), and biopriming (control and Trichoderma). Twenty-five seeds per pot were sown. After seedling establishment, each pot was thinned to eight plants. At the end of the experiment, the EC of the perlite in plastic pots was tested at regular intervals and the final values were recorded to be 0.54, 3.13, and 5.92 dS/m in the pots with 0, 3, and 6 dS/m salinity treatments, respectively. All the observations were recorded 28 days after sowing using the youngest, fully expanded leaves. Such leaf samples were collected from three plants per treatment, per replicate.
Observed data
Seedling emergence was recorded at daily intervals and the emergence rate was calculated according to Ellis and Roberts (Citation1981). The root and shoot length of the four seedlings (28 days old) were randomly measured. The leaf greenness (total chlorophyll index) was estimated using a portable SPAD-502 chlorophyll metre (Minolta, Japan).
All extraction procedures were carried out at 4°C. Soybean leaf (0.1 g FW per sample) was ground in 2 ml of potassium phosphate buffer (100 mM, pH = 7.8) containing 0.2 mM a-dithiothreitol and10 μM EDTA, and mixed for 15 min. The homogenate was centrifuged at 12,000 g for 15 min, and then the supernatant was used for assays. Catalase (CAT, EC 1.11.1.6) activity was determined spectrophotometrically (two measurements per extract) following H2O2 consumption at 240 nm. The results were expressed as an enzyme unit, i.e. as μmol H2O2 decomposed min−1 (Bailly et al. Citation1998).
Guaiacol peroxidase (POD, EC 1.11.1.7) was extracted from the soybean leaves in accordance with the work of MacAdam et al. (Citation1992) with less modification. Fresh leaves (0.1 g FW) were homogenised in a 1 ml extraction buffer using a pre-chilled pestle and mortar at 4°C. The homogenate was centrifuged at 12,000 g for 15 min at 4°C. Then, 100 μl enzyme extract was added to 3 ml of 100 mM phosphate buffer (pH = 6) containing 200 mM guaiacol and 30 mM hydrogen peroxide. Subsequently, at the end of the reaction time (5 min) and lab temperature, the absorbance (two measurements per extract) was read at 470 nm. The extinction coefficient of tetra-guaiacol (26.6 mM−1 cm−1) was used to calculate the unit of this enzyme, i.e. μmol tetra-guaiacol produced min−1.
The proline content of leaves was determined according to Bates et al. (Citation1973). In brief, leaf tissue (0.2 g FW) was homogenised in 4 ml of sulphosalicylic acid (3%) and centrifuged at 10,000 g for 30 min. About 2 ml of the supernatant was taken in a test tube, and 2 ml of glacial acetic acid and 2 ml ninhydrin reagent were added. The reaction mixture was boiled in a water bath at 100°C for 30 min. After cooling the reaction mixture, 4 ml of toluene was added and vortexed for 30 s, the absorbance of the upper phase was read with a spectrophotometer at 520 nm using toluene as a blank. The proline content (μmol g−1 FW) was measured using l-proline as the standard.
Statistical analysis
Data were analysed using the SAS 6.2 programme (SAS Institute Inc. Citation1998). The Proc-general linear model procedure was used to perform the ANOVA test and to determine the statistical significance of experimental factor effects. An analysis of the variance was carried out according to the factorial layout, based on an RCB design with four replications. The comparison of the means was carried out using Tukey’s test at a probability of p < .05. The whole experiment was repeated twice and the obtained data were pooled based on the equality of the variances, according to the result of Bartlett’s sphericity test.
Results
In our research, the salinity treatments of 0, 3, and 6 dS/m were applied. An analysis of the variances () indicated significant differences among the experimental factors and two- and three-way interactions for nearly all the germinations, seedling growth, and biochemical parameters except the seedling emergence percentage for which only the main effects were statistically significant. The germinability and some biochemical consequences of salinity on invigoration treatments (seed vigour level and biopriming) were evaluated in relation to the percentage and rate of seedling emergence, root, and shoot length, leaf greenness, catalase and guaiacol peroxidase activity, and proline content in the roots and leaves.
Table 1. ANOVA showing F-ratios and significance levels of the data for germination, growth and biochemical parameters of soybean grown under three levels of salinity stress after biopriming of seed lots of different vigour with Trichoderma harzianum.
The effect of NaCl damage on early seedling establishment of soybean is shown in and . Soybean seedlings slowly emerged (reduction percentage up to 25.5 greater than control), as different NaCl concentrations were added to the growth medium (). Additionally, the data showed that the percentage and the rate of seedling emergence responses to applied NaCl concentrations were markedly influenced by the seed vigour status (, (A,B)). The deteriorated seed lot had the minimum percentage of seedling emergence with about 34% reduction compared to non-aged control (). High-vigour seeds had a more positive response to biopriming with Trichoderma ((B)). As a result, the biopriming of vigorous seeds enhanced the rate of seedling emergence about twofold compared to the aged, low-vigour seed lot.
Figure 1. Soybean seedling emergence influenced by (A) interaction of seed vigour level and salt stress and (B), interaction of seed vigour level and biopriming with Trichoderma.
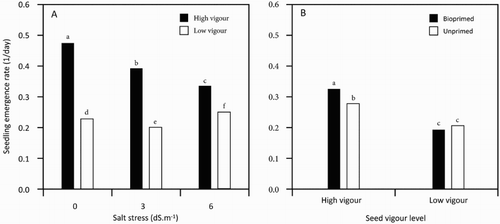
Table 2. Effect of salinity, seed vigour, and biopriming with Trichoderma on soybean seedling emergence.
The highest root () and shoot ((A,B)) lengths were found in the bio- and unprimed high-vigour seed lot including normal, non-saline conditions. The root and shoot lengths significantly decreased as the salt stress increased ( and (A,B)). The minimum root length was recorded in the unprimed, low-vigour seed lot at 6 dS/m (3.05 cm), followed by the same treatment at 3 dS/m (3.40 cm). Under mild salt stress (3 dS/m), the root in the bioprimed high-vigour seeds were longer than in the case of other treatments, including this concentration of NaCl (). The root length was reduced to almost more than 50% from 6.33 cm at 0 dS/m in bioprimed high-vigour seeds to 3.05 cm at 6 dS/m in untreated low-vigour ones. Taking into account the different NaCl concentrations, root and shoot lengths of seedlings from the high-vigour seed lot were significantly greater than that of the poor-vigour ones. Overall, it could be concluded that seedlings produced from bioprimed seeds had significantly higher shoot and root lengths than the control group under both non-saline and saline conditions.
Figure 2. Root length of soybean seedling influenced by seed vigour level and biopriming with Trichoderma under salt stress.
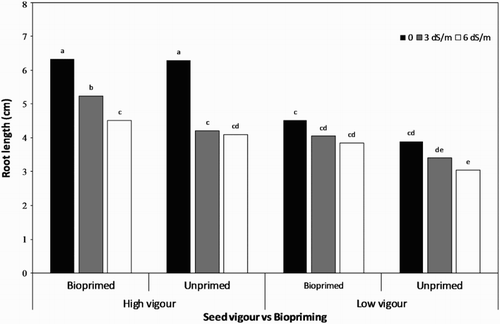
Figure 3. Shoot length of soybean seedling influenced by (A), interaction of seed vigour level and salt stress and (B) interaction of biopriming with Trichoderma and salt stress.
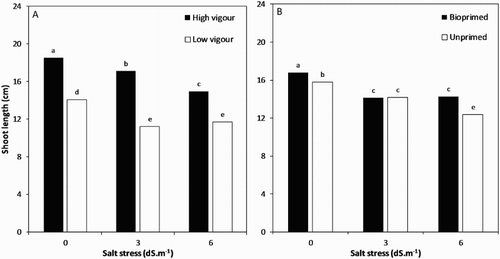
The leaf greenness (total chlorophyll index) averaged over the treatment combinations indicated that it significantly decreased (from 28.62 at 0 dS/m to 21.85 at 6 dS/m) with increases in salt concentrations (). The highest chlorophyll index under the non-saline condition was recorded in bioprimed high-vigour seeds (33.88), closely followed by the unprimed ones (32.11). Among treatment combinations (seed vigour level and biopriming) maximum leaf greenness was recorded in the bioprimed high-vigour seed lot (mean at salinity stress = 29.52), which was higher than that in the untreated low-vigour one (mean at salinity stress = 20.32).
Figure 4. Leaf greenness of soybean seedling influenced by seed vigour level and biopriming with Trichoderma under salt stress.
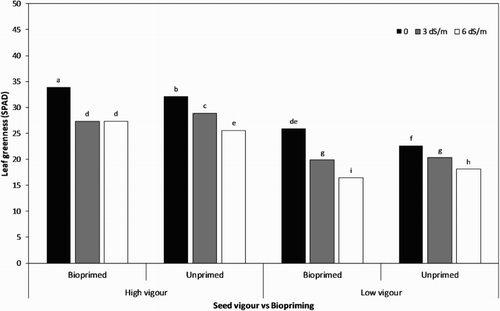
The root and leaf proline contents were significantly influenced by all the experimental factors. The proline content of the root increased at a higher salinity level of 6 dS/m (81.4 μmol g−1) compared with 0 dS/m (59.8 μmol g−1) in all the treatments ((A)). The treatment bioprimed low-vigour seed lot had the maximum root proline content (104.3 μmol g−1) at 6 dS/m followed by the same treatment combination (95.5 μmol g−1) at 3 dS/m, while the minimum root proline content was observed in the unprimed vigorous seed lot (46.46 μmol g−1) under a non-saline condition. Considering the mean value of treatment combinations, the low-vigour seed lot exhibited approximately 24% greater leaf proline content compared to the high-vigour one (). The proline content of leaf significantly increased as the NaCl concentration increased, particularly in soybean seeds pre-treated with Trichoderma ((B)).
Figure 5. (A) Root proline content of soybean seedling influenced by seed vigour level and biopriming with Trichoderma under salt stress. (B) Leaf proline content of soybean seedling influenced by interaction of biopriming with Trichoderma and salt stress.
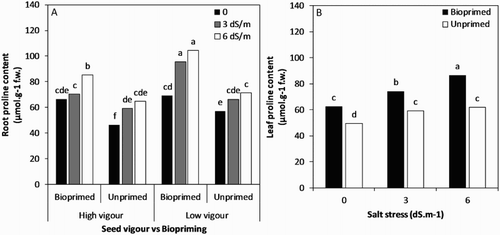
In general, an increase in the catalase activity was recorded under salt stress ((A,B)). However, a greater increase was observed in bioprimed seed lots. The maximum increase at 6 dS/m over no stress (relevant control) was observed in the bioprimed low-vigour seed lot (over 2-fold in root and 1.7-fold in leaf), followed by the same treatment combination at 3 dS/m ((A,B)); the lowest significant increase was noticed in the untreated low-vigour seed lot (27.4% in root) under mild salt stress ((A)).
Figure 6. (A) Root and (B) Leaf catalase activity influenced by seed vigour level and biopriming with Trichoderma under salt stress.
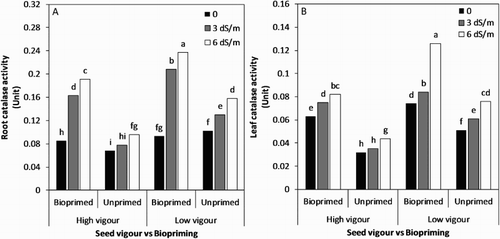
The activity of guaiacol peroxidase from soybean roots and leaves increased substantially with an increase in NaCl ((A,B)). The treatment combinations (seed biopriming and vigour level) differed significantly both under control (0 dS/m) and saline conditions. A significantly higher level of root guaiacol peroxidase activity was found in the Trichoderma-treated high-vigour seed lot as the salt stress increased up to 6 dS/m compared to the untreated seeds ((A)). The maximum increase at 6 dS/m over no stress was obtained in the bioprimed high-vigour seed lot (36.3% in root), followed by the bioprimed low-vigour one at the same salinity level. Overall, the greatest guaiacol peroxidase activity in leaf was recorded in the bioprimed seeds under saline conditions ((B)).
Discussion and conclusions
Considering the recent documentations on improvements in crop growth and yield under abiotic stresses, especially salinity, seed enhancement through the introduction of high-vigour seeds and the application of biopriming (seed pre-treatment with bio-inoculants such as Trichoderma) technique could be an efficient and convenient approach. In this context, one may point to the enhancing effect of seed vigour and biopriming with Trichoderma on salt tolerance capability of soybean (Khajeh-Hosseini et al. Citation2003) and wheat (Rawat et al. Citation2011), respectively. According to the results of previous authors, seed vigour not only affects seedling establishment (Perry Citation1984; Roberts and Osei-Bonsu Citation1988; Ghassemi-Golezani Citation1992; Finch-Savage Citation2000; Elias and Copeland Citation2001; Khajeh-Hosseini et al. Citation2003; Ghassemi-Golezani et al. Citation2008b), but can also greatly influence stress (including salinity, drought, and cold stresses) tolerance in field crops. On the other hand, Trichoderma-mediated changes in different physiological and metabolic aspects of plant growth under environmental stresses can lead to improvements in stress tolerance capability (Brotman et al. Citation2013). The results of the present research provided straightforward experimental indication that high-vigour seeds and seed biopriming with salt-tolerant isolate of Trichoderma harzianum simultaneously improved seedling growth under salinity in Glycine max.
In general, salinity caused substantial loss in the soybean seedling establishment. Our findings are in agreement with those of some other researchers, indicating that, in soybean, seedling establishment is significantly decreased by salt stress (Mannan et al. Citation2009; Dogan, Citation2011; Fan et al. Citation2013). As it is known, NaCl decreased the rate of germination due to the dehydration-induced slower imbibition. High amounts of salt unsettled the ionic equilibrium of plants and also caused imbalance in mineral nutrients, which influenced seed germination and seedling growth (Parida and Das Citation2005). It is fairly obvious from our work that high vigorous seeds show superiority compared to the aged and less vigorous ones in inducing salt tolerance during the early soybean seedling development at all stress levels. Khajeh-Hosseini et al. (Citation2003) reported a reduced seedling emergence in aged soybean seeds under salinity stress. The low germinability of deteriorated seeds is likely due to the interruption occurring at the beginning of the germination process in such seed lots (Bailly et al. Citation1998; Basra et al. Citation2003; Verma et al. Citation2003). On the other hand, a higher emergence rate was observed in the biopriming of vigorous seeds with Trichoderma. It is well documented that Trichoderma pre-treatment strengthens seeds and helps them germinate even under stress conditions (Shukla et al. Citation2015).
The increasing concentrations of NaCl caused more inhibitory impacts on soybean seedling growth parameters. It is possible that the decline in the root and shoot growth in NaCl-affected seedlings could be due to plasmolysis (Parida and Das Citation2005), downregulated photosynthesis, and disturbance in mineral supply (Jamil et al. Citation2007). However, seedlings produced from bioprimed vigorous seeds indicated higher root and shoot length. These results are in conformity with those of the other researchers (Khajeh-Hosseini et al. Citation2003; Ghassemi-Golezani et al. Citation2008b), who reported that high-vigour seeds produce vigorous seedlings that tolerate abiotic stress better than poorly vigorous ones. As mentioned by some authors, the reduction in seedling performance is the ultimate result of seed vigour loss (Verma et al. Citation2003). The application of Trichoderma in plants extends roots, which helps in greater water uptake, thereby enhancing the plants’ capability to tolerate environmental stresses (Arora et al. Citation1992). It is well understood that Trichoderma increases seedling height and biomass. On the other hand, seedling vigour, i.e. higher root and shoot length, is considered to have dilution effects on ion accumulation in plant shoots (Fan et al. Citation2013).
Seedlings exposed to NaCl generally had leaves with low greenness. Reduction in leaf greenness was probably due to the inhibitory effect of the acquired ions on the biosynthesis pathway of chlorophyll (Jamil et al. Citation2007), thus inducing the destruction of the chloroplast ultra-structure and the loss of pigment complexes (Sudhir and Murthy Citation2004). It has been indicated that leaf chlorophyll content diminishes in salinity-susceptible plants such as common bean (Yasar et al. Citation2008) and soybean (Mannan et al. Citation2009). In the present research, the maximum leaf greenness was recorded in the Trichoderma-treated high-vigour seed lot while the minimum leaf greenness was in the control (unprimed and aged) seedlings under all three salinity levels, respectively. Rawat et al. (Citation2011) found that the application of Trichoderma as a biopriming tool led to the prevention of NaCl-induced leaf chlorosis in wheat seedlings. On the other hand, a few reports about the increase in leaf chlorophyll content in seedlings obtained from high-vigour seeds (Ghassemi-Golezani et al. Citation2008a; Huang et al. Citation2017) have been reported. However, the high salt-mediated leaf chlorosis was inevitable in all the seed invigoration pre-treatments.
Some amino acids, such as free proline, have been reported to accumulate in higher plants under environmental stresses. High proline levels can increase the salt tolerance of plants through stabilising antioxidative enzymes and equilibrating the osmotic potential with that of external medium (Gill and Tuteja Citation2010). In addition, these osmolytes interact with macromolecules including enzymes and maintain their functionality (Parida and Das Citation2005). The higher proline concentration in seedlings from Trichoderma-treated seeds could confer some adaptive advantages under salinity stress (Rawat et al. Citation2011). Gill and Tuteja (Citation2010) reported that proline protected cellular functions by scavenging ROS. In soybean (Dogan Citation2011), wheat (Rawat et al. Citation2011), and pea (Ozturk et al. Citation2012), the same trends of elevation in leaf proline content with an increase in NaCl concentration were recorded. On the other hand, proline accumulation in seedlings obtained from the aged seed lot could also be considered as a stress-induced marker for oxidative damage (Kong et al. Citation2015).
An increase in the activities of catalase and guaiacol peroxidase from soybean root and leaf, crucial to the detoxification of ROS, was greater in the Trichoderma-treated seed lots under salt stress. To mitigate and repair the damage mediated by ROS, plants have developed a complex antioxidant system. The primary components of this system include enzymes such as superoxide dismutase, catalase, and peroxidases (Walters et al. Citation2010). Kibinza et al. (Citation2011) reported the key role of antioxidative enzymes mediating oxidation protection during seed ageing and repair during the subsequent priming treatment of sunflower seeds. As reported by Brotman et al. (Citation2013), salt stress tolerance in cucumber seedlings induced by Trichoderma is dependent on the activation of the plant antioxidant defence machinery. On the other hand, it can be concluded from the susceptibility of seedlings produced from aged, low-vigour seeds to NaCl that an increase in catalase activity was a result of deterioration-induced peroxidation (McDonald Citation1999); this was not necessarily indicative of stress tolerance in such poor-vigour plants.
The findings of this experiment demonstrated that the use of non-aged, high-quality seeds and the application of T. Harzianum increased the capability of soybean to grow successfully under salinity. The Trichoderma isolate concomitant with the high-vigour seed lot was considered the best in providing salt tolerance to soybean seedlings. Evidence found in the present work shows that salinity tolerance is promoted by root growth and leaf greenness, ROS scavenging by catalase and peroxidise, and the production of proline as a compatible osmolyte. These effects suggest that both metabolic performance and antioxidant capacity are increased in the presence of the salt-tolerant isolate of Trichoderma. This research merits attention as it provides new approaches to the introduction of high-quality seeds and the application of Trichoderma through seed biopriming in soybean plants for increased salinity resistance.
Acknowledgements
The authors wish to thank the Research and post-graduation Committee of University of Mohaghegh Ardabili for a research grant in support of this project.
Disclosure statement
No potential conflict of interest was reported by the authors.
References
- Arora DK, Elander RP, Mukherji KG. 1992. Handbook of applied mycology. New York (NY): Markel Dekker.
- Ashraf M, Foolad MR. 2007. Roles of glycine betaine and proline in improving plant abiotic stress resistance. Environ Exp Bot. 59:206–216. doi: 10.1016/j.envexpbot.2005.12.006
- Bailly C. 2004. Active oxygen species and antioxidants in seed biology. Seed Sci Res. 14(2):93–107. doi: 10.1079/SSR2004159
- Bailly C, Benamar A, Corbineau F, Côme D. 1998. Free radical scavenging as affected by accelerated ageing and subsequent priming in sunflower seeds. Physiol Plant. 104(4):646–652. doi: 10.1034/j.1399-3054.1998.1040418.x
- Bandeoglu E, Eyidogan F, Yucel M, Oktem HA. 2004. Antioxidant responses of shoots and roots of lentil to NaCl-salinity stress. Plant Growth Regul. 42:69–77. doi: 10.1023/B:GROW.0000014891.35427.7b
- Basra SMA, Ahmad N, Khan MM, Iqbal N, Cheema MA. 2003 Assessment of cottonseed deterioration during accelerated ageing. Seed Sci Technol. 31(3):531–540. doi: 10.15258/sst.2003.31.3.02
- Bates LS, Waldren RP, Teare ID. 1973. Rapid determination of free proline for water stress studies. Plant Soil. 39(1):205–207. doi: 10.1007/BF00018060
- Begum MM, Sariah M, Puteh AB, Zainal-Abidin MA, Rahman MA, Siddiqui Y. 2010. Field performance of bioprimed seeds to suppress Colletotrichum truncatum causing damping-off and seedling stand of soybean. Biol Control. 53:18–23. doi: 10.1016/j.biocontrol.2009.12.001
- Brotman Y, Landau U, Cuadros-Inostroza A, Takayuki T, Fernie AR, Chet I, Viterbo A, Willmitzer L, Howlett BJ. 2013. Trichoderma-plant root colonization: escaping early plant defense responses and activation of the antioxidant machinery for saline stress tolerance. PLOS Pathog. 9(3):1–15. doi: 10.1371/journal.ppat.1003221
- Chen K, Arora R. 2013. Priming memory invokes seed stress-tolerance. Environ Exp Bot. 94:33–45. doi: 10.1016/j.envexpbot.2012.03.005
- Dogan M. 2011. Antioxidative and proline potentials as a protective mechanism in soybean plants under salinity stress. African J Biotechnol. 10(32):5972–5978.
- Ellis RH, Roberts EH. 1981. The quantification of ageing and survival in orthodox seeds. Seed Sci Technol. 9:373–409.
- Elias SG, Copeland LO. 2001. Physiological and harvest maturity of canola in relation to seed quality. Crop Sci. 93:1054–1058.
- Fan XD, Wang JQ, Yang N, Dong YY, Liu L, Wang FW, Wang N, Chen H, Liu WC, Sun YP, et al. 2013. Gene expression profiling of soybean leaves and roots under salt, saline-alkali and drought stress by high-throughput Illumina sequencing. Gene. 512(2):392–402. doi: 10.1016/j.gene.2012.09.100
- Finch-Savage WE. 2000. Influence of seed quality on crop establishment, growth and yield. In: Basra AS, editor. Seed quality: basic mechanisms and agricultural implications. New York (NY): The Haworth Press; p. 361–384.
- Garg N. 2004. Variability in response of chickpea (Cicer arietinum L.) cultivars to salt stress in germination and early growth of the seedlings. Ind J Plant Physiol. 9(1):21–28.
- Ghassemi-Golezani K. 1992. Effects of seed quality on cereal yields [dissertation]. Reading: University of Reading.
- Ghassemi-Golezani K, Khomari S, Valizadeh M, Alyari H. 2008a. Changes in chlorophyll content and fluorescence of leaves of winter rapeseed affected by seedling vigor and cold acclimation duration. Int J Food Agric. Environ. 6(3&4):196–199.
- Ghassemi-Golezani K, Khomari S, Valizadeh M, Alyari H. 2008b. Effects of seed vigour and the duration of cold acclimation on freezing tolerance of winter oilseed rape. Seed Sci Technol. 36:767–775. doi: 10.15258/sst.2008.36.3.26
- Gill SS, Tuteja N. 2010. Reactive oxygen species and antioxidant machinery in abiotic stress tolerance in crop plants. Plant Physiol Biochem. 48(12):909–930. doi: 10.1016/j.plaphy.2010.08.016
- Gomes MP, Garcia QS. 2013. Reactive oxygen species and seed germination. Biologia. 68(3):351–357. doi: 10.2478/s11756-013-0161-y
- Hameed A, Afzal I, Iqbal N. 2010. Seed priming and salinity induced variations in wheat (Triticum aestivum L.) leaf protein profile. Seed Sci Technol. 38(1):236–241. doi: 10.15258/sst.2010.38.1.25
- Hamilton CE, Gundel PE, Helander M, Saikkonen K. 2012. Endophytic mediation of reactive oxygen species and antioxidant activity in plants: a review. Fung Divers. 54:1–10. doi: 10.1007/s13225-012-0158-9
- Harman GE. 2006. Overview of mechanisms and uses of Trichoderma spp. Phytopathol. 96:190–194. doi: 10.1094/PHYTO-96-0190
- Harman GE, Petzoldt R, Comis A, Chen J. 2004. Interactions between Trichoderma harzianum strain T22 and maize inbred line Mo17 and effects of this interaction on diseases caused by Pythium ultimum and Colletotrichum graminicola. Phytopathology. 94:147–153. doi: 10.1094/PHYTO.2004.94.2.147
- Huang M, Zhang R, Chen J, Cao F, Jiang L, Zou Y. 2017. Morphological and physiological traits of seeds and seedlings in two rice cultivars with contrasting early vigor. Plant Prod Sci. 20:95–101. doi: 10.1080/1343943X.2016.1229571
- Jamil M, Rehman S, Lee KJ, Kim JM, Kim HS, Rha ES. 2007. Salinity reduced growth PS2 photochemistry and chlorophyll content in radish. Sci Agric. 64(2):111–118. doi: 10.1590/S0103-90162007000200002
- Javadi A, Khomari S, Sofalian O. 2016. Seed vigor and boron and calcium nutrition influence oilseed rape germinability and seedling growth under salt stress. J Plant Nutr. 39(12):1688–1696. doi: 10.1080/01904167.2015.1093138
- Khajeh-Hosseini M, Powell AA, Bingham IJ. 2003. The interaction between salinity stress and seed vigour during germination of soybean seeds. Seed Sci Technol. 31(3):715–725. doi: 10.15258/sst.2003.31.3.20
- Kibinza S, Bazin J, Bailly C, Farrant JM, Corbineau F, El-Maarouf-Bouteau H. 2011. Catalase is a key enzyme in seed recovery from ageing during priming. Plant Sci. 181:309–315. doi: 10.1016/j.plantsci.2011.06.003
- Kong L, Huo H, Mao P. 2015. Antioxidant response and related gene expression in aged oat seed. Front Plant Sci. 6:158.
- MacAdam JW, Nelson CJ, Sharp RE. 1992. Peroxidase activity in the leaf elongation zone of tall fescue. I. Spatial distribution of ionically bound peroxidase activity in genotypes differing in length of the elongation zone. Plant Physiol. 99(3):872–878. doi: 10.1104/pp.99.3.872
- Mahajan S, Tuteja N. 2005. Cold, salinity and drought stresses: an overview. Arch Biochem Biophys. 444:139–158. doi: 10.1016/j.abb.2005.10.018
- Manchanda G, Garg N. 2008. Salinity and its effects on the functional biology of legumes. Acta Physiol Plant. 30:595–618. doi: 10.1007/s11738-008-0173-3
- Mannan MA, Karim MA, Khaliq QA, Haque MM, Mian MAK, Ahmed JU. 2009. Proline accumulation, water status and chlorophyll content in leaf in relation to salt tolerance in soybean. Indian J Plant Physiol. 14(2):130–134.
- McDonald MB. 1999. Seed deterioration: physiology, repair and assessment. Seed Sci Technol. 27(1):177–237.
- Ozturk L, Demir Y, Unlukara A, Karatas I, Kurunc A, Duzdemir O. 2012. Effects of long-term salt stress on antioxidant system, chlorophyll and proline contents in pea leaves. Rom Biotechnol Lett. 17(3):7227–7236.
- Parida AK, Das AB. 2005. Salt tolerance and salinity effects on plants: a review. Ecotox Environ Safe. 60(3):324–349. doi: 10.1016/j.ecoenv.2004.06.010
- Perry DA. 1984. Factors influencing the establishment of cereal crops. Asp Appl Biol. 7:65–83.
- Rao AS, Reddy KS. 2010. Nutrient management in soybean. In: Singh G, editor. The soybean: botany production and uses. London: CAB International; p. 161–190.
- Rawat L, Singh Y, Shukla N, Kumar J. 2011. Alleviation of the adverse effects of salinity stress in wheat (Triticum aestivum L.) by seed biopriming with salinity tolerant isolates of Trichoderma harzianum. Plant Soil. 347:387–400. doi: 10.1007/s11104-011-0858-z
- Reddy PP. 2013. Recent advances in crop protection. New Delhi: Springer.
- Rehman S, Harris PJC, Bourne WF. 1999. Effect of artificial ageing on the germination, ion leakage and salinity tolerance of Acacia tortilis and A. coriacea seeds. Seed Sci Technol. 27(1):141–149.
- Roberts EH, Osei-Bonsu K. 1988. Seed and seedling vigour. In: Summerfield RJ, editor. World crops: cool season food legumes. Dordrecht: Kluwer Academic Publishers; p. 897–910.
- Rifai MA. 1969. A revision of the genus Trichoderma. Mycol Pap. 116:1–56.
- SAS Institute Inc. 1998. SAS stat view reference. Cary (NC): SAS Institute.
- Shoresh M, Harman GE, Mastouri F. 2010. Induced systemic resistance and plant responses to fungal bio-control agents. Annu Rev Phytopathol. 48:21–43. doi: 10.1146/annurev-phyto-073009-114450
- Shukla N, Awashi RP, Rawat L, Kumar J. 2015. Seed biopriming with drought tolerant isolates of Trichoderma harzianum promote growth and drought tolerance in Triticum aestivum. Ann Appl Biol. 166(2):171–182. doi: 10.1111/aab.12160
- Singh G, Shivakumar BG. 2010. The role of soybean in agriculture. In: Singh G, editor. The soybean: botany production and uses. London: CAB International; p. 24–47.
- Sudhir P, Murthy SDS. 2004. Effects of salt stress on basic processes of photosynthesis. Photosynthetica. 42(4):481–486. doi: 10.1007/S11099-005-0001-6
- Verma SS, Verma U, Tomer RPS. 2003. Studies on seed quality parameters in deteriorating seeds in Brassica (Brassica campestris L.). Seed Sci Technol. 31(2):389–396. doi: 10.15258/sst.2003.31.2.15
- Wahid A, Rasul E, Rao AR. 1999. Germination of seeds and propagules under salt stress. In: Pessarakli M, editor. Handbook of plant and crop stress. New York (NY): Marcel Dekker; p. 153–167.
- Walters C, Ballesteros D, Vertucci VA. 2010. Structural mechanics of seed deterioration: standing the test of time. Plant Sci. 179:565–573. doi: 10.1016/j.plantsci.2010.06.016
- Yasar F, Ellialtioglu S, Yildiz K. 2008. Effect of salt stress on antioxidant defense systems, lipid peroxidation, and chlorophyll content in green bean. Russian J Plant Physiol. 55(6):782–786. doi: 10.1134/S1021443708060071