ABSTRACT
The Newlyn Tidal Observatory is the most important sea level station in the United Kingdom. It commenced operations in 1915 as part of the Second Geodetic Levelling of England and Wales, and the mean sea level determined from the tide gauge during the first six years (May 1915–April 1921) defined Ordnance Datum Newlyn (ODN) which became the national height datum for the whole of Great Britain. The 100 years of sea level data now available have contributed significantly to many studies in oceanography, geology, and climate change. This paper marks the centenary of this important station by reviewing the sea level (and, more recently, detailed land level) measurements and Newlyn's contributions to UK cartography, geodesy and sea-level science in general. Recommendations are made on how sea and land level measurements at Newlyn might be enhanced.
1. Introduction
The end of April 1915 saw the start of sea level measurements by the Ordnance Survey (OS) at the Tidal Observatory at Newlyn on the tip of the Cornish Peninsula in Southwest England, about 10 km from Land's End. This tide gauge station has since provided a geodetic datum for the whole of Great Britain (Ordnance Datum Newlyn, ODN) and an important record of sea level change over a century that has been applied to research in geology, oceanography, and climate change. The purpose of this paper is to describe the Newlyn station, its equipment and the data sets that it has provided, to mark the anniversary of a station that is of fundamental importance to UK geodesy.
The Newlyn tide gauge was by no means the first in the United Kingdom. The extended measurement of high and low waters can be traced back to 1764 at Liverpool (Woodworth Citation1999), while the first automatic (or self-recording) tide gauges were installed at Sheerness and other locations in the Thames in the 1830s (Palmer Citation1831; Matthäus Citation1972). A number of UK tide gauge stations have records from before Newlyn commenced operations, and particularly interesting long records are to be found from Aberdeen, North Shields, Liverpool and Sheerness.
However, there are several aspects that make Newlyn special. One is a geodetic aspect, in its use in providing the ODN datum for the country during the Second Geodetic Levelling of England and Wales. Newlyn was chosen by the OS as the location for its south of England sea level measurements. That part of the Cornish coast was considered to be ideal, since it was thought to be a relatively stable area, having granite bedrock, and to be some distance from major rivers. The second is an oceanographic aspect, in that Newlyn is located nearer to the continental shelf edge than most other UK tide gauges. As a consequence, the sea level variations that its tide gauge records tend to be representative of those of the deep ocean, rather than the shallow waters of the North and Irish Seas, and to have fewer of the large storm surges that occur in shallower water areas. A third aspect is a practical one, in that it was installed, and has always been operated, by scientific organisations; the OS handed responsibility for the Newlyn tide gauge to the Natural Environment Research Council (NERC) in 1983. That has meant that the gauge has been better maintained than almost all others in the United Kingdom.
In section 2, we summarise some of the 19th century geodetic background which led to the establishment of the Newlyn Tidal Observatory. The Observatory itself and its technical history are discussed in section 3. The derivation of ODN, and its importance as a national datum are described in section 4. Section 5 gives an overview of the sea and land level records from Newlyn, emphasizing how levels have changed since ODN was established a century ago. Finally, section 6 points to how Newlyn must be maintained as one of the main UK tide gauges, with possible upgrades to its equipment in order to monitor sea level change effectively on all timescales.
2. Geodetic background
The 19th century saw the establishment of national height systems in many countries with the objectives of providing vertical reference levels, related to Mean Sea Level (MSL), for construction and mapping purposes, and of deriving basic scientific data for research into long-term crustal movements and changes in the level of the sea (Kelsey Citation1972). A height system required the use of tide gauge measurements to determine an MSL datum, and the transfer of that datum throughout the country by means of levelling.
The First Geodetic Levelling of England and Wales was one of the first of such national campaigns, with conventional spirit levelling undertaken during 1840–1860 to the standards of the time. A provisional datum was chosen as being 100 feet below a benchmark on St. John's Church, Liverpool. However, based on Sir George Biddell Airy's analysis of the tidal observations around the coast of Ireland in 1842 (Airy Citation1845; Pugh Citation1982), it was decided to instead adopt MSL as the datum plane to which all heights on Ordnance Survey maps should be shown (Jolly and Wolff Citation1922). Consequently, tidal observations were taken at the Victoria Dock, Liverpool, over a 10-day period during 7–16 March 1844 (measurements were made every 5 minutes for one-hour periods around high and low waters). The mean tide value over this period was found to be 43.14 feet above the provisional datum, and due to the uncertainty owing to the short observation period, this was rounded to an exact 43 feet, giving a convenient number to be subtracted from the provisional heights already determined.
Similar campaigns were undertaken in the 19th century in other European countries. Examples include the Netherlands, where the national datum is called Normaal Amsterdams Peil (NAP); and France, where the datum is Nivellement Général de la France (NGF), with the datums defined in terms of MSL measured at Amsterdam and Marseille, respectively. A particularly interesting tide gauge, along with a special building to house it, was constructed at Marseille (Coulomb Citation2014; Wöppelmann et al. Citation2014). In 1864, the International Association of Geodesy (IAG) requested maritime countries to undertake sea level observations at as many sites as possible and to link them by conventional spirit levelling, in order to connect the datums between national networks (Wöppelmann et al. Citation2014). This international activity would later be taken up by organizations such as the United European Levelling Network (UELN) (Rossiter Citation1967).
As standards and methods evolved, and in some cases where it was realized that changes in land levels were an important factor, it became necessary for national levelling campaigns to be repeated. In the case of the United Kingdom, while the conventional spirit levelling and datum of the First Geodetic Levelling had been adequate to control the mapping undertaken during the late 19th century, they were known by comparison to tidal measurements around the coast to be not as suitable as they should be. In addition, many of the benchmarks used were not as stable as required or had disappeared. Consequently, by 1911 it had become evident that a Second Geodetic Levelling, conducted to the then modern, conventional precise levelling standards and with more accurate sea level information, was necessary (the deficiencies in the First Geodetic Levelling are summarized in chapter 1 of Jolly and Wolff Citation1922).
The Second Geodetic Levelling was undertaken by the OS, with much reduced resources at a time of war, between 1912 and 1921, although the network was not extended to Scotland until as late as 1936–1952 (Kelsey Citation1972). This new campaign made use of a set of secure Fundamental Bench Marks (FBMs) installed in solid rock (rather than on buildings, etc., as before) approximately 30 miles apart at strategic points in the levelling network. A Third Geodetic Levelling took place throughout Great Britain during 1951–1959. These FBMs are still the physical realization of ODN today.
The OS also established three new tide gauges at Dunbar (in 1913), Newlyn (in 1915, and ) and Felixstowe (in 1917), with the intention of determining an average MSL datum for Great Britain by making an overall adjustment of the MSL measured at the three locations. The Dunbar and Newlyn Tidal Observatories were particularly solid stone constructions. All three were located at some distance from major river estuaries, unlike Liverpool in the First Geodetic Levelling.
Figure 1. (a) The 43 stations of the main present-day National Tide Gauge Network (also known as the “A Class Network”) shown by dots, indicating the location of Newlyn in SW England and some other long-term tide gauge stations mentioned in the text. Dunbar is not included in the present National Network and is shown by a triangle. Felixstowe is also not in the present network but is close to the station at Harwich shown by a dot. (b) Map showing the location of Newlyn on the south coast of Cornwall to the south-west of the larger town of Penzance. (c) Sketch of Newlyn harbour showing the North and South Piers, Tidal Observatory, and the locations of benchmarks.
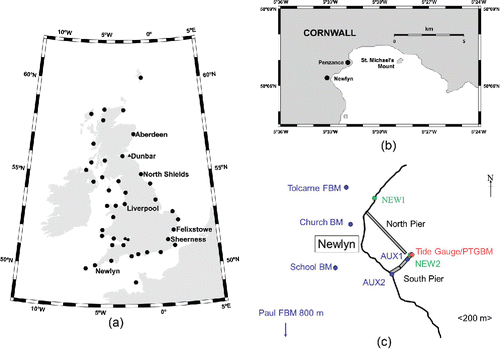
Figure 2. A view to the north-east, from Newlyn Harbour to St. Michael's Mount, showing the lighthouse and Tidal Observatory to its right, both painted red and white. The white GPS antenna on top of the lighthouse platform can be clearly seen. Photograph taken in 2010. A Wikimedia Commons image.
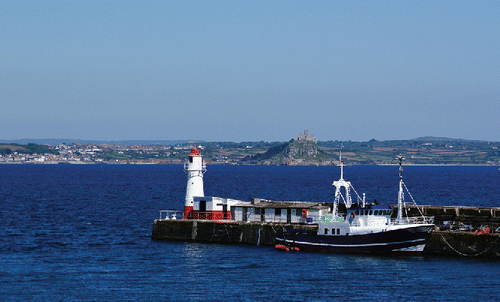
Although MSL from the tide gauges at Newlyn and Felixstowe agreed to 0.04 feet (0.012m), well within the probable error of the conventional precise levelling between them, when the levelling was completed to Dunbar the apparent difference of MSL was 0.81 feet (0.247m), far higher than the estimated probable error of ±0.16 feet (±0.048m). It was concluded that there must be a real difference in MSL between Newlyn and Dunbar, even allowing for different meteorological effects at both stations. Because of this variation, rather than taking the mean of the different levels, it was decided to fix the levelling datum to MSL as observed at Newlyn alone, for the period 1 May 1915 to 30 April 1921 (Jolly and Wolff Citation1922).
The MSL at Newlyn, computed as the average of the hourly values of sea level over this six-year period, had a value of 9.412 feet above the Observatory Zero Datum. That datum was defined as 25 feet below the Observatory Benchmark, which is a brass mark adjacent to the tide gauge (; similar benchmarks and definitions of observatory zero were used at Dunbar and Felixstowe). This level of 15.588 feet (4.751 m) below the mark was denoted as Ordnance Datum Newlyn (ODN). ODN was adopted subsequently as the national datum for the United Kingdom, eventually replacing the earlier Ordnance Datum Liverpool (ODL). Differences between ODN and ODL were computed for marks common to both networks (e.g., ODN was determined to be 0.13 feet below ODL at Liverpool, see the table on page 10 of Jolly and Wolff Citation1922) and were provided in the form of maps showing the variation of the difference around the country (e.g., plate III of Jolly and Wolff Citation1922).
3. The Newlyn tide gauges
The Newlyn Tidal Observatory is located in a small building next to the lighthouse at the end of the South Pier in Newlyn Harbour ( and ). Sea level measurements were originally made by means of an inlet (diameter 9 inches) on the harbour side of the pier that allows water into and out of a rectangular stilling well (measuring 63 by 49 inches, equivalent to a circular well with diameter 1.6 m), equipped with a float and chart-recording gauge (). On the frame of the tide gauge was a contact point from which soundings could be taken to the water surface in the well using an accurate steel tape, the end of which had a silver needle that completed an electrical circuit when it touched the surface, causing a bell to ring or light to flash; these are now called dipping measurements. Such soundings throughout a tidal cycle provided a comparison of the real water level in the well to that recorded on the gauge, as a check on offset, scale error and hysteresis in the measurements (this is called a Van de Casteele test; see Míguez Martín et al. Citation2008). The height of the contact point with respect to the Observatory Benchmark, and in turn to the nearby FBMs, was checked periodically by conventional precise levelling.
Figure 4. An original diagram of the Tidal Observatory indicating the inlet to the stilling well on the harbour side of the South Pier, the Cary Porter tide gauge and its float, and dipping measurements using a steel tape from the Contact Point to the water surface. ‘MSL 1915–1921’ represents Ordnance Datum Newlyn.
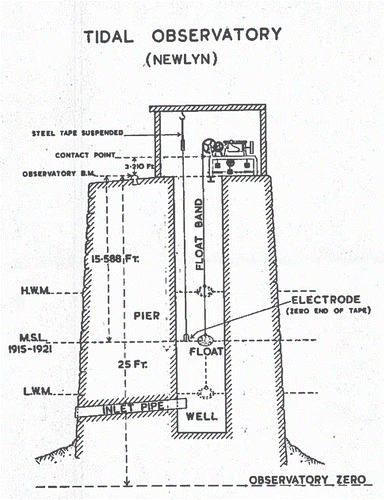
The original tide gauge was provided by the Cary and Porter company () using weekly charts, with the first measurements on Wednesday, 21 April 1915 (). This equipment was operated as the primary sea level sensor for most of the 20th century, with charts processed by the OS. Meteorological measurements were made alongside the sea level measurements. Unfortunately, while the hourly digitizations of the Cary Porter tide gauge charts by the OS exist from the beginning of the station, the charts themselves from before the 1940s no longer survive; it is believed that they were lost in the bombing of Southampton during the Second World War. (Most of the remaining OS archives were transferred to The National Archives some years ago and clearly further research is needed to establish if they survive there. However, the loss of the charts would be consistent with the destruction of most of the UK levelling records prior to 1939; Kelsey Citation1972.) This means that many interesting higher-frequency signals in the tide gauge record, such as that due to the Grand Banks tsunami of 1929, which crossed the Atlantic and was observed by Portuguese tide gauges (Fine et al. Citation2005), are no longer available for study.
Figure 6. The first measurements of sea level, which would have been obtained from inspection of the chart, entered into the station ledger on 21 April 1915.
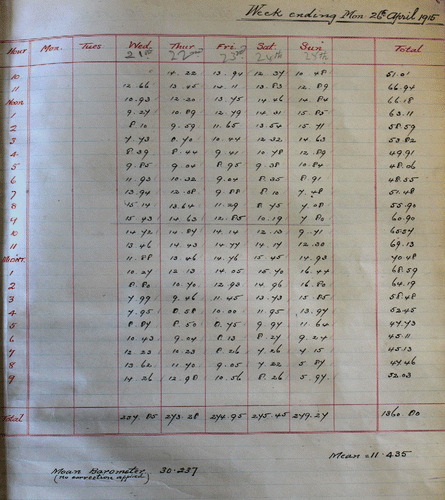
In the late 1970s, the National Tide Gauge Committee (formed from the OS, the Natural Environment Research Council (NERC), and other interested bodies) noted that new technologies were becoming available and encouraged the Institute of Oceanographic Sciences (IOS, a component of NERC) to compare measurements from the float gauge inside the well to those from an external pneumatic gauge. Consequently, IOS installed an Aanderaa bubbler gauge from June 1978 to May 1979. Subsequent data comparisons (Pugh Citation1981) showed that, while tidal constituent amplitudes were the same, there was a lag of 2.7 minutes in the well phase for M2. This lag was determined to be due to the small area of an orifice, connecting the inlet pipe to the well, compared with the large cross-sectional area of the well itself. shows that water enters from the ocean through a 9-inch diameter, 12-foot long pipe, but in fact the orifice from the pipe to the well has only a small diameter (thought to be 1.5 inches on drawings but reported by divers to be more likely 1.25 inches). Theoretical considerations based on Noye (Citation1974) for the dimensions of the Newlyn stilling well and its orifice were consistent with the observed lag (Pugh Citation1981). In 1987, divers widened this orifice to 3 inches.
It was also observed that the non-tidal residuals in the semidiurnal frequency band were more than double for the well (4.44 cm2) than for the external pneumatic gauge (2.18 cm2). The most likely reasons were irregular partial blocking of the connecting pipes, given that divers had reported biological activity, including eels and crabs, in and around the pipe entrance. A separate reason for data differences concerned the weekly fitting of the paper charts for the well tide gauge. Of some 25 of the most significant discontinuities in the non-tidal residual time series, 19 occurred on a Monday, the day for regular chart exchange. The operators, among the most skilled and dedicated associated with any UK tide gauge, could not get the fittings to be at exactly the correct level and time. Another problem may have been small time drifts, as the gauge clockwork drive was also regularly wound on a Monday. Partly as a result of these comparisons, an Aanderaa pressure gauge system, with external sensors, was installed from 1981, alongside the traditional well gauge.
The OS maintained the tide gauge at Newlyn, along with some 29 other tide gauges, until 31 December 1983, when the responsibility for all tidal observations was transferred to NERC. In turn, that role was delegated by NERC to one of its laboratories, then called the IOS (Bidston Observatory) and subsequently renamed the Proudman Oceanographic Laboratory (POL). POL is now part of the National Oceanography Centre (NOC), which also hosts the British Oceanographic Data Centre (BODC) that has the responsibility for quality control and archiving of the data.
A number of developments took place in 1983. An “A Class Network” bubbler pressure gauge was installed in August following experimentation with pressure gauge technology at IOS over the previous decade (Pugh Citation1972). Since that time, the bubbler has been regarded as the main sensor. In November, the Cary Porter was replaced with a Munro chart recorder which is still operational, with the charts archived by BODC (). (There is no record of why the Cary Porter gauge was replaced. A lack of spare parts is a possible reason.) A data telecommunications and data logging system called DATARING was installed to provide for the real-time transmission of the bubbler tide gauge data to the UK Met Office; that system has since been replaced by more modern, but functionally similar, data transmission equipment. In 1996, a mid-tide pressure point was installed for the precise control of the datum of the bubbler pressure measurements (Woodworth et al. Citation1996). In 2010, the original pressure point of the bubbler gauge, which had been installed on the south (ocean) side of the pier, was damaged in a storm and moved to the harbour side.
Figure 7. The Munro tide gauge installed in 1983 and located above the stilling well. The white L-piece on the front of its frame is the Contact Point from which dipping measurements are made in order to confirm that the gauge is recording to the correct datum. The dipper itself is shown on top of the gauge. (Photograph Les Bradley)
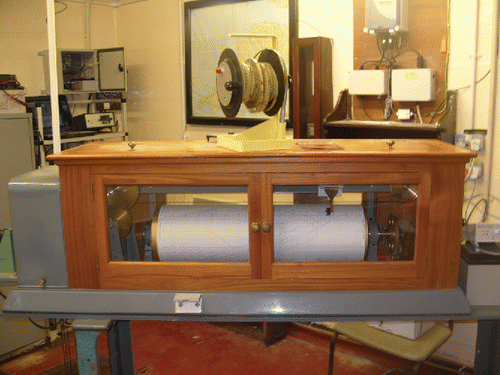
Quality-controlled delayed-mode sea level data from all of these sensors can be obtained from BODC (www.bodc.ac.uk) expressed relative to Admiralty Chart Datum (ACD) which at Newlyn is 3.05 m below ODN. Real-time data may be obtained via the UK National Tidal and Sea Level Facility (www.ntslf.org) or the Intergovernmental Oceanographic Commission Sea Level Monitoring Facility (ioc-sealevelmonitoring.org).
Each record in the BODC quality-controlled data set contains a flag such as a blank (good data), N (missing value), T (interpolated value), and M (improbable value). In the case of Newlyn data, there are many records flagged as M from the 1940s to the 1960s which have been assessed as being due to bad timing of the chart record. In these cases, bad timing presents difficulties in a tidal analysis of separating the tide from the non-tidal signal. However, the heights can safely be used for MSL computation. The same M flag is also used for bad data in the 2000s, which have been assessed as having bad heights as well as times, and these data must not be used if Newlyn data are downloaded for study.
4. The use of ODN as a national datum
ODN, the datum adopted as mean sea level at Newlyn, is still the national height datum for mainland Great Britain today. However, it took some time for this new height datum to populate through to the Ordnance Survey mapping.
After the conversion of the geodetic levelling to the new Newlyn datum was completed, the problem arose as to how best to convert the existing large scale plans (i.e., plans of 1:10000 and larger), which used the old Liverpool datum, to the use of the new Newlyn height datum. Initially, the existing lower order levelling observations (secondary and tertiary level connections) were closed onto the new (Second Geodetic) control. This was used up until about 1929 when it proved in some cases to be impossible to achieve a satisfactory closure. Subsequently new levelling to secondary and tertiary standards was carried out based on the new control.
By 1950, the lower order levellings (secondary and tertiary) were not yet based entirely on the Newlyn datum, with only about 60% having been relevelled and adjusted to that datum. The remaining 40% were still related to the original Liverpool datum and could not be converted directly to Newlyn without relevelling due to anomalies in the lower order levelling between geodetic levelling lines, arising from either errors or subsequent subsidence.
It was estimated that the relevelling to Newlyn would be completed by 1956 and it was this desire to adjust all lower order levelling to a reliable geodetic framework that in part led to the Third Geodetic Levelling of Great Britain (Kelsey Citation1972). Height information displayed on Ordnance Survey maps published since March 1956 is based on Newlyn and the Third Geodetic Levelling.
Although adopted as the national height datum, Newlyn could not be adopted universally due to limitations in accurately transferring height across large stretches of open water. As a result, many island groups remain on their own local datum based on assumed mean sea level, many from the 19th century. Islands which have their altitudes based on a local mean sea level datum include the Isles of Scilly (Hugh Town, St. Marys, 1887), Isle of Man (Douglas Harbour, 1865), Outer Hebrides (Stornoway, 1977), and Shetland Isles (Lerwick, 1900). Orkney mainland was levelled in 1977 to ODN but many of the smaller islands remain on a local datum at Pierowall, Isle of Westray from 1879.
ODN is still referred to as Mean Sea Level on Ordnance Survey mapping today but it should be noted that the datum was only aligned to mean sea level for the period 1915–1921 and sea level has risen since (section 5).
The national height datum ODN is still physically related to the Primary Tide Gauge Bench Mark (PTGBM) at Newlyn, and realized by the network of FBMs. Lower order benchmarks are no longer maintained and have not been checked for more than 30 years. Instead, professional users are recommended to establish their own benchmarks using Global Navigation Satellite System (GNSS) techniques and the quasi-geoid model OSGM02 (Forsberg et al. Citation2002). OSGM02 is a “height-corrector” surface fitted to the FBMs and allows GNSS ellipsoidal heights to be converted to heights relative to ODN. The new version of this corrector surface is due to be released in 2016.
It is possible that Great Britain will follow the example of other countries such as Canada, in that future height determination may use a “purely gravimetric geoid” rather than one fitted to traditional levelling. It has long been suspected that discrepancies between the levelling surface realized by the Second and Third Geodetic Levellings, and the sea surface topography as determined at tide gauges, are due to some systematic error in the levelling (section 5.4). A move away from this approach would result in a new datum being defined (though likely also related to mean sea level). However, this is not a change to the national datum to be taken lightly and would require consultation with numerous stakeholders.
5. The Newlyn sea level record
Newlyn has a predominantly semi-diurnal tide with amplitudes of the main M2 and S2 constituents of 171 and 57 cm, respectively, on average (Amin Citation1985). This local tide has been found to vary from year to year and to have undergone a long-term change that is not fully understood; these changes are referred to in section 5.3.
For study of the long-term changes in sea and land levels discussed below, it is necessary for the semi-diurnal and diurnal astronomical tidal components to be removed from the record, prior to averaging the remaining components to obtain MSL change over different timescales. As explained in section 3, for most of its history Newlyn tide gauge data were obtained from a conventional float and chart recorder gauge, with the charts subsequently digitized to provide hourly heights of sea level. These hourly heights would subsequently be filtered to remove the tide and provide daily, monthly and annual averages of MSL; see Pugh and Woodworth (Citation2014) for a description of such data reduction methods. Since 1993, data have been available in the form of 15-minute average values, which can also be filtered to provide daily, monthly and annual means.
5.1. Long-term changes in Newlyn MSL
The MSL provided by a tide gauge is called a relative sea level measurement, that is, sea level relative to the height of the nearby land, as represented by the heights of benchmarks in either solid rock or buildings (the locations of benchmarks near to the Newlyn tide gauge are shown in ). As a consequence, the MSL record can contain contributions from both the ocean itself, such as a rise in level associated with climate change, and from changes in land level, as in geological processes such as Glacial Isostatic Adjustment (GIA). Recent estimates of the GIA contribution to the MSL record at Newlyn vary from approximately 0.4 mm/year, based on the ICE-6G ice model of Prof. W.R. Peltier (www.atmosp.physics.utoronto.ca/∼peltier/), to about 0.7 mm/year (Shennan et al. Citation2012 and see below) due to land level submergence (or subsidence). The area is relatively little affected by natural tectonic processes (earthquakes, see BGS Citation2015) or anthropogenic ones (e.g., ground water or hydrocarbon extraction).
MSL data for Newlyn and other UK tide gauges are readily available from both BODC and the Permanent Service for Mean Sea Level (PSMSL; Holgate et al. Citation2013). shows that MSL at Newlyn has risen significantly over the past century, at an average rate of 1.8 mm/year (with a standard error of approximately 0.1 mm/year; see discussion of sea level trend in this record in Rossiter (Citation1967), Thompson (Citation1980), Woodworth (Citation1987), Woodworth et al. (Citation1999, Citation2009a), Araújo and Pugh (Citation2008), and Haigh et al. (Citation2009)). This rate is slightly more than in the northern parts of the UK where positive vertical land movement (emergence), primarily due to GIA, is more important (Shennan and Woodworth Citation1992; Shennan and Horton Citation2002; Bradley et al. Citation2009; Teferle et al. Citation2009; Woodworth et al. Citation2009a; Hansen et al. Citation2012). This rate is also similar to the global average over a similar period (Church et al. Citation2014) although, because of the submergence at Newlyn discussed in the next section and based on evidence from other UK tide gauges, it is believed that the real (absolute) rate of sea level change during the 20th to 21st centuries in this region has been closer to 1.4 mm/year (Woodworth et al. Citation2009a).
Figure 8. The record of monthly MSL at Newlyn during the past century. The average rates of change of MSL for the complete record and for the recent period 1993–2014 are 1.8 and 3.8 mm/year respectively and are shown by the black lines. Data from www.psmsl.org
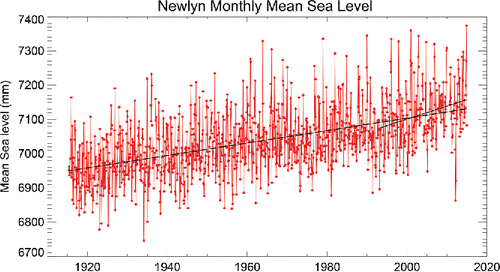
However, the observed rate of sea level change at Newlyn over 1993–2014 has been much larger at 3.8 mm/year (we use 1993 somewhat arbitrarily for the start of the modern era in sea level monitoring as that was when precise altimeter information from space became available). This highest rate in the record may represent the start of a long-term acceleration in sea level due to climate change (Church et al. Citation2014), or simply be a feature of the decadal variability in MSL that has been evident throughout the Newlyn record (and indeed in all tide gauge records). shows that high rates were observed in previous 22-year periods, including those centered on approximately 1926, 1950, and 1980 (with rates of approximately 3 mm/year), with the lowest rates centered on 1934 and 1968 (approximately 0 mm/year), with such accelerations and decelerations in the record similar to those seen in other parts of the world (Woodworth et al. Citation2009b). The variability and long-term trend in the Newlyn MSL record are similar to those at Brest (Wöppelmann et al. 2006), although some differences become apparent in a detailed comparison (Douglas Citation2008), and at other stations in the North Sea area (Wahl et al. Citation2013). Note that anomalously low recent values in February/March 2012 were recorded correctly at Newlyn, being also observed at other nearby stations (St. Mary's, Devonport and Brest).
5.2. Changes in Newlyn land level
Studies of long-term change in MSL depend upon knowing whether the land on which the tide gauge is located is stable or whether there is a significant change in land level, which may occur on short timescales (e.g., a rapid change due to earthquakes) or long ones (e.g., a slow change due to GIA). The stability can be monitored in several ways.
A first method involves repeated conventional precise levelling surveys between the various benchmarks adjacent to the tide gauge, and between those and OS FBMs several km inland. The Observatory Benchmark (), which is denoted the PTGBM, was first connected to the OS primary levelling network in 1915 and was last verified by conventional precise levelling (line G001) in 1990. The local network () is formed from seven benchmarks: the PTGBM, two marks on the South Pier (AUX1 and AUX2), two in Newlyn village and two FBMs. AUX1 is about 50 m from the PTGBM and AUX2 is located where the pier meets the land, about 250 m from the PTGBM. The two marks in the village are about 550 m and 750 m from the PGTBM on a church and an old school, respectively. The two FBMs are at Tolcarne, about 900 m to the North West of the PTGBM, and at Paul, about 1.4 km to the Southwest. The FBMs are founded on stable ground (see plate X of Jolly and Wolff Citation1922), whereas all of the other inland benchmarks are OS flush brackets set into walls. The local network as whole was first connected to the PTGBM in 1952 and last verified by conventional precise levelling (line G001) in 1990. The repeated surveys showed no significant changes in height (i.e., less than 0.1 mm) within the local network from 1952–1990, suggesting that the pier on which the tide gauge is located did not experience any uplift or subsidence relative to any of the benchmarks, including the two FBMs founded on stable ground.
Unfortunately, while checks on changes in the relative heights of the nearby benchmarks (PTGBM, AUX1, and AUX2) are made every few years, they are rarely extended to the FBMs. (Standard practice in the UK network is now to carry out levelling of benchmarks near to a tide gauge each year for three years for new installations, then at two-year intervals, or possibly longer if a site appears to be stable.) Instead, conventional precise levelling of that type has been replaced by the use of GNSS technology, of which the Global Positioning System (GPS) has been the most widely employed form of GNSS to date. During the 1990s, there were approximately a dozen epochal (or episodic) GPS campaigns each lasting typically 5 days at Newlyn, with measurements made at new benchmarks (NEW1 and NEW2) that were connected to the PTGBM and other earlier marks by repeated conventional precise levelling surveys. No evidence for differential movement between the various marks was found.
In the late 1990s, GPS measurements at tide gauges began using permanently installed GPS receivers and antennas, this technique originally being called continuous GPS (CGPS) and now referred to as continuous GNSS (CGNSS) (Neilan et al. Citation1998; Bevis et al. Citation2002). Important considerations were for the GPS antenna to have a clear view of the sky in all directions for elevation angles above 15 degrees and to be located as near as possible to the tide gauge. Two options considered were to locate the antenna at AUX1, which is an OS flush bracket set into the sea wall, or on the roof of the Tidal Observatory (i.e., immediately above the tide gauge). The former afforded a clear view of the sky, whereas the latter had restricted visibility due to the 10 m high lighthouse, a substantial steel structure next to the Tidal Observatory. In the end, it was decided to locate the GPS antenna on the observation platform of the lighthouse, at about 7 m above the pier. This would keep the GPS antenna within a few meters of the tide gauge, whilst raising it above the obstruction caused by the lighthouse and also providing security. The GPS antenna has a reasonably clear view of the sky, although it is not perfect due to the proximity of a weather vane. The GPS receiver was then housed in the Tidal Observatory, where there is mains power and telecommunications.
The original GPS and current GNSS instrumentation follow international guidelines (e.g., Bevis et al. Citation2002) in terms of using a dual-frequency code and phase measuring GPS or GNSS receiver and choke ring antenna. The original GPS receivers were an ASHTECH Z-XII3, installed in September 1998, and an ASHTECH UZ-12, installed in August 2009, which recorded GPS data at 30-second epoch intervals and were downloaded every hour by telephone modem. The original antenna was an ASH700936D_M SNOW, also installed in September 1998, which was located on a monument consisting of a 3 m carbon fiber/stainless steel cylindrical pipe, with a flat, circular mounting surface at its top end that is slightly smaller than the diameter of the base of the GPS antenna. The pipe was mounted on a stainless steel triangular plate that was fixed to the floor of the observation platform on the southwest side of the lighthouse. The present GNSS receiver is a TRIMBLE NETR9, installed in May 2013, which records GNSS (GPS, GLONASS, Galileo, and Beidou) data at 1-second and 30-second epoch intervals and is downloaded every hour by broadband router. The present GNSS antenna is a TRM59900.0 SCIS, also installed in May 2013, which is located on the same monument as the previous GPS antenna.
Vertical connections between the triangular plate, the tide gauge Contact Point, the PTGBM, and other benchmarks can be carried out using conventional precise levelling. By mounting a tripod on the roof of the Observatory and using an inverted staff, a conventional precise levelling connection can be made between the triangular plate and a staff located on NEW2 on the pier. Conventional precise levelling can then be used to connect NEW2 to other marks.
The data from the Newlyn CGPS/CGNSS station are archived by the NERC British Isles continuous GNSS Facility (BIGF) at the University of Nottingham and made available to international data banks (e.g., Système d'Observation du Niveau des Eaux Littorales, SONEL, www.sonel.org). From these data, time series of the north, east, and height coordinate components for Newlyn relative to the center of the Earth can be constructed as described by Teferle et al. (Citation2009) and Santamaría-Gómez et al. (Citation2012). The latest coordinate time series created by the NERC BIGF for data from 1998–2014 are shown in . These were obtained using Bernese GNSS software version 5.2 (BSW5.2) (Dach et al. Citation2007; Dach Citation2013) and Coordinate Analysis of Time Series (CATS) software (Williams Citation2003), through a reprocessing and reanalysis of the daily GPS data from Newlyn and a global network of about 200 CGPS/CGNSS stations that form part of the International GNSS Service (IGS), using C13 (CODE repro2/repro_2013) reanalyzed satellite orbit and earth orientation parameter products; the IGb08 reference frame; mitigation of first and higher order (second and third order and ray bending) ionospheric effects; a-priori modelling of troposphere effects using VMF1G and mitigation using zenith path delay and gradient parameters; I08.ATX models for antenna phase center variations; and models for solid Earth tides, ocean tidal loading and atmospheric tidal loading that are consistent with IERS (Citation2010) conventions.
Figure 9. Coordinate time series for the Newlyn CGPS/CGNSS station for the period from 1998 to 2014, created by the NERC British Isles continuous GNSS Facility using the BSW5.2 and CATS software. WRMS indicates the weighted root-mean-square of daily coordinate values about smoothed fits to the data that include a linear trend, and annual and semi-annual signals in the time series due to periodic or seasonal effects, which are most apparent in the Height values (blue lines), and offsets in the time series at the three vertical lines. From the Height time series, the linear rate of vertical land movement is estimated as −0.7 mm/year over this period.
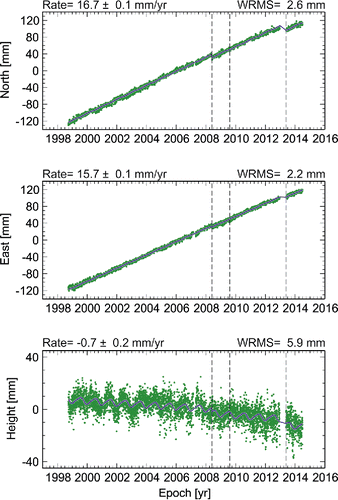
The coordinate time series include linear velocity estimates that for north and east components represent the horizontal motion of the Newlyn CGPS/GNSS station on the Eurasian plate as 16.7 +/− 0.1 and 15.7 +/− 0.1 mm/year, and for the height component represent the vertical land movement as −0.7 +/− 0.2 mm/year (i.e., subsidence), accounting for annual and semi-annual signals in the time series due to periodic or seasonal effects and accounting for offsets in the time series at the three vertical lines, which are artefacts resulting from minor disturbances and changes to the equipment: in May 2008 when the antenna monument was disturbed during repair work to the lighthouse; in August 2009 when the receiver changed from an ASHTECH Z-XII3 to an ASHTECH UZ-12; and in May 2013 when the receiver changed from an ASHTECH UZ-12 to a TRIMBLE NETR9 and the antenna changed from an ASH700936D_M SNOW to a TRM59900.00 SCIS.
Rates of relative land level change and vertical land movements in the south-west have also been estimated by geological methods (e.g., Gehrels Citation2006), from geology in combination with geodynamic modelling (Bradley et al. Citation2009; Shennan et al. Citation2012), and, in earlier work, by using CGPS constrained by Absolute Gravity (Teferle et al. Citation2006, Citation2009) (noting that such constraints are not applied in this paper as with the latest results there is now much closer agreement between the vertical land movements estimated by CGPS (−0.7 +/− 0.2 mm/year) and Absolute Gravity (−1.1 +/− 0.8 mm/year)). In general, while one can say that the Newlyn tide gauge appears to be located in a geologically stable area with regard to any localized variations, the rates of relative land level change and vertical land movements appear to be negative (i.e., subsidence) throughout the south of England (e.g., see of Teferle et al. Citation2009; of Shennan et al. Citation2012).
Recently, scientists have applied other new geodetic techniques to the measurement of sea and land levels at Newlyn. These methods include GPS reflectometry, in which sea level information can be obtained with the use of data from the same GPS receiver used for measuring land movements, using a technique similar to that of Larson et al. (Citation2013).
In addition, Persistent Scatterer Interferometry (PSI), that has provided a detailed synoptic overview of emergence (uplift) and submergence (subsidence) in the London area (Aldiss et al. Citation2014), has been applied to the areas around four UK tide gauges (Newlyn, Liverpool, North Shields, and Sheerness). Adamska (Citation2012) found that the technique worked best in the more dense urban areas of Liverpool and North Shields; however, the results for Newlyn are also of interest. was made using the GAMMA software (Werner et al. Citation2003) using the Interferometric Point Target Analysis (IPTA) algorithm and 34 ERS satellite images over 1995–1999. In order to investigate changes in land level around the Newlyn tide gauge, simple statistics were applied to the PS points whereby a 1×1 km box was drawn and then divided into an inland area and a coastal area that included the vicinity of the gauge. An additional inland PS point was also identified to act as an assumed stable reference in a similar manner to conventional precise levelling. The coastal area followed the shore of the town and included 16 PS points, the inland area comprised almost the whole of the town and included 134 PS points, and the inland PS point was about 450 m to the southwest of the tide gauge. With reference to the inland PS point, the inland area had an average velocity of −0.1 ± 0.4 mm/year, and the coastal area had an average velocity of 0.0 ± 0.6 mm/year, consistent with the repeated conventional precise levelling surveys mentioned above, that showed no significant changes within the local network from 1952–1990. The PSI technique will be applied again to Newlyn and other tide gauge locations as new data sets become available (e.g., from the Sentinel-1 satellites).
Figure 10. PSI relative vertical land movements in the area around the Newlyn tide gauge for the period from 1995 to 1999 from Adamska (Citation2012). In general, the bluish color indicates uplift, reddish indicates subsidence and shades of green suggest areas that are stable. Units are mm/year.
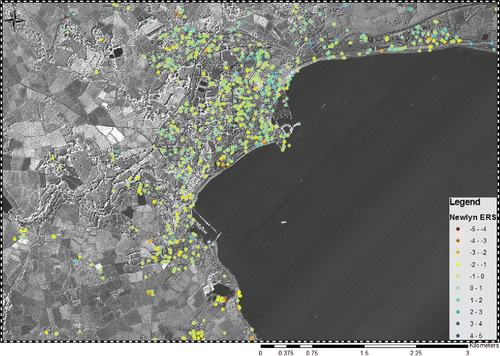
5.3. Other variability in the Newlyn sea level record
One can look at other components of the sea level record, in addition to changes in MSL. For example, the smaller red and blue dots in show time series of annual 99 and 1 percentile levels respectively. These are the levels each year for which sea level is exceeded 1% and 99% of the time, respectively. In other words, sea level is higher than the 99 percentile level for about 88 hours in any given year and lower than the 1 percentile for about 88 hours. Any periods of high tides and storm surges in the year are likely to be in the 88 hours above the 99 percentile. The average for each series is set to zero.
Figure 11. Time series of the annual 99 and 1 percentile sea levels (smaller red and blue dots respectively). The average for each series is set to zero. The larger red and blue dots show the time series of annual maximum and minimum water levels relative to the long term means for the 99 and 1 percentiles.
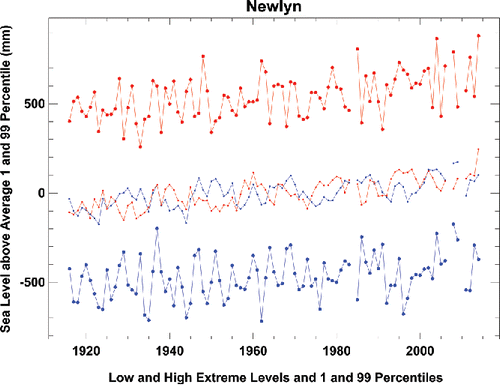
The use of 99 and 1 percentiles in this way is sometimes preferred over the choice of the actual annual maximum and minimum water levels (i.e., 100 and 0 percentiles, respectively) since they provide a description of change in high and low water characteristics without the greater year-to-year variability inherent in the true extremes. The larger red and blue dots show those annual maximum and minimum water levels relative to the long-term means for the 99 and 1 percentiles. These time series can indeed be seen to be considerably noisier than the 99 and 1 percentiles.
The long-term trend in 99 and 1 percentile level is 2.0 and 1.6 mm/year for 1916–2014 compared to the trend of 1.8 mm/year in median sea level. Trends for the extremes are more difficult to calculate because of the greater variability in their records. Those for high and low extreme levels are 2.1 and 1.3 mm/year. The larger rates of increase in high waters over low waters have been noted by several authors, with a possible contributor being increasing local tidal amplitudes. For example, Woodworth et al. (Citation1991) looked at changes in Mean Tidal Range (the difference between Mean High and Mean Low Waters, which is approximately twice the amplitude of M2) and found a trend of 0.39 ± 0.09 mm/year during 1916–1984. Araújo and Pugh (Citation2008) investigated the amplitude of M2 itself, finding a trend of 0.19 ± 0.03 mm/year during 1915–2005, or roughly half the MTR trend, a value confirmed by Haigh et al. (Citation2010). This secular change in the Newlyn tide is not fully understood, nor are recent tidal changes observed in some other parts of the world (Woodworth Citation2010).
Araújo and Pugh (Citation2008) additionally pointed to a 2.3° decrease in M2 phase lag after 1984 when the bubbler gauge replaced the float tide gauge as the main sensor. The S2 phase lag decreased by 2.2° and that of M4 decreased by 4.9°. These decreases are consistent with the previous discussion if the stilling well introduced a small lag in the float measurements, the bubbler recording sea level outside the well. They also noted a reduction in the variance of tidal residuals after 1984, consistent with previous experience.
Information on the highest sea levels at Newlyn and other UK tide gauges, their individual tidal and nontidal (i.e., storm surge) contributions, and the coastal flooding that followed can be found at www.surgewatch.org (Haigh et al. Citation2015). Note that the web page lists the skew surge, which is defined as the maximum observed sea level during the surge event minus the maximum predicted astronomical tide, where the two maxima would be during the same high tide but could be at slightly different times. This contrasts with the definition of the surge itself, which is the difference between the observed level and astronomical tide prediction; see Pugh and Woodworth (Citation2014).
The highest sea level observed at Newlyn of 6.44 m above Chart Datum occurred on 3 February 2014 during a winter of several major storms around the UK coast (Kendon and McCarthy Citation2015; Wadey et al. Citation2015). This high water level can be explained by a contribution from the astronomical tide (estimated at 5.97 m) together with a skew-surge of 0.47 m. Sea levels of that magnitude have return periods of approximately 40 years (Wadey et al. Citation2014), a return period being the average length of time between events of a similar magnitude (see chapter 12 of Pugh and Woodworth Citation2014).
Most of the large storm surges at Newlyn have magnitudes of around 0.5 m (as can be inferred from the amount by which the annual maximum water levels exceed the 99 percentile values shown in ). These surges are much smaller than those that occur in the North Sea or elsewhere around the UK coast (e.g.. see McRobie et al. Citation2005 for a discussion of the 1953 storm surge flooding in the southern North Sea). An exception for Newlyn listed in www.surgewatch.org is the unusual skew-surge of 0.78 m on 27 October 2004 that was 0.2 m larger than any other in the record. This large skew-surge combined with what was only a modest high astronomical tide to give a high overall level; an even higher astronomical tide (potentially 0.5 m higher) would most probably have resulted in significant damage. (Note that in this event, the surge and skew surge were in fact similar in magnitude or, in other words, the surge occurred near to tidal high water.) Changes in extremes of tidal and nontidal origin in the English Channel as a whole have been studied by Haigh et al. (Citation2010), and as part of pan-European sea level variability by Vilibić and Šepić (Citation2010).
One may look back through the past century to the contributions that the Newlyn tide gauge has made to sea level science. Many of these contributions may be found in oceanographic text books (e.g., Pugh and Woodworth Citation2014), and so we list only a few of them here to indicate the different topics studied.
Doodson (Citation1924) may be mentioned as one of the first investigations of meteorological effects on sea level at Newlyn. As Newlyn is located nearer to deep water than most UK tide gauges, its sea level response to air pressure changes is dynamically simpler than those elsewhere, and an approximately inverse barometer relationship between sea level and air pressure is found. (This also results in the storm surges mentioned above being smaller than in the North Sea.) This means that other oceanographic signals in the data are more accessible for study, including how UK sea levels are affected by large-scale atmospheric and oceanic processes such as the North Atlantic Oscillation (NAO) (Thompson Citation1986; Tsimplis et al. Citation2005) and the circulation of the Celtic Sea (e.g., Pugh and Thompson Citation1986). The variability in sea level on monthly timescales and longer measured by the Newlyn tide gauge is not dominated by local processes but corresponds closely to that of the neighboring open sea as measured for the past two decades by satellite altimetry (Woodworth and Hibbert Citation2015).
There are more recent examples of studies of sea level variability at Newlyn on shorter timescales. The south coast of England experiences occasional meteotsunamis, generated by rapid atmospheric changes, and tsunamis, generated by distant submarine earthquakes. For example, Tappin et al. (Citation2013) discuss the 27 June 2011 meteotsunami, driven by convective cells extending from the Bay of Biscay to the English Channel, that had a roughly 20 cm signal at Newlyn and roughly 40 cm at Portsmouth.
In addition, there are several examples of tsunamis in the Newlyn tide gauge record resulting from distant undersea earthquakes; these signals are small due to the distance from the event and attenuation on the continental shelf, but they provide useful information to tsunami modelers on tsunami propagation times. For example, the Newlyn records of 28 February 1969 show oscillations of 0.1 m amplitude, which start suddenly and persist for more than 24 hours following a magnitude 7.9 earthquake 130 miles from the coast of Portugal (Pugh and Vassie Citation1980). Another example is given in which shows a small signal of about 6 inches peak-to-peak in the Newlyn tidal chart for 25 November 1941 from the tsunami (and possibly associated seiching) following a magnitude 8.2 earthquake centered 500 miles off the Portuguese coast. Both tsunamis are described by Baptista and Miranda (Citation2009). The latter is instructive in showing oscillations at Newlyn with a period of approximately 15–20 minutes, which would not be sampled properly by the present bubbler gauge integration period of 15 minutes, which emphasizes the importance of having higher-frequency sampling in future. Newlyn data from the bubbler and float gauges, both of which demonstrated an increase of sea level variability at approximately 1400 UTC on 27 December 2004, were useful in understanding the propagation of the Sumatra tsunami (Woodworth et al. Citation2005).
Figure 12. Part of the Newlyn chart for 25 November 1941 showing a small tsunami signal following an undersea earthquake off the coast of Portugal (Baptista and Miranda Citation2009). The earthquake itself was just after 6 pm and the oscillations at Newlyn began around 10:30 pm. Each vertical line on the chart corresponds to an hour and the thick line marks midnight. Each thick horizontal line corresponds to one foot sub-divided into tenths of a foot. The dots indicate the hourly digitizations of sea level.
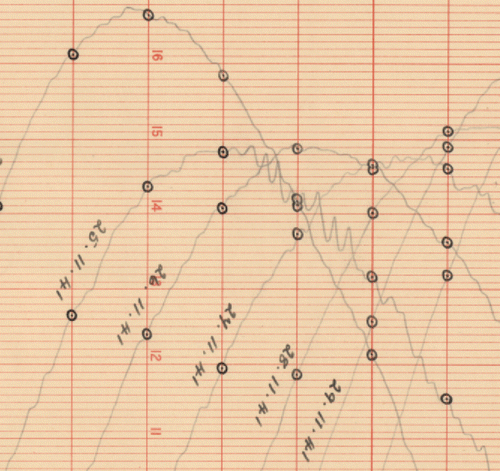
Over longer timescales, Newlyn data have been used extensively in studies of changes in MSL and extreme sea levels, as we have reviewed above. The data have been used to develop new methods of tidal analysis (Munk and Cartwright Citation1966). In addition, they have been employed in the development of extreme sea level computation methods for coastal engineering (e.g., Blackman and Graff Citation1978; Pugh and Vassie Citation1980; Dixon and Tawn Citation1992), these methods becoming of greater importance as sea level rises.
5.4. Spatial variation of UK MSL
We may return to the topic of the spatial, rather than temporal, variation of UK MSL. The difference of several decimeters between MSL at Newlyn and Dunbar (both measured to the ODN datum) obtained during the early studies of the Second Geodetic Levelling was the first observation of what appeared to be an anomalous north-south slope of UK sea level. This problem has been studied over many decades with different versions of the levelling and tide gauge data sets and had not been fully resolved (e.g., see Edge Citation1959; Thompson Citation1980; Ziebart et al. Citation2008). The problem is that if the MSL difference is real, then it implies an ocean circulation between north and south, which has to be explained dynamically. However, oceanographers knew that there was no such current (or at least not large enough). An alternative explanation for the anomalous slope instead suggested systematic errors in the levelling (Hipkin et al. Citation2004; Penna et al. Citation2013).
This topic has to a great extent now been consigned to history. There will never be a Fourth Geodetic Levelling in a similar way to the first three (in spite of the Introduction by Sir Charles Close in Jolly and Wolff (Citation1922), looking forward to another levelling campaign around the year 2000). Instead, it is now possible to determine ellipsoidal heights of MSL (i.e., heights above a reference ellipsoid) at tide gauges using GPS/GNSS measurements of their benchmarks. Values of the geoid, obtained from advanced space techniques, can be subtracted from the MSL values to give the Mean Dynamic Topography, which can in turn be compared to ocean estimates of spatial changes in sea level (e.g., Woodworth et al. Citation2012). Such studies for the UK have confirmed that there is very little real MSL difference from north to south (e.g., see early studies of this topic by Ashkenazi et al. Citation1994), and that the apparent latitudinal variation in MSL, relative to ODN, had been due to the ODN levelling errors (Penna et al. Citation2013). Similar studies in Australia and North America have also resolved their anomalous historical sea level slopes (Featherstone and Filmer Citation2012; Higginson et al. Citation2015).
6. The Newlyn tidal observatory present and future
The Newlyn Tidal Observatory was installed at a time when most of Europe was at war and resources at the OS were limited (the first Newlyn tide gauge measurements were made at the end of April 1915 in the same week as the start of the Gallipoli Campaign). Nevertheless, it has always been maintained to a good standard (and certainly better than most other UK tide gauges), its data have played a fundamental role in UK geodesy and oceanography, and Newlyn data have been included in almost all international studies of sea level change during the 20th to 21st centuries (e.g., Church et al. Citation2014). The Newlyn installation outlived the similar tidal observatory at Dunbar, where recording was stopped in 1950 because of increasing siltation, and what was intended by the OS to be only a temporary installation at Felixstowe. The funding for the operation of Newlyn tide gauge is now provided by the UK Environment Agency, while the maintenance itself continues to be undertaken by NOC. The tide gauge remains an important component of the UK National Tide Gauge Network (; www.ntslf.org) and the Global Sea Level Observing System (GLOSS) (IOC Citation2012), and as such is an essential contributor to UK sustained observations of the marine environment (Tamisiea et al. Citation2014).
It is essential that the Newlyn Tidal Observatory continues to be maintained to the highest possible standard. At the time of writing, there are no plans to augment the present bubbler and float tide gauges with other technologies, such as a radar gauge which is becoming a standard in international sea level recording; the South Pier and the buildings do not readily lend themselves to using an open-air radar, although a guided-wave radar in the stilling well may be a possibility (IOC Citation2015). However, more modest upgrades might be considered, for example by providing 6- or even 1-minute values of sea level, instead of 15 minutes, which would be consistent with recording in other countries, as has been recommended for the UK National Tide Gauge Network for many years (Woodworth et al. Citation2004), and would provide a better description of storm surges, tsunamis and other higher frequency signals in the tidal record. The venerable Munro float gauge, which can record such rapid sea level changes on its chart, should not be retired until there are suitable high-frequency measurements provided by the bubbler or other gauge.
In addition, given the many developments in GNSS processing that are taking place (e.g. use of data from the GLONASS, Galileo and BeiDou satellites as well as from the US GPS constellation; high data rate applications such as GPS/GNSS reflectometry; and coastal GPS/GNSS-meteorology), the continued installation of state-of-the-art GNSS equipment at such an important geodetic location as Newlyn will be essential.
Through such foresight and investment, the geodesists and oceanographers of a century from now will benefit considerably from 200 years of the highest quality sea and land level data at Newlyn for application to the scientific issues of that time.
Acknowledgements
We are grateful to the tide gauge specialists at the Ordnance Survey and National Oceanography Centre who have maintained the Newlyn Tidal Observatory so well for a century. In particular, we thank the local contacts at Newlyn including Joe Hutchings, Roy Mitchelmore, his daughter Dawn Turner and her family, who have been involved in maintaining the gauge since the 1950s. We are grateful to Isabel Araújo and Ivan Haigh for providing information, and especially to Guy Wöppelmann who stimulated us to write this article following his own description of the importance of the Marseille tide gauge in French geodesy. Part of this work was funded by UK Natural Environment Research Council National Capability funding. Some of the figures in this paper were generated using the Generic Mapping Tools (Wessel and Smith Citation1998).
References
- Adamska, L. M. 2012. Use of persistent scatterer interferometry for the enhancement of vertical land movement measurement at tide gauges around the British Coast. PhD Thesis. University of Nottingham, UK.
- Airy, G. B. 1845. On the laws of the tides on the coasts of Ireland, as inferred from an extensive series of observations made in connection with the Ordnance Survey of Ireland. Philosophical Transactions of the Royal Society of London 135:1–124.
- Aldiss, D., H. Burke, B. Chacksfield, R. Bingley, N. Teferle, S. Williams, D. Blackman, R. Burren, and N. Press. 2014. Geological interpretation of current subsidence and uplift in the London area, UK, as shown by high precision satellite-based surveying. Proceedings of the Geologists' Association 125:1–13.
- Amin, M. 1985. Temporal variations of tides on the west coast of Great Britain. Geophysical Journal of the Royal Astronomical Society 82:279–299.
- Araújo, I. B. and D. T. Pugh. 2008. Sea levels at Newlyn 1915–2005: Analysis of trends for future flooding risks. Journal of Coastal Research 24:203–212.
- Ashkenazi, V., R. M. Bingley, A. H. Dodson, and T. F. Baker. 1994. The determination of tide gauge heights in the United Kingdom by GPS. In Report of the Surrey Workshop of the IAPSO Tide Gauge Bench Mark Fixing Committee. Carter, W. E. (ed.). Report of a meeting held 13–15 December 1993 at the Institute of Oceanographic Sciences Deacon Laboratory, NOAA Technical Report NOSOES0006, 71–75. Available at http://www.psmsl.org/train_and_info/training/reading/geodesy.php. Last accessed 1 January 2016.
- Baptista, M. A. and J. M. Miranda. 2009. Revision of the Portuguese catalog of tsunamis. Natural Hazards and Earth System Sciences 9:25–42. Available at www.nat-hazards-earth-syst-sci.net/9/25/2009/. Last accessed 1 January 2016.
- Beckley, B. D. Ray, R., Holmes, S., Zelensky, N., Lemoine, F., Yang, X., Brown, S., Desai, S., Mitchum, G. and Hausman, J. 2013. Integrated multi-mission ocean altimeter data for climate research: TOPEX/Poseidon. In Jason-1 and OSTM/Jason-2 User's Handbook, version 2. Pasadena: California Institute of Technology. Available at podaac.jpl.nasa.gov. Last accessed 1 January 2016.
- Bevis, M., W. Scherer, and M. Merrifield. 2002. Technical issues and recommendations related to the installation of continuous GPS stations at tide gauges. Marine Geodesy 25:87–99.
- Blackman, D. L. and J. Graff. 1978. The analysis of annual extreme sea levels at ports in southern England. Proceedings of the Institution of Civil Engineers 65:339–357.
- Bradley, S. L., G. A. Milne, F. N. Teferle, R. M. Bingley, and E. J. Orliac. 2009. Glacial isostatic adjustment of the British Isles: New constraints from GPS measurements of crustal motion. Geophysical Journal International 178:14–22.
- British Geological Survey (BGS). Earthquakes in the UK. 2015. Available at http://www.bgs.ac.uk/discoveringGeology/hazards/earthquakes/UK.html. Last accessed 1 January 2016.
- Church, J. A., Clark, P. U., Cazenave, A., Gregory, J. M., Jevrejeva, S., Levermann, A., Merrifield, M. A., Milne, G. A., Nerem, R. S., Nunn, P. D., Payne, A. J., Pfeffer, W. T., Stammer, D. and Unnikrishnan, A. S. 2014. Sea level change. In Climate Change 2013: The Physical Science Basis. Working Group I Report of the Fifth Scientific Assessment of the Intergovernmental Panel on Climate Change. Cambridge, UK: Cambridge University Press.
- Coulomb, A. 2014. Le marégraphe de Marseille - 130 ans d'observation du niveau de la mer. Paris: Presses des Ponts.
- Dach, R. 2013. Bernese GNSS Software: New features in version 5.2, Astronomical Institute, University of Bern, Bern, Switzerland.
- Dach, R., U. Hugentobler, P. Fridez, and M. Meindl. 2007. Bernese GPS Software Version 5.0. Astronomical Institute, University of Bern, Bern, Switzerland.
- Dixon, M. J. and J. A. Tawn. 1992. Trends in U.K. extreme sea levels: A spatial approach. Geophysical Journal International 111:607–616.
- Doodson, A. T. 1924. Meteorological perturbations of sea-level and tides. Geophysical Journal of the Royal Astronomical Society 1 (Supplement S4): 124–147.
- Douglas, B. C. 2008. Concerning evidence for fingerprints of glacial melting. Journal of Coastal Research 24:218–227.
- Edge, R. C. A 1959. Some considerations arising from the results of the second and third geodetic levellings of England and Wales. Bulletin Géodésique 52:28–36.
- Featherstone, W. E. and M. S. Filmer. 2012. The north-south tilt in the Australian Height Datum is explained by the ocean's mean dynamic topography. Journal of Geophysical Research 117:C08035.
- Fine, I. V., A. B. Rabinovich, B. D. Bornhold, R. E. Thomson, and E. A. Kulikov. 2005. The Grand Banks landslide-generated tsunami of November 18, 1929: Preliminary analysis and numerical modeling. Marine Geology 215:45–57.
- Forsberg, R., G. Strykowski, J. Illiffe, M. Ziebart, P. Cross, and C. Tscherning. 2002. OSGM02: A geoid model of the British Isles. Stage Two (Final) Report. Kort- og Matrikelstyrelsen, Copenhagen.
- Gehrels, R. 2006. Sea-level rise and coastal subsidence in southwest England. Transactions of the Devonshire Association for the Advancement of Science 138:25–42.
- Haigh, I., R. Nicholls, and N. Wells. 2009. Mean sea level trends around the English Channel over the 20th century and their wider context. Continental Shelf Research 29:2083–2098.
- Haigh, I., R. Nicholls, and N. Wells. 2010. Assessing changes in extreme sea levels: Application to the English Channel, 1900–2006. Continental Shelf Research 30:1042–1055.
- Haigh, I. D., M. P. Wadey, S. L. Gallop, H. Loehr, R. J. Nicholls, K. Horsburgh, J. M. Brown, and E. Bradshaw. 2015. A user-friendly database of coastal flooding in the United Kingdom from 1915–2014. Scientific Data 2:150021.
- Hansen, D. N., F. N. Teferle, R. M. Bingley, and S. D. P Williams. 2012. New estimates of present-day crustal/land motions in the British Isles based on the BIGF network. In Geodesy for Planet Earth. Kenyon, S., M. C. Pacino, and U. Marti (Eds.), International Association of Geodesy Symposia 136. Berlin: Springer-Verlag, 666–671.
- Higginson, S., K. R. Thompson, P. L. Woodworth, and C. W. Hughes. 2015. The tilt of mean sea level along the east coast of North America. Geophysical Research Letters 42:1471–1479.
- Hipkin, R., K. Haines, C. Beggan, R. Bingley, F. Hernandez, J. Holt, and T. Baker. 2004. The geoid EDIN200 and mean sea surface topography around the British Isles. Geophysical Journal International 157:565–577.
- Holgate, S. J., A. Matthews, P. L. Woodworth, L. J. Rickards, M. E. Tamisiea, E. Bradshaw, P. R. Foden, K. M. Gordon, S. Jevrejeva, and J. Pugh. 2013. New data systems and products at the Permanent Service for Mean Sea Level. Journal of Coastal Research 29:493–504.
- IERS. 2010. IERS Conventions. Petit, G. and B. Luzum (eds.). International Earth Rotation Service Technical Note 36. Frankfurt am Main: Verlag des Bundesamts für Kartographie und Geodäsie.
- IOC. 2012. The Global Sea Level Observing System (GLOSS) Implementation Plan–2012. UNESCO/Intergovernmental Oceanographic Commission, IOC Technical Series No. 100. Available at ioc.unesco.org. Last accessed 1 January 2016.
- IOC. 2015. Manual on Sea Level Measurement and Interpretation. Volume V: Radar Gauges. Manuals and Guides 14. Intergovernmental Oceanographic Commission (in preparation).
- Jolly, H.P.L.G. and A. J. Wolff. 1922. The Second Geodetic Levelling of England and Wales 1912–1921. London: Ordnance Survey by HMSO.
- Kelsey, J. 1972. Geodetic aspects concerning possible subsidence in southeastern England. Philosophical Transactions of the Royal Society of London A, 272:141–149.
- Kendon, M. and M. McCarthy. 2015. The UK's wet and stormy winter of 2013/2014. Weather 70:40–47.
- Larson, K. M., J. Löfgren, and R. Haas. 2013. Coastal sea level measurements using a single geodetic GPS receiver. Advances in Space Research 51:1301–1310.
- Míguez Martín, B. L. Testut, and G. Wöppelmann. 2008. The Van de Casteele test revisited: An efficient approach to tide gauge error characterization. Journal of Atmospheric and Oceanic Technology 25:1238–1244.
- Matthäus, W. 1972. On the history of recording tide gauges. Proceedings of the Royal Society of Edinburgh B 73:26–34.
- McRobie, A., T. Spencer, and H. Gerritsen. 2005. The Big Flood: North Sea storm surge. Philosophical Transactions of the Royal Society of London A, 363:1263–1270.
- Munk, W. H. and D. E. Cartwright. 1966. Tidal spectroscopy and prediction. Philosophical Transactions of the Royal Society of London A, 259:533–581.
- Neilan, R., P. A. Van Scoy, and P. L. Woodworth, P. L. (Eds.). 1998. Proceedings of the workshop on methods for monitoring sea level: GPS and tide gauge benchmark monitoring and GPS altimeter calibration. Workshop organized by the International GPS Service and Permanent Service for Mean Sea Level, Jet Propulsion Laboratory, 17–18 March 1997.
- Noye, B. J. 1974. Tide-well systems. Journal of Marine Research 32:129–194.
- Palmer, H. R. 1831. Description of a graphical register of tides and winds. Philosophical Transactions of the Royal Society of London 121:209–213.
- Penna, N. T., W. E. Featherstone, J. Gazeaux, and R. J. Bingham. 2013. The apparent British sea slope is caused by systematic errors in the levelling-based vertical datum. Geophysical Journal International 194:772–786.
- Pugh, D. T. 1972. The physics of pneumatic tide gauges. International Hydrographic Review 49(2):71–97.
- Pugh, D. T. 1981. Comparative Tests of Sea Level Data from the Newlyn Tide Well and an Aanderaa Pneumatic System. Report No. 119. Wormley, UK: Institute of Oceanographic Sciences.
- Pugh, D. T. 1982. A comparison of recent and historical tides and mean sea-levels off Ireland. Geophysical Journal of the Royal Astronomical Society 71:809–815.
- Pugh, D. T. and J. M. Vassie. 1980. Applications of the joint probability method for extreme sea level computations. Proceedings of the Institution of Civil Engineers, Part 2 69:959–975.
- Pugh, D. T. and K. R. Thompson. 1986. The subtidal behaviour of the Celtic Sea - I. Sea level and bottom pressures. Continental Shelf Research 5:239–319.
- Pugh, D. T. and P. L. Woodworth. 2014. Sea-level Science: Understanding Tides, Surges, Tsunamis and Mean Sea-level Changes. Cambridge: Cambridge University Press.
- Rossiter, J. R. 1967. An analysis of annual sea level variations in European waters. Geophysical Journal of the Royal Astronomical Society 12:259–299.
- Santamaría-Gómez, A., M. Gravelle, X. Collilieux, M. Guichard, B. M. Míguez, P. Tiphaneau, and G. Wöppelmann. 2012. Mitigating the effects of vertical land motion in tide gauge records using a state-of-the-art GPS velocity field. Global and Planetary Change 98–99:6–17.
- Shennan, I. and P. L. Woodworth. 1992. A comparison of late Holocene and twentieth century sea-level trends from the UK and North Sea region. Geophysical Journal International 109:96–105.
- Shennan, I. and B. Horton. 2002. Holocene land- and sea-level changes in Great Britain. Journal of Quaternary Science 17:511–526.
- Shennan, I., G. Milne, and S. Bradley. 2012. Late Holocene vertical land motion and relative sea-level changes: lessons from the British Isles. Journal of Quaternary Science 27:64–70.
- Tamisiea, M.E, C. W. Hughes, S. D. P Williams, and R. M. Bingley. 2014. Sea level: Measuring the bounding surfaces of the ocean. Philosophical Transactions of the Royal Society of London A, 372:20130336.
- Tappin, D. R., A. Sibley, K. Horsburgh, C. Daubord, D. Cox, and D. Long. 2013. The English Channel ‘tsunami’ of 27 June 2011: A probable meteorological source. Weather 68:144–152.
- Teferle, F. N., R. M. Bingley, S. D. P Williams, T. F. Baker, and A. H. Dodson. 2006. Using continuous GPS and absolute gravity to separate vertical land movements and changes in sea-level at tide-gauges in the UK. Philosophical Transactions of the Royal Society of London 364:917–930.
- Teferle, F. N., R. M. Bingley, E. J. Orliac, S. D. P Williams, P. L. Woodworth, D. McLaughlin, T. F. Baker, I. Shennan, G. A. Milne, S. L. Bradley, and D. Hansen. 2009. Crustal motions in Great Britain: Evidence from continuous GPS, absolute gravity and holocene sea-level data. Geophysical Journal International 178:23–46.
- Thompson, K. R. 1980. An analysis of British monthly mean sea level. Geophysical Journal of the Royal Astronomical Society 63:57–73.
- Thompson, K. R. 1986. North Atlantic sea-level and circulation. Geophysical Journal of the Royal Astronomical Society 87:15–32.
- Tsimplis, M. N., D. K. Woolf, T. J. Osborn, S. Wakelin, J. Wolf, R. Flather, A. G. P Shaw, P. L. Woodworth, P. Challenor, D. Blackman, F. Pert, Z. Yan, and S. Jevrejeva. 2005. Towards a vulnerability assessment of the UK and northern European coasts: The role of regional climate variability. Philosophical Transactions of the Royal Society of London 363:1329–1358.
- Vilibić, I. and J. Šepić. 2010. Long-term variability and trends of sea level storminess and extremes in European Seas. Global and Planetary Change 71:1–12.
- Wadey, M. P., I. D. Haigh, and J. M. Brown. 2014. A century of sea level data and the UK's 2013/14 storm surges: An assessment of extremes and clustering using the Newlyn tide gauge record. Ocean Science 10:1031–1045.
- Wadey, M. P., J. M. Brown, I. D. Haigh, T. Dolphin, and P. Wisse. 2015. Assessment and comparison of extreme sea levels and waves during the 2013/2014 storm season in two UK coastal regions. Natural Hazards and Earth System Sciences Discussions 3:2665–2708.
- Wahl, T., I. D. Haigh, I.D., P. L. Woodworth, F. Albrecht, D. Dillingh, J. Jensen, R. J. Nicholls, R. Weisse, and G. Wöppelmann. 2013. Observed mean sea level changes around the North Sea coastline from the beginning of the 19th century to the present. Earth Science Reviews 124:51–67.
- Werner, C., U. Wegmuller, T. Strozzi, and A. Wiesmann. 2003. Interferometric point target analysis for deformation mapping. IGARSS'03, Toulouse, France, 21–25 July.
- Wessel, P. and W. H. F Smith. 1998. New, improved version of generic mapping tools released. EOS Transactions of the American Geophysical Union 79:579–579.
- Williams, S. D. P 2003. The effect of coloured noise on the uncertainties of rates estimated from geodetic time series. Journal of Geodesy 76:483–494.
- Wöppelmann, G., N. Pouvreau, A. Coulomb, B. Simon, and P. Woodworth. 2008. Tide gauge datum continuity at Brest since 1711: France's longest sea-level record. Geophysical Research Letters 35:L22605.
- Wöppelmann, G., M. Marcos, A. Coulomb, B. Martín Míguez, P. Bonnetain, C. Boucher, M. Gravelle, B. Simon, and P. Tiphaneau. 2014. Rescue of the historical sea level record of Marseille (France) from 1885 to 1988 and its extension back to 1849–1851. Journal of Geodesy 88:869–885.
- Woodworth, P. L. 1987. Trends in UK mean sea level. Marine Geodesy 11:57–87.
- Woodworth, P. L., S. M. Shaw, and D. L. Blackman. 1991. Secular trends in mean tidal range around the British Isles and along the adjacent European coastline. Geophysical Journal International 104:593–610.
- Woodworth, P. L., J. M. Vassie, R. Spencer, and D. E. Smith. 1996. Precise datum control for pressure tide gauges. Marine Geodesy 19:1–20.
- Woodworth, P. L. 1999. High waters at Liverpool since 1768: The UK's longest sea level record. Geophysical Research Letters 26:11.
- Woodworth, P. L., M. N. Tsimplis, R. A. Flather, and I. Shennan. 1999. A review of the trends observed in British Isles mean sea level data measured by tide gauges. Geophysical Journal International 136:651–670.
- Woodworth, P. L., D. E. Smith, R. A. Flather, T. F. Baker, and L. J. Rickards. 2004. Proposals for the development of the UK national tide gauge network. Proudman Oceanographic Laboratory Internal Document No. 161.
- Woodworth, P. L., D. L. Blackman, P. Foden, S. Holgate, K. Horsburgh, P. J. Knight, D. E. Smith, E. A. Macleod, and E. Bradshaw. 2005. Evidence for the Indonesian tsunami in British tidal records. Weather 60:263–267.
- Woodworth, P. L., N. Teferle, R. Bingley, I. Shennan, and S. D. P Williams. 2009a. Trends in UK mean sea level revisited. Geophysical Journal International 176:19–30.
- Woodworth, P. L., N. J. White, S. Jevrejeva, S. J. Holgate, J. A. Church, and W. R. Gehrels. 2009b. Evidence for the accelerations of sea level on multi-decade and century timescales. International Journal of Climatology 29:777–789.
- Woodworth, P. L. 2010. A survey of recent changes in the main components of the ocean tide. Continental Shelf Research 30:1680–1691.
- Woodworth, P. L., C. W. Hughes, R. J. Bingham, and T. Gruber. 2012. Towards worldwide height system unification using ocean information. Journal of Geodetic Science 2(4):302–318.
- Woodworth, P. L. and A. Hibbert. 2015. Sea level monitoring in the British Overseas Territories. Journal of Operational Oceanography 8:123–132.
- Ziebart, M. K., J. C. Iliffe, R. Forsberg, and G. Strykowski. 2008. Convergence of the UK OSGM05 GRACE-based geoid and the UK fundamental benchmark network. Journal of Geophysical Research 113:B12401.