Abstract
It is preferable to prepare internally consistent maps of arid regions on a global scale in order to understand the present conditions of arid regions, especially deserts and soil degradation areas. We attempted to delimit arid regions at a global scale by combining climate data, i.e. aridity index (AI), and vegetation data, i.e. vegetation index. The annual AI was estimated by the ratio of mean annual precipitation to mean annual potential evapotranspiration, using the Thornthwaite method. The long-term mean of yearly maximum normalized difference vegetation index (NDVIymx) was used as an indicator of the vegetation condition. Arid regions of the world were classified into four categories, namely A, severe deserts, where both aridity and vegetation indices are very small; G, semi-arid regions, where the vegetation index is proportionally related to the AI; I, irrigated areas and oases, where the vegetation is relatively abundant despite severe dryness; and S, soil degradation areas, where the vegetation is poor despite relatively humid conditions. The Sahel from Niger to Chad, the Sahel in Darfur, and the Ordos Plateau in China are within Category S. The standard deviation of NDVIymx is very small/large in severe deserts/semi-arid areas, respectively. Thus, the Sahara desert was clearly distinguished from the Sahel; the latter belongs to Category G and drought occurs frequently here. In Category S zones, the standard deviation of NDVIymx is relatively small compared with that within the Category G zone because the return rainfall does not seem to promptly restore productivity. Category S was divided into three subdivisions according to the degree of degradation, expressed by the ratio of the AI to vegetation index. Category G was also divided into four classes, according to degree of vegetation (or aridity). The distribution of Category S is comparable to the soil degradation areas mapped by Global Assessment of Human-Induced Soil Degradation (GLASOD) data. True deserts, where the standard deviation of NDVIymx is very small, were selected from the ‘severe desert’ group. Desert areas were classified as true deserts, severe deserts, grassland deserts (Category G), and soil degradation deserts (Category S).
1. Introduction
It is believed that desert areas are spreading at a steady rate on a global scale, and that areas affected by desertification continue to expand rapidly. However, both desert and desertification are ambiguous concepts. There is no consensus on the appropriate method to determine a desert, especially desertification status. Most researchers have defined ‘desert’ according to their discipline (Tucker, Newcomb, and Dregne Citation1994). For the time being, a standardized assessment of desertification remains unresolved (Veron, Paruelo, and Oesterheld Citation2006). According to research for global land cover mapping, the accuracy of mapping a desert region is as good as that of an evergreen broadleaf forest, in comparison with other categories where accuracy is far from satisfactory (Gong et al. Citation2012). However, arid land is generally treated as ‘bare land’ or ‘grassland.’ In order to quantitatively estimate the extent of desert regions or desertification, it is clearly necessary to define these terms according to objective criteria that have physical and biological meaning.
Desertification is mainly defined as a process of land degradation that reduces its productivity. Desert areas are considered to be areas completely desertified. Generally, land degradation areas are formed when the fertile soil has been lost by water and/or wind erosion, etc. This would make it easier to identify the characteristics of degradation areas using vegetation indices such as the normalized difference vegetation index (NDVI) obtained by remote sensing satellite data, because NDVI quantifies the photosynthetic capacity of vegetation (Wessels et al. Citation2004). Several previous studies have used NDVI to assess the condition of vegetation in arid and semi-arid regions (Huang and Siegert Citation2006). However, there has been no prior systematic study on the identification of soil degradation areas (including desert areas) at a global scale, because it is difficult to distinguish degradation areas from semi-arid regions in which droughts occur frequently. Drought reduces plant growth; however, if the drought ends and rainfall returns, plant growth recovers to its former level, whereas soil degradation has a long-lasting effect with permanent and sometimes irreversible consequences for production (Le Houerou Citation1996). Therefore, in order to distinguish desert areas, areas where droughts occur frequently, and soil degradation areas, it is necessary to investigate the long-term variations in NDVI observations. It is also desirable to propose a degree of desertification, such as a desertification risk index, based on the use of climatic factors and vegetation data.
In order to analyse and assess future changes in arid areas (deserts, semi-arid regions and land degradation areas), it is necessary to understand the present status of arid regions. It is also important to identify the location of irrigated areas, including oases, because such areas have large populations and high production, despite their small area.
The purpose of this article is to prepare a map of global distribution of arid regions using objective criteria that have physical and biological meaning. We classified arid and semi-arid regions throughout the world using climate and vegetation data. Usually, arid areas are delimited by climatic aridity indices or vegetation indices, which are employed separately. In this article, the delimitation methodology combining both aridity and vegetation indices was proposed. Four main categories were defined, corresponding to the aridity and vegetation indices: Category A, which is highly arid regions where the vegetation is sparse (severe deserts); Category G, where the vegetation condition depends on the degree of aridity (the dry savanna in subtropical regions and grasslands (steppe) in mid-latitudes); Category I, where greenery is abundant despite dry conditions (irrigation regions or oases); and Category S, where the vegetation is poor despite relatively wet conditions. If desertification is considered as soil degradation, Category S coincides with the areas affected by desertification. In addition, the degree of soil degradation, i.e. the desertification risk index, was defined as the ratio of the aridity index (AI) to vegetation index. Using these four categories, we prepared a map of global aridity based on means values for 20 years, from 1982 to 2001.
2. Aridity index and vegetation index
The AI shows the degree of climatic dryness and vegetation index indicates the abundance of vegetation. Both indices are defined in a variety of ways for the purposes of analysis, and these definitions all have advantages and disadvantages. We attempted to delimit arid regions in the simplest possible form, wherein vegetation was expressed as the yearly maximum NDVI (NDVIymx) and climate was expressed as the annual AI, determined by the ratio of annual precipitation to annual potential evapotranspiration, based on Thornthwaite method (1948).
We selected areas between latitudes 55° S to 55° N in order to exclude tundra areas, where NDVIymx is small, because of the low vegetation growth resulting from the cold conditions. In addition, poor vegetation in mountainous areas is due to low temperature and strong winds, and hence, areas with an elevation of 3 km or greater were also excluded. Almost all arid regions associated with hot or warm climates are included within the selected region.
2.1. Aridity index
The degree of dryness is not determined by precipitation alone. If the temperature is high/low, evaporation is large/small. Therefore, the degree of dryness is normally expressed as precipitation divided by potential evapotranspiration, giving the AI. Generally, AI is expressed as annual base, that is by PE p −1 (in which P is the annual precipitation and E p is the annual potential evapotranspiration). The AI is an ambiguous term because potential evapotranspiration cannot actually be measured, but can only be inferred. The Thornthwaite method, which is an empirical formula, was used by Meigs (Citation1953) in the production of a map of world aridity for UNESCO. The values of E p in the Map of the World Distribution of Arid Regions (UNESCO Citation1977) was estimated using the Penman method (1984), which determined Ep based on physical considerations. This radiative method requires data parameters such as solar radiation, wind velocity, relative humidity, etc., which cannot be observed easily in arid areas. The values of E p in the World Atlas of Desertification of UNEP (Middleton and Thomas Citation1992, Citation1997) also used the Thornthwaite method (1948), due to a lack of required data and the unreliability of data in arid areas.
In this study, we used the Thornthwaite method to determine potential evapotranspiration. In this method, the quantity is determined empirically as a function of the mean monthly temperature only, except that the average number of daylight hours for the month is also taken into consideration. It is a reasonable assumption that radiation (net radiation R n in the Penman method) is closely linked to temperature. The close relationship between radiation and temperature holds in cool and temperate arid zones except in the Mediterranean area, where radiation is greatest in spring but temperature is highest in summer. The Thornthwaite method systematically underestimates E p for dry conditions and overestimates for moist and cold environments (Middleton and Thomas Citation1997; Hulme Marsh, and Jones Citation1992; Krishna Kumar, Rupa Kumar, and Pakhecha Citation1987). Thus, AI = PE p −1, as estimated by the Thornthwaite method, is overestimated in arid regions and underestimated for moist climates. In the World Atlas of Desertification, annual potential evapotranspiration values by the Thornthwaite method E pt (units: metres) were adjusted to better fit those calculated by the Penman method E pp according to Hulme and Marsh (Citation1990) or Hulme, Marsh, and Jones (Citation1992), as follows:
2.2. Vegetation index
The vegetation index is obtained on a global scale in real time from satellite remote sensing images by the same sensor systems. The vegetation index of arid areas where bare soil is visible is not a vegetation index in the original sense of the term. In arid areas, it is considered preferable to use a soil-adjusted normalized vegetation index such as the soil-adjusted vegetation index (SAVI) (Huete Citation1988), modified soil-adjusted vegetation index (MSAVI) (Qi et al. Citation1994), etc. According to Leprieur, Kerr, and Pichon (Citation1996), NDVI and MSAVI are proportionally related in arid regions. In this article, we used NDVI for simplicity, because it is not yet certain that soil-adjusted indices are appropriate to apply to land conditions at a global scale, and because NDVI is widely used for studying arid and semi-arid environments. In arid areas, vegetation index increases as the amount of greenery increases, ranging from values close to zero in the severe deserts to values of approximately 0.6 in steppe climates. Thus, the vegetation index is effective for delimiting arid areas.
Because NDVI varies in space and in time, generally, the yearly average, integrated values of NDVI in the growing season are used as the representative value. We selected yearly maximum NDVI (NDVIymx) to represent the vegetation condition for the following reasons: (1) yearly averaged vegetation index includes values in the snow-covered season; (2) the integrated value in the rainy season is ambiguous for analysis at a global scale; and (3) typical biogeological landscape is expressed on the basis of the wettest period in arid regions, even if this is of short duration. For example, the Mongolian Steppe experiences polar climate during winter and tundra climate during spring. However, the vegetation characteristics and landscape for this group are determined during the wet summer season, when the biomass is the greatest. Further, in the case of the Sahel zone, for example, a year's total primary production can occur within 2–3 weeks (Tucker et al. Citation1985).
2.2.1. Pathfinder NDVI
We used the NDVI derived from the National Aeronautics and Space Administration (NASA)/National Oceanic and Atmospheric Administration (NOAA)/Pathfinder Land (PAL) data, which were obtained by the Advanced Very High Resolution Radiometer (AVHRR). Green leaves absorb part of the visible part of the solar radiation spectrum and reflect light in the near-infrared. Thus, the NDVI is expressed as follows:
We used the monthly maximum NDVI data (resolution 8 km × 8 km), which are derived from the highest NDVI values within a 10 day maximum in daily Global Area Coverage (GAC) data.
2.2.2. Data preprocessing
We used NDVI data from 1982 to 2001. The discontinuities resulting from the NOAA-9 to NOAA-11 switch and the residual trends due to the satellite orbit drift were removed by Gutman and Ignatov (Citation1995). The data from 1982 to 1993 have been reprocessed with a more sophisticated calibration (Smith et al. Citation1997). However, the Pathfinder NDVI data set since 1994 has not been reprocessed. We reprocessed the data to obtain a consistent NDVI time-series for this study. The data processing we conducted is described below.
We created a histogram for a yearly maximum normalized vegetation index (NDVIymx) from PAL data. illustrates histograms for 1982, as an example. The histogram shows a distribution with two peaks for severe deserts (peak 1) and areas with lush greenery (peak 2). shows histograms of NDVIymx for each year from 1982 to 2001. Histograms of reprocessed NDVIymx from 1982 to 1993 show that the positions of the two peaks coincide with each other, whereas peak positions are different in many years after 1994. When averaged, the two peak values of the 12 sample years (from 1982 to 1993) where data have been reprocessed are denoted as s 1 and s 2; s 1 = 0.08 and s 2 = 0.72.
Figure 1. Histogram of original 125 NDVIymx (for 1982) globally between latitude 55°N and 55°S at an altitude below 3 km. Histogram vertical axis shows area.
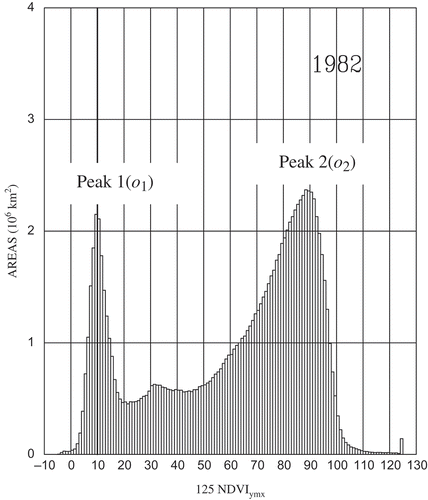
With the assumption of linear transformation, the original NDVIymx values for each year (NDVIo) were transformed to standardized NDVIymx values (NDVIs) so that the two peaks o 1 and o 2, which are values of the histograms of NDVIo, coincide with the two peaks of NDVIs (s 1 and s 2, respectively). As the linear transformation equation is expressed as NDVIs = a(NDVI)o + b (a and b are constants), the following equation is derived:
Hereafter, the analysed NDVIymx in our study indicates the standardized NDVIymx.
3. Results and discussion
3.1. Geographical distribution of aridity index
Conventionally, arid regions are described by the AI. The four categories of UNEP-delimited (Middleton and Thomas Citation1992, Citation1997) arid regions are as follows: hyperarid (AI < 0.05), arid (0.05 ≤ AI < 0.20), semi-arid (0.20 ≤ AI < 0.50), and dry subhumid (0.50 ≤ AI < 0.65) for the period 1951–1980. shows AI averaged over 30 years, from 1961 to 1990, estimated by the Thornthwaite method using temperature and precipitation data of CRU. Resolution is 0.5° in longitude and latitude. Ten categories based on AI are shown; there are ten graduations in increments of 0.05, between AI values of –0.05 and +0.50, and one graduation of 0.50 to 0.65. Distribution tendency of AI from hyperarid up to dry–subhumid closely matches the aridity map from UNEP.
3.2. Geographical distribution of NDVIymx
We calculated long-term average values of NDVIymx from the Pathfinder NDVI data set for the 20 year period from 1982 to 2001. (Extraordinary data in Sahara Desert and Arabian Desert in 2001 were excluded from analysis.) Horizontal resolution is 8 km × 8 km. illustrates the global distribution of values of NDVIymx that are less than 0.55. NDVIymx is shown segmented into 11 increments of 0.05 between –0.05 and +0.55. The value of NDVIymx increases from severe deserts to grasslands, in order to include areas where the vegetation index is lower. In general, the distribution of NDVIymx seems to be similar to the trends in the AI, which increases from dryland to wetland.
3.3. Categorization of arid areas in the AI–NDVIymx plane
We attempted to classify arid areas by the relationship between vegetation index and AI. Since one NDVIymx pixel (8 km × 8 km) is smaller than an AI pixel (0.5° in longitude and latitude), AI is uniquely specified for each NDVIymx pixel. shows a scatter plot of AI and NDVIymx for pixels of size 8 km × 8 km. illustrates the relationship between the long-term mean of AI (1961–1990) and NDVIymx (1982–2001). Pixel data are plotted every ten pixels in both latitude and longitude to illustrate distribution characteristics. There appears to be a linear relationship between AI and NDVIymx in arid regions, where AI is less than about 0.5, although there is a large scatter in the data. On the other hand, no relationship is evident between AI and NDVIymx in humid areas, where AI is larger than about 0.5. This tendency was also reported by Potter and Brooks (Citation1998), Gamo (Citation1999), and Suzuki, Xu, and Motoya (Citation2006).
Figure 5. Scatter plots of long-term mean of aridity index AI (1961–1990) and NDVIymx (1982–2001). Categories: A, severe deserts; I, irrigated areas and oases; G, semi-arid regions (savanna, steppe); H, humid and vegetation affluent regions; S, soil degradation areas in arid regions. G1–G4 indicate the vegetation condition from sparse to dense. Ss, Sm, and Sw indicate the severity of soil degradation (strong, moderate, and weak, respectively). Line (a) shows NDVIymx = 1.367AI, that is EquationEquation (4)(4). Line (b) shows NDVIymx = 3.2AI derived from EquationEquation (6)
(6).
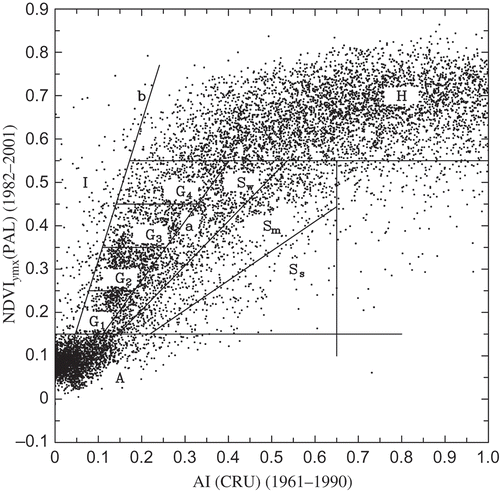
Water is generally scarce in arid areas. Hence, rainfall is consumed effectively and promptly for plant growth. Therefore, NDVIymx increases with an increase in the index of dry condition, expressed by AI = PE p −1. shows that NDVIymx increases linearly for most pixels, with AI less than 0.4–0.5. Since the variation in the range of annual potential evapotranspiration E p is much narrower compared with that of annual precipitation P (in the semi-arid category, G, E p = 0.8–1.4 m; P = 0.05–0.8 m), NDVIymx is believed to be linearly related to precipitation. Tucker, Dregne, and Newcomb (Citation1991) reported that the yearly mean NDVI increases linearly with yearly precipitation P in the Sahel zone in Africa. On the other hand, in humid areas, indicated by a large AI, the vegetation condition is determined not by water content but by temperature and several other factors. Thus, there is no relationship between NDVIymx and AI.
There seems to be proportional relationship between NDVIymx and AI for values of AI less than about 0.4–0.5. According to the linear regression method, the constants of the linear regression line (c and d are constants) become (c = 0.93, d = 0.08) and (c = 1.041, d = 0.07) in the case of AI < 0.5 and AI < 0.4, respectively, under the common condition −0.10 < NDVIymx < 0.55. The correlation coefficients are 0.81 and 0.80, respectively. Although the linear relationship between NDVIymx and AI is ascertained, it is difficult to determine appropriate constants of the linear relationship (c and d) due to the large scatter in the data. Here, we uniquely specified the constants (c and d) at which the number of pixels becomes largest for each AI, from the relationship between AI and NDVIymx. shows the frequency distribution of NDVIymx for specific AI values ranging from 0.10 to 0.48. shows the modal distribution, which indicates the relationships between AI and NDVIymx, based on the maximum frequency. Up to an AI of approximately 0.45, a linear relationship exists between NDVIymx and AI, expressed as follows:
This mode line is shown in , labelled ‘a.’ A pixel on the left/right side of this proportional line ‘a’ indicates that the vegetation condition in this area is better/worse than the corresponding vegetation condition at a pixel on the proportional line shown by EquationEquation (4)(4). As shown in , we attempted to classify arid regions within four broad categories. Category A denotes severe deserts where AI and NDVIymx are both small; Category I denotes areas where there is abundant vegetation despite dry climate, such as irrigated areas and oases. The range of Category I was determined by referring to a map of irrigated areas and oases. Category G is the arid areas that occur between Category I and the line of EquationEquation (4)
(4). Category G represents semi-arid regions where NDVIymx increases as AI increases. Category G seems to include tropical savanna in low latitudes and steppe in middle latitudes. Category G was divided into four subcategories (G1, G2, G3, and G4) according to the value of NDVIymx (or AI). Category S was determined where pixels exist on the right side of EquationEquation (4)
(4). Category S denotes arid areas where vegetation is poor despite the comparatively wet climate; in other words, it seems to indicate the areas of soil degradation. The degree of severity of soil degradation was defined by change of NDVIymxAI−1. Category S was divided into three subcategories (Sw, Sm, and Ss), representing weak, medium, and strong soil degradation conditions. Category H denotes humid areas, and includes the region where NDVIymx is larger than 0.55, except region I. The individual classification methods are described later, in detail. The pixel data, which are classified into 10 categories in , are plotted on the geographical map (). The horizontal resolution is 8 km × 8 km in latitude and longitude. Gamo (Citation1999) also classified arid regions in the Africa and Eurasia regions into the same four categories. However, in that case, the dividing line between zones G and S was arbitrarily assumed.
Figure 8. Map of global distribution of arid regions defined in Figure 5. Category: A, severe deserts; I, irrigated areas and oases; G, semi-arid regions (savanna, steppe); S, soil degradation areas in arid regions. G1–G4: vegetation condition (sparse ∼ dense). Ss, Sm, and Sw: severity of soil degradation (strong, moderate, and weak, respectively). H, humid and vegetation affluent regions; M: mountainous areas higher than 3 km; C, cool and cold areas located further north/south than 55° N/55° S in latitude.
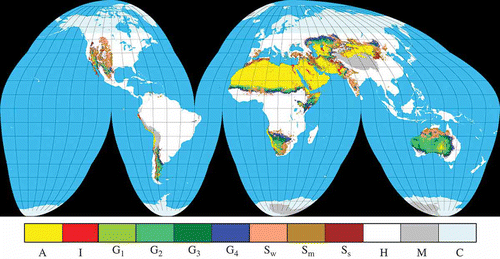
3.3.1. Category A (severe deserts)
‘Severe desert’ is an imprecise term. We defined severe desert as the area where NDVIymx is very small. As mentioned below, the standard deviation of NDVIymx over the long term is also very small in many severe deserts, which shows that the vegetation index NDVIymx is very small and very stable. Severe deserts are clearly categorized as areas where the NDVIymx is 0.15 or less, as in the following equation:
Severe deserts classified as Category A. In Africa, the deserts included in the category are Sahara D (hereafter D represents Desert), Danakil D (northeast of the Ethiopian Plateau), northern area of Somali Peninsula (Horn of Africa), Chalbi D surrounding Lake Turkana, and Namib D (coastal desert along Cold Benguela Current). The deserts in Asia included in this category are the Arabian Peninsula occupied by Arabian Desert comprising Syria D, Nahud D, Dahna D, Rub Al Khali D, etc.; deserts from the Iran Plateau to Indus River Plain (Kavir D, Lut D, Registan D, Tahr D, etc.); Karakum D (east of the Caspian Sea), Kyzyl-Kum D (south of the Aral Sea); Saryesik-Atyrau D (south of Lake Balkhash); deserts extending north of the Tibetan Plateau comprising Taklamakan D (Tarim Basin), Gurbantunggut D (Jungal Basin), Qaidam D (Qaidam Basin), Badain Jaran D (north of Chi-ren Mountains), Gobi D (stony desert), and others. The category also includes the Gobi-Altai district. In Australia, the southern part of Simpson D surrounding Lake Eyre is included in this category. In the Americas, the coastal desert by the Peru Cold Current, i.e. Peruvian D and Atakama D, fall under this category. Sites of severe deserts are also listed in . Category A occupies 11.1% of the total land surface of Earth.
Table 1. Areas include in Category A: severe deserts. Symbols show the severity of desert: true desert (Aa), very severe desert (Ab), and severe desert (Ac) (for details, see Section 3.4, standard deviation of NDVIymx)
3.3.2. Category I (irrigated areas and oases)
Category I denotes areas with plentiful vegetation despite high aridity, signifying irrigated areas and oases. Most irrigated areas exist in the basins of large rivers. Category I can be easily confirmed on a map, as shown in following equation:
Irrigated areas and oases (Category I) in Africa include the Nile River Basin; Blue Nile River Basin; White Nile River Basin; the area surrounding Lake Chad; the estuary of the Orange River at the south of Namib D; coastal regions of the Somali Peninsula; and many small oases in Sahara D. In Asia, regions in this category include the Central Arabian Peninsula, which is the location of an extensive agricultural complex utilizing centre-pivot, swing-arm irrigation systems; and the Mesopotamia Basin, known as the Fertile Crescent. Many small oases and irrigated areas along the coast of the Red Sea; the Indus River Basin where heavy flooding occurred in 2010; southern coast of Caspian Sea; river basins of Amu Darya and Syr Darya that flow into the Aral Sea; the delta of the Volga River, which flows into the Caspian Sea; a series of oases at the northern and southern edges of the Taklamakan D; oases at the southern edge of the Gurbantunggut D; oases at the eastern foot of the Altai Mountains; and oases at the northern foot of Chi-ren Mountains known as Hexi corridor are included in this category. Several oases in the Great Artesian Basin in Australia; Colorado River Basin, Sacramento River Basin, Columbia River Basin, Baja California, and east coast of the Gulf of California in the Americas also fall in this category. Irrigated areas and oases in the Peruvian D, south of the Atakama D, and irrigated areas in the Monte D and Patagonian D are also included in Category I. Sites of irrigated areas and oases are also listed in . Category I occupies 0.8% of all land surface. A detailed world irrigation map was produced by Thenkabail et al. (Citation2009), whose results are similar in relation to the arid regions identified in this study. Category I does not always accurately present cultivated areas. The Mesopotamian marshlands have been lost mainly as a result of drainage and damming. Irrigated areas along the Am Darya have shrunk due to drainage, and the Aral Sea is also continuing to shrink.
Table 2. Category I: irrigated areas and oases
3.3.3. Category G (semi-arid regions)
Category G shows semi-arid regions, which seem to represent dry savanna at low latitudes and ‘steppe’ at middle latitudes. The terms ‘savanna’ and steppe are also imprecise. Here, Category G is defined as arid zones located between the mode line, that is EquationEquation (4)(4); and the Category I zone in . Category G seems to show semi-arid regions where vegetation conditions are related to the state of aridity. From , region G was defined as being encompassed by the following four equations:
Furthermore, Category G is subdivided into four classes according to vegetation condition, as follows:
The degrees of aridity and vegetation are tabulated by classes G1–G4 in . The Category G region encompasses the following: Sahel (central Mali), Sahel (Chad), Somali Peninsula, areas surrounding Lake Turkana, west Morocco, northwest coast of Algeria, Kalahari D in Africa, west-central area of the Kazakh Steppe (which is part of the Eurasian Steppe), Mongolian Steppe in the eastern part of Mongolia, southern hillsides of the Zagros Mountains, northern hillsides of the Hindu-Kush Mountains, upstream region of the Indus River, and the belt-like region between the Thar D and Deccan Plateau from Ahmadabad to New Delhi in Asia; most Australian deserts (i.e. Great Sandy D, Gibson D, Great Victoria D, and Simpson D); many parts of Mojave D, Sonoran D, and Chihuahuan D, and Monte D in dry Pampa and Patagonian D (from centre to east) in the Americas. The areas belonging to Category G occupy 8.7% of the Earth's land surface.
Table 3. Category G: semi-arid regions (savanna, steppe). Symbols G1–G4 indicate the vegetation conditions from sparse to dense
3.3.4. Category S (soil degradation areas)
Category S denotes areas where vegetation is poor compared with the humidity level. Such areas may occur due to soil degradation or soil erosion. That is, Category S seems to represent areas affected by desertification. The degree of severity of soil degradation (a kind of desertification risk index) was defined on a trial basis by the value of NDVIymxAI−1, as shown in . Here, for descriptive purposes, Category S is divided into Sw, Sm, and Ss, according to weak, medium, and strong degradation condition, respectively. Soil degradation also occurs in humid climates (Le Houerou Citation1996). In order to omit land degradation areas in wet climates, including densely populated urban areas, pixels with an AI greater than 0.65 were not used. The threshold value of AI (0.65) is the same as for the subhumid zone used in the World Atlas of Desertification (Middleton and Thomas Citation1992, Citation1997). Equations defining the three subcategories are as follows:
Category S includes the following regions: west-central region of Sahel (5° W–10° E, south Niger–north Nigeria), east Sahel (22° E–26° E, including Darfur in south Sudan), north hillside of Ethiopian Plateau, surrounding area of the Atlas Mountains, areas to the south, east, and north of Kalahari D, and central to south Madagascar in Africa. The eastern half of the Iberian Peninsula (the soil is highly salinized) in Europe is also included in this category. In Asia, regions from the Anatolian Peninsula to the Zagros Mountains, southern hillside of the Hindu-Kush Mountains, Rajasthan east of Thar D, west part of Deccan Plateau, river-mouth of the Volga, east part of Kazakh Steppe (which extends south, surrounding Balkhash Lake), west and central Mongolian Steppe, Ordos D (including Kubuqi D and Mu Us D), areas between east Mongolian Steppe (Category G) and Ordos D, Horqin D (or Horqin Sandy Land, which is the nearest desert to Beijing) are included in this category. Areas of severe land degradation in China coincide with the regions in the desertification map described by Zha and Gao (Citation1997). In Australia, Kimberley Plateau, Tanami D, and Barkly Tableland from west to east fall under Category S. In the Americas, most of the Great Basin D, Great Plains from 30° N to 50° N, the southern part of Chihuahuan D, and the west part of Patagonian D fall under this category. Sites of soil degradation as well as the subcategories are listed in . Regions in Category S occupy 7.6% of all global land surface.
Table 4. Category S: soil degradation areas. Symbols show the severity of soil degradation: Ss: strong;+ Sm, moderate; and Sw, weak. Symbols in the ‘GLASOD’ column show the degree of desertification, s: strong, m: moderate, and l: light (for details, see Section 3.5, soil degradation map by GLASOD)
The spatial distribution of each category and subcategory are shown in , indicating that arid regions occupy 28.2% of the Earth's land surface. According to the Finer Resolution Observation and Monitoring of Global Land Cover (FROM-GLC) project (Gong et al. Citation2012), barelands and grasslands cover 16.5% and 13.4% of the Earth's land surface, respectively. If the barelands belong to arid regions or mountainous areas, the total area F of FROM-GLC composed of barelands and grasslands is seen to correspond to the area E derived from the relation arid regions (28.2%) – areas in Category I ( 0.8%) + high land areas above 3 km between latitudes 55° S to 55° N (2.7%). Since E (30.1%) is similar to F (29.9%), the data tabulated in appear to be reliable.
Table 5. Area and percentage of Earth's land surface for individual categories
3.4. Standard deviation of NDVIymx
We considered another approach for distinguishing between deserts, areas affected by drought, and areas affected by soil degradation. Drought is a temporary but normal occurrence, usually leaving little permanent aftermath. Vegetation is improved with increased precipitation, although droughts can easily occur in these areas (e.g. Tucker, Dregne, and Newcomb Citation1991). The area where standard deviation of NDVIymx (SDNDVI) is large seems to correspond to areas affected by drought, which are areas belonging to Category G. In contrast, soil degradation (desertification) has a long-lasting effect with permanent and sometimes irreversible consequences for production potential. SDNDVI seems to be not especially large in soil degradation areas; shows the geographical distribution of SDNDVI over 20 years, from 1982 to 2001, for the same regions analysed in . illustrates a histogram of the SDNDVI for all, and also for individual categories. SDNDVI for severe deserts (Category A) is small and sharply concentrated, while SDNDVI for Category G is large and widely distributed. SDNDVI for Category S is smaller and narrower than that for Category G. In the Sahara and the Sahel, Category G areas are clearly delineated by the difference in standard deviation values, whereas SDNDVI in the Sahel is affected by soil degradation (belonging to Category S) and not so large. The same tendency is observed at the transition zone between the Gobi D (Category A) and Ordos D (Category S).
Figure 9. Standard deviation of NDVIymx (SDNDVI) for 20 years (1982–2001). M, mountainous areas higher than 3 km; C, cool and cold areas located further north/south than 55° N/55° S in latitude.
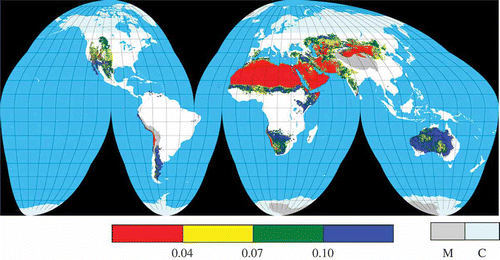
Figure 10. Histogram of standard deviation of NDVIymx (SDNDVI) globally between 55°N and 55°S below altitude of 3 km. Histogram vertical axis shows area. A, Category A (severe deserts); G, Category G (semi-arid regions); S, Category S (soil degradation areas); ALL, Categories A + G + S + I + H. (I, Category I (irrigated areas or oases); H, Category H (humid and vegetation affluent regions)). Line (c): SDNDVI = 0.03.
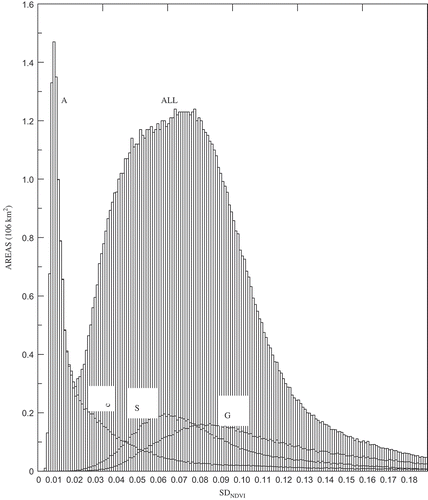
Arid regions of high SDNDVI belonging to Category G are observed at the following sites: Sahel labelled as Category G, south Somalia, area surrounding Lake Turkana, and north Kalahari D in Africa; northern Mesopotamia, north Kazakh Steppe, and east Mongolian Steppe in Asia; northeast, east, and west Australian Deserts; and Sonoran D, Chihuahuan D, Monte D, and Patagonia D in the Americas. All of the above regions coincide with semi-arid regions categorized as G except northeastern Australia, which is classified as a weak degree of soil degradation, Sw.
Arid regions of small SDNDVI are as follows: Sahel labelled as Category S, south Atlas, eastern Iberian Peninsula, Anatolian Peninsula, surrounding Zaglos Mountains, south Kazakh Steppe, west-central Mongolian Steppe, Ordos Plateau, and areas between the east Mongolian Steppe and Ordos Plateau, Horqin D, central and northwest of Australian Mainland, and Great Basin D, and Great Plains in the Americas. All of the above regions except Australia coincide with soil degradation areas categorized as S.
From the histogram of SDNDVI (see ), there are areas that belong to Category A with a large SDNDVI. This area seems to encompass areas where some vegetation appears due to occasional precipitation. Here, the severe desert is divided into a superdry region (SDNDVI of less than 0.03) and an ordinary dry region (SDNDVI of more than 0.03), mapped in . Severe deserts are divided into ‘Aa’ (true deserts), ‘Ab’ (very severe deserts), and ‘Ac’ (severe deserts), according to the rate of the superdry region for an individual severe desert (see ). ‘Aa’ shows almost all pixels belong to a superdry region; ‘Ab’ shows around half the pixels belong to a superdry region; and ‘Ac’ shows almost all pixels belong to an ordinary dry region. The true deserts shown in coincide with the desert areas that are generally regarded as being very severe deserts.
3.5. Soil degradation map by GLASOD
We compared areas grouped in Category S with soil degradation areas classified by the Global Assessment of Human-Induced Soil Degradation (GLASOD). The GLASOD database (Oldeman, Hakkeling, and Sombroek Citation1990) is compiled by the International Soil Reference and Information Centre (ISRIC), as commissioned by the United Nations Environment Programme (UNEP). The GLASOD database was prepared by more than 250 soil scientists from every continent. The degree of soil degradation was classified into extreme, strong, moderate, and light. shows the distribution of soil degradation types caused by water and wind erosion (DEG1 of GLASOD data). Areas of severe desert (Category A) were omitted, considering severe deserts to have been completely desertified. Irrigated areas (Category I) were not omitted, because soil degradation continues to expand or intensify in these areas. Degrees of soil degradation in GLASOD are strong, moderate, and light. Very few grid cells were classified as ‘extreme’ soil degradation (only the coastal region of Somalia and some parts of the north hillside of the Ethiopian Plateau). Here, for descriptive purposes, ‘extreme’ was included in ‘strong.’ The degree of soil degradation for each area is shown in .
Figure 12. Degree of soil degradation, as described using GLASOD (DEG1) data. A, severe deserts; M, mountainous areas higher than 3 km; C, cool and cold areas located further north/south than 55° N/55° S in latitude.
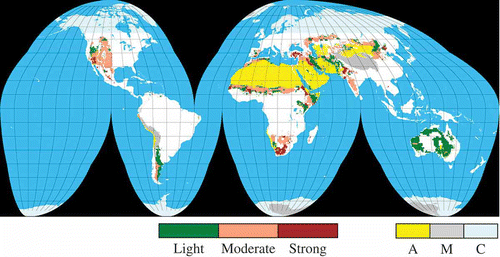
Many arid regions categorized as G (semi-arid) coincide with ‘light’ degree of desertification in GLASOD. Almost all irrigated areas and oases (Category I) have been affected by desertification across a broad spectrum from ‘light’ to ‘strong’ (for simplicity, comparisons of individual regions for Category G and I with GLASOD are not tabulated). As shown in , areas categorized as S (soil degradation) coincide with soil degradation areas classified as moderate–strong by GLASOD, although GLASOD soil degradation categories (strong–moderate–light) do not always coincide with degrees of soil degradation (Ss–Sm–Sw).
4. Conclusions
We produced a map of global distribution of arid regions using unified criteria that have both physical and biological meaning. We combined both indices on a two-dimensional graph of the long-term means of AI and vegetation index. We selected yearly AI as representative of climate, and yearly maximum NDVI (NDVIymx) as representative of vegetation. The long-term mean of vegetation index distinguishes deserts and areas affected by drought.
In order to obtain a consistent NDVI time-series, we reprocessed the Pathfinder data set. The histogram of areas for NDVIymx distribution showed two peaks in areas of low NDVIymx (severe deserts) and high NDVIymx (regions where vegetation is rich). Original NDVIymx values for each year were transformed to standardized NDVIymx so that the two peaks for each year became the same.
From the modal distribution of NDVIymx at individual AI, a linear relationship was found between AI and NDVIymx in arid regions where AI is less than about 0.45, although there is a large scatter in the data values.
Arid areas are usually delimitated separately by aridity indices or vegetation indices. Our methodology combined both aridity and vegetation indices, distinguishes irrigated areas, including oases and soil degradation areas. We delineated arid regions into four regions: (1) Category A (severe deserts) where NDVIymx is very small; (2) Category I (irrigated areas and oases) where vegetation is abundant despite dry conditions; (3) Category G (savanna, steppe) where the vegetation condition is related to the state of aridity; and (4) Category S (soil degradation areas) where the vegetation condition is worse in spite of wet conditions. Delimiting lines of Category I were determined by comparison with areas shown on a physical map. Category G is divided into four subcategories according to vegetation condition.
A type of desertification risk index is proposed on the relationship between AI and vegetation index (NDVIymx). Category S is divided into three subcategories (severe–moderate–light) according to NDVIymxAI−1. Soil-degraded areas categorized as S coincide with the moderate–strong stage of GLASOD desertification sites.
According to the histogram of the standard deviation of NDVIymx (SDNDVI), the SDNDVI for severe deserts (Category A) is small and sharply concentrated, while SDNDVI for Category G is large and widely distributed, because vegetation is improved due to increased precipitation, although droughts remain common. SDNDVI for Category S is smaller and narrower than for Category G, because soil degradation, unlike drought events, prevents the rapid recovery of vegetation following precipitation. Thus, the boundary of the Sahara D and the Sahel zone belonging to Category G is clearly determined by the difference of SDNDVI, while the boundary between the Sahara D and soil-degraded Sahel zone is not so clearly defined. The boundary between the Gobi D (Category A) and Ordos D (Category S) is vague, because of the same tendency.
Severe deserts are divided into three types of desert (true, very severe, and severe) according to the ratio of the small SDNDVI for individual severe deserts. Deserts are classified into five groups, namely, true deserts (e.g. Sahara D), very severe deserts (e.g. Karakum D), severe deserts (e.g. Tahr D), deserts belonging to Category G (e.g. Kalahari D), and deserts belonging to Category S (e.g. Ordos D including Kubqi D and Mu Us D). In this way, the map allows some simple comparisons between different arid regions of the world.
Our delimitation methodology appears to be applicable to other NDVI data sets, e.g. the NDVI data set of the Moderate Resolution Imaging Spectroradiometer (MODIS). When MODIS NDVIymx values were standardized by EquationEquation (3)(3), the delimitation map indicated a similar tendency to our study (details not shown here, for simplicity).
The distribution of deserts, arid areas, and soil degradation areas varies between studies. Such variations reflect differences in conforming data, category definitions, and threshold values. This article simply presents one set of guidelines. It is our hope that this article has presented a method that can easily be confirmed by other researchers, and that it will serve as the basis for an improved classification methodology in the future. The interannual change and trend of vegetation condition for desert and soil degradation areas will be discussed in future studies.
Acknowledgements
The authors would like to thank Nobuko Saigusa for suggestions about aridity indices, Koichi Masuda for help in applying the Thornthwaite method, Kaoru Tachiiri for transforming GLASOD polygon data to grid data, and Koki Iwao for transformation between Mercator and Goode homolosine projections. Thanks are also expressed to Masayasu Hayashi, Ryoichi Imasu, Wan Jiemin, Ma Yaomin, Ma Shaomin, Wen Jun, Kaz Higuchi, and Hiroaki Kondo for their contributions. We thank Editage for editing this article for language.
References
- Gamo , M. 1999 . Classification of the Arid Regions by Climate and Vegetation . Arid Land Studies , 9 : 17 – 26 .
- Gong , P. , Wang , J. , Yu , L. , Zhao , Y. , Zhao , Y. , Liang , L. , Niu , Z. , Huang , X. , Fu , H. , Liu , S. , Li , C. , Li , X. , Fu , W. , Liu , C. , Xu , Y. , Wang , X. , Cheng , Q. , Hu , L. , Yao , W. , Zhang , H. , Zhu , P. , Zhao , Z. , Zhang , H. , Zheng , Y. , Ji , L. , Zhang , Y. , Chen , H. , Yan , A. , Guo , J. , Yu , L. , Wang , L. , Liu , X. , Shi , T. , Zhu , M. , Chen , Y. , Yang , G. , Tang , P. , Xu , B. , Ciri , C. , Clinton , N. , Zhu , Z. , Chen , J. and Chen , J. 2012 . Finer Resolution Observation and Monitoring of Global Land Cover: First Mapping Results with Landsat TM and ETM+ Data . International Journal of Remote Sensing , 34 : 2607 – 2654 .
- Gutman , G. and Ignatov , A. 1995 . Global Land Monitoring from AVHRR: Potential and Limitations . International Journal of Remote Sensing , 16 : 2301 – 2309 .
- Huang , S. and Siegert , F. 2006 . Land Cover Classification Optimized to Detect Areas at Risk of Desertification in North China Based on SPOT VEGETATION Imagery . Journal of Arid Environments , 67 : 308 – 327 .
- Huete , A. R. 1988 . A Soil Adjusted Vegetation Index (SAVI) . Remote Sensing of Environment , 25 : 295 – 309 .
- Hulme , M. and Marsh , R. 1990 . Global Mean Monthly Humidity Surfaces, for 1930–59, 1960–89 and Projected for 2030 , Norwich : Report prepared for UNEP/GEMS/GRID, Climatic Research Unit, University of East Anglia .
- Hulme , M. , Marsh , R. and Jones , P. D. 1992 . Global Changes in a Humidity Index between 1931–60 and 1961–90 . Climate Research , 2 : 1 – 22 .
- Krishna Kumar , K. , Rupa Kumar , K. and Pakhecha , P. R. 1987 . Comparison of Penman and Thornthwaite Methods of Estimating Potential Evapotranspiration for Indian Conditions . Theoretical Applied Climatology , 38 : 140 – 146 .
- Le Houerou , H. N. 1996 . Climate Change, Drought and Desertification . Journal of Arid Environment , 3 : 133 – 185 .
- Leprieur , C. , Kerr , Y. H. and Pichon , J. M. 1996 . Critical Assessment of Vegetation Indices from AVHRR in a Semi-Arid Environment . International Journal of Remote Sensing , 17 : 2549 – 2563 .
- Meigs , P. 1953 . World Distribution of Arid and Semi-Arid Homoclimates . Arid Zone Hydrology, UNESCO, Arid Zone Research Series , 1 : 203 – 209 .
- Middleton , N. and Thomas , D. , eds. 1992 . World Atlas of Desertification, UNEP , 1 – 69 . London : Edward Arnold .
- Middleton , N. and Thomas , D. , eds. 1997 . World Atlas of Desertification, UNEP , 1 – 182 . London : Edward Arnold .
- New , M. , Hulme , M. and Jones , P. 1999 . Representing Twentieth-Century Space-Time Climate Variability. Part I: Development of a 1961–90 Mean Monthly Terrestrial Climatology . Journal of Climate , 12 : 829 – 856 .
- Oldeman , L. R. , Hakkeling , R. T. A. and Sombroek , W. G. 1990 . World Map of the Status of Human-Induced Soil Degradation , 1 – 18 . International Soil Reference and Information Centre . An Explanatory Note (Global Assessment of Soil Degradation GLASOD)
- Penman , H. L. 1984 . Natural Evaporation from Open Water, Bare Soil and Grass . Proceedings of the Royal Society, Series A , 193 : 120 – 145 .
- Potter , C. S. and Brooks , V. 1998 . Global Analysis of Empirical Relations between Annual Climate and Seasonality of NDVI . International Journal of Remote Sensing , 19 : 2921 – 2948 .
- Qi , J. , Chehbouni , A. , Huete , A. R. , Kerr , Y. H. and Sorooshian , S. 1994 . A Modified Soil Adjusted Vegetation Index . Remote Sensing of the Environment , 48 : 119 – 126 .
- Smith , P. M. , Kalluri , S. N. V. , Prince , S. D. and DeFries , R. 1997 . The NOAA/NASA Pathfinder AVHRR 8-km Land Data Set . Photogrammetric Engineering & Remote Sensing , 63 : 12 – 32 .
- Suzuki , R. , Xu , J. and Motoya , K. 2006 . Global Analyses of Satellite-Derived Vegetation Index Related to Climatological Wetness and Warmth . International Journal of Climatology , 26 : 425 – 438 .
- Thenkabail , P. S. , Biradar , C. M. , Noojipady , P. , Dheeravath , V. , Li , Y. , Velpuri , M. , Gumma , M. , Gangalakunta , O. R. P. , Turral , H. , Cai , X. , Vithanage , J. , Schull , M. A. and Dutta , R. 2009 . Global Irrigated Area Map (GIAM), Derived from Remote Sensing, for the End of the Last Millennium . International Journal of Remote Sensing , 30 : 3679 – 3733 .
- Thornthwaite , C. W. 1948 . An Approach Towards a Rational Classification of Climate . Geographical Review , 38 : 55 – 94 .
- Tucker , C. J. , Dregne , H. E. and Newcomb , W. W. 1991 . Expansion and Contraction of the Sahara Desert between 1980 and 1990 . Science , 253 : 299 – 301 .
- Tucker , C. J. , Newcomb , W. W. and Dregne , H. E. 1994 . AVHRR Data Sets for Determination of Desert Spatial Extent . International Journal of Remote Sensing , 15 : 3547 – 3565 .
- Tucker , C. J. , Vanpraet , C. L. , Sharman , M. J. and Van Ittersum , G. 1985 . Satellite Remote Sensing of Total Herbaceous Biomass Production in the Senegalese Sahel: 1980–1984 . Remote Sensing of Environment , 17 : 233 – 249 .
- UNESCO . 1977 . Map of the World Distribution of Arid Regions , 1 – 54 . Paris : UNESCO . Explanatory Note. MAB Technical Notes No. 7
- Veron , S. R. , Paruelo , J. M. and Oesterheld , M. 2006 . Assessing Desertification . Journal of Arid Environment , 66 : 751 – 763 .
- Wessels , K. J. , Prince , S. D. , Frost , P. E. and van Zyl , D. 2004 . Assessing the Effects of Human-Induced Degradation in the Former Homelands of Northern South Africa with a 1 km AVHRR NDVI Time-Series . Remote Sensing of Environment , 91 : 47 – 67 .
- Zha , Y. and Gao , J. 1997 . Characteristics of Desertification and its Rehabilitation in China . Journal of Arid Environments , 37 : 419 – 432 .