ABSTRACT
The application of zinc (Zn) fertiliser to soil has been proposed as a mitigation strategy to decrease plant cadmium (Cd) concentrations. The strategy is untested in New Zealand and it is unclear whether it is effective in soils that are not Zn deficient. We undertook a glasshouse experiment and measured what effect applying Zn had on Cd concentrations in spinach (Spinacia olearacea L.) cultivated in a soil with a high extractable Zn concentration (7.3 mg kg−1) and total Cd concentration of 0.48 mg kg−1. The addition of 5–20 kg Zn ha−1 as zinc sulphate decreased Cd concentrations in spinach leaves after 64 days growth by 19–28% and in roots by up to 42%. In contrast, the addition of Zn increased Zn concentrations in spinach roots and leaves by 55% and 112% respectively. The decrease in Cd concentrations in spinach was likely a result of competition with Zn that restricted Cd uptake into the root. The results indicate there may be potential in using Zn fertiliser to decrease plant Cd concentrations. However, investigation needs to be undertaken to evaluate its effectiveness for soil types with different properties and Zn status, other food crops which may respond differently to spinach and the results confirmed in a field experiment.
KEYWORDS:
Introduction
Cadmium (Cd) is a non-essential heavy metal and incidental impurity in many phosphorus (P) fertilisers (McLaughlin et al. Citation1996). The long-term use of P fertiliser has resulted in accumulation of Cd in some agricultural soils (Baerug and Singh Citation1990; Kelliher et al. Citation2017). There is concern about Cd accumulation in soil because Cd is readily absorbed by plants (Chaney and Hornick Citation1978; Baldantoni et al. Citation2016) and can enter the human food chain through the crops and crop-based products we consume in our diet (Rietra et al. Citation2017; Song et al. Citation2017). Chronic exposure to Cd above maximum food limits may pose a threat to human health (Järup and Åkesson Citation2009), specifically through its toxicity to the renal cortex, and adverse effects on the cardiovascular, pulmonary, and musculoskeletal systems.
As a result of the risk Cd can pose to food safety and human health, there has been a large amount of research undertaken to develop strategies to mitigate plant uptake of Cd. One simple strategy that has been shown to decrease plant Cd concentrations is the addition of zinc (Zn) fertiliser to soil (Choudhary et al. Citation1995; Grant and Bailey Citation1997; Brennan and Bolland Citation2014; Fahad et al. Citation2015; Qaswar et al. Citation2017). Paul and Chaney (Citation2017) found the application of 200 kg Zn ha−1 decreased Cd concentrations in spinach by 63% compared to the control. Several mechanisms have been reported to be responsible for the decrease in Cd uptake from the addition of Zn. It has been suggested that because Zn and Cd are chemically similar, both ions can compete for (i) sorption onto and uptake into plant roots, and (ii) transporter sites involved in movement of Cd and Zn from the roots to other parts of the plant (Hart et al. Citation2002; Zare et al. Citation2018).
Other studies however have shown that while the addition of Zn can decrease plant Cd concentrations, its effectiveness can vary depending on the rate and form Zn is applied to the soil, the plant species being cultivated, and how Zn is applied (Choudhary et al. Citation1995; Oliver et al. Citation1997; Zhao et al. Citation2005; Khoshgoftarmanesha et al. Citation2013; Fahad et al. Citation2015). Of particular importance is the Zn status of the soil (Oliver et al. Citation1994; Brennan and Bolland Citation2014). Torun et al. (Citation2013) found the one-off application of only 5 mg Zn kg−1 to a Zn-deficient soil decreased Cd concentrations on average by 73% in 13 canola genotypes. Others have reported that when Zn was applied to soils that were not considered Zn deficient, it was less effective (MacLean Citation1976; Forster et al. Citation2018). Grant and Bailey (Citation1998) showed the application of 10 kg Zn ha−1 to a soil that was not Zn deficient had no significant effect on wheat grain Cd concentrations.
In New Zealand, food standards for Cd have periodically been exceeded in different food crops including vegetables such as spinach (Cavanagh et al. Citation2019). At present, few mitigations are available that have consistently been shown to reduce plant uptake of Cd in New Zealand. The application of Zn fertiliser has been suggested as a potential mitigation option (MAF Citation2011). However, this has not been tested in New Zealand.
It is also uncertain how effective adding Zn to soil will be at decreasing plant uptake of Cd in vegetable crops such as spinach, because many soils used to grow vegetables appear to have high available Zn concentrations compared to soils used to grow other crops. Gray (unpublished Citation2019) for example found ethylenediaminetetraacetic acid (EDTA)-extractable Zn concentrations in soils ranged from 3.4–36.7 mg kg−1 (median 9.7 mg kg−1) from 10 sites used to grow onions and spinach in three regions of New Zealand. These values are high compared to EDTA-extractable Zn concentrations that have been reported in soils used to grow other crops in New Zealand such as lucerne (0.68 mg kg−1 n = 147) (McLaren et al. Citation1984) and wheat (0.94 mg kg−1 n = 23) (Curtin et al. Citation2008). The concentrations are also a lot higher than the critical limits in soils reported by Alloway (Citation2009) used to diagnose Zn deficiencies which are 1–1.5 mg Zn kg−1 in soils extracted using EDTA, as well as the critical limit of 0.8 mg kg−1 for EDTA-extractable Zn based on dry matter (DM) production of wheat from soils in Canterbury (Haynes Citation1997). The aim of this study was to use a glasshouse experiment to determine whether applying Zn fertiliser to soil can reduce Cd concentrations in spinach in a soil that is not Zn deficient.
Materials and methods
Soil
A topsoil (0–15 cm) was sampled from a long-term market garden site in Pukekohe (37°14 S 174° 55 E), in the North Island of New Zealand. The soil is a Patumahoe silty clay, classified in the New Zealand Soil Classification as Orthic Granular soil (Hewitt Citation2010) or a Typic Haplohumult (USDA). It is one of the most important soils used for growing vegetable crops accounting for about 25% of vegetable production in New Zealand (Martindale et al. Citation2018). The soil was sieved moist to pass through a 10 mm sieve, and a subsample taken. The subsample was dried to a constant weight and ground to pass through a 2 mm sieve before chemical and physical analysis.
Glasshouse experiment
Five rates of Zn (equivalent to 0, 2, 5, 10 and 20 kg Zn ha−1) as reagent grade zinc sulphate were dissolved in deionised water and applied to the soil surface. Zinc sulphate was used as it is a common form of Zn fertiliser applied to crops in New Zealand. Treated soil was mixed thoroughly, and 1.8 kg of moist soil added to plastic pots (15 cm diameter) and left to equilibrate in the glasshouse for one week prior to planting. A subsample of treated soil was dried, sieved and analysed for soil pH, soluble Cd and Zn and total Zn as described below. Five spinach (Spinacia olearacea L. var Pacer) seeds were planted per pot. Once established, spinach was thinned to four plants per pot. There were four replicates of each treatment and pots were arranged in a randomised complete block design. Plants were grown in a glasshouse maintained at 15° C (dark 8 h) and 20° C (light 16 h). The soil moisture content was maintained at 65% of water holding capacity by watering to weight throughout the experiment. The only nutrient added was nitrogen (N) applied at a rate equivalent to 200 kg ha−1 and divided into two equal applications. Soil analysis indicated other plant nutrients were in adequate supply based on values reported by Reid and Morton (Citation2019) (). Spinach was grown for 64 days before both the plant roots and leaves were harvested.
Table 1. Selected properties of soil (0–15 cm) used in the glasshouse experiment.
Soil analysis
Soil pH was determined in a 1:2.5 soil:water solution by potentiometric analysis (Blakemore et al. Citation1987). Available potassium (K), magnesium (Mg) and calcium (Ca) were extracted in a 1 M neutral ammonium acetate extract (Blakemore et al. Citation1987), and analysed by Inductively Coupled Plasma-Optical Emission Spectrometry (ICP-OES). Cation exchange capacity (CEC) was calculated by summing concentrations of extractable cations and extractable acidity. Bioavailable P (Olsen P) was determined following bicarbonate extraction (Olsen et al. Citation1954). Total carbon (C) and nitrogen (N) were determined by combustion using an Elementar Vario-Max CN Elemental analyser. Amorphous iron (Fe) and aluminium (Al) oxide concentrations were determined by oxalate-extraction (McKeague and Day Citation1966). Total recoverable Cd and Zn was determined by nitric acid/hydrochloric digestion (US EPA 200.2) followed by analysis using Inductively Coupled Plasma-Mass Spectrometry (ICP-MS). EDTA-extractable Zn was determined using the method described by Sherrel et al. (Citation1990) and analysed for Zn by ICP-OES. Soluble Cd and Zn were determined in 0.05 M Ca(NO3)2 (1:10 ratio for 16 h) (Gray et al. Citation1999) and analysed by ICP-MS. Particle size analysis was measured using the pipette method (Claydon Citation1989).
Plant analysis
At harvest, plant roots and aboveground leaf material were washed thoroughly and dried at 65°C until a constant weight was obtained. Dried plant samples were ground (<1 mm) and then digested using the nitric acid and hydrogen peroxide microwave method described by Cindric et al. (Citation2015), and analysed for Cd by ICP-MS and Zn by ICP-OES.
Quality control
Quality control measures for plant Cd and Zn analysis included the use of blanks, duplicate samples and analysis of the certified reference material NIST 1515 Apple Leaf. The duplicate results were within 5%, blanks were less than the detection limit of 0.010 mg kg−1 for Cd and 2 mg kg−1 for Zn, and the certified reference values were within 5% of the reported values. Titanium was also measured in plant material to confirm there had been no soil contamination.
Statistical analysis
Soil and plant variables were compared between treatments using analysis of variance (ANOVA). Each ANOVA consisted of a treatment factor and block effect. Effects were considered significant if they differed at the 5% probability level based on Fisher's unprotected least significant difference (LSD) test. Analysis was carried out using GenStat for windows v18.
Results
Soil
Properties of the experimental soil prior to the application of Zn treatments are given in . Soil pH (6.5) and the total C (3.2%) are both within the range reported for soils for commercial sites used to grow vegetables in New Zealand (Cavanagh et al. Citation2019). The total soil Cd concentration (0.48 mg kg−1) was slightly higher than the mean Cd concentration of 0.45 mg kg−1 reported for agricultural soils in New Zealand (Abraham Citation2018), although is within the range (0.07–1.35 mg kg−1) reported for commercial sites used to grow vegetables in New Zealand (Cavanagh et al. Citation2019). Total Zn (56 mg kg−1) was at the lower end of the range of values (53–188 mg kg−1) reported for soils from commercial sites used to grow vegetables in New Zealand (Cavanagh et al. Citation2019). In contrast, EDTA-extractable Zn (7.3 mg kg−1), which is recognised as a better measure of Zn availability than total soil Zn (McLaren et al. Citation1984) was high.
Dry matter yields
Dry matter yields for spinach roots and leaves are given in . On average, DM in spinach leaves were about four times higher than the DM in the roots. The application of Zn had no significant effect on the DM yield in spinach roots or leaves (). The lack of a yield response confirms the soil was not Zn deficient for spinach production.
Table 2. Mean ± SEM dry matter (DM) yields in spinach roots and leaves after 64 days in response to increasing zinc (Zn) application rates. Means followed by the same letter in the same column are not significantly different (P < 0.05).
Zinc concentrations in spinach
Average Zn concentrations ranged from 122 to 228 mg kg−1 DM in spinach roots (a) and from 65 to 138 mg kg−1 DM in spinach leaves (b). The application of Zn significantly increased Zn concentrations in spinach roots, although only at the two highest Zn treatments (10 and 20 kg Zn ha−1), which were 33% and 55% higher than the control (a). In contrast, Zn concentrations in spinach leaves increased at each Zn treatment level (b).
Cadmium concentrations in spinach
Average Cd concentrations in spinach roots ranged from 2.40–4.20 mg kg DM (a). Cadmium concentrations in spinach leaves were lower, ranging from 0.72–1.00 mg kg DM (0.09–0.12 mg kg−1 fresh weight (FW)) (b). Cadmium in spinach leaves slightly exceeded concentrations reported for baby leaf spinach harvested from commercial sites across New Zealand (0.005–0.110 mg kg−1 FW) (Cavanagh et al. Citation2019). Cadmium concentrations in both spinach roots and leaves decreased with increasing Zn application. The application of 20 kg Zn ha−1 decreased Cd concentrations in spinach leaves by 28% compared to the control. Further, Cd concentrations in spinach roots decreased by up to 42% at the highest Zn treatment. Cadmium concentrations in spinach roots were positively correlated with Cd in spinach leaves (r = 0. 822 P < 0.001) (). In contrast, Cd concentrations in spinach leaves were negatively correlated with Zn concentrations in spinach leaves (r = −0.586 P < 0.01), and there was no significant relationship between Cd and Zn concentrations in spinach roots ().
Figure 2. Mean (± SEM) cadmium (Cd) concentrations (mg kg−1 DM) in spinach root (A) and leaf (B) for contrasting rates of zinc (Zn) application to soil. Means followed by the same letter are not significantly different (P < 0.05).
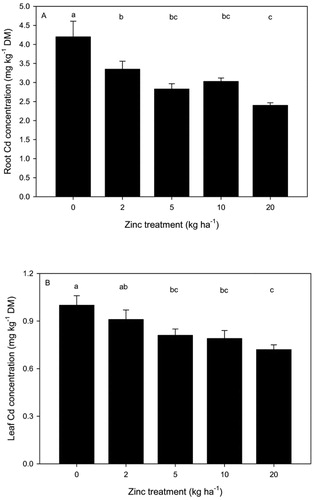
Table 3. Linear correlation coefficients (r) between soluble Cd and Zn concentrations in the soil, spinach root and leaf dry matter (DM) yields at 64 days and spinach root and leaf zinc (Zn) and cadmium (Cd) concentrations.
Soil pH and soluble Zn and Cd concentrations
The application of Zn had no significant effect on soil pH (). The application of Zn resulted in a four-fold increase in soluble Zn concentrations from 135 µg kg−1–552 µg kg−1 (). In contrast, Zn had no significant effect on soluble Cd concentrations (). Soluble Zn concentrations were negatively correlated with Cd concentrations in both spinach roots (r = −0.669 P < 0.01) and spinach leaves (r = −0.615 P < 0.01), and positively correlated with Zn concentrations in spinach root (r = 0.793 P < 0.001) and leaves (r = 0.955 P < 0.001) ().
Table 4. Mean ± SEM soil pH, extractable soil zinc (Zn) and cadmium (Cd) concentrations in response to increasing Zn application rates. Means followed by the same letter in the same column are not significantly different (P < 0.05).
Discussion
Cadmium concentrations in spinach
The application of Zn fertiliser to soil has been proposed as a simple mitigation to reduce plant uptake of Cd, although as yet it is untested in New Zealand. This study showed the addition of 5–20 kg Zn ha−1 decreased Cd concentrations in spinach leaves by 19–28%. Importantly, the application of Zn also decreased Cd concentrations in spinach to values below the maximum limit of 0.1 mg kg−1 FW for Cd outlined in the Food Standards of Australia and New Zealand (FSANZ) standard 1.4.1 (FSANZ Citation2013).
The decrease in Zn is within the range of values that have previously been reported for different food crops. Grant and Bailey (Citation1997) found the application of 10 kg Zn ha−1 significantly decreased Cd concentrations in flaxseed at two field sites by up to 8%. Cadmium concentrations in brown rice decreased by 17% from the application of 45 kg Zn ha−1 (Huang et al. Citation2019). McLaughlin et al. (Citation1995) reported Cd concentrations in potato tubers decreased between 25–50% from the addition of Zn fertiliser to soil at rates of up to 100 kg ha−1.
Plant growth dilution can sometimes be a complicating factor when trying to assess the effectiveness of the Zn application on plant Cd concentrations. Jiao et al. (Citation2004) for example reported that lower plant Cd concentrations in both flax and durum wheat were a combination of both growth dilution along with the direct influence of Zn application. Oliver et al. (Citation1994) found that even though wheat grain yields at six of the 12 sites investigated increased in response to Zn application, growth dilution only partly contributed to the lower Cd concentrations at two sites. Other studies (Grant and Bailey Citation1998; Paul and Chaney Citation2017; Forster et al. Citation2018) including the present investigation report that the application of Zn had no effect on plant yield, and therefore growth dilution was not a factor contributing to the lower plant Cd concentrations.
It was also found that despite the soil used in this study not being Zn deficient (EDTA-extractable Zn 7.3 mg kg−1), the application of Zn fertiliser was still effective at decreasing Cd concentrations in spinach. This is contrary to the findings of many previous studies which report that the application of Zn is most effective at decreasing plant Cd concentrations in soils that are marginally or severely Zn deficient (Oliver et al. Citation1994; Torun et al. Citation2013; Brennan and Bolland Citation2014). This is important if, as reported (Gray unpublished Citation2019), soils used to grow vegetables in New Zealand are not generally deficient in Zn.
Mechanisms
It has been reported that Zn and Cd can compete for both exchange sites on the root apoplast, and symplastic uptake into plant roots (Hart et al. Citation2002). Zinc and Cd also compete for the same transporter sites involved in the transport of Cd from the roots to the shoots via the xylem, transfer from the xylem to the phloem and transport through the phloem system (Cakmak et al. Citation2000; Hart et al. Citation2002; Jiao et al. Citation2004; Zare et al. Citation2018). The addition of Zn not only decreased Cd concentrations in spinach roots and leaves, it also increased Zn concentrations in the plant. Soluble Zn concentrations in the soil also increased with the addition of Zn and were more than an order of magnitude higher than soluble Cd concentrations. It appears the addition of even low rates of Zn (5 kg ha−1) was sufficient to increase soluble Zn in the soil to concentrations that were able to effectively compete with Cd for exchange sites on and uptake into spinach roots, that helped minimise Cd accumulation in the edible portions of spinach.
Management considerations
The amount of Zn that is required to be applied to soil to decrease plant Cd concentrations is an important factor to consider when evaluating Zn as a mitigation strategy. This is because like Cd, Zn can accumulate in soils to concentrations that may have a negative effect on soil health such as microbial growth and the activity of soil enzymes (Alloway Citation2004). In this study, the application of between 5 and 20 kg Zn ha−1, increased total soil Zn concentration from 56 mg kg−1 (baseline measure) to 63.5 and 84.5 mg Zn kg−1 respectively (data not shown). There are no New Zealand guidelines for critical total Zn concentrations in agricultural soils that have been derived using New Zealand data. In Europe, Smolders et al. (Citation2009) reported predicted no effect concentrations (PNECs) of between 94–246 mg kg−1 Zn for the protection of soil biota, such as soil microbes, plants and soil invertebrates, for two reference soils that had some characteristics in common with the soil used in the present study (pH, clay content, CEC). Without taking into account how much Zn would be removed from the soil through processes such as plant uptake, leaching and soil erosion, the results of this study suggest that the application of 5 kg Zn ha−1 may only result in a modest annual increase in total soil Zn over the medium term. In comparison, the application of up to 20 kg Zn ha−1 may be less sustainable, with total soil Zn possibly exceeding critical limits within two years.
Another factor that also needs to be considered is what residual effect Zn may have on plant Cd concentrations. Oliver et al. (Citation1994) found that the application of up to 5 kg Zn ha−1 decreased Cd concentrations in wheat grain by up to 50% in marginal to severely Zn deficient soils. However, they also reported that Cd concentrations decreased in wheat grown 1–4 years after the application of Zn fertiliser. This could mean a single application of Zn may be effective over several crops and therefore require less frequent application of Zn fertiliser.
In addition to spinach, the maximum limit of 0.1 mg kg−1 FW for Cd in wheat grain has also periodically been exceeded at some sites in New Zealand (Gray et al. Citation2001; Gray et al. Citation2019). Many of the soils used to grow wheat in New Zealand have low available Zn (< 1.9 mg kg−1 EDTA-extractable Zn) (Curtin et al. Citation2008) and are potentially Zn deficient for wheat (Haynes Citation1997). Given that it has been reported Zn is most often effective at decreasing plant Cd concentrations in soils that are marginally or severely Zn deficient, there is clearly potential to investigate whether the application of Zn to these cropping soils could also decrease Cd in wheat grain, along with other crops where Cd uptake has been identified as an issue.
Future research
Although this study found the application of Zn resulted in a significant decrease in plant Cd concentrations, future research still needs to determine whether the decease is consistent across other soil types with different properties that might affect Zn and Cd availability. Investigation into the form and method of application of Zn could also be considered. In this study, zinc sulphate was incorporated into the soil prior to planting of spinach. Other studies (e.g. Forster et al. Citation2018) have reported that foliar applications of Zn-EDTA were more effective at reducing plant uptake of Cd than zinc sulphate applied to the soil at planting compared to the untreated control. Further, the effect of Zn application on Cd uptake needs to be assessed using other important food crops, beyond spinach.
Conclusion
The addition of 5–20 kg Zn ha−1 to soil significantly decreased Cd concentrations in spinach leaves between 19–28%. This was despite the results of this study showing that the soil was not deficient in Zn for spinach. The decrease in Cd concentration in spinach was likely a result of Zn inhibiting sorption of Cd onto and then into the root. The results of this study indicate that while Zn may have some potential as a mitigation tool to reduce plant uptake, future investigation needs to be undertaken to evaluate its effectiveness in soils with different properties and Zn status, for food crops other than spinach and the results confirmed in field experiments.
Acknowledgements
Chikako van Koten provided statistical advice and analysis.
Disclosure statement
No potential conflict of interest was reported by the author(s).
ORCID
Colin William Gray http://orcid.org/0000-0002-5397-8243
Additional information
Funding
References
- Abraham E. 2018. Cadmium in New Zealand agricultural soils. New Zealand Journal of Agricultural Research. doi:10.1080/00288233.2018.1547320.
- Alloway BJ. 2004. Zinc in soils and crop nutrition. IZA Publications. International Zinc Association, Brussels. 1–116.
- Alloway BJ. 2009. Soil factors associated with zinc deficiency in crops and humans. Environmental Geochemistry and Health. 31:537–548. doi: 10.1007/s10653-009-9255-4
- Baerug T, Singh BR. 1990. Cadmium levels in soils and crops after long-term use of commercial fertilizers. Norwegian Journal of Agricultural Science. 4:251–260.
- Baldantoni D, Morra L, Zaccardelli M, Alfani A. 2016. Cadmium accumulation in leaves of leafy vegetables. Ecotoxicology and Environmental Safety. 123:89–94. doi: 10.1016/j.ecoenv.2015.05.019
- Blakemore LC, Searle PL, Daly BK. 1987. Methods for chemical analysis of soils. New Zealand Soil Bureau Scientific Report 80. Lower Hutt, New Zealand, NZ Soil Bureau.
- Brennan RF, Bolland MDA. 2014. Application of increasing levels of zinc to soil reduced accumulation of cadmium in lupin grain. Journal of Plant Nutrition. 37:147–160. doi: 10.1080/01904167.2013.859700
- Cakmak I, Welch RM, Erenoglu B, Römheld V, Norvell WA, Kochian LV. 2000. Influence of varied zinc supply on re-translocation of cadmium (109Cd) and rubidium (86Rb) applied on mature leaf of durum wheat seedlings. Plant and Soil. 219:279–284. doi: 10.1023/A:1004777631452
- Cavanagh JE, Yi Z, Gray CW, Munir K, Lehto N, Robinson B. 2019. Cadmium uptake by onions, lettuce and spinach in New Zealand – implications for management. Science of the Total Environment. 668:780–789. doi: 10.1016/j.scitotenv.2019.03.010
- Chaney RL, Hornick SB. 1978. Accumulation and effects of cadmium on crops. Proceedings of the first International cadmium Conference. San Francisco, London: Metals Bulletin Ltd. p. 125–140.
- Choudhary M, Bailey LD, Grant CA, Leisle D. 1995. Effect of Zn on the concentration of Cd and Zn in plant tissue of two durum wheat lines. Canadian Journal of Plant Science. 75:445–448. doi: 10.4141/cjps95-074
- Cindric IJ, Zeiner M, Pozgaj M. 2015. Elemental characterisation of the medical plant Alchemilla velebitica. Journal of Trace Elements in Medicine and Biology. 31:274–278. doi: 10.1016/j.jtemb.2014.09.008
- Claydon JJ. 1989. Determination of particle size distribution in fine-grained soil: Pipette method. Dept. of Scientific and Industrial Research. Lower Hutt, New Zealand.
- Curtin D, Martin RL, Scott CL. 2008. Wheat (Triticum aestivum) response to micronutrients (Mn, Cu, Zn, B) in Canterbury, New Zealand. New Zealand Journal of Crop and Horticultural Science. 36:169–181. doi: 10.1080/01140670809510233
- Fahad S, Hussain S, Khan F, Wu C, Saud S, Hassan S, Ahmad N, Gang D, Ullah A, Huang J. 2015. Effects of tire rubber ash and zinc sulfate on crop productivity and cadmium accumulation in five rice cultivars under field conditions. Environmental Science and Pollution Research. 22:12424–12434. doi: 10.1007/s11356-015-4518-3
- Forster SM, Rickertsen JR, Mehring GH, Ransom JK. 2018. Type and placement of zinc fertilizer impacts cadmium content of harvested durum wheat grain. Journal of Plant Nutrition. 41:1471–1481. doi: 10.1080/01904167.2018.1457687
- [FSANZ] Food Standards Australia New Zealand. 2013. Standard 1.4.1 code schedule 19 contaminants and natural toxicants. Australian Gov. [accessed 2020 Mar 4]. http://www.comlaw.gov.au/Details/F2013C00140.
- Grant CA, Bailey JD. 1998. Nitrogen, phosphorus and zinc management effects on grain yield and cadmium concentration in two cultivars of durum wheat. Canadian Journal of Plant Science. 78:63–70. doi: 10.4141/P96-189
- Grant CA, Bailey LD. 1997. Effects of phosphorus and zinc fertilizer management on cadmium accumulation in flaxseed. Journal of the Science of Food and Agriculture. 73:307–314. doi: 10.1002/(SICI)1097-0010(199703)73:3<307::AID-JSFA732>3.0.CO;2-3
- Gray CW, McLaren RG, Roberts AHC. 2001. Cadmium concentrations in some New Zealand wheat grain. New Zealand Journal of Crop Horticultural Science. 29:125–136. doi: 10.1080/01140671.2001.9514170
- Gray CW, McLaren RG, Roberts AHC, Condron LM. 1999. Cadmium phytoavailability in some New Zealand soils. Soil Research. 37:461–477. doi: 10.1071/S98070
- Gray CW, Yi Z, Munir K, Lehto NJ, Robinson BH, Cavanagh JE. 2019. Cadmium concentrations in New Zealand wheat – effect of cultivar type, soil properties and crop management. Journal of Environmental Quality. 48:701–708. doi: 10.2134/jeq2018.12.0430
- Hart JJ, Welch RM, Norvell WA, Kochian LV. 2002. Transport interactions between cadmium and zinc in roots of bread and durum wheat seedlings. Physiologia Plantarum. 116:73–78. doi: 10.1034/j.1399-3054.2002.1160109.x
- Haynes RJ. 1997. Micronutrient status of a group of soils in Canterbury, New Zealand, as measured by extraction with EDTA, DTPA and HCl, and its relationship with plant response to applied Cu and Zn. Journal of Agricultural Science. 129:325–333. doi: 10.1017/S0021859697004735
- Hewitt AE. 2010. New Zealand soil classification—3rd edition. Lincoln: Manaaki Whenua Press.
- Huang G, Ding C, Zhou Z, Zhang T, Wang X. 2019. A tillering application of zinc fertilizer based on basal stabilization reduces Cd accumulation in rice (Oryza sativa L.). Ecotoxicology and Environmental Safety. 167:338–344. doi: 10.1016/j.ecoenv.2018.10.044
- Järup L, Åkesson A. 2009. Current status of cadmium as an environmental health problem. Toxicology and Applied Pharmacology. 238:201–208. doi: 10.1016/j.taap.2009.04.020
- Jiao Y, Grant CA, Bailey LD. 2004. Effects of phosphorus and zinc fertilizer on cadmium uptake and distribution in flax and durum wheat. Journal of the Science of Food and Agriculture. 84:777–785. doi: 10.1002/jsfa.1648
- Kelliher FM, Gray CW, Noble ADL. 2017. Superphosphate fertiliser application and cadmium accumulation in a pastoral soil. New Zealand Journal of Agricultural Research. 60:404–422. doi: 10.1080/00288233.2017.1363058
- Khoshgoftarmanesha AH, SanaeiOstovar A, Sadrarhamic A, Chaney R. 2013. Effect of tire rubber ash and zinc sulfate on yield and grain zinc and cadmium concentrations of different zinc-deficiency tolerance wheat cultivars under field conditions. European Journal of Agronomy. 49:42–49. doi: 10.1016/j.eja.2013.02.013
- MacLean AJ. 1976. Cadmium in different plant species and its availability in soils as influenced by organic matter and additions of lime P, Cd and Zn. Canadian Journal of Plant Science. 56:129–138.
- MAF. 2011. Cadmium and New Zealand agriculture and horticulture: A strategy for long term risk management. Technical Paper 2011/03. Ministry Agric. For., Wellington, New Zealand. https://www.mpi.govt.nz/dmsdocument/10073/loggedIn (accessed 26 Mar. 2019).
- Martindale M, Hicks D, Singleton P. 2018. Soil information inventory: Patumahoe and related soils. Auckland Council soil information inventory, SII 16.
- McKeague JA, Day JH. 1966. Dithionite- and oxalate-extractable Fe and Al as aids in differentiating various classes of soils. Canadian Journal of Soil Science. 46:13–22. doi: 10.4141/cjss66-003
- McLaren RG, Swift RS, Quin BF. 1984. EDTA extractable copper, zinc, and manganese in soils of the Canterbury plains. New Zealand Journal of Agricultural Research. 27:207–217. doi: 10.1080/00288233.1984.10430423
- McLaughlin MJ, Maier NA, Freeman K, Tiller KG, Williams CMJ, Smart MK. 1995. Effect of potassic and phosphatic fertilizer type, fertilizer Cd concentration and zinc rate on cadmium uptake by potatoes. Fertilizer Research. 40:63–70. doi: 10.1007/BF00749863
- McLaughlin MJ, Tiller KG, Naidu R, Stevens DP. 1996. Review: the behaviour and environmental impact of contaminants in fertilizers. Soil Research. 34:1–54. doi: 10.1071/SR9960001
- Oliver DP, Hannam R, Tiller KG, Wilhelm NS, Merry RH, Cozens GD. 1994. The effects of zinc fertilization on cadmium concentrations in wheat grain. Journal of Environmental Quality. 23:705–711. doi: 10.2134/jeq1994.00472425002300040013x
- Oliver DP, Wilhelm NS, McFarlane JD, Tiller KG, Cozens GD. 1997. Effect of soil and foliar applications of zinc on cadmium concentration in wheat grain. Australian Journal of Experimental Agriculture. 37:677–681. doi: 10.1071/EA97017
- Olsen SR, Cole CV, Watanabe FS, Dean LA. 1954. Estimation of available phosphorus in soils by extraction with sodium bicarbonate. USDA Circular 939. U.S. Government Printing Office, Washington D.C.
- Paul ALD, Chaney RL. 2017. Effect of soil amendments on Cd accumulation by spinach from a Cd-mineralized soil. Journal of Environmental Quality. 46:707–713. doi: 10.2134/jeq2016.07.0251
- Qaswar M, Hussain S, Rengel Z. 2017. Zinc fertilisation increases grain zinc and reduces grain lead and cadmium concentrations more in zinc-biofortified than standard wheat cultivar. Science of the Total Environment. 605–606:454–460. doi: 10.1016/j.scitotenv.2017.06.242
- Reid JB, Morton JD. 2019. Nutrient management for vegetable crops in New Zealand. Horticulture New Zealand, Wellington. [accessed 2019 Jun 12]. http://www.fertiliser.org.nz/site/resources/booklets.aspx.
- Rietra RPJJ, Mol G, Rietjens IMCM, Romkens PFAM. 2017. Cadmium in soil, crops and resultant dietary exposure. Rep. 2784. Wageningen Environmental Research Wageningen, the Netherlands. doi:10.18174/403611.
- Sherrel CG, Percival NS, Gee TM. 1990. Effects of cobalt application on the cobalt status of pastures. 1. Pastures with history of regular cobalt application. New Zealand Journal of Agricultural Research. 33:295–304. doi: 10.1080/00288233.1990.10428422
- Smolders E, Oorts K, Van Sprang P, Schoeters I, Janssen CR, McGrath SP, McLaughlin MJ. 2009. Toxicity of trace metals in soil as affected by soil type and aging after contamination: using calibrated bioavailability models to set ecological soil standards. Environmental Toxicology and Chemistry. 28:1633–1642. doi: 10.1897/08-592.1
- Song Y, Wang YBN, Mao WF, Sui HX, Yong L, Yang DJ, Jiang DG, Zhang L, Gong YY. 2017. Dietary cadmium exposure assessment among the Chinese population. PLoS ONE. 12:e0177978. doi: 10.1371/journal.pone.0177978
- Torun AA, Tolay I, Aytac Z, Yuksel O, Yardim P. 2013. Shoot cadmium concentration and accumulation of different canola (Brassica napus ssp. oleifera L.) genotypes under different zinc, nitrogen and sulfur treatments. Journal of Food Agriculture and Environment. 11:689–695.
- Zare AA, Khoshgoftarmanesha AH, Malakoutib MJ, Bahrami HA, Chaney RL. 2018. Root uptake and shoot accumulation of cadmium by lettuce at various Cd:Zn ratios in nutrient solution. Ecotoxicology and Environmental Safety. 148:441–446. doi: 10.1016/j.ecoenv.2017.10.045
- Zhao ZQ, Zhu YG, Smith FA, Smith SE. 2005. Cadmium uptake by winter wheat seedlings in response to interactions between phosphorus and zinc supply in soils. Journal of Plant Nutrition. 28:1569–1580. doi: 10.1080/01904160500203457