ABSTRACT
A collection of 163 strains of black yeast-like fungi from the CBS Fungal Biodiversity Center (Utrecht, The Netherlands), has been screened for the ability to grow on hexadecane, toluene and polychlorinated biphenyl 126 (PCB126) as the sole carbon and energy source. These compounds were chosen as representatives of relevant environmental pollutants. A microtiter plate-based culture assay was set up in order to screen the fungal strains for growth on the selected xenobiotics versus glucose, as a positive control. Growth was observed in 25 strains on at least two of the tested substrates. Confirmation of substrate assimilation was performed by cultivation on closed vials and analysis of the headspace composition with regard to the added volatile substrates and the generated carbon dioxide. Exophiala mesophila (CBS 120910) and Cladophialophora immunda (CBS 110551), both of the order Chaetothyriales and isolated from a patient with chronic sinusitis and a polluted soil sample, respectively, showed the ability to grow on toluene as the sole carbon and energy source. Toluene assimilation has previously been described for C. immunda but this is the first account for E. mesophila. Also, this is the first time that the capacity to grow on alkylbenzenes has been demonstrated for a clinical isolate. Assimilation of toluene could not be demonstrated for the human opportunistic pathogen Pseudoallescheria boydii (CBS 115.59, Microascales), but the results from microtiter plate assays suggest that strains of this species are promising candidates for further studies. The outstanding abilities of black yeast-like fungi to thrive in extreme environments makes them ideal agents for the bioremediation of polluted soils, and for the treatment of contaminated gas streams in biofilters. However, interrelations between hydrocarbonoclastic and potentially pathogenic strains need to be elucidated in order to avoid the possibility of biohazards occurring.
KEYWORDS:
Introduction
Contamination of the entire biota by man-made compounds (xenobiotics) is now a worldwide phenomenon affecting natural environments, agricultural sustainability and food safety. Aromatic hydrocarbons, such as benzene, toluene, ethylbenzene and xylene isomers (collectively known as BTEX), form one of the most abundant categories of pollutants, being as they are important components of crude oil and fuels. Moreover, since BTEX compounds are released in the partial combustion of coal and other fuels, their emissions from engines are subject to strict environmental regulations in developed countries (Mehlman et al. 1992). These toxic compounds are usually difficult to remove, due, in part, to their wide dispersal in the ecosystem, leading to concentration levels which still constitute a hazard but where chemical or physical removal is not economically viable.
Among the emerging technologies for air pollution control, biofiltration is a promising, relatively cost-effective, alternative. Basically, contaminated gases are passed through a bed filter made of solid support media, where the biomass is present as an active layer. Nowadays, most of the biofilters available on the market are based on the activity of selected bacteria (de Lorenzo Citation2009). Nevertheless, the use of bacteria-based biofilters has its drawbacks, such as reduced performance upon dehydration and acidification of the media (Agathos et al. Citation1997; Auria et al. Citation2000).
Bioremediation performed by fungi has begun to be recognized as a promising alternative. Recent research has revealed a high diversity of fungi with degrading abilities towards aliphatic and aromatic hydrocarbons, together with remarkable levels of tolerance and adsorption of heavy metals (Prenafeta-Boldú et al. Citation2001a, Citation2004, Citation2006; Tan and Cheng Citation2003). Fungi exhibit many positive characteristics that confer higher competitiveness compared to bacteria: the hyphal growth, which allows the fungi to spread easier and faster through a large volume of material; the production of extracellular enzymes that can contribute to a more efficient bioremediation process; and a more general ability to withstand a wide range of environmental conditions.
In recent decades two fungal groups in particular, the black yeasts and the microcolonial fungi, have begun to be recognized for their potential with regard to bioremediation purposes. Some of these fungi have in fact been isolated from different polluted sources, such as industrial spills, car gasoline tanks, railway sleepers and air biofilters. The common characteristic of these fungal group members is the presence of melanin, constitutively expressed and deposited at the cell wall level. In particular, the genera Exophiala and Cladophialophora (order Chaetothyriales), and Pseudallescheria (order Microascales) have a high potential to grow in polluted environments and to metabolize hydrocarbons as the sole source of carbon and energy (April et al. 1998; de Hoog et al. Citation2003, Citation2006, 2011).
The outstanding ability of the black fungi to thrive in environments with extreme conditions, with respect to temperature, water availability, free radicals, UV irradiation and scarcity of nutrients, is beneficial from the point of view of their use in another extreme environment, as a biofilter (Butler and Day Citation1998; de Hoog Citation1999). On the other hand, these two fungal orders are also characterized by the fact that they encompass an unusually high number of opportunistic pathogens, which might hinder the application of fungi in bioremediation (Prenafeta-Boldú et al. Citation2006).
In this study, we developed a fast and efficient prescreening method with the aim of finding new fungal species with pollutant degradation abilities. Fungal cultures were incubated with selected pollutants as the sole carbon and energy source and biomass growth was measured by optical density. The results of the prescreening facilitates narrowing the field of strains to be further investigated for their degradation abilities by performing carbon mass balances experiments in sealed vials.
In particular, a culture collection of 163 fungal strains, mainly members of the dematiaceous fungi group, has been tested for growth on toluene, hexadecane and PCB 126 as the sole carbon and energy sources. These compounds are representative members of the aromatic and aliphatic hydrocarbons, and polychlorinated biphenyls, respectively.
Materials and methods
Fungal strains and cultivation
A total of 163 fungal strains were provided by the CBS-KNAW Fungal Diversity Center (Utrecht, The Netherlands). They belong to 3 different orders and a total of 9 genera (). We have included more than one strain for each species in the collection, in order to verify how the performance of the species can vary between strains isolated from different environmental or clinical sources. The strains were collected from all five continents, with a prevalence of sites from European and American countries. The isolation sources of the strains were quite diverse, ranging from environmental samples from, for example, gasoline tank, soil, dung, process water, and mud samples, to clinical samples from, for example, keratitis, sinusitis, sputum and granuloma. The strains were sent as freeze-dried cultures in glass ampoules. They were revived by pouring into 2 ml malt peptone solution [MEA: 2% malt extract, 0.1% bacto-peptone] before being kept room temperature for at least 5 h. They were then grown at room temperature on Petri dishes containing MEA.
Table 1. Orders and genera of the screened fungi collection.
Microtiter plate-screening
To screen the fungal collection for the ability to use hydrocarbons as sole carbon source, we set up a growth test in Microtiter plates and measured the optical density of the fungal cultures over time. A cell suspension of each fungus was obtained by treating the biomass with a Rybolizer (FastPrep-24 Instrument, MP Biomedicals, Santa Ana, CA) for 5 sec at 4 m/s in the presence of sterile 0.9% NaCl solution and glass beads (Carl Roth, Karlsruhe, DE). Each fungal strain was then cultivated on the Microtiter plate in duplicate under two different conditions, both used as positive controls: (i) a trace element solution with glucose and vitamin solution, and (ii) a glucose solution (for the exact composition of the trace element, vitamin and glucose solutions consult the Supplementary Material).
In addition, the fungal strains were cultivated in quadruplicate when grown in the presence of each hydrocarbon (toluene, hexadecane or PCB126). In the latter case, 50 µL of hexadecane (99% analytical grade, Alfa Easar) or PCB126 (10 ng/μL PCB 126 in isooctane, Dr. Ehrenstorfer GmbH) were added to 150 μL of trace element media and vitamins. Toluene (Merck KGaA, Darmstadt, DE), however, was not added directly to the media due to its volatile character. Between measurements, the toluene microtiter plates were stored in a glass vacuum desiccator together with a beaker filled with toluene.
The microtiter plates of hexadecane and PCB 126 screening, however, were kept on a shaker at room temperature between measurements. For positive control wells, 200 µL of trace element media plus vitamins and glucose were added. In the negative control wells, 200 μL of trace elements media plus vitamins were added. In each well, with the exception of the abiotic blanks, 20 µl of fungal suspension were added. The OD measurements were performed with the microplate reader Infinite M 1000 (Tecan, Männedorf, CH) every 2 days for a total of 40 days. The trace element solution or glucose solution without fungal inoculation was used as the blank for the OD readings. To calculate the change in OD 700, the blanks were subtracted from the OD 700 values of all 163 strains.
Growth tests in sealed vials
To validate the efficiency of the microtiter plate screening, 25 strains were selected for a subsequent quantitative assessment of the toluene assimilation capacity by monitoring of both toluene consumption and carbon dioxide accumulation in the head-space. Batch cultures (25 mL) were incubated under static conditions at 25°C, as described previously (Prenafeta-Boldú et al. Citation2001a).
In order to prevent toluene leakage and ensure enough oxygen content, 250-mL Boston flasks sealed with Teflon Miniert valves (Phase Separations, Waddinxveen, The Netherlands) were used. The bottles were filled with 25 mL of buffered mineral medium (Hartmans and Tramper Citation1991) with a pH of 7 and then autoclaved at 120°C for 15 min. The sterile filtrated vitamin solution was added afterwards under sterile conditions. The amount of added toluene resulted in concentrations in the liquid phase below the known toxicity level for black yeast (Prenafeta-Boldú et al. Citation2001a), and according to the water/air partition coefficient (Amoore and Hautala Citation1983). The toluene (6.08 mg) was added with a Hamilton microsyringe. Three types of batch reactors were prepared:
Negative Control: 25 mL mineral media + 0.3 mL of inoculum;
Positive Control: 25 mL mineral media + 0.3% glucose + toluene + 0.3 mL inoculum;
Hydrocarbons: 25 mL mineral media + toluene + 0.3 mL inoculum.
The inocula were prepared as suspensions of fungal spores by transferring a 1-cm2 agar plug from biomass pregrown on MEA plates to sterile water. After vortexing, spore suspensions were injected into the bottles with a needle, under sterile conditions. The inoculation was performed after the addition of the volatile carbon sources, when the water/air partition had reached equilibrium. The bottles were kept at 25°C in the incubator under static conditions. The fungus Cladophialophora immunda was used as a positive control due to its well-known capacity to grow on toluene (Prenafeta-Boldú et al. Citation2001a).
Fungal growth was monitored by visual observation. The degradation of toluene was measured by Gas Chromatography with a Flame Ionization Detector (GC-FID, Trace 2000 series, Thermo Quest CE Instruments). The method settings for toluene measurements were the following: oven temperature at 180°C; hold time of 2.00 min; sample injection in split mode. Then 100 μL of the headspace of the Teflon coated bottles was injected, by means of a Hamilton micro syringe, into the GC-column. To calculate the amount of toluene, a calibration curve had been generated beforehand.
The CO2 production was evaluated by a Thermal Conductivity Detector-Gas Chromatographer (GC-TCD, Varian CP-3800, Varian, Palo Alto, CA). The GC-column temperature was set at 90°C and both the detector and injector temperatures were set as 180°C. For injecting the sample volume of 200 μL, a Hamilton SGE syringe was used. Furthermore, two standards were run to determine the performance of the instrument. The measurement was carried out over 30 days, with the starting point for toluene measurements assigned as day 0. The CO2 measurement commenced whenever hydrocarbon depletion was measured or growth could be seen optically. Results were corrected by a daily factor to account for instrument variability which was calculated by measuring two standards before and after the measurements.
Screening of the genomes of hydrocarbonoclastic black yeasts for genes related to toluene degradation
The sequence of the genes reported to be involved in the fungal degradation pathway of toluene (Parales et al. Citation2008) were obtained from UniProt and KEGG. The genomes of Cladophialophora psammophila, Cladophialophora. immunda (WGS No. JSEJ01, Sterflinger et al. Citation2015) Exophiala xenobiotica and Exophiala mesophila (WGS No. JTCI01 and JSEI01, Tafer et al. Citation2015) were searched for homologs to these sequences with the help of the Scipio (Keller et al. Citation2008) protein mapper. For a comparison with a model yeast, the genome of Saccharomyces cerevisiae (strain S288C) was also searched for homologs to these genes. The Scipio score threshold was set to 0.3, the tile size set to 5 and the minimum sequence identity set to 50%. All the annotated genomes of the black fungi group were searched through NCBI BLAST (http://blast.ncbi.nlm.nih.gov/Blast.cgi) for a peptide sequence, SQEEIDAVI, that has been found in the toluene monooxygenase of C. saturnica (CBS 114326) with a similarity of 89% with different cytochrome P450 (Luykx et al. 2003).
Results
Microtiter plate screening
In the analyzed data set, 73 strains out of 163 grew in the presence of at least one of the hydrocarbons (). Some fungal strains were able to grow up to levels comparable with the positive controls, yet others have exhibited only a slight rise in the optical density from the beginning to the end of the measurements. One strain, Pseudoallescheria boydii (CBS 115.59), was able to grow in the presence of all three hydrocarbons. A total of 14 strains were able to grow in the presence of two of the three hydrocarbons, while 19 grew in the presence of only one hydrocarbon.
Table 2. List of the screened fungal collection.
The two positive controls, (i) glucose with vitamins and trace elements and (ii) glucose without vitamins and trace elements, exhibited similar results for all the fungi tested. Therefore, we can assume that neither the lack of vitamins nor of trace elements inhibit growth of the strains. Optically, it was possible to check for contamination, for example, if there was fungal growth outside the wells or on the plate cover. Nine strains were not able to grow after reviving the freeze-dried culture.
Growth tests on sealed vials
To test the efficiency of the microplate technique as a prescreening tool for selection of fungal strains able to grow on hydrocarbons and related contaminants as the sole source of carbon and energy, carbon mass balances were carried out in closed batch cultures. For this purpose, 25 fungal strains were chosen according to their positive growth scores on the microtiter plate screening, including at least one of the substrates and one strain among those that did not grow in presence of any of the three pollutants. These 25 strains are listed in together with their country of origin and collection site.
Table 3. List of fungi selected for the GC-screening.
After a few days of inoculum, fungal growth was observed in all glucose-amended positive controls, comparable to samples with sole hydrocarbon source (data not shown). In addition, a comparison with the negative controls was needed, as some of the fungal strains were able to sporulate without any carbon source, giving rise to wrong data interpretation if based only on biomass observations.
From among the 25 strains tested, two strains, Cladophialophora immunda (CBS 110551) and Exophiala mesophila (CBS 120910), showed the ability to completely degrade toluene. This was confirmed by a concurrent CO2 and toluene uptake in the test cultures.
However, 10 other strains, which from microtiter plate screening seemed able to grow on toluene, showed neither significant CO2 production nor toluene degradation. In fact, the observed optical density was related to fungal sporulation, based on comparisons with negative controls. The other 13 strains exhibiting positive results only for hexadecane or PCB126 at the first screening, gave effectively negative results for toluene assimilation.
As shown in , the performance of the fungi with respect to biodegradation abilities are strain-specific, rather than representative of the whole species, and are most probably related to the different selective pressures acting in different isolation sources.
Cladophialophora immunda
The microtiter plate screening of Cladophialophora immunda (strain 17, CBS 110551) was negative for both hexadecane and PCB 126, but positive for toluene. The OD 700 for toluene screening varied between the two plates, but showed an increasing trend (). Optical detection of the GC bottles also showed more growth in the sample with a sole hydrocarbon source than in the negative control (data not shown).
Figure 1. Graph of toluene microtiter plate-screening of Cladophialophora immunda (strain 17, CBS 110551). Optical density (700 nm) is plotted against time (days). The graph is derived from the average data points of duplicates. Three growth curves are represented: medium plus hydrocarbon (H), medium plus glucose (G), medium plus glucose, vitamins and trace elements (GVT).
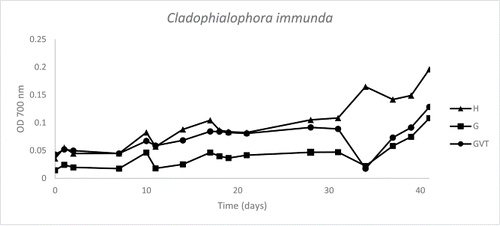
In , the results of toluene degradation and CO2 production from Chladophialophora immunda over time are shown. At the point where toluene is completely degraded, CO2 also reached saturation level. Around 65% of the toluene carbon was recovered as C-CO2.
Exophiala mesophila
In the microtiter plate screening, Exophiala mesophila (strain 64, CBS 120910) exhibited clear growth on toluene () and hexadecane, while no growth could be detected in presence of PCB 126 (data not shown). In , the toluene degradation and CO2 production from Exophiala mesophila over time are shown. The analysis of the headspace showed that the strain is able to completely degrade toluene into CO2. Specifically, around 65% of the C-toluene was recovered as C-CO2.
Figure 3. Graph of toluene microtiter plate-screening of Exophiala mesophila (strain 64, CBS 120910). Optical density (700 nm) is plotted against time (days). The graph is derived from the average data points of duplicates. Three growth curves are represented: medium plus hydrocarbon (H), medium plus glucose (G), medium plus glucose, vitamins and trace elements (GVT).
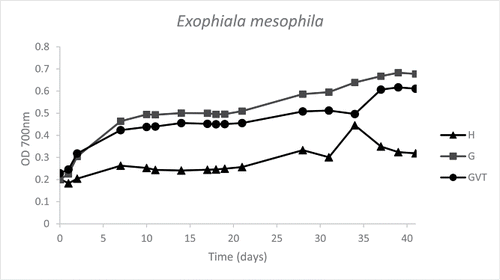
Screening of the genomes of hydrocarbonoclastic black yeasts for genes related to toluene degradation
A toluene degradation pathway in fungi was first proposed for Cladosporium sphaerospermum (reclassified as Cladophialophora saturnica CBS 114326; Badali et al. Citation2008) according to enzyme assays, oxygen uptake and substrate consumption (Weber et al. 1995). This pathway was subsequently extended to other toluene-growing fungi, including C. immunda (Prenafeta-Boldú et al. 2001b). Here, the genomes of Cladophialophora immunda and Exophiala mesophila were examined for the presence of genes belonging to the pathway, together with the genome of the model yeast Saccharomyces cerevisiae S288c, and two other dematiaceous fungi known for their ability to degrade xenobiotics, Cladophialophora psammophila, and Exophiala xenobiotica (Badali et al. Citation2001; de Hoog et al. Citation2006). In the genome of S. cerevisiae, only the first two enzymes of the pathway, cytochrome P450 and the benzyl alcohol dehydrogenase, are present. In the other four genomes, however, three genes of the pathway appear to be missing, namely p-hydroxybenzoate hydroxylate, muconolactone isomerase and β-ketoadipate enol-lactone hydrolase ().
Figure 5. Toluene degradation pathway in fungi. Enzymes that are differently present in the five genomes are highlighted (modified from Parales et al. 2008).
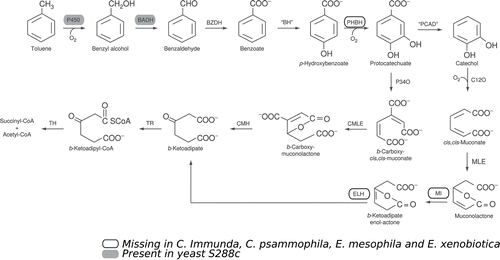
In the enzymatic study of Luykx et al. (2003) performed on C. saturnica CBS 114326, a direct connection between toluene metabolism and cytochrome P450 was established (Luykx et al. 2003). The characterization of the toluene monooxygenase revealed, among other things, that the protein has an internal peptide sequence, SQEEIDAVI, that shared similarities with several mammalian cytochrome P450, a soybean P450 and eukaryotic alkane inducible P450 enzymes with a similarity of 89%. By analyzing a restricted group of fungi from our collection for which the genome annotation is available, we found the same sequence, with 89% identity, in the genomes of Cladophialophora and Exophiala species, but not in Scedosporium apiospermum and Phialophora americana ().
Table 4. List of fungal genomes analyzed for the presence of the conserved peptide sequence of cytochrome P450.
Discussion
Anthropogenic pollution is becoming a ubiquitous phenomenon, giving rise to the need to address the problem with new and economically sustainable practices. From this perspective, bioremediation offers an efficient and cost-effective alternative to standard remediation procedures.
Although bacteria have been the most frequently used organisms in environmental biotechnology applications to date, certain fungi show better degrading performances in several polluted environments characterized by growth-limiting conditions, such as extreme pH, salinity, presence of toxic chemicals, oligotrophy, etc. (Harms et al. Citation2011; Prenafeta et al. Citation2006).
The necessity to explore fungal biodiversity to find new candidates for bioremediation purposes has led to the development of the relatively fast and simple screening method presented here. A range of preselection of fungal strains capable of growth in the presence of polluting molecules was assayed on hexadecane, toluene, and PCB 126, representing aliphatic and aromatic hydrocarbons, and halogenated aromatic compounds, respectively.
The screening has proven to be a fast method, since, in forty days, or even less for some species, it has been possible to state whether or not a fungus could actively grow in the presence of a specific xenobiotic. Moreover, the use of 96-well plates for the fungal growth makes it possible to comfortably handle a large quantity of strains simultaneously. As a result, this method is high-throughput and can be exploited to screen the effect of any other molecule on the fungal growth.
The objects of our study are black meristematic fungi, a functional group that shares the common trait of a strongly melanized cell wall and resistance to extreme environments with respect to water and nutrient availability, pH, and UV radiation (Sterflinger Citation2006). For each species included in the experiment, tests were conducted on different strains, which had, in turn, been isolated from diverse sources: environmental (either unspoilt or polluted) and medical.
The wood decay fungi normally present in the soil, for example, are also well known for their potential in the biodegradation of recalcitrant organopollutants (Yadav and Reddy Citation1993). Their ecological role as decomposers is based on a set of ligninolytic enzymes that are, however, coincidentally involved in degrading aromatic hydrocarbons (Baldrian et al. Citation2000; Pozdnyakova Citation2012), including BTEX (Yadav and Reddy Citation1993). Yet, in ligninolytic fungi, their ability to biodegrade xenobiotics arises as a result of the unspecific and very high redox potential of peroxidases and laccases involved in lignin co-metabolic breakdown (Hammel Citation1995).
Regarding black yeasts, however, the assimilatory metabolism of xenobiotics is due to a well-arranged pathway of energy-yielding reactions that leads to the degradation of those substrates. As in all noncoincidental metabolisms, the evolution of this capability must be the result of the selective pressure of specific environmental factors which have yet to be fully understood.
A dual ecology also characterizes several related species of the order Chaetothyriales, particularly in the genera Exophiala and Cladophialophora, which have been found not only in hydrocarbon-rich environments but also in human tissue (de Hoog et al. Citation2006; Prenafeta-Boldú et al. Citation2001a, Citation2006). In some cases, both ecological traits, assimilation of aromatic hydrocarbons and pathogenicity, appear to be clearly differentiated in otherwise closely related and even con-specific species, such as Cladophialophora psammophila and C. bantiana (Badali et al. Citation2008). Despite their phylogenetic proximity, the first species was isolated from polluted soil efficiently growing on toluene and proved to be nonpathogenic, while the second is perhaps the most dangerous fungus known to date as the agent responsible for fatal encephalitis.
The common chemical nature of alkylbenzenes and their metabolites, as well as some neurotransmitters, has been concluded to be a possible explanation of this ecological adaptation. Hence, it is of fundamental importance to ascertain to what extent hydrocarbon metabolism and virulence may be coincident, in order to guarantee biotechnological applications that are reasonably free of biohazard.
In this respect, the results of our screening show that the degrading capability of the fungi from our collection might not be restricted to those isolated from a polluted source, since strains of medical origin and other unpolluted sources were also able to grow in presence of the tested hydrocarbons.
Seventy-three fungal strains were able to grow with at least one of the tested model pollutants. Surprisingly, substrate assimilation could only be verified for two strains and on toluene. This very limited outcome depicts the difficulties related to the capacity of fungi, particularly from among the black yeasts, to thrive under growth-limiting conditions, and points to the fact that assimilation of alkylbenzenes is restricted to relatively few strains. Similar poor results have been obtained in previous substrate specificity surveys on culture collections when compared to environmental enrichments (Prenafeta-Boldú et al. Citation2001a). Thus, the proposed microtiter prescreening method must be regarded as an initial stage in the selection of potential candidates to be used in bioremediation purposes, but more in-depth studies are still needed to prove their biodegradation capacities.
The two fungi exhibiting positive growth for toluene, Cladophialophora immunda and Exophiala mesophila were isolated from a gasoline station and a patient with chronic sinusitis, respectively. The ability of C. immunda to grow with toluene as the sole carbon source has already been documented (Prenafeta-Boldú et al. Citation2001a), and one strain in particular (CBS 110551) was able to convert up to the 65% of the toluene in CO2, in terms of carbon mass equivalents. Concerning E. mesophila (CBS 120910), toluene uptake was also related to significant CO2 recovery, and this work is the first account of toluene assimilation by this species. Considering the clinical origin of the strain, this is definitely a remarkable result as it demonstrates that strains from clinical origin can also assimilate alkylbenzenes.
Besides the well-separated assimilation of aromatics and pathogenicity among sibling species (e.g., C. psammophila and C. bantiana), or within strains of the same species, e.g. Exophiala oligosperma (de Hoog et al. Citation2003), it is now clear that both the metabolic capacity to grow on alkylbenzenes and virulence can be coincident in the same strain.
We also approached the study of the toluene degradation pathway through genome analysis of C. immunda and E. mesophila, and also of other two black fungi with xenobiotic degradation abilities, C. psammophila and E. xenobiotica. Three of the genes belonging to the toluene degradation pathway hypothesized for the fungi (Weber et al. 1995) were not found in any of the analyzed genomes, most probably due to the assembly of the sequences.
The search for the internal peptide sequence characterizing a cytochrome P450 monooxygenase reveals a selective presence in the two genera, Exophiala and Cladophialophora, in which the toluene assimilation is predominant. The presence of the protein known to oxidize toluene at the methyl group, indicates a quite conserved toluene metabolic pathway in fungi. Finding the missing genes responsible for the subsequent attack on the benzyl alcohol is the next important goal to reach in order to confirm the pathway proposed by Prenafeta-Boldú et al. (2001). The aim of the ongoing research of our group is to identify, by means of RNA-seq technology, which genes are differentially expressed when the fungus is exposed and grows with toluene as the sole carbon and energy source.
Supplementary Material
Download MS Word (14.8 KB)Acknowledgments
The computational results presented were achieved, in part, through use of the Vienna Scientific Cluster (VSC).
Funding
This project was financed by the Austrian Science Fund (FWF) project (P 25119-B16). The equipment of the VIBT-Extremophile Center used in this study was financed by BOKU-Equipment GesmbH.
References
- Agathos SN, Hellin E, Ali-Khodja H, Deseveaux S, Vandermesse F, Naveau H. 1997. Gas-phase methyl ethyl ketone biodegradation in a tubular biofilm reactor: microbiological and bioprocess aspects. Biodegradation 8:251–264.
- Amoore JE, Hautala E. 1983. Odor as an aid to chemical safety: odor thresholds compared with threshold limit values and volatilities for 214 industrial chemicals in air and water dilution. J Appl Toxicol 3:272–290.
- April TM, Abbott SP, Foght JM, Currah RS. 1998. Degradation of hydrocarbons in crude oil by the ascomycete Pseudallescheria boydii (Microascaceae). Can J Microbiol 44:270–278.
- Auria R, Frere G, Morales M, Acuña ME, Revah S. 2000. Influence of mixing and water addition on the removal rate of toluene vapors in a biofilter. Biotechnol Bioeng 68:448–455.
- Badali H, Gueidan C, Najafzadeh MJ, Bonifaz A, van den Ende AH, de Hoog GS. 2008. Biodiversity of the genus Cladophialophora. Stud Mycol 61:175–191.
- Badali H, Prenafeta-Boldu FX, Guarro J, Klaassen CH, Meis JF, de Hoog GS. 2001. Cladophialophora psammophila, a novel species of Chaetothyriales with a potential use in the bioremediation of volatile aromatic hydrocarbons. Fung Biol 115:1019–1029.
- Baldrian P, in der Wiesche C, Gabriel J, Nerud F, Zadrazil F. 2000. Influence of cadmium and mercury on activities of ligninolytic enzymes and degradation of polycyclic aromatic hydrocarbons by Pleurotus ostreatus in soil. Appl Environ Microb 66:2471–2478.
- Butler MJ, Day AW. 1998. Fungal melanins: a review. Can J Microbiol 4:1115–1136.
- de Hoog, GS (ed.). 1999. Ecology and evolution of black yeasts and their relatives. Stud Mycol 43:1–208.
- de Hoog GS, Vicente V, Caligiorne RB, Kantarcioglu S, Tintelnot K, Gerrits van den Ende AHG, Haase G. 2003. Species diversity and polymorphism in the Exophiala spinifera clade containing opportunistic black yeast-like fungi. J Clin Microbiol 1:4767–4778.
- de Hoog GS, Vicente VA, Najafzadeh MJ, Harrak MJ, Badali H. 2011. Waterborne Exophiala species causing disease in cold-blooded animals. Persoonia 27:46–72.
- de Hoog GS, Zeng JS, Harrak MJ, Sutton DA. 2006. Exophiala xenobiotica sp. nov., an opportunistic black yeast inhabiting environments rich in hydrocarbons. Anton Van Leeuwen 90:257–268.
- de Lorenzo V. 2009. Recombinant bacteria for environmental release: what went wrong and what we have learnt from it. Clin Microbiol Infect 15:63–65.
- Hammel KE. 1995. Mechanisms for polycyclic aromatic hydrocarbon degradation by ligninolytic fungi. Environ Health Perspect 103:41–43.
- Harms H, Schlosser D, Wick LY. 2011. Untapped potential: exploiting fungi in bioremediation of hazardous chemicals. Nat Rev Microbiol 9:177–192.
- Hartmans S, Tramper J. 1991. Dichloromethane removal from waste gases with a trickle bed reactor. Bioproc Eng 6:83–92.
- Keller O, Odronitz F, Stanke M, Kollmar M, Waack S. 2008. Scipio: Using protein sequences to determine the precise exon/intron structures of genes and their orthologs in closely related species. BMC Bioinform 9:278.
- Luykx DM, Prenafeta-Boldú FX, de Bont JA. 2003. Toluene monooxygenase from the fungus Cladosporium sphaerospermum. Biochem Biophys Res Comm 312:373–379.
- Mehlman MA. 1992. Dangerous and cancer-causing properties of products and chemicals in the oil refining and petrochemical industry: VIII. Health effects of motor fuels: carcinogenicity of gasoline: Scientific update. Environ Res 59:238–249.
- Parales RE, Parales JV, Pelletier DA, Ditty JL. 2008. Diversity of microbial toluene degradation pathways. Adv Appl Microbiol 64:1–73.
- Pozdnyakova N. 2012. Involvement of the ligninolytic system of white-rot and litter-decomposing fungi in the degradation of polycyclic aromatic hydrocarbons. Biotechnol Res Int 2012 ID 243217 doi:10.1155/2012/243217.
- Prenafeta-Boldú FX, Ballerstedt H, Gerritse J, Grotenhuis JTC. 2004. Bioremediation of BTEX hydrocarbons: effect of soil inoculation with the toluene-growing fungus Cladophialophora sp. strain T1. Biodegradation 15:59–65.
- Prenafeta-Boldú FX, Kuhn A, Luykx DMAM, Anke H, Groenestijn JW Van, de Bont JAM. 2001a. Isolation and characterisation of fungi growing on volatile aromatic hydrocarbons as their sole carbon and energy source. Mycol Res 105:477–484.
- Prenafeta-Boldú FX, Luykx DMAM, Vervoort J, de Bont JAM. 2001b. Fungal metabolism of toluene: monitoring of fluorinated analogs by 19F nuclear magnetic resonance spectroscopy. Appl Environ Microbiol 67(3):1030–1034.
- Prenafeta-Boldú FX, Summerbell R, de Hoog GS. 2006. Fungi growing on aromatic hydrocarbons: biotechnology's unexpected encounter with biohazard? FEMS Microbiol Rev 30:109–130.
- Sterflinger K. 2006. Black yeasts and meristematic fungi: Ecology, diversity and identification. In: Gabor R, Rosa C, editors. Biodiversity and Ecophysiology of Yeasts. The Yeast Handbook. Berlin Heidelberg: Springer, p501–514.
- Sterflinger K, Lopandic K, Blasi B, Poynter C, de Hoog S, Tafer H. 2015. Draft genome of Cladophialophora immunda, a black yeast and efficient degrader of polyaromatic hydrocarbons. Genome Announc 3(1):e01283–14. doi:10.1128/genomeA.01283-14.
- Tafer H, Lopandic K, Blasi B, Poyntner C, Sterflinger K. 2015. Draft genome sequence of Exophiala mesophila, a black yeast with high bioremediation potential. Genome Announc 3(2):e00203–15. doi:10.1128/genomeA.00203-15.
- Tan T, Cheng P. 2003. Biosorption of metal ions with Penicillium chrysogenum. Appl Biochem Biotechnol 104:119–128.
- Weber FJ, Hage KC, de Bont JA. 1995. Growth of the fungus Cladosporium sphaerospermum with toluene as the sole carbon and energy source. Appl Environ Microbiol 61:3562–3566.
- Yadav JS, Reddy CA. 1993. Degradation of benzene, toluene, ethylbenzene, and xylenes (BTEX) by the lignin-degrading basidiomycete Phanerochaete chrysosporium. Appl Environ Microbiol 59:756–762.