ABSTRACT
Leptospermum scoparium (‘Mānuka’) is native to New Zealand and South Eastern Australia. Throughout this range the species typically exists as a diploid. Only a single tetraploid accession has ever been reported and all the polyploid cultivars currently available are derivatives of that plant. Polyploidy in other species is known to influence vegetative and floral form, phenology and fertility. Floral diameter was therefore measured for the tetraploid L. scoparium ‘Keatleyi’, the triploid L. scoparium ‘Martinii’ and three diploid seedlings. The floral diameters of the tetraploid and triploid were significantly greater than those from diploid plants. However, the diploid flowers were also significantly different from each other, indicating that factors other than polyploidy influence this trait. Additionally, given the very limited number of polyploid clones available, methods were tested to generate more tetraploid plants for future study. Colchicine was effective at producing tetraploid seedlings with 6 mg/ml, the highest concentration tested, yielding the largest number of tetraploids. This method is now being used to generate tetraploids from a range of L. scoparium material.
Introduction
Leptospermum scoparium (‘Mānuka’) is endemic to New Zealand and South Eastern Australia where it occurs in a diverse range of habitats (Allan Citation1961; Thompson Citation1989). It is a species noted for its phenotypic diversity (Allan Citation1961; Dawson Citation1997a, Citation1997b; Perry et al. Citation1997; Porter and Wilkins Citation1999; Douglas et al. Citation2004; Stephens et al. Citation2005; Williams et al. Citation2014) and also for its large number of reported uses. Leptospermum scoparium has been used as an herbal tea (Brooker et al. Citation1987), a medicine (Crowe Citation1981; Brooker et al. Citation1987), as a cut flower (Zieslin and Gottesman Citation1983, Citation1986; Bicknell Citation1995; Burge et al. Citation1996), an ornamental shrub (Lammerts Citation1945; Dawson Citation1997a, Citation1997b), for the production of essential oils (Perry et al. Citation1997; Porter et al. Citation1998; Christoph et al. Citation1999; Douglas et al. Citation2004), a nursery species for the re-establishment of native forest, a ground-durable timber (Stephens et al. Citation2005), and for firewood, charcoal and for smoking fish. It is also the source of nectar for a highly valued honey (Butz Huryn Citation1995).
The most recent, comprehensive revision of the genus Leptospermum listed 79 species (Thompson Citation1989), with a further eight described since that time (Dawson Citation1990) raising the number of described species to 87. Most are endemic to Australia with outliers in South East Asia, New Guinea and New Zealand. Of the Leptospermum species studied for ploidy, the majority have been recorded as diploid with a somatic chromosome number of 2n = 2x = 22 (Smith-White Citation1948; Dawson Citation1990). Smith-White (Citation1948) reported on the cytology of 20 Leptospermum species from Australia, noting that three (L. minutifolium, L. myrtifolium and L. parvifolium) were tetraploid (2n = 4x = 44), whilst the rest were diploid. Dawson (Citation1990) reported that Leptospermum scoparium is typically a diploid species; however, the cultivars L. scoparium ‘Martinii’ and L. scoparium ‘Helene Strybing’ and L. scoparium ‘Lambethii’ are triploids and the cultivar L. scoparium ‘Keatleyi’ is tetraploid (). Variety L. scoparium ‘Keatleyi’ originated from a single plant discovered in Northland, New Zealand by Captain Keatley in 1917 (Dawson Citation1990, Citation1997b; Bicknell et al. Citation2018). Leptospermum scoparium ‘Keatleyi’ has pink flushed flowers and lanceolate leaves, characters typical of L. scoparium var. incanum, the predominant variety occurring in Northland (Allan Citation1961). Captain Keatley passed material on to the nurseryman Fred Walker of Whanganui, NZ, who successfully propagated it and disseminated plants amongst nurserymen throughout New Zealand and beyond. The three triploid cultivars; L. scoparium ‘Martinii’, L. scoparium ‘Lambethii’ and L. scoparium ‘Helene Strybing’ are all reported to be seedlings of L. scoparium ‘Keatleyi’ (Dawson Citation1997a). Each arose from a L. scoparium ‘Keatleyi’ plant in a different location; L. scoparium ‘Martinii’ in Whanganui, NZ; L. scoparium ‘Lambethii’ from a collection in Australia and L. scoparium ‘Helene Strybing’ from a plant in the Strybing arboretum, USA. Leptospermum scoparium ‘Martinii’ is still readily available in New Zealand, where it is popular for its large pink flowers and floriferous nature. We recently reported that most of the material being sold as L. scoparium ‘Keatleyi’ in New Zealand is incorrectly labelled as it is triploid rather than tetraploid (Bicknell et al. Citation2018). The origin of this material is unclear but the plants resemble L. scoparium ‘Martinii’ in form and it is possible that the two varieties were mixed at some stage. Fortunately an old plant of L. scoparium ‘Keatleyi’ was identified in an Auckland garden and this plant is now being multiplied for re-release (Bicknell et al. Citation2018).
Figure 1. Flowering shoots of the diploid variety ‘Plant 1’ (left), triploid variety Leptospermum scoparium ‘Martinii’ (centre) and the tetraploid variety L. scoparium ‘Keatleyi’ (right).
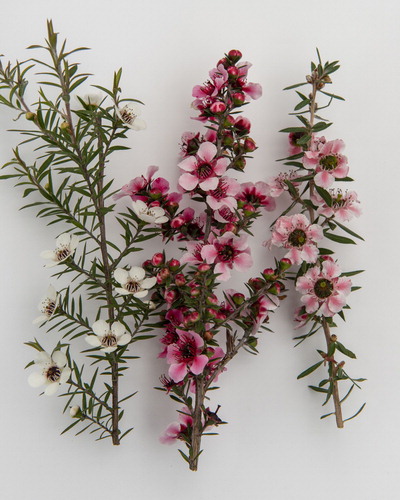
As part of a wider programme studying genetic variation in L. scoparium, we are attempting to quantify the influence of ploidy on plant form, floral organ size, nectar production, flower display time, self-fertility and nectar composition. A preliminary study was conducted, therefore, measuring flower diameter in the known triploid cultivar L. scoparium ‘Martinii’ and the tetraploid cultivar L. scoparium ‘Keatleyi’ along with three diploid accessions derived from Northland populations of L. scoparium var. incanum. Unfortunately, as only a single tetraploid variety of L. scoparium is currently available and all known triploids are derivatives of that plant, studies such as this are currently limited to material confounded by co-ancestry. This makes it difficult to separate genotypic and ploidy effects when studying trait segregation and expression. Consequently, techniques for producing more tetraploids were explored and are described in this paper.
Materials and methods
Measurement of floral diameter
90 seedlings of L. scoparium var. incanum were obtained from commercial sources and field-grown for 4 years in a sheltered horticultural site at Palmerston North, New Zealand. From this population, three plants were selected: one with small, one with intermediate and one with large flowers (Plants 1, 2 and 3). All were determined to be diploids using flow cytometry.
A plant of L. scoparium ‘Martinii’ was obtained from a commercial source and a plant of L. scoparium ‘Keatleyi’ was obtained from a recently rediscovered plant in an Auckland garden (Bicknell et al. Citation2018). The ploidy of L. scoparium ‘Martinii’ and L. scoparium ‘Keatleyi’ were confirmed by flow cytometry. Floral corolla diameter at full opening was measured on a sample of flowers of each accession. The mean flower diameters were compared between lines using a linear mixed model, estimating a separate variance for each type. Comparisons among means were made using 5% least significant differences.
Surface sterilisation, colchicine treatment and germination of seed
Seed capsules were collected from a diploid plant in a private garden in Pohara, Golden Bay, NZ, in January 2017 and allowed to dry slowly at room temperature. The mother plant had small, white, single flowers typical of the wild type and did not appear to be a cultivated variety but its provenance is unknown. Seed collected from dried capsules was stored with silica gel at 4°C until needed. All of the experiments described were conducted within 6 months of seed harvest.
Initial attempts to introduce the seed into aseptic culture were unsuccessful because of repeated fungal contamination. Several different seed surface-sterilisation treatments were tested (data not shown) before concluding that immersion in a 1% sodium hypochlorite solution with 0.5% Tween 20 for 70 min reduced the contamination to a manageable level without adversely influencing seed viability. A 500 mg aliquot of seed was then treated in this manner, rinsed five times with 50 ml of sterilised water, then divided into five 15 ml disposable centrifuge tubes, each containing approximately 8 ml of water. Colchicine stock solution was added to give final concentrations of; 0.5. 1.0, 2.0, 4.0, 6.0 mg/ml, and the tubes held on a gentle agitator for 48 h at room temperature. Only one replicate of each colchicine treatment was used. At the end of the treatment period, the colchicine solution was removed and the seed rinsed five times in 15 ml of sterile water. The seed was then sown onto plating medium that did not contain sucrose, to slow the growth of any contaminating microorganisms. Over the next 3 weeks the plates were monitored and seedlings recovered onto fresh plates on occasions when contamination was observed. Once it was clear that the plates were free of contaminants, the seedlings were transferred onto plating medium containing 30 g/L sucrose to encourage plant growth.
The plating medium comprised MS salts (Murashige and Skoog Citation1962) at half strength, B5 vitamins (Gamborg et al. Citation1968) and 7.5 g L−1 Bacto-agar (New Zealand Manuka Bioactives Ltd). When used, sucrose was added at 30 g L−1. The pH of the medium was adjusted to pH 5.7 with either 0.1 N NaOH or 0.1 N HCl prior to autoclaving at 121°C for 15 min. The culture vessels used for initiation were 8 cm Petri plates with approximately 25 mL of medium. Culture vessels used subsequently were disposable 290 ml (80 mm base diameter × 60 mm deep) tubs with snap-on lids (Alto Packaging Ltd, Hamilton, New Zealand), containing approximately 50 mL of medium. The in vitro plant material was maintained at 25 ± 2°C with a 16/8 h light/dark cycle and a light intensity of 35–45 µmol m−2 s−1 at shelf level provided by ‘Cool White’ fluorescent tubes.
Once the seedlings were approximately 4 cm tall, they were transferred into potting mix, acclimatised in a mist tent and then transferred into the glasshouse. The mortality rate during the acclimatisation step, however, was highly variable. To mitigate the influence of plant mortality on our study of the impacts of colchicine treatment, flow cytometry was conducted on material while it was still in tissue culture.
Ploidy quantification
Ploidy was determined by flow cytometry using Trifolium repens as an internal standard (https://cvalues.science.kew.org) and the cultivars L. scoparium ‘Crimson Glory’, L. scoparium ‘Martinii’, L. scoparium ‘Keatleyi’ as 2x, 3x and 4x ploidy standards, respectively. The method followed that of Otto (Citation1990). Briefly, a small amount of leaf tissue was chopped finely with a razor blade in 400 μl of extraction buffer (citric acid 0.5%; Tween 0.5%) and filtered through 30 μm mesh (Partec filter). A 1.6 ml aliquot of staining solution (2 mg/ml DAPI in saturated dibasic sodium phosphate to give a final concentration of DAPI 2.5 μg/ml) was then added and the samples left to stand for at least 2 min. DAPI fluorescence was measured in a Partec Space Flow Cytometer with relative fluorescence compared with the karyotyped control plants. Data were analysed using Flowmax software: only readings with a coefficient of variation of less than 3% were used.
Results
Floral diameter
and present the results of the floral diameter study. The floral diameters of the triploid and tetraploid cultivars were found to be similar, and to be greater than the average diameters of the diploid plants studied. Amongst the diploids studied, flower diameter was found to be a variable trait. Significant differences were recorded amongst the accessions measured.
Figure 2. Flower diameter of three diploid varieties (plants 1, 2 and 3), one triploid variety (Leptospermum scoparium ‘Martinii’) and one tetraploid variety (L. ‘Keatleyi’). Means are indicated by the horizontal bars.
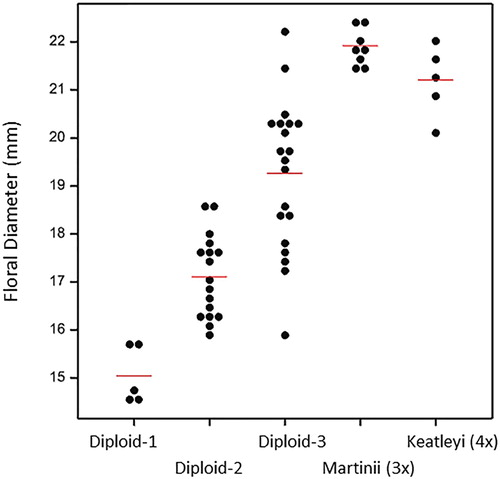
Table 1. Mean flower diameter (mm) of three diploid, one triploid and one tetraploid accession of Leptospermum scoparium. Means with different LSD letters are significantly different (0.05 LSD) from each other.
Formation of tetraploids using colchicine
lists the recovery rates of different ploidy types following treatment with a range of colchicine concentrations. In addition to diploids and tetraploids, some mixoploids (plants that displayed a clear mixture of both diploid and tetraploid cell types) were also produced.
Table 2. The influence of colchicine concentration on the recovery of mixoploid and tetraploid seedlings. For each treatment 60 seedlings were tested. Numbers in parentheses are percentages.
Increased colchicine concentration led to increased numbers of recovered tetraploids with the highest concentration tested (6.0 mg/ml) yielding the highest percentage of tetraploids (8.3%). It was noted that higher rates of colchicine also increased mortality and resulted in a slowing of subsequent growth in culture. For this analysis, ‘non-survival’ included seedlings that lost their terminal meristem and were unable to develop beyond the open cotyledon stage.
Discussion
We are interested in the potential role that ploidy might play in the development, phenology, fertility and biochemistry of L. scoparium. Higher ploidy is often reported to result in larger organ sizes, in particular larger flowers and larger leaves (Ramsey and Schemske Citation2002; Ranney Citation2006; Sattler et al. Citation2016; Eng and Ho Citation2019). This effect can be seen in and in the measured floral diameter values (, ). There is evidence, however, that other genetic factors also influence flower diameter in L. scoparium as the recorded values for the three diploid accessions varied significantly (). Additionally, flower diameter was seen to be highly variable on a single plant (), indicating that environmental and/or physiological factors also influence the expression of this trait in L. scoparium.
Higher ploidy in flowering plants, particularly triploidy and pentaploidy, are also reported to reduce fertility as a consequence of imbalanced meiotic division and the production of dysfunctional gametes (Ranney Citation2006). The flowers of diploid, wild-type L. scoparium are readily self-fertile and in New Zealand they are visited by a wide variety of insects, both native and introduced (Primack Citation1978; Primack and Lloyd Citation1980; Newstrom and Robertson Citation2005; Stephens et al. Citation2005). Pollination and fertilisation, therefore, can occur soon after flower opening in this species. Bee visitation is clearly desirable for commercial honey production but the resulting pollination may significantly reduce flower display life and nectar production (Primack Citation1985; Fung and Thomson Citation2017). We are unaware of any study specifically addressing this question in L. scoparium. However, in other species, successful pollination is known to stimulate petal senescence (Eason et al. Citation2007) and to end nectar production, directing the plant's resources away from insect attraction and towards seed development (see Fung and Thomson Citation2017 for review). Dawson (Citation1990) noted that the average pollen viability of the triploids L. scoparium ‘Martinii’ and L. scoparium ‘Helene Strybing’ and the tetraploid L. scoparium ‘Keatleyi’ remained above 40% (as measured using Alexander's stain), which was similar to that of several of the diploids tested. Dawson also noted, however, that seed set was very low for all of the polyploid plants studied, suggesting that pollen membrane integrity (as indicated by Alexander's stain) may not be well correlated to seed viability in polyploid plants of L. scoparium.
Prior to initiating a comprehensive study of the influence of ploidy in L. scoparium, we believe that a wider range of polyploid material is required. Currently, all of the known polyploids are closely related. Only a single tetraploid variety of L. scoparium is known (L. scoparium ‘Keatleyi’) and all of the available triploids are seedlings of that plant. Any study using this material alone would be confounded by co-ancestry, preventing the assessment of genotype × ploidy interactions. To address this difficulty, we developed a technique for producing tetraploid plants of L. scoparium using the chromosome doubling agent colchicine (Eng and Ho Citation2019). Treatment of L. scoparium seed led to the formation of both tetraploid and mixoploid plants. Mixoploidy arises from the incomplete conversion of a diploid plant, resulting in a chimeric mixture of diploid and tetraploid cell lineages within a plant body. It can arise following treatment with a chromosome doubling agent because each cell is influenced independently and chimeras arise following the initial cell divisions (Tambong et al. Citation1998). As a plant grows, one lineage typically predominates and the mixoploidy effect diminishes (Roy et al. Citation2001; Doležel et al. Citation2007). As we determined seedling ploidy in tissue culture, soon after colchicine treatment, the high rate of observed mixoploidy may be a reflection of incomplete lineage selection in these plants. In hindsight it would have been interesting to retest the mixoploid plants at a later date to monitor possible conversion to either diploid or tetraploid types. The sampling method used, however, was destructive so this was not a possibility.
The highest rate of colchicine tested (6.0 mg/ml) resulted in the highest levels of tetraploids recovered (8.3%). This level of colchicine also resulted in higher seedling mortality and, initially, in reduced plant vigour. In practical terms, the high mortality is not necessarily a difficulty if extra seed can be used to compensate for seedling losses. The reduced vigour of treated plants was seen to persist for several months in culture but, typically treated seedlings swiftly regained vigour after they are removed from tissue culture. For this reason we now try to remove seedlings from culture as quickly as possible after treatment.
Using the findings of this work, experiments are now in progress to produce more polyploid forms of L. scoparium. It is intended to then use these plants to test the impact of polyploidy on the development, phenology, fertility and biochemistry of L. scoparium across a range of genotypes and ploidy states.
Acknowledgements
The authors would like to thank David Lewis and Mary Christey for their editorial assistance. We would also like to thank Graeme Platt and Mike Clearwater for helping locate L. scoparium ‘Keatleyi’.
Disclosure statement
No potential conflict of interest was reported by the authors.
ORCID
Ross Bicknell http://orcid.org/0000-0001-5429-3142
Murray Boase http://orcid.org/0000-0001-7747-670X
Edmond Morgan http://orcid.org/0000-0002-5353-2639
Correction Statement
This article has been republished with minor changes. These changes do not impact the academic content of the article.
References
- Allan HH. 1961. Flora of New Zealand. Wellington: R. E. Owen. 1085 p.
- Bicknell R. 1995. Breeding cut flower cultivars of Leptospermum using interspecific hybridisation. New Zealand Journal of Crop and Horticultural Science. 23(4):415–421. doi: 10.1080/01140671.1995.9513918
- Bicknell R, Boase M, Morgan E. 2018. Rediscovery of the tetraploid Leptospermum scoparium variety ‘Keatleyi’. New Zealand Garden Journal (Journal of the Royal New Zealand Institute of Horticulture). 21(2):10–12.
- Brooker SG, Cambie RC, Cooper RC. 1987. New Zealand medicinal plants. Auckland: Reed. 117 p.
- Burge GK, Bicknell R, Dobson B. 1996. Postharvest treatments to increase water uptake and the vase life of Leptospermum scoparium Forst. New Zealand Journal of Crop and Horticultural Science. 24(4):371–378. doi: 10.1080/01140671.1996.9513974
- Butz Huryn VM. 1995. Use of native New Zealand plants by honey bees (Apis mellifera L.): a review. New Zealand Journal of Botany. 33(4):497–512. doi: 10.1080/0028825X.1995.10410621
- Christoph F, Kubeczka KH, Stahl-Biskup E. 1999. The composition of commercial Manuka oils from New Zealand. Journal of Essential Oil Research. 11(6):705–710. doi: 10.1080/10412905.1999.9712001
- Crowe A. 1981. A field guide to the native edible plants of New Zealand. Auckland: Godwit Publishing.
- Dawson M. 1997a. A history of Leptospermum scoparium in cultivation: garden selections. New Plantsman. 4:67–78.
- Dawson M. 1997b. A history of Leptospermum scoparium in cultivation-discoveries from the wild. New Plantsman. 4:51–59.
- Dawson MI. 1990. Leptospermum scoparium (manuka) – chromosome variation of cultivars. Horticulture in New Zealand. 1:15–19.
- Doležel J, Kubaláková M, Paux E, Bartoš J, Feuillet C. 2007. Chromosome-based genomics in the cereals. Chromosome Research. 15:51–66. doi: 10.1007/s10577-006-1106-x
- Douglas MH, van Klink JW, Smallfield BM, Perry NB, Anderson RE, Johnstone P, Weavers RT. 2004. Essential oils from New Zealand manuka: triketone and other chemotypes of Leptospermum scoparium. Phytochemistry. 65(9):1255–1264. doi: 10.1016/j.phytochem.2004.03.019
- Eason JR, Debenham M, McLachlan A, Morgan E. 2007. Novel red-flowered Gentiana: an emerging export cut flower crop from New Zealand. Acta Horticulturae. 755:25–267.
- Eng W-H, Ho W-S. 2019. Polyploidization using colchicine in horticultural plants: a review. Scientia Horticulturae. 246:604–617. doi: 10.1016/j.scienta.2018.11.010
- Fung HF, Thomson JD. 2017. Does lack of pollination extend flower life? Journal of Pollination Ecology. 21:86–91.
- Gamborg OL, Miller RA, Ojima K. 1968. Nutrient requirements of suspension cultures of soybean root cells. Experimental Cell Research. 50(1):151–158. doi: 10.1016/0014-4827(68)90403-5
- Lammerts W. 1945. New double flowering Leptospermum hybrids. Journal of the Californian Horticultural Society. 6(3):250–257.
- Murashige T, Skoog F. 1962. A revised medium for the rapid growth and bioassays with tobacco tissue cultures. Physiologia Plantarum. 15:473–497. doi: 10.1111/j.1399-3054.1962.tb08052.x
- Newstrom L, Robertson A. 2005. Progress in understanding pollination systems in New Zealand. New Zealand Journal of Botany. 43(1):1–59. doi: 10.1080/0028825X.2005.9512943
- Otto F. 1990. DAPI staining of fixed cells for high-resolution flow cytometry of nuclear DNA. Methods in Cell Biology. 33:105–110. doi: 10.1016/S0091-679X(08)60516-6
- Perry NB, Brennan NJ, Van Klink JW, Harris W, Douglas MH, McGimpsey JA, Smallfield BM, Anderson RE. 1997. Essential oils from New Zealand manuka and kanuka: chemotaxonomy of Leptospermum. Phytochemistry. 44(8):1485–1494. doi: 10.1016/S0031-9422(96)00743-1
- Porter NG, Smale PE, Nelson MA, Hay AJ, Van Klink JW, Dean CM. 1998. Variability in essential oil chemistry and plant morphology within a Leptospermum scoparium population. New Zealand Journal of Botany. 36(1):125–133. doi: 10.1080/0028825X.1998.9512551
- Porter NG, Wilkins AL. 1999. Chemical, physical and antimicrobial properties of essential oils of Leptospermum scoparium and Kunzea ericoides. Phytochemistry. 50(3):407–415. doi: 10.1016/S0031-9422(98)00548-2
- Primack RB. 1978. Variation in New Zealand montaine and alpine pollinator assemblages. New Zealand Journal of Ecology. 1:66–73.
- Primack RB. 1985. Longevity of individual flowers. Annual Review of Ecology and Systematics. 16:15–37. doi: 10.1146/annurev.es.16.110185.000311
- Primack RB, Lloyd DG. 1980. Andromonoecy in the New Zealand montane shrub manuka, Leptospermum scoparium (Myrtaceae). American Journal of Botany. 67:361–368. doi: 10.1002/j.1537-2197.1980.tb07661.x
- Ramsey J, Schemske DW. 2002. Neopolyploidy in flowering plants. Annual Review of Ecology and Systematics. 33(1):589–639. doi: 10.1146/annurev.ecolsys.33.010802.150437
- Ranney TG. 2006. Polyploidy: from evolution to new plant development. Combined Proceedings International Plant Propagators’ Society. 56:137–142.
- Roy A, Leggett G, Koutoulis A. 2001. In vitro tetraploid induction and generation of tetraploids from mixoploids in hop (Humulus lupulus L.). Plant Cell Reports. 20(6):489–495. doi: 10.1007/s002990100364
- Sattler MC, Carvalho CR, Clarindo WR. 2016. The polyploidy and its key role in plant breeding. Planta. 243:281–296. doi: 10.1007/s00425-015-2450-x
- Smith-White S. 1948. Cytological studies in the Myrtaceae. 2. chromosome numbers in the Leptospermoideae and Myrtoideae. Proceedings of the Linnean Society of New South Wales. 73(Parts 1–2):15–38.
- Stephens JMC, Molan PC, Clarkson BD. 2005. A review of Leptospermum scoparium (Myrtaceae) in New Zealand. New Zealand Journal of Botany. 43(2):431–449. doi: 10.1080/0028825X.2005.9512966
- Tambong J, Sapra V, Garton S. 1998. In vitro induction of tetraploids in colchicine-treated cocoyam plantlets. Euphytica. 104(3):191–197. doi: 10.1023/A:1018609020397
- Thompson J. 1989. A revision of the genus Leptospermum (Myrtaceae). Telopea. 3(3):301–449. doi: 10.7751/telopea19894902
- Williams S, King J, Revell M, Manley-Harris M, Balks M, Janusch F, Kiefer M, Clearwater M, Brooks P, Dawson M. 2014. Regional, annual, and individual variations in the dihydroxyacetone content of the nectar of manuka (Leptospermum scoparium) in New Zealand. Journal of Agricultural and Food Chemistry. 62(42):10332–10340. doi: 10.1021/jf5045958
- Zieslin N, Gottesman V. 1983. Involvment of ethylene in the abscission of flowers and petals of Leptospermum scoparium. Physiologia Plantarum. 58:114–118. doi: 10.1111/j.1399-3054.1983.tb04152.x
- Zieslin N, Gottesman V. 1986. Environmental factors involved in growth, flowering and post-harvest behavior of flowers of Leptospermum scoparium. Israel Journal of Botany. 35:101–108.