ABSTRACT
Leptospermum scoparium J. R. et G. Forst. (Myrtaceae), called mānuka or kahikātoa by Māori, shows distinct foliage chemotypes in different regions of Aotearoa New Zealand, including one with rare antimicrobial triketones. To extend knowledge of mānuka chemotypes geographically and historically, we have developed a miniaturised method for analyses of herbarium samples needing just a few small leaves analysed by headspace solid phase microextraction gas chromatography (HS-SPME-GC). This method was validated on herbarium samples of known chemotypes. Geographical knowledge was extended by analysing herbarium samples collected on New Zealand's offshore islands, ranging from the Manawa/Three Kings in the North to Rakiura/Stewart Island in the South. The chemotypes were generally similar to the nearest populations on the main North or South islands. Historically, volatiles were identified from herbarium samples collected by the first botanists from Europe in 1769, showing that the oil glands in mānuka leaves act as ‘chemical time capsules’.
Introduction
Leptospermum scoparium J. R. et G. Forst. (Myrtaceae) (Allan Citation1961; Stephens et al. Citation2005) is mānuka or kahikātoa to Māori, and has many rongoā (medicinal) and other uses (Riley Citation1994). Currently mānuka is best known for the antimicrobial honey produced from its nectar (Jull et al. Citation2008; Smallfield et al. Citation2018), but mānuka oil, steam distilled from foliage, also has antimicrobial properties (Porter and Wilkins Citation1998; Stephens et al. Citation2005) and has been reported as virucidal (Reichling et al. Citation2005). The active ingredients in mānuka oil are rare triketones, mainly leptospermone (see ) (van Klink et al. Citation2005), but these have only been found at high concentrations in oils from Tairāwhiti, the East Cape of the North Island of Aotearoa New Zealand () (Perry et al. Citation1997; Douglas et al. Citation2004).
Figure 2. Map of Aotearoa New Zealand showing the islands included, selected original chemotype sites, other herbarium sample collection sites and possible collection sites of the Endeavour botanists.
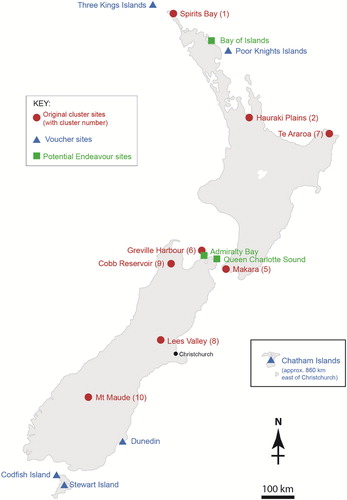
Mānuka is one of New Zealand's most widespread native shrubs (Stephens et al. Citation2005; Dawson and Lucas Citation2011) and analyses of foliage oils from most regions of the main North and South Islands () revealed other distinctive chemotypes with potential value, e.g. a single population rich in the perfume and flavour compound geranyl acetate (Douglas et al. Citation2004). Analyses of herbarium samples would allow us to extend our knowledge of mānuka chemotypes geographically and historically. Geographically, existing herbarium samples could give first indications of potential new chemotypes from the remote smaller islands around New Zealand (), as well as minimising costly fieldwork. Historically, early herbarium samples could give us indications of changes in the regional ranges of chemotypes, reflecting biogeography and human influences (Stephens et al. Citation2005). Recently, the high value of mānuka honey has led to commercial plantings of mānuka transferred between regions. Historical knowledge of the natural chemical diversity and regional chemotypes of mānuka will complement analyses of genetic diversity (Chagné et al. Citation2017). L. scoparium in New Zealand is extremely variable in growth form and habit, and this variation has not been taxonomically addressed since Kirk (Citation1899) and Cockayne (Citation1917).
Previous studies using chemical analyses of herbarium specimens included bracken samples up to 50 years old to map the distribution of the natural carcinogen ptaquiloside across Europe (Rasmussen and Pedersen Citation2017). We used herbarium specimens of similar ages to study the distribution of phenylanthraquinones in Bulbinella species across New Zealand and the sub-Antarctic Islands (Richardson et al. Citation2017). The natural product classes in these studies were non-volatile, whereas we wanted to study the volatiles in mānuka. It was possible that these volatiles could be lost on storage, as we found for volatiles from herbarium samples of Gingidia in the family Apiaceae (Sansom et al. Citation2013).
We now describe a rapid head space solid phase microextraction method (HS-SPME) (Arthur and Pawliszyn Citation1990) to sample mānuka foliage volatiles for gas chromatography (GC) analyses, applicable to very small samples from herbarium specimens with a wide range of ages. There have been a few reports of the use of HS-SPME-GC to study volatiles of medicinal plants (Belliardo et al. Citation2006), including a comparison of sampling methods to screen volatiles in another Myrtaceae Myrtus communis (Moradi et al. Citation2012). We report the volatiles from a broad geographic and historical spread of mānuka herbarium samples, using just a few mānuka leaves rather than the 150–750 g of foliage steam distilled in a previous study (Douglas et al. Citation2004).
Materials and methods
Plant material
Up to 20 leaves were collected from each of 51 L. scoparium voucher specimens from: the Plant & Food Research collection (Douglas et al. Citation2004); the University of Otago Herbarium (OTA), Dunedin; the Allan Herbarium (CHR), Lincoln; and the Auckland War Memorial Museum (AK). Fresh foliage was collected from three plants of the triketone chemotype growing at Plant & Food Research, Palmerston North, in March 2013. Details of all samples are given in Supporting Information Table S1.
Analyses
Sample preparation: Up to 20 leaves from air-dried foliage were ground in liquid N2 and 10 mg subsampled into a 20 mL SPME vial (Supelco) silanised by a standard method (Knapp Citation1979).
HS-SPME: A SPME fibre (PDMS, 100 µm, Supelco) was conditioned at 250°C for 30 min before first use and for 15 min at the beginning of each day. Each foliage sample was equilibrated in a vial at 50°C for 10 min followed by extraction onto a SPME fibre for 20 min at 50°C, then immediate fibre desorption in the GC inlet (see below).
GC: Analyses were performed on an Agilent 7890A gas chromatograph with a CTC Analytics PAL system autosampler and an Agilent 5975C inert XL MSD with triple axis detector, under the control of Enhanced ChemStation software. SPME fibre desorption was 1 min in the injector at 260°C with the flow split 20:1 between waste and a 30 m Agilent HP5-ms column with a 0.25 mm ID and 0.25 µm film. The carrier gas was H2 (1.5 mL/min) and column heated from 50°C to 250°C at 5°C/min. The outlet flow was split using deactivated silica columns between MSD (0.5 m × 0.1 mm ID) and FID (2 m × 0.18 mm ID). Detection was by mass spectrometry (the MS transfer line was held at 270°C, the MS source was held at 230°C and the MS quad held at 150°C) over the mass range 35–300 Da for identification. Flame ionisation detection (300°C) was used for quantitation. Peak identification details are given in Supporting Information Table S2.
LC-MS: The LC-MS system consisted of a Thermo Electron Corporation (San Jose, CA, USA) Finnigan Surveyor MS pump, Thermo Accela Open Auto sampler (PAL HTC-xt with DLW), Finnigan Surveyor PDA plus detector and a ThermaSphere TS-130 column heater (Phenomenex, Torrance, CA, USA). A 2-μL aliquot of each prepared extract was separated with a mobile phase flowing at 200 µL/min consisting of 0.1% formic acid in water (A) and 0.1% formic acid in acetonitrile (B) by reverse phase chromatography (C18 SecurityGuardTM cartridge 4 × 2 mm, 10 µm and Luna (II) C18, 5 µm, 100 Å, 150 × 3 mm column, Phenomenex, Torrance, CA, USA) maintained at 30°C with a flow rate of 200 µL/min. A gradient was applied: time (min) A%/B% starting at (0) 90/10, to (40) 0/100, (45) 0/100, (47) 90/100, (52) 90/10. The eluent was scanned by PDA (200–600 nm) and API-MS (LTQ, 2D linear ion-trap, Thermo-Finnigan, San Jose, CA, USA) with electrospray ionisation (ESI) in the negative and positive mode. Data were acquired for parent masses from m/z 150–1500 amu with MS4. Data were processed with the aid of Xcalibar®2.1 (Thermo Electron Corporation). A reference sample of grandiflorone had a retention time of 38.8 min with [M-H] at m/z 313 in negative ESI mode. Various tentatively identified (see Haberlein and Tschiersch Citation1998) methylated flavonoids (e.g. m/z 267, m/z 283, m/z 285, m/z 295, m/z 297) were observed from the negative ESI LC-MS traces.
Statistical analysis: Principal Components Analysis was based on a Sums of Squares and Products in Genstat (version 14) software (VSNi, Hemel Hempstead, England).
Results and discussion
Analytical method for herbarium samples
To be able to use just a few small leaves from mānuka herbarium samples for analyses, we needed a miniaturised method rather than the 150–750 g of foliage previously steam distilled (Douglas et al. Citation2004). We chose HS-SPME-GC for its potential sensitivity, simplicity and speed (Ouyang and Jiang Citation2017). Conditions were developed using a model mix of mānuka leaf components with a range of volatilities: from monoterpenes and sesquiterpenes to volatile triketones (example structures in , a fuller account of the method development is given in Supporting Information, Appendix S1). A polydimethylsiloxane (PDMS) fibre was most suitable of the different types available (IOFI Working Group on Methods of Analysis Citation2010), and 20 min extraction at 50°C gave a balance between recovery and speed. Recovery of leptospermone, with its hydrogen bonding keto–enol group () (van Klink et al. Citation1999), was erratic in initial analyses using SPME vials as purchased, but was acceptably consistent when the vials were silanised. Bartelt (Citation1997) also noted erratic recovery by SPME from non silanised glass containers, especially for compounds of higher molecular weight or polarity. The selected HS-SPME conditions favoured compounds of medium volatility (sesquiterpenes), so the mānuka leaf volatile profiles by this method differed quantitatively from the profiles of steam distilled mānuka oils analysed by direct GC injection (Douglas et al. Citation2004).
To test the utility of this HS-SPME-GC method to distinguish the currently known mānuka chemotypes on stored herbarium samples, we analysed a few leaves each of reference voucher specimens from our earlier study (Douglas et al. Citation2004). Replicate HS-SPME-GC analyses of a composite mānuka leaf sample (mixed from different chemotypes) gave repeatable peak areas (standard deviations 4–14% of peaks as % of total peak area, three analyses tightly clustered in Principal Components Analysis, PCA, ). Herbarium samples from three individual shrubs at each of eight sites were chosen to represent eight of the ten chemotypes we previously proposed (see for collection sites, central North Island chemotypes 3 and 4 not represented because they only differ from neighbouring chemotype 2 in relative proportions of some sesquiterpene hydrocarbons) (Douglas et al. Citation2004). Relative concentrations of the 19 main mānuka leaf volatiles are reported in .
Figure 3. Principal Components Analysis of main mānuka volatiles from known chemotypes (Douglas et al. Citation2004) by HS-SPME-GC-FID. Samples are labeled with their chemotype number, and C = composite check sample. PC1 (41% of total variance) with major contributions from β-caryophyllene +0.61 and trans-calamenene −0.56; PC2 (32%) with major contributions from β-selinene −0.63 and β-caryophyllene +0.60.
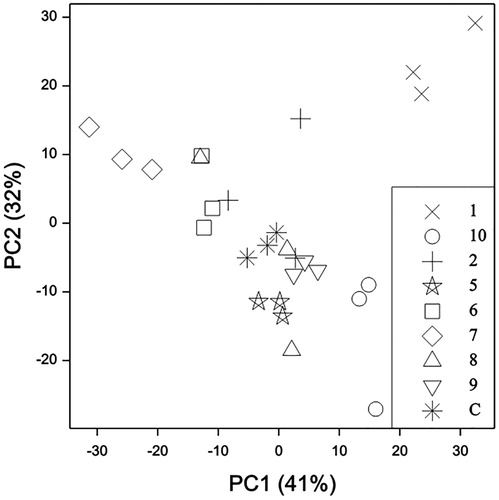
Table 1. Main mānuka volatiles (% FID) from known chemotypes by HS-SPME-GC of herbarium samples.
The marker compounds for each mānuka chemotype (Douglas et al. Citation2004) were found at relatively high levels in the HS-SPME-GC analyses of the representative herbarium samples (). In particular, the antimicrobial triketones flavesone and leptospermone were highest from the East Cape herbarium samples (chemotype 7, ). These samples were also well distinguished on the first Principal Component (PC1, ) on the basis of the high trans-calamenene and low β-caryophyllene signals, as previously noted (Douglas et al. Citation2004). The high monoterpene chemotype 1 was well separated from the other samples by HS-SPME-GC analyses of the representative herbarium samples (). These samples did stand out for their α-pinene signals being much higher than any of the other samples, but due to the sampling bias noted above, α-pinene was only 1% of the total volatiles by HS-SPME-GC (), whereas α-pinene was around 20% of the total volatiles in steam-distilled essential oils from this chemotype analysed by direct GC injection (Douglas et al. Citation2004). Therefore this HS-SPME-GC method applied to small herbarium samples does distinguish mānuka chemotypes, but does not predict the actual composition that could be achieved by commercial steam-distillation. A plot (not presented) of PC3 (11%) and PC4 (6%) also separated out samples from chemotype 5 and chemotype 6, predominantly based on their geranyl acetate, trans-calamenene and aromadendrene contents.
Extended geographic study of mānuka chemotypes
With this HS-SPME-GC method validated on herbarium samples of known chemotypes from the main North and South Islands, we were able to extend the geographic study of mānuka chemotypes by analysing herbarium voucher samples from New Zealand's main offshore islands (except the sub-Antarctic islands and Kermadec Island, where mānuka is not present (Allan Citation1961)). These were collected at various times by various botanists from the following island groups: Manawatāwhi/Three Kings (3 samples), Tawhiti Rahi/Poor Knights (1), Rekohu/Wharekauri/Chatham (7), Whenua Hou/Codfish (1) and Rakiura/Stewart (5) (Table S1 and ). The analysis results are detailed in .
Table 2. Main mānuka volatiles (% FID) in herbarium samples from New Zealand offshore islands by HS-SPME-GC
All of these mānuka samples showed the same main GC peaks as previously identified. The samples from Three Kings and Poor Knights island groups gave 4–15% monoterpenes in their HS-SPME volatiles (), aligning them with chemotype 1 (). These islands are off the coast of Northland () where this monoterpene chemotype is dominant (Douglas et al. Citation2004). Samples from the Chatham Islands gave mainly sesquiterpenes similar to those in chemotype 10, the general South Island chemotype (). The Stewart and Codfish Island samples were also similar to chemotypes 8, 9, or 10, the South Island chemotypes ().
None of these island samples showed high triketone concentrations, so we have not found any new sources for antimicrobial mānuka leaf oil production. In our previous work mānuka growing on Rangitoto Ki Te Tonga/D’Urville Island had the highest concentration of triketones away from the East Cape, which we speculated may have been due to transfer by Māori traders (Douglas et al. Citation2004). Northern South Island occurrences of Arthropodium cirratum are now known to have been translocated by iwi from the East Cape (Shepherd et al. Citation2018).
Extended historical study of mānuka chemotypes
During the work described above we noted that the total volatiles contents of these stored herbarium samples were not obviously less from older samples. For example, the mānuka foliage collected in 1954 from the Chatham Islands had total volatiles within the range found for stored voucher samples from our 1999–2001 collections (). However, several other older samples were below the low end of this range, so we analysed freshly dried samples (East Cape triketone chemotype grown at Palmerston North, ) and some 1950–1963 voucher samples from the Dunedin area (full analytical data in Supporting Information Table S3). Both of these sample sets showed similar total volatiles () suggesting that much older mānuka samples might contain enough volatiles for analysis by our miniaturised method.
Figure 4. Total volatiles by HS-SPME-GC from mānuka herbarium samples by collection year. The line shows the range for 24 samples collected between 1999 and 2001 (Douglas et al. Citation2004).
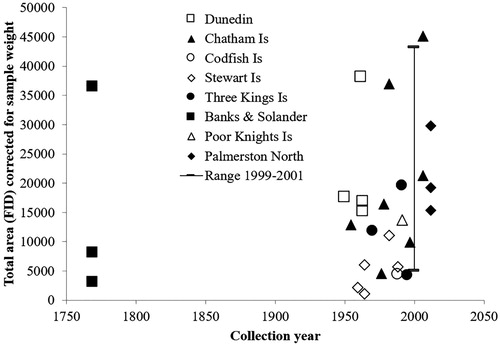
Leaves from mānuka samples collected during the 1769–1770 exploration of Cook's Endeavour around New Zealand were generously provided from AK. This was only the second visit of Europeans to New Zealand, and the first with scientific sampling of the flora (Adams Citation1986). Banks and Solander collected mānuka from all eight sites where they landed in New Zealand (Cook Citation1893) (), but the individual vouchers were not assigned to a specific site (Ewen Cameron personal communication). We were delighted to find that the total volatiles from these eighteenth century samples were similar to the totals from recent samples (). The main compounds found were the same as those in recent mānuka samples (). The major volatiles from BS1 were methyl cinnamate, β-selinene, α-selinene and trans-calamenene (), suggesting that this plant belongs to South Island chemotype 8, 9 or 10. The major peak in the chromatogram of BS2 was α-amorphene, similar to the samples from Three Kings and Poor Knights Islands (), so this may have been collected from the Bay of Islands () as this was the only landing site in this region (Cook Citation1893). Sample BS3 contained β-selinene, α-selinene and trans-calamenene as the major peaks, and geranyl acetate, flavesone and leptospermone as minor peaks, suggesting that this plant belongs to chemotype 6. Therefore, it is likely that BS3 was collected from the Admiralty Bay or Queen Charlotte Sound landings (Cook Citation1893), as chemotype 6 is centred in Greville Harbour in the Marlborough Sounds (). This method could be used to suggest the provenance of other herbarium samples of uncertain origin.
Table 3. Main mānuka volatiles (% FID) in herbarium samples collected by Banks and Solander on the Endeavour Journey (1769–1770) by HS-SPME-GC.
The three Cook herbarium samples were also subjected to liquid chromatography combined with mass spectrometry (LC-MS) analyses to determine whether the less volatile triketone grandiflorone and/or the rare methylated flavonoids had retained their integrity upon storage. These analyses were not quantitative, but LC-MS peaks were observed that had matching retention times and mass spectra of that of grandiflorone in all three samples. In addition, all three samples had other peaks indicative of the methylated flavonoids typical of mānuka (Haberlein and Tschiersch Citation1998), which is not surprising since these have also been reported from the leaf oil glands (Killeen et al. Citation2015).
Conclusions
This discovery that volatiles are stably stored in mānuka (Myrtaceae) leaves for centuries contrasts with our finding that volatiles are lost rapidly from herbarium samples of Gingidia in the family Apiaceae (Sansom et al. Citation2013). The major monoterpene, β-phellandrene, was not detectable after one year's storage of Gingidia foliage at room temperature. However, in L. scoparium and other Leptospermum species ‘ … spherical secretory cavities (schizogenous oil glands) occur in the leaves of all species’ (Johnson Citation1980) and we have shown that the triketones are stored within these oil glands (Killeen et al. Citation2015). We suspect that the mono- and sesquiterpenes and phenyl propanoid volatiles are also accumulated in these sealed off glands, as they are in other Myrtaceae (Goodger et al. Citation2018). List et al. (Citation1995) examined oil cell (gland) development in another commercial Myrtaceae species, Melaleuca alternifolia, and reported ‘ … the mature oil gland must be incapable of oil synthesis or catabolism. Intercellular deposits between cells in the lining function to apoplastically isolate the gland cavity and its oil contents from the remainder of the leaf … ’. We found that mānuka leaves had to be finely ground before HS-SPME-GC analyses because intact leaves gave insufficient release of volatiles, presumably because of these robust oil gland linings result in ‘chemical time capsules’, preserving secondary metabolite signatures.
This stability of secondary metabolites in leaves and the sensitive analytical technique described above will allow even more detailed sampling in studies of the biogeography and history of mānuka. For example, we note that mānuka grown from East Cape seed at Palmerston North () retained the triketone chemotype (Supporting Information Table S3), as we had previously found for East Cape mānuka seed grown on the South Island (Perry et al. Citation1997).
Supporting Information
Download MS Word (43.3 KB)Acknowledgements
We thank: K. Bhati for preliminary HS-SPME-GC work; N. Joyce for assistance with LC-MS; J. Lord for OTA samples, I. Schonberger and P. Heenan for CHR samples; E. Cameron for AK samples; E. Morgan for fresh foliage from Palmerston North; and B. Smallfield and P. de Lange for contacts and comments.
Disclosure statement
No potential conflict of interest was reported by the authors.
ORCID
Catherine E. Sansom http://orcid.org/0000-0002-0034-524X
John W. van Klink http://orcid.org/0000-0002-0843-567X
Nigel B. Perry http://orcid.org/0000-0003-3196-3945
Additional information
Funding
Notes
* Dedicated to the memory of our great friend and scientist, Malcolm Douglas (1938–2018), who loved exploring and collecting across Aotearoa New Zealand, e.g. (Douglas et al. Citation2004).
References
- Adams B. 1986. The flowering of the Pacific. Sydney: William Collins Pty Ltd.
- Allan HH. 1961. Flora of New Zealand. Indigenous Tracheophyta. Psilopsida, Lycopsida, Filicopsida, Gymnospermae, Dicotyledones. Wellington, DSIR.
- Arthur CL, Pawliszyn J. 1990. Solid phase microextraction with thermal desorption using fused silica optical fibers. Analytical Chemistry. 62:2145–2148. doi: 10.1021/ac00218a019
- Bartelt RJ. 1997. Calibration of a commercial solid-phase microextraction device for measuring headspace concentrations of organic volatiles. Analytical Chemistry. 69:364–372. doi: 10.1021/ac960820n
- Belliardo F, Bicchi C, Cordero C, Liberto E, Rubiolo P, Sgorbini B. 2006. Headspace-solid-phase-microextraction in the analysis of the volatile fraction of aromatic and medicinal plants. Journal of Chromatographic Science. 44:416–429. doi: 10.1093/chromsci/44.7.416
- Chagné D, Thrimawithana A, Bowatte D, Hilario E, Crowhurst R, Smallfield B, Boase M, Lewis D, de Lange P, Schwinn K. 2017. Simple sequence repeat (SSR) markers for New Zealand mānuka (Leptospermum scoparium) and transferability to kānuka (Kunzea spp.). New Zealand Journal of Crop and Horticultural Science. 45:216–222. doi: 10.1080/01140671.2017.1298631
- Cockayne L. 1917[1916]. Notes on New Zealand floristic botany, including descriptions of new species &c. (no. 2). Transactions and Proceedings of the New Zealand Institute. 49:56–65.
- Cook J. 1893. Captain Cook’s journal. First Voyage London, Elliot Stock, 62 Paternoster Row. See Project Gutenberg http://www.gutenberg.org/files/8106/8106-h/8106-h.htm.
- Dawson J, Lucas R. 2011. New Zealand’s native trees. Nelson: Craig Potton.
- Douglas MH, van Klink JW, Smallfield BM, Perry NB, Anderson RE, Johnstone P, Weavers RT. 2004. Essential oils from New Zealand manuka: triketone and other chemotypes of Leptospermum scoparium. Phytochemistry. 65:1255–1264. doi: 10.1016/j.phytochem.2004.03.019
- Goodger JQD, Senaratne SL, Nicolle D, Woodrow IE, Niinemets Ü. 2018. Differential metabolic specialization of foliar oil glands in Eucalyptus brevistylis Brooker (Myrtaceae). Tree Physiology. 38:1451–1460. doi: 10.1093/treephys/tpy077
- Haberlein H, Tschiersch K-P. 1998. On the occurrence of methylated and methoxylated flavonoids in Leptospermum scoparium. Biochemical Systematics and Ecology. 26:97–103. doi: 10.1016/S0305-1978(97)00084-7
- IOFI Working Group on Methods of Analysis. 2010. Guidelines for solid-phase micro-extraction (SPME) of volatile flavour compounds for gas-chromatographic analysis, from the working group on methods of Analysis of the International Organization of the Flavor Industry (IOFI). Flavour and Fragrance Journal. 25:404–406. doi: 10.1002/ffj.1991
- Johnson CT. 1980. The leaf anatomy of Leptospermum Forst. (Myrtaceae). Australian Journal of Botany. 28:77–104. doi: 10.1071/BT9800077
- Jull A, Walker N, Parag V, Molan P, Rodgers A. 2008. Randomized clinical trial of honey-impregnated dressings for venous leg ulcers. British Journal of Surgery. 95:175–182. doi: 10.1002/bjs.6059
- Killeen DP, van Klink JW, Smallfield BM, Gordon KC, Perry NB. 2015. Herbicidal β-triketones are compartmentalized in leaves of Leptospermum species: localization by Raman microscopy and rapid screening. New Phytologist. 205:339–349. doi: 10.1111/nph.12970
- Kirk T. 1899. The students’ flora of New Zealand. Wellington: Government Printer.
- Knapp DR. 1979. Handbook of analytical derivatisation reactions. New York: John Wiley & Sons.
- List S, Brown PH, Walsh KB. 1995. Functional anatomy of the oil glands of Melaleuca alternifolia (Myrtaceae). Australian Journal of Botany. 43:629–641. doi: 10.1071/BT9950629
- Moradi M, Kaykhaii M, Ghiasvand AR, Shadabi S, Salehinia A. 2012. Comparison of headspace solid-phase microextraction, headspace single-drop microextraction and hydrodistillation for chemical screening of volatiles in Myrtus communis L. Phytochemical Analysis. 23:379–386. doi: 10.1002/pca.1368
- Ouyang G, Jiang R. 2017. Solid phase microextraction: recent developments and applications. Berlin (Germany): Springer.
- Perry NB, Brennan NJ, van Klink JW, Harris W, Douglas MH, McGimpsey JA, Smallfield BM, Anderson RE. 1997. Essential oils from New Zealand manuka and kanuka: chemotaxonomy of Leptospermum. Phytochemistry. 44:1485–1494. doi: 10.1016/S0031-9422(96)00743-1
- Porter NG, Wilkins AL. 1998. Chemical, physical and antimicrobial properties of essential oils of Leptospermum scoparium and Kunzea ericoides. Phytochemistry. 50:407–415. doi: 10.1016/S0031-9422(98)00548-2
- Rasmussen LH, Pedersen HÆ. 2017. Screening for ptaquiloside in ferns: using herbarium specimens for qualitative mapping purposes. Phytochemical Analysis. 28:575–583. doi: 10.1002/pca.2707
- Reichling J, Koch C, Stahl-Biskup E, Sojka C, Schnitzler P. 2005. Virucidal activity of a b-triketone-rich essential oil of Leptospermum scoparium (Manuka oil) against HSV-1 and HSV-2 in cell culture. Planta Medica. 71:1123–1127. doi: 10.1055/s-2005-873175
- Richardson ATB, Lord JM, Perry NB. 2017. Phenylanthraquinones and flavone-C-glucosides from the disjunct Bulbinella in New Zealand. Phytochemistry. 134:64–70. doi: 10.1016/j.phytochem.2016.11.014
- Riley M. 1994. Maori Healing and Herbal. Paraparaumu: Viking Sevenseas N.Z. Ltd.
- Sansom CE, Heenan PB, Perry NB, Smallfield BM, van Klink JW. 2013. Chemosystematic analysis of volatiles from New Zealand and Australian Gingidia species. Chemistry & Biodiversity. 10:2226–2234. doi: 10.1002/cbdv.201300203
- Shepherd LD, Bulgarella M, de Lange PJ. 2018. Genetic structuring of the coastal herb Arthropodium cirratum (Asparagaceae) is shaped by low gene flow, hybridization and prehistoric translocation. PLoS ONE. 13:e0204943. doi: 10.1371/journal.pone.0204943
- Smallfield BM, Joyce NI, van Klink JW. 2018. Development and compositional changes in Leptospermum scoparium nectar and the relevance to mānuka honey bioactives and markers. New Zealand Journal of Botany. 56:183–197. doi: 10.1080/0028825X.2018.1446450
- Stephens JMC, Molan PC, Clarkson BD. 2005. A review of Leptospermum scoparium (Myrtaceae) in New Zealand. New Zealand Journal of Botany. 43:431–449. doi: 10.1080/0028825X.2005.9512966
- van Klink JW, Brophy JJ, Perry NB, Weavers RT. 1999. . b-Triketones from Myrtaceae: Isoleptospermone from Leptospermum scoparium and papuanone from Corymbia dallachiana. Journal of Natural Products. 62:487–489. doi: 10.1021/np980350n
- van Klink JW, Larsen L, Perry NB, Weavers RT, Cook GM, Bremer PJ, MacKenzie AD, Kirikae T. 2005. Triketones active against antibiotic-resistant bacteria: synthesis, structure-activity relationships and mode of action. Bioorganic & Medicinal Chemistry. 13:6651–6662. doi: 10.1016/j.bmc.2005.07.045