Abstract
A hindcast simulation of storm surge and inundation from tropical cyclone Phalin, which made landfall at Odisha, India, on 12 October 2013, was carried out using ADCIRC model. Model-simulated inundation extent matched well with field surveys at Ganjam, Odisha, within a few days of landfall. Further, the model reproduced the temporal evolution of the surge residual with respect to observations from a tide gauge at Paradip (correlation 0.8, RMSE 0.26 m). However, the model marginally underestimated the magnitude with respect to observations, which can be attributed to the lack of wave setup in the model and uncertainty in wind and pressure information. The experiment also involved the use of two idealized scenarios, that is, variation of landfall timings with the ebbing and high tide phase. These scenarios were required for better understanding the sensitivity of inundation to the phase of tide in the model. Simulation with landfall at flooding (ebbing) tide showed greater (lower) inundation than the real scenario. Results from idealized scenarios confirmed the significance of the accuracy needed in forecasting landfall time. Our results clearly indicate that the overall performance of the model is good and therefore is of potential use as a tool to forewarn disaster management authorities.
Introduction
Cyclone Phailin was one of the strongest tropical cyclones ever recorded over the North Indian Ocean that made landfall at Odisha coast during October 2013 with a maximum wind speeds of 200 kmph. It was the strongest cyclone after the Orissa Super Cyclone that made landfall in Odisha in 1999. Phailin originated from the South China Sea from remnant cyclonic circulation and entered the Andaman sea on 6 October 2013. It intensified rapidly and became a Very Severe Cyclonic Storm on the forenoon of 10 October 2013. Phailin crossed the Odisha coast near Gopalpur (Odisha) around 1600 UTC on 12 October 2013 with a maximum surface wind speed of 200–210 kmph. shows the observed track of Phailin. Accurate track forecasts provided by the India Meteorological Department (IMD) and thorough evacuation efforts by the Odisha government saved many lives during Phailin.
Tropical cyclones heavily impact Indian coasts at least once a year. They have potentially devastating consequences for coastal communities. A surge and its associated inundation caused by a cyclone that makes landfall is a serious concern along the coast. A thorough review of this problem of storm surges in the Bay of Bengal was carried out by many workers (Rao et al. Citation1994; Murty et al. Citation1986; Gönnert et al. Citation2001; Dube et al. Citation1997; Dube et al. Citation2000). Salek Citation(1998) explained the widespread nature of surges in the Meghana estuary due to coastal trapping and the prevalent funneling effect. Cyclone-induced surge and its associated inundation can be particularly high if the time of landfall coincides with high tide conditions. Similarly, the impact could be less if the time of landfall coincides with low tide conditions. Flather Citation(1994) concluded that the timing of cyclone landfall and its coincidence with high tide determined the area affected by flooding during 1991 Bangladesh cyclone. Proudman (Citation1955a, Citationb) theoretically examined the interaction between tide and surge along an estuary. Banks Citation(1974) illustrated that nonlinear effects involving tide and surge may be significant in the entire North Sea region. Tide–surge interactions contribute to the total storm tide height at the coast (Johns et al. Citation1985). Lewis et al. Citation(2013b) studied the flood risk inundation in the Northern Bay of Bengal using freely available data sources. In their study they explained the importance of publicly available data for use in coastal flood risk management in data-poor regions. The study of Flather Citation(1994) showed the overall significance of accurate predictions of cyclone track, cyclone development, landfall time, and their significance in areas with large tidal range.
Many reports corresponding to different global ocean basins describe casualties and damage to life and property caused by coastal flooding (Nicholls et al. Citation1999). The problem of storm surges should be addressed by prone countries combining their efforts in an integrated manner. About 90% of damage to life and property is caused by inland intrusion of seawater. Flierl and Robinson Citation(1972) documented many cases where the occurrence of abnormally high sea levels in the Bay of Bengal led to coastal flooding and inundation. The study of Murty et al. Citation(1984) discovered that 60% of the deaths due to storm surges occurred in the low-lying coastal areas of countries bordering the Bay of Bengal.
The inundation due to surge is high if the time of landfall coincides the high tide condition. Though, thorough assessment of coastal flood risk requires prediction of water levels and inundation extent (Dawson et al. Citation2003), no modeling studies were heretofore available for the east coast of India which examined the sensitivity of inland inundation to phase of the tide during cyclone landfall. The aim of this study is to simulate and examine the surge heights and inundation extents due to cyclone Phailin and understanding the effect of tidal phase relative to cyclone landfall time on inland inundation extent. We have modeled the storm surge and its associated inland inundation due to cyclone Phailin using Advanced Circulation (ADCIRC) model. In addition, we have examined the change in surge height as well as inundation extent with idealized scenarios by varying landfall times prior and after by 3 hours to the actual time of landfall. The results of simulations and comparisons with available observations are presented in subsequent sections.
Numerical Model
The storm surge and its associated inland inundation extent due to Phailin were modeled using the ADCIRC model (Luettich et al. Citation1992). This model has a proven capability to simulate storm surge propagation in a complex coastal environment with a high degree of accuracy. Additional capabilities include a wetting and drying algorithm to study inland inundation of water from storm surge. Considering various case studies, the U.S. Federal Emergency Management Agency (FEMA) in 2002 accepted the robustness of ADCIRC. The development of ADCIRC was a joint effort of the U.S. Army Corps of Engineers, the University of North Carolina, and the University of Notre Dame (Luettich et al. Citation1992; Luettich and Westerink Citation1991; Westerink and Luettich Citation1991; Westerink and Gray Citation1991). ADCIRC has the flexibility of allowing high grid resolutions in the nearshore that relax away from the shore line. This finite element code also allows computations in a distributed grid environment. A detailed description of the finite-element-based hydrodynamic model ADCIRC-2DDI is available in Luettich et al. Citation(1992).
Wind Forcing Module
The surface wind field associated with a tropical cyclone is the primary requirement for modeling of storm surges. Most meteorological models are not yet available at high resolution to provide accurate wind fields for storm surge computations (Jason et al. Citation2008) where as parametric wind models produce comparable storm surges in many cases (Houston et al. Citation1999; Mattocks et al. Citation2006). Considering the same, a dynamic storm model (Jelesnianski and Taylor Citation1973) was used in the present study to derive the wind fields at the sea surface. Complete description of the model can be obtained from Jelesnianski and Taylor (1973), NOAA technical memorandum.
Study Area
The modeling domain covers the coastal stretch of Northern Andhra Pradesh and Odisha, which includes the study area (area affected by Phailin). The Odisha coast of India is one of the most vulnerable coasts in India, with extremely high sea levels associated with severe tropical cyclones. According to IMD's reports, the coastal stretch of India between Paradip and Balasore in Odisha state was classified as a very high risk zone. The October 1999 Super cyclone which made landfall near Paradip resulted in widespread loss of life and property. Flooding in this region is predominantly due to the presence of deltas formed by rivers such as Mahanadi and also due to the high tidal range. Ebbing and flooding tidal phases influence the height, duration and arrival time of a peak surge along the coast. The present study was configured to the part of Odisha coast in order to compute the surge height and its associated inland extent of inundation due to the cyclone Phailin. depicts the model domain and grid that was used for the present study.
Methodology
Bathymetry and topography data was obtained from General Bathymetric Chart of the Ocean (GEBCO, http://www.gebco.net) with a grid spacing of 30 arc seconds from the British Oceanographic Data Centre (BODC). GEBCO 30 arc seconds data has been chosen because of its high resolution. An irregular triangular gridded mesh was generated using a software package Surface water Modeling System (SMS). The mesh contains 307,136 nodes and 612,210 elements with a minimum grid spacing of 50 m along the coast. A 15m height contour was considered as a vertical sidewall (rigid boundary) in order to see the extent of inland inundation from the coastline, presuming that the storm surge height will not exceed 15 m. The grid covers an offshore length of about 930 km as seen in . It was understood that higher resolution grids are critical at the coast, especially in areas of shallow bathymetry and topography (Blain et al. Citation1994; Luettich et al. Citation1995) in order to get better estimates of surge heights. Hence, the resolution of model grids was enhanced in shallow waters to obtain better estimates of tides and storm surges near the coast and was relaxed in deeper waters to optimize computation time. is the enlarged version of for the Gopalpur region, where the cyclone made its landfall. It can be clearly seen from this figure that the grid resolution was enhanced along the coastline and relaxed both onshore and offshore, away from the coastline.
The water level along the open boundary was obtained from the Le Provost tidal data base and was represented by 13 tidal constituents (K1, M2, N2, O1, P1, S2, K2, L2, 2N2, MU2, NU2, Q1, and T2) based on Finite Element Solution (FES) Version 95.2 (Le Provost et al. Citation1998). FES95.2 solutions is a 0.5° × 0.5° gridded version of the full finite element solutions.
Parametric wind models produce comparable storm surges in many cases. They are also advantageous in providing high resolution wind field and barometric pressure values at arbitrary locations with a very small amount of input data. Considering the same, the dynamic storm model (Jelesnianski and Taylor Citation1973) was used that uses the storm data from IMD to generate the surface wind field. Model integration was carried out for 72 hours and was performed with a High Performance Cluster (HPC) IBM, 9125-F2A system available at ESSO-INCOIS, having a Processor Clock Speed of 4704; 256 processors of HPC were used for the present simulation. The computational clock time for the simulation was about 35 minutes. Model-simulated tide and water levels were validated against the observed water levels recorded by the tide gauge at Paradip, Odisha, which is the nearest available tide gauge station. The total water level due to a storm is a combination of wind stress associated with the storm and the astronomical tide forcing.Therefore, the predicted tide has been subtracted from the observed and the model computed total water levels, in order to estimate contributions to sea level due to the storm, that is, meteorological forcing. Inter-comparison between the observed and model computed residuals was carried out at the Paradip tide station location. Field survey was done using GPS and measured the horizontal extent of inundation from the coastline. Model-simulated inland inundation extent at 29 different points was validated against field survey records. Idealized scenarios were also examined by varying landfall timings relative to tide, in order to better understand the sensitivity of inland inundation to the phase of the local tide in the study region. In Scenario-1, cyclone landfall was made 3 hours prior (ebbing tide) to the actual time of landfall. In Scenario-2, cyclone landfall was made 3 hours after (near high tide) the actual time of landfall.
Results
Cyclone Phailin crossed the South Odisha coast near Gopalpur, around 1600 UTC on 12 October 2013. The model integrated the 72 hours that preceded Phailin's landfall. The model-computed maximum wind speed associated with the cyclonic storm was about 60 m/s. IMD reported that the observed maximum wind speed was 58 m/s and the observed maximum central pressure drop was 66 hpa. The maximum storm tide (surge + tide) computed by the model at Ganjam, Odisha was 2.3 m above the mean sea level. Spatial depiction of the model-computed storm tide due to Phailin is given in and its enlarged view which shows the inundation extent at the Ganjam region is shown in . It is evident from that a vast coastal stretch was affected by storm tides greater than 1 m, primarily on the right-side of the track. Temporal evolution of storm tide and residual water level (water level after removing the tide component from storm tide) at Ganjam is shown in . Unfortunately there was no observational water level data available at Ganjam.
Figure 3. (a) Model computed storm tide (surge + tide), (b) Enlarged view of figure-3a near Ganjam, shows the inundation extent, (c) Temporal evolution of model computed surge residual (storm tide–tide) in meters at Ganjam. Red thick line is the track of Phailin.
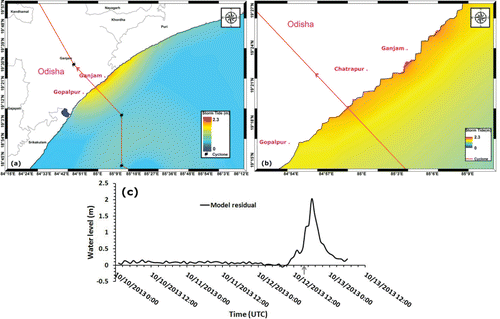
Since there was no tide gauge station in the close vicinity of Ganjam, a comparison of model-computed water levels was carried out using observations from the tide gauge station at Paradeep, which is about 230 km from the landfall point. shows the comparison of modeled and observed storm tide at Paradeep. It is clear that the model simulated water levels fairly match trends seen in the observed water levels. Also from , we can see that the landfall occurred during rising tide which had an amplitude of −0.3 m. The inter-comparison of observed and modeled residual water levels is shown in . A residual maximum of 0.45 m was estimated by the model as compared to the observed value of 0.67 m. From it is evident that model-computed water levels remained close to observed levels till the afternoon of 11 October and thereafter, a small underestimation occurred in the model simulation.
Figure 4. (a)Comparison of model computed storm tide in meters with that of observed at Paradeep. Arrow line indicates the land fall time, (b) Comparison of modeled residual with that of observed at Paradeep. Arrow line indicates the landfall time.
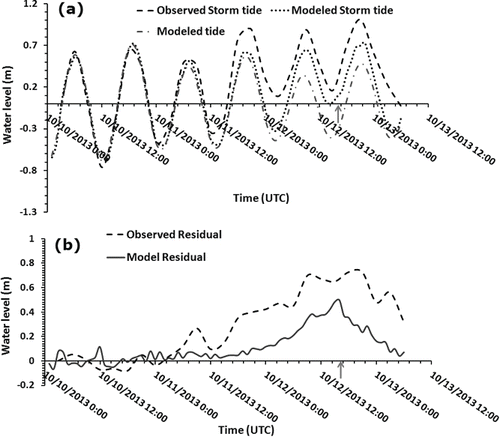
Statistics between modeled and observed residuals revealed a correlation of 0.81 (with a confidence level of more than 95%) and RMSE of 0.21. The forecast parameters with Scatter Index (SI) of 11% was found and SI of less than 30% is widely accepted by user community for operational planning (Woodcock and Greenslade Citation2007). The observed and modeled residuals were tabulated in . Comparison of modeled and observed residuals at Paradeep in general, follows the trend closely. It is further observed from that the water level of 2.3 m resulted in a maximum inundation extent of about 430 m near Ganjam. The modeled inundation extent varied between 50 m and 430 m and it increased in areas where the topographical slope is less than 1:100. Poststorm surveyed data at 29 coastal locations were used to validate the model simulated inundation extent. Comparison of model-simulated inundation extent with observed values are shown in .
Table 1 Comparison of modeled and observed surge residuals due to Phailin.
Table 2 Validation of modeled inundation extent with field observations (inundation extent is the horizontal distance in meters from the zero contour line)
Though cyclone Phailin made landfall during rising tide, we examined the sensitivity of inland inundation to the phase of local tide in the study region. A couple of idealized scenarios were performed by varying landfall timings relative to the phase of local tide. In Scenario 1 (3 hours before the actual time of landfall), landfall was made during ebbing tide of amplitude of −0.4 m. In Scenario 2 (3 hours after the actual time of landfall), landfall was made during a period near high tide with an amplitude of around 0.4 m. depict the inundation extents computed by the model with Scenario 1, actual scenario, and computations in Scenario 2, respectively. Inter-comparison of model-computed inundation extents with actual case and idealized scenarios are given in .
Figure 5. Spatial depiction of inundation extent (a) with cyclone had its landfall by 3 hours prior to the actual time of landfall, (b) with actual time of landfall, (c) with cyclone had its landfall by 3 hours after the actual time of landfall.
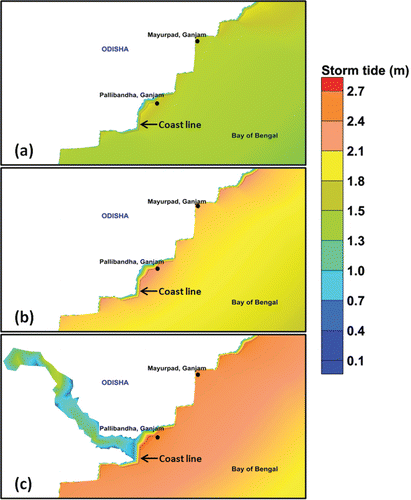
Table 3 Inter-comparison between modeled inundation extent with actual landfall time of Phailin and with idealized scenarios.
Discussion
The modeled surge heights matched satisfactorily with observations with the correlation of 0.81 (with a confidence level of more than 95%) and RMSE of 0.21; however, a marginal underestimation was observed. shows that the model underestimated surge residual from the afternoon of 11 October. Balakrishnan Nair et al. (2014) recently reported in their study that the wave heights due to Phailin drastically increased from the afternoon of 11 October. As the wave information is not provided in the model which leads to underestimation in surge residual from that instance. The study of Arujo et al. Citation(2011) confirmed that the inclusion of radiation stress gradient due to breaking waves, in addition to atmospheric pressure and surface wind drag, was critical for the improvement of model performance. In addition, the underestimation in residual may also leads from the possible errors in near shore bathymetry, wind and pressure fields of the cyclone. The studies of Lewis et al. (Citation2014, 2013b) noticed that the natural variability of cyclone parameters translates into large uncertainty both for storm surge height and inundation extent. Moreover, the Paradeep tide gauge station is located 230 km away from the cyclonic eye and the model winds may be relatively weak at that distance. It was noticed from Figures 3c and 4b, there was a 2-hour difference in occurrence time of peak surge at Ganjam and Paradeep, which could be due to the orientation of the coastline with respect to the storm path. It was also observed that the residual maxima occurred during the transition period from low to high tide. Similarly feature was observed in the study of Horsburg and Wilson Citation(2007).
The modeled inundation extent matched satisfactorily with observations, however small differences were observed at few locations. These might be attributed to the absence of wave information in the model, possible errors in wind and pressure fields, errors in near shore topography and the absence of precipitation information to the model. The Lewis et al. (2013) study enlightens the importance of spatially fine peak storm tide heights that include wave effects, for flood risk managers. It can be noted from that there is a considerable difference in model-computed inundation extent with actual and idealized runs. Model-computed maximum inundation of about 5.6 km through the Rushikulya River in the Ganjam district is observed in Scenario 2. From Scenario 2 it may be deduced that if landfall occurred around 2000 UTC of October 2014, then the inland intrusion of water would have been greater and may have reached across the banks of the river, causing the villages alongside to be inundated. Scenario 2 results also indicates the importance of predicting landfall time relative to the phase of tide especially in river deltaic regions. While there was no significant change in inundation extent in areas of steep slope, the overall inundation extent was greater in Scenario 2. The overall performance of the model in simulating the surge and its associated inundation extent was good and thus it is recommended that the model may be used for operational generation of forecasts. Furthermore, the future work comprises (i) inclusion of wave radiation stresses in the surge model to investigate additional effect of wave set-up on surge heights and inundation extent and (ii) identifying whether resolving waves or accurately prescribing wind-fields is the most important process for improving storm surge modeling in the study region. This paper also focuses on inundation extent due to surge heights, whilst accurate inundation forecast requires high resolution topography data. Therefore, future work should explore the contribution of topographic data accuracy in modeling of inundation extent.
Conclusions
The storm surge impact of the cyclone Phailin on coastal areas was subdued since the cyclone made landfall during low tide conditions. Though phase of the model simulated surge heights matched well with that of observed surge heights, a marginal underestimation was seen in amplitude from the afternoon of 11 October 2014. This may be attributed to the lack of wave setup information in the model simulation and possible errors in near shore bathymetry. This could also be due to the uncertainty in wind and pressure fields measurements of the cyclone. Comparison of modeled inundation extent with field observations shows that the model results were matched satisfactory with observations. However, a marginal differences were found in inundation extent values which may mainly attributed to errors in onshore topography and lack of wave setup information in the model simulation. With the Use of high resolution data sets such as CARTOSAT, SRTM, ALTM, etc., we could resolve the errors raised due to the topography. Model results from idealized scenarios indicate the sensitivity of inundation extent to the time of landfall relative to phase of the tide. Results from idealized scenarios confirm the need of accuracy in prediction of landfall time relative to phase of the tide. Since SI between modeled and observed surge heights was found to be less than 30% and this can be widely accepted for operational planning. The overall performance of the model is promising and can thus be used as a tool for coastal disaster management authorities to draw suitable strategies to mitigate the effects of such events.
Acknowledgements
The authors are thankful to the Integrated Coastal and Marine Area Management Project Directorate team for conducting the post-storm survey for inland extent of inundation. Thanks are also due to the ADCIRC development team for providing the model. This is INCOIS contribution 231.
References
- Arujo, M. A. V. C., A. Mazzolari, and A. Trigo-Teixeira. 2011. Wave set-up in the modeling of storm surge at Viana do Castelo (Portugal). Journal of Coastal Research SI 64:971–975.
- Balakrishnan Nair, T. M., P. G. Remya, R. Harikumar, and K. G Sandhya. 2014. Wave forecasting and monitoring during very severe cyclone Phailin in the Bay of Bengal. Current Sciences 106(8):1121–1125.
- Blain, C. A., J. J. Westerink, and R. A. Luettich. 1994. The influence of domain size on the response characteristics of a hurricane storm surge model. Journal of Geophysical Research 99(C9):18467–18479.
- Banks, J. E. 1974. A mathematical model of a river-shallow sea system used to investigate tide, surge and their interaction in the Thames-southern North Sea region. Philosophical Transactions of the Royal Society 275:567–609.
- Dube, S. K., A. D. Rao, P. C. Sinha, T. S. Murty, and N. Bahulayan. 1997. Storm surge in the Bay of Bengal and Arabian Sea: The problem and its prediction. Mausam 48:283–304.
- Dube, S. K., P. Chittibabu, A.D. Rao, P. C. Sinha, and T. S. Murty. 2000. Sea levels and coastal inundation due to tropical cyclones in Indian coastal regions of Andhra and Odisha. Marine Geodesy 23:65–73.
- Dawson, R. J., J. W. Hall, P. B. Sayers, and P. D. Bates. 2003. Flood risk assessment for shoreline management planning. Proceedings of the International Conference on Coastal Management, Brighton, 15–17 October.
- Flather, R. A. 1994. A storm surge prediction model for the Northern Bay of Bengal with application to the cyclone disaster in April 1991. Journal of Physical Oceanography 24:172–190.
- Flierl, G.R. and A. R. Robinson. 1972. Deadly surges in the Bay of Bengal: Dynamics and storm-tide tables. Nature 239:213–215.
- Gönnert, G., S. K. Dube, T. S. Murty, and W. Siefert. 2001. Global Storm Surges: Theory, Observations and Applications. Heide in Holstein: Westholsteinische Verlagsanstalt Boyens & Co.
- Horsburg, K. J. and C. Wilson. 2007. Tide-surge interaction and its role in the distribution of surge residuals in the North Sea. Journal of Geophysical Research 112(C080030).
- Houston, S. H., W. A. Shaffer, M. D. Powel, and J. Chen. 1999. Comparisons of HRD and SLOSH surface wind fields in hurricanes: Implications for storm surge modeling. Weather Forecast 14(5):671–686.
- Jason, F., C. Fulcher, R. Luettich, B. Estrade, G. Allen, and H. Winer. 2008. A real time storm surge forecasting system using ADCIRC. Estuarine and Coastal Modeling 893–912.
- Jelesnianski, C. P., and A. D. Taylor 1973. NOAA Technical Memorandum. ERL WMPO-3, 33.
- Johns, B., A. D. Rao, S. K. Dube, and P. C. Sinha. 1985. Numerical modeling of tide–surge interaction in the Bay of Bengal. Philosophical Transactions of the Royal Society London A313:507–535.
- Le Provost, C., F. Lyard, J. M. Molines, M. L. Genco, and F. Rabilloud. 1998. A hydrodynamic ocean tide model improved by assimilating a satellite altimeter-derived data set. Journal of Geophysical Research 103(C3):5513–5529.
- Luettich, R. A. and J. J. Westerink. 1991. A solution for the vertical variation of stress, rather than velocity, in a three-dimensional circulation model. International Journal for Numerical Methods in Fluids 12:911–928.
- Luettich, R. A., J. J. Westerink, and N. W. Scheffner. 1992. ADCIRC: An advanced three dimensional circulation model for shelves coasts and estuaries, report 1: Theory and methodology of ADCIRC-2DDI and ADCIRC-3DL. Dredging Research Program Technical Report DRP-92-6, US Army Engineers Waterways Experiment Station, Vicksburg, MS.
- Luettich, R. A. and J. J. Westerink. 1995. Continental shelf scale convergence studies with a barotropic model. In Quantitative Skill Assessment for Coastal Ocean Models, Coastal and Estuarine Studies Series. Lynch, D. R. and A. M. Davies (eds.). pp. 349–371.
- Lewis, M., P. Bates, K. Horsburgh, J. Neal, and G. Schumann. 2013b. A storm surge inundation model of the northern Bay of Bengal using publicly available data. Quarterly Journal of the Royal Meteorological Society 139:358–369.
- Lewis, M., P. Bates, and K. Horsburgh. 2013a. Understanding the variability of an extreme storm tide along a coastline. Estuarine, Coastal and Shelf Science, 123:19–25.
- Lewis, M., K. Horsburgh, and P. Bates. 2014. Bay of Bengal cyclone extreme water level estimate uncertainty. Natural Hazards 72(2):983–996.
- Mattocks, C., C. Forbes, and L. Ran. 2006. Design and implementation of a real-time storm surges and flood forecasting capability for the state of North Carolina. UNC-CEP Technical Report. 30 November.
- Murty, T. S. 1984. Storm surges-meteorological ocean tides. Bulletin 212 Fish Research Board of Canada, Ottawa.
- Murty, T.S., R. A Flather, and R. F. Henry. 1986. The storm surge problem in the Bay of Bengal. Progress in Oceanography 16:195–233.
- Nicholls, R. J., F. M. J. Hoozemans, and M. Marchand. 1999. Increasing flood risk and wetland losses due to global sea-level rise: Regional and global analyses. Global Environmental Change 9:S69–87.
- Proudman, J. 1955a. The effect of friction on a progressive wave of tide and surge in an estuary. Proceedings of the Royal Society A 233:407–418.
- Proudman, J. 1955b. The propagation of tide and surge in an estuary. Proceedings of the Royal Society A 231:8–24.
- Rao, A. D., S. K. Dube, and P. Chittibabu. 1994. Finite difference techniques applied to the simulation of surges and currents around Sri Lanka and Southern Indian Peninsula. Computational Fluid Dynamics 3:71–77.
- Salek, J. A. 1998. Coastal trapping and funneling effect on storm surges in the Meghna estuary in relation with the cyclones hitting Noakhali-Cox's Bazar coast of Bangladesh. Journal of Physical Oceanography 28:227–249.
- Westerink, J. J. and W.G. Gray. 1991. Progress in surface water modeling. Reviews of Geophysics 29:210–217.
- Westerink, J. J. and R. A. Luettich. 1991, July. Tide and storm surge predictions in the Gulf of Mexico using model ADCIRC-2D. Report to U.S. Army Engineer Waterways Experiment Station.
- Woodcock, F. and D .J. M. Greenslade. 2007. Consensus of numerical model forecasts of significant wave heights. Weather Forecast 22:792–803.