Abstract
The Jason-2 satellite altimeter mission was launched in June 2008, extending the record of precision sea level measurements that was initiated with the launch of TOPEX/Poseidon in 1992 and continued with the launch of Jason-1 in December 2001. We have used the measurements from these three missions to construct a seamless record of global mean sea level change from 1993 to the present. We present the results of our calibration activities, including data comparisons during the “tandem period” of the missions, during which we solve for biases between the missions, as well as comparisons to independent tide gauge sea level measurements. When the entire record is assembled, the average rate of sea level rise from 1993–2009 is 3.4 ± 0.4 mm/year. There is considerable interannual variation due to ENSO-related processes, which include the period of lower sea level rise over the last three years of the time series during the recent La Nina event.
Keywords:
1. Introduction
The satellite era has brought a wealth of new data for measuring long-term sea level change and has provided a means of monitoring the impact due to climate change. The Jason-2 altimeter mission was launched in June 2008, extending the record of precise altimetry initiated by the TOPEX/Poseidon (T/P) mission (August 1992) and later extended by the Jason-1 mission (December 2001). In order to assemble a precise climate data record of sea level change, however, great care must be taken to determine the measurement biases between these different missions and to understand and monitor changes in the biases during these missions. It is also important to understand the differences in measurement corrections and other parameters that affect the measurements between the different altimeter systems. While comparisons between the different missions have proven to be a critical tool for understanding and quantifying bias changes and uncertainties, tide gauge measurements are also vital to monitor the long-term stability of these instruments. This paper summarizes the results of these calibration techniques, and presents a new updated estimate of the 17-year record of global mean sea level change from the combination of these three missions.
2. The Tandem Mission Intercomparisons
With the launch of each new altimeter mission, a “tandem period” when the satellites flew together essentially simultaneously along the same 10-day repeating groundtrack, was implemented so that the measurements between the old and the new missions could be intercompared. As such, there was a 210-day tandem period between TOPEX/Poseidon and Jason-1 (after which T/P was moved to an interleaved orbit with Jason-1) and a 180-day tandem period (shortened due to a Jason-1 safehold condition in 2008) between Jason-1 and Jason-2 (after which Jason-1 was moved to an interleaved orbit with Jason-2). Since the two satellites fly over nearly the same region within a minute of each other, the two satellites should be observing the same real ocean variability. When the two measurements are differenced, real variability (and any correlated errors) will cancel out, leaving residuals that are caused by instrument and model correction differences (e.g., CitationChambers et al. 2003a). The tandem period phase for T/P and Jason-1 was vital for detecting a number of problems in both the Jason-1 and TOPEX altimeters, including a problem in the sea state bias model of TOPEX (CitationChambers et al. 2003b), errors in the TOPEX and Jason radiometers related to the yaw regime (CitationChambers et al. 2003a; CitationBrown et al. 2004; CitationDesai and Haines 2004; CitationObligas et al. 2004; CitationZlotnicki and Desai 2004), and geographically correlated errors related to differences in the reference frames used to compute orbits (CitationBeckley et al. 2007). These tandem periods continue to provide an opportunity to assess (or reassess) the performance of all three satellites.
The altimeter data are released to the science team in the form of Geophysical Data Records (GDRs). Initially, Interim GDRs are released, but these have inferior orbits and the measurement corrections are often not final. The first official release is the GDR-A. As the science and data processing teams study the results from the GDRs, they often develop improvements to the processing in the form of improved orbits, improved measurement corrections, improved waveform tracking, and so forth. This leads to the GDRs being re-released to the science team. For Jason-1, the data processing team has recently moved from GDR-B processing to GDR-C processing, the same standards as used with the new Jason-2 data. The effect of this change from GDR-B to GDR-C data has been more significant than expected, however. Each improvement in the GDR data releases moves the scientific community closer to creating a Climate Data Record (CDR) for sea level change.
One of the early results of the Jason-1/TOPEX tandem phase was an improvement of the sea state bias (SSB) models for both TOPEX (CitationChambers et al. 2003b) and Jason-1 (CitationLabroue et al. 2004). Once the data were corrected for these new SSB models, there were no significant residuals biases between the two altimeters that were correlated with significant wave height (CitationChambers et al. 2003a). However, a new SSB model was introduced for the GDR-B re-processing of Jason-1, based on longer spans and retracked data, using the techniques of CitationLabroue et al. (2004). This SSB model, however, caused both a global bias shift as well as much larger regional biases between the TOPEX and Jason-1 GDR-B data (). These bias changes were clearly correlated with significant wave weight (). When this was identified, a new SSB model was created for the Jason-1 GDR-C processing. This model removed both the regional patterns in the relative bias () as well as the wave-height dependence ().
Figure 1 Geographic mean differences during the tandem missions between T/P vs Jason-1 (GDR-C) (top left), T/P vs Jason-1 (GDR-B) (top right), Jason-1 (GDR-C) vs Jason-2, and Jason-1 (GDR-B) vs Jason-1 (GDR-C).
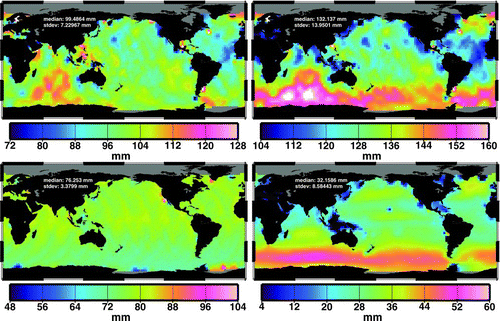
Figure 2 T/P, Jason-1, and Jason-2 intermission measurement biases binned into different ranges of Significant Wave Height (SWH).
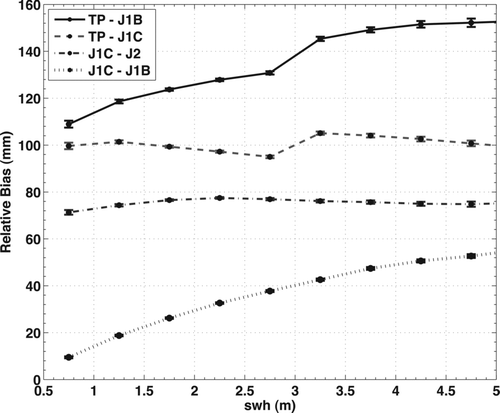
These SSB errors mainly contributed to a global bias change between TOPEX and Jason-1 of 36 mm, although this was able to be removed from global mean sea level studies by computing the mean relative bias during the overlap (e.g., CitationLeuliette et al. 2004). However, another problem in the Jason-1 GDR-B data led to bias changes that were undetected for several years until GDR-C reprocessing. The bias changes are caused by thermal shock to the Jason-1 radiometer (e.g., CitationBrown et al. 2004; Brown et al. this issue). Jason-1 has had a history of significant bias changes in the wet path delay computed from the Jason Microwave Radiometer (JMR). At the first Ocean Surface Topography Science Team Meeting, JMR engineers reported two significant anomalies in the instrument. In November 2002, the path delay correction became biased by about 3–4 mm. In November 2003, it became biased again by another 7–8 mm in the same direction. This error created an offset of more than 1 cm, and it was several years before correction products were available to fix the problem. At the same time, a new calibration algorithm was developed (Brown et al. this issue) to find such jumps and correct them in the GDR processing. During GDR-C reprocessing, it was discovered that another JMR bias change had occurred in early 2006 (), which caused a substantial impact on global mean sea level computed from GDR-B data. This bias error was large enough to cause GMSL from GDR-B data to be nearly 1 mm/year too high for the period from July 2003 until June 2007, which partially explains the misclosure of the sea level budget between Jason-1, GRACE, and Argo (CitationWillis et al. 2008; CitationLeuliette and Miller 2009).
Figure 3 Global mean sea level differences between the GDR-B and GDR-C processing of Jason-1 altimeter data.
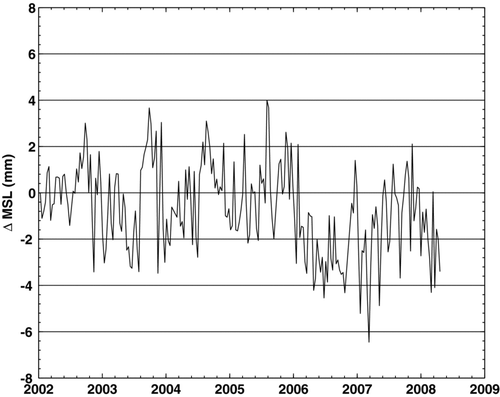
For nearly all sea level studies, but especially studies of global mean sea level, it is important to correct the altimeter data for relative measurement biases (), where the biases are computed using difference in GMSL as described in CitationLeuliette et al. (2004). Neither the changes in the SSB models nor the calibration of the radiometer have reduced the overall bias between Jason-1 and TOPEX (). Changing the SSB model for Jason-1 does move the relative bias up or down by several cm; however, it does not completely remove it. For GDR-C processed Jason-1 data, we computed the average cycle-per-cycle differences in GMSL with respect to T/P for Jason-1 cycles 1–21 and determined that there is a 99.6 ± 1.3 mm bias (90% confidence) between T/P and Jason-1 (Jason-1 SSH biased high). Similarly, the average cycle-per-cycle GMSL difference between Jason-1 and Jason-2 for Jason-2 cycles 1–20 suggests that the bias between Jason-1 (GDR-C processing) and Jason-2 is 75.3 ± 0.6 mm (Jason-2 SSH biased high), despite the fact that the Jason-2 altimeter is an exact duplicate of the Jason-1 altimeter. All independent assessments of the TOPEX absolute bias suggest it is near zero (e.g., CitationHaines et al. 2003). We can find no correction that accounts for the full 75 mm bias, although biases in the ionosphere and wet troposphere corrections can account for up to 5 mm of the bias. The dominant cause has to be a range bias in the altimeter. The fact that two identical altimeters can have a bias that differs by more than 7 cm underscores the critical nature of having these tandem mission calibration/validation periods, so that such biases can be precisely determined. Using T/P as a reference, we applied a 100 mm bias to the Jason-1 data and a 175 mm bias to the Jason-2 data. The source of the bias between Jason-1 and Jason-2 is mainly a combination of: 1) differences in the truncation of the pulse repetition frequency (PRF) used in the ground processing and 2) differences in the Ku-band characterization parameters (CitationDesjonqueres 2009), but a residual bias of 12 mm is still unexplained. The source of the relative bias between T/P and Jason-1 is currently unknown.
3. Tide Gauge Calibration
Monitoring of the instruments once they are on orbit is a critical piece of the data calibration. The basic method for making the tide gauge calibration is described by CitationMitchum (1998), and subsequent improvements and the addition of corrections for vertical land motion at the tide gauges are detailed by CitationMitchum (2000). Early in the T/P mission, the tide gauges successfully captured the so-called algorithm error before this error was actually discovered (CitationMitchum 1998). This error was relatively large and therefore not a stringent test of the method, but more subtle errors due to radiometer drift were also suggested by the tide gauge analysis at the same time. These smaller drift errors were subsequently confirmed by independent measurements (CitationKeihm et al. 2000). Over time, these comparisons of altimeter heights and tide gauge sea levels have become an important tool used after launch for improving the altimetric measurements of global sea level change. Note carefully that this method does not replace the need for overlap between missions and the direct determination of vertical offsets such as those detailed above. The tide gauge calibration is, however, extremely valuable for quantifying time-dependent errors (i.e., drift errors) in the altimetric time-series.
For the purposes of this paper, we need to briefly review several aspects of the tide gauge calibration method. Quantitative results relevant to the global sea level variations will be given in the next section. First, the dominant rate error in the tide gauge calibration is the uncertainty arising from imperfect knowledge of the land motion rates at the tide gauges. Because the tide gauges measure sea level relative to the adjacent land and are not typically known in an absolute reference frame, one must make land motion corrections in order to make absolute ocean height measurements. When the altimetric and tide gauge heights are differenced, land motions and altimeter drift cannot be separated, meaning that the land motion uncertainty places a limit on our ability to determine altimeter drift rates. At present, this error is estimated to be 0.4 mm/yr (CitationMitchum 2000), and this error must be added to the error obtained from fitting trends to the altimeter height series, which are typically much smaller. In determining the rate error estimate on sea level changes, the land motion errors and the fitting errors are assumed to be independent. Second, the tide gauge method not only provides a drift time-series but also gives a time-series of the errors in these estimates by propagating the errors at each tide gauge through to the final globally averaged drift estimate series. These errors can then be compared to the scatter in the altimetric time-series of global sea level as a check on both measurement systems and the tide gauge method itself. Third, the altimeter, tide gauge difference series are corrected for tide model errors by removing variance at the tidal alias frequencies. Differences at the tidal frequencies are expected to arise from errors in the model used to correct the altimetric heights and also from local deformations of the tidal signals near topography, which is where the tide gauges are. The specific tidal components that are fit and removed from the difference series depends on the length of the time-series. This means that shorter records are expected to have somewhat higher noise than longer ones, which is especially relevant for Jason-2.
4. Global Mean Sea Level Variations
The bias-corrected global mean sea level computed from all three missions is shown in . As discussed in previous studies (e.g., CitationChen et al. 1998; CitationNerem et al. 1999; CitationLeuliette et al. 2004), there is a regular annual variation associated with the ocean thermal and water cycles. Removing the seasonal variations () reveals significant interannual variations that are correlated with El Nino and La Nina events (). There is a significant trend in the time-series of 3.1 mm/yr. If a correction is applied for the changing shape of the ocean basins due to glacial isostatic adjustment (GIA) (0.3 mm/yr), the rate of sea level rise is 3.4 mm/yr. Note that GMSL has been lower than the mean rate since the end of 2005. Until late 2005, the rate was 3.7 mm/yr; since then it has been 2.3 mm/yr, although sea level recovered to the mean trend near the end of the time series. We believe this is due to the recent La Nina event. As evidence, we show a comparison in between the detrended global mean sea level variations and the Multivariate ENSO Index (MEI). The correlation is significant, at the 95% level. This could be caused either by a change in ocean heat content associated with ENSO or by a change in land/ocean precipitation patterns during ENSO. In any case, we do not believe that the recent lower sea level values are representative of a change in the long-term trend of sea level rise. It is simply a result of the large interannual variations in sea level that have been observed over the entire length of the altimeter sea level record.
Figure 5 Global mean sea level variations from three different altimeter missions after the application of intermission measurement biases (0.3 mm/year GIA correction applied).
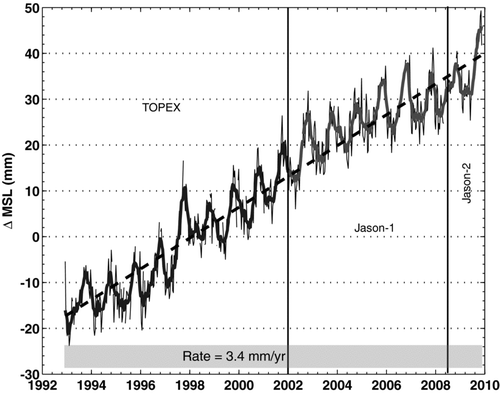
Concerning the uncertainties in these quantities, first, the error bar assigned to the sea level change rates is 0.4 mm/yr. This is due almost completely to the uncertainty in tide gauge calibration of drift. Formal errors based on point-to-point uncertainty or the variance of residuals is typically less than 0.1 mm/yr. This is consistent with recent independent estimate of the uncertainty based on studies of the uncertainty of the corrections and orbits that are used for the sea level calculation (CitationAblain et al. 2009). The residuals from the best fit trend in have a standard deviation of 4.0 mm. The log-log spectrum of the residuals is quite linear with a slope on the order of -0.75, which is nearly white statistically. Even after correcting for serial correlation, the error of the fit is less than 0.1 mm/yr, which is insignificant compared to the 0.4 mm/yr error associated with the drift estimation discussed above. The spectrum of the residuals (not shown) does not show significant peaks, although the semidiurnal tidal alias of about 60 days shows slightly elevated variance levels.
If we simultaneously fit the global series to the MEI in addition to the seasonal variations and the trend, the variance of the residuals drops to 3.7 mm, which is a 16% reduction in the variance. Although this variance reduction is not large, it is highly significant statistically, and this supports our conclusion concerning both the importance of interannual variations and the identification of El Nino and La Nina events as the dominant signal.
shows a comparison of global mean sea level between TOPEX, Jason-1, and Jason-2 during their respective tandem periods. For TOPEX and Jason-1, the rms difference of the two time series is 3.5 mm. For Jason-1 and Jason-2, there is better rms agreement at 1.3 mm, probably because the spacecraft and instruments are identical. For TOPEX and Jason-1, the differences are probably mainly due to orbit errors, in addition to differences in the wet troposphere corrections.
Figure 8 Comparison of TOPEX and Jason-1 global mean sea level (left–RMS difference of 3.5 mm) and Jason-1 and Jason-2 global mean sea level (right–RMS difference of 1.3 mm) during their respective tandem periods.
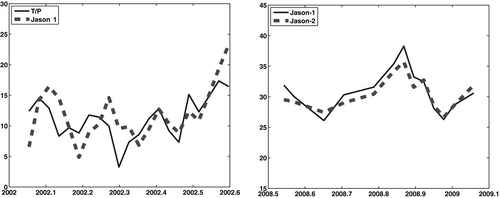
Turning to the tide gauge drift estimation for our global mean sea level series, the difference series has a trend of −0.04 mm/yr with an error of 0.4 mm/yr. This (statistically zero) trend is interpreted to mean that the offsets we have determined between TOPEX and Jason-1 and between Jason-1 and Jason-2 are trustworthy since an error in the offset parameter would lead to a trend in the altimeter minus tide gauge differences. The drift series for the entire record has a random error of 4 mm and an average error due to land motion uncertainty of about 2 mm. This leads to a total error that is only slightly smaller than the 5.8 mm scatter in the drift estimate for the complete time series. This consistency between the propagated errors in the tide gauge calibration and the scatter in the final time series increases our confidence in our error estimates. The cycle to cycle errors are slightly larger for the Jason-only series (). This is due to the smaller number of tidal corrections applied to these shorter series, as discussed above. The log-log spectrum of the complete drift time-series is quite linear, as is the spectrum of the residuals from the global mean sea level curve, albeit with a slightly lower slope of −0.5. This difference in the spectral slopes is not statistically significant, but does suggest additional sources of low frequency error in the mean sea level time series that are the topic of ongoing investigation.
Finally, we also computed sea level trends based on 4-year-long subsets of the mean sea level curve shown in . We chose a random time, drew a 4-year long series centered at this time, computed the trend, and repeated this process 100 times. This resampling (with replacement) calculation leads to a mean trend of 3.0 mm/yr with a standard deviation of 1.0 mm/yr. Although the series is short for this type of simulation, the calculation certainly supports our assertion above that the decrease in the global sea level change rate over the past four years is not significant given the level of interannual variability observed in the complete time series.
5. Conclusions
A continuous series of satellite altimeter sea level measurements from the T/P, Jason-1, and Jason-2 missions have been calibrated and assembled to form a climate data record for sea level change. They show that sea level has been rising over the last 17 years at a mean rate of 3.4 ± 0.4 mm/yr after corrected for glacial isostatic adjustment (GIA). However, there is considerable interannual variation due to ENSO processes, so the rate average over any individual four-year period can be significantly different. A longer time-series is required to definitely show that sea level rise is accelerating.
References
- Ablain , M. , Cazenave , A. , Guinehut , S. and Valladeau , G. 2009 . A new assessment of global mean sea level from altimeters highlights a reduction of global slope from 2005 to 2008 in agreement with in situ measurements . Ocean Sciences , 5 : 193 – 201 .
- Beckley , B. D. , Lemoine , F. G. , Luthcke , S. B. , Ray , R. D. and Zelensky , N. P. 2007 . A reassessment of global and regional mean sea level trends from TOPEX and Jason-1 altimetry based on revised reference frame and orbits . Geophys. Res. Lett. , 34 : L14608 doi: 10.1029/2007GL030002
- Brown , S. , Ruf , C. , Keihm , S. and Kitiyakara , K. 2004 . Jason-1 microwave radiometer performance and on-orbit calibration . Mar. Geodesy , 27 : 199 – 220 .
- Chambers , D. P. , Ries , J. C. and Urban , T. J. 2003a . Calibration and verification of Jason-1 using global along-track residuals with TOPEX . Marine Geodesy , 26 : 305 – 318 .
- Chambers , D. P. , Hayes , S. A. , Ries , J. C. and Urban , T. J. 2003b . New TOPEX sea state bias models and their effect on global mean sea level . J. Geophys. Res. , 108 ( C10 ) : 3305 doi: 10.1029/2003JC001839
- Chen , J. L. 1998 . Seasonal globe water mass budget and mean sea level variations . Geophys. Res. Lett. , 25 : 3555 – 3558 .
- Desai , S. D. and Haines , B. J. 2004 . Monitoring measurements from the Jason-1 microwave radiometer and independent validation with GPS . Mar. Geodesy , 27 : 221 – 240 .
- Desjonqueres , J.-D. POSEIDON3 instrument investigations corrections and upgrades . Ocean Surface Topography Science Team (OSTST) Meeting . June , in Seattle, Washington.
- Haines , B. J. , Dong , D. , Born , G. H. and Gill , S. K. 2003 . The Harvest experiment: Monitoring Jason-1 and TOPEX/Poseidon from a California offshore platform . Marine Geodesy , 26 : 239 – 260 .
- Keihm , S. J. , Zlotnicki , V. and Ruf , C. S. 2000 . TOPEX microwave radiometer performance evaluation, 1992–1998 . IEEE Trans. Geosci. Remote Sensing , 38 : 1379 – 1386 .
- Labroue , S. , Gaspar , P. , Dorandeu , J. , Zanife , O. Z. , Mertz , F. , Vincent , P. and Choquet , D. 2004 . Nonparameteric estimates of the sea state bias for the Jason-1 radar altimeter . Mar. Geodesy , 27 : 453 – 483 .
- Leuliette , E. , Nerem , R. and Mitchum , G. 2004 . Calibration of TOPEX/Poseidon and Jason altimeter data to construct a continuous record of mean sea level change . Mar. Geod. , 27 : 79 – 94 .
- Leuliette , E. W. and Miller , L. 2009 . Closing the sea level rise budget with altimetry, Argo, and GRACE . Geophys. Res. Lett. , 36 : L04608 doi: 10.1029/2008GL036010
- Mitchum , G. T. 1998 . Monitoring the stability of satellite altimeters with tide gauges . J. Atmos. Oceanic Tech. , 15 : 721 – 730 .
- Mitchum , G. T . 2000 . An improved calibration of satellite altimetric heights using tide gauge sea levels with adjustment for land motion . Marine Geodesy , 23 : 145 – 166 .
- Nerem , R. S. , Chambers , D. P. , Leuliette , E. W. , Mitchum , G. T. and Giese , B. S. 1999 . Variations in global mean sea level associated with the 1997–1998 ENSO event: Implications for measuring long term sea level change . Geophysical Research Letters , 26 ( 19 ) : 3005 – 3008 .
- Obligas , E. , Tran , N. and Eymard , L. 2004 . An assessment of Jason-1 microwave radiometer measurements and products . Mar. Geodesy , 27 : 255 – 277 .
- Willis , J. K. , Chambers , D. P. and Nerem , R. S. 2008 . Assessing the globally averaged sea level budget on seasonal to interannual time scales . J. Geophys. Res. , 113 : C06015 doi: 10.1029/2007JC004517, 2008
- Zlotnicki , V. and Desai , S. D. 2004 . Assessment of Jason microwave radiometer's measurement of wet tropspheric path delay using comparisons with SSM/I and TMI . Mar. Geodesy , 27 : 241 – 253 .