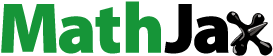
ABSTRACT
A new eco-friendly bucky gel nano sorbent consisting of magnetic grapheneoxide (MGO) and an ionic liquid (IL) was used based on dispersive extraction technique followed by cold vapor atomic absorption spectroscopy for determination of mercury in river water, milk, omega-3 supplements, and lipstick. The optimum conditions for extraction were 50 mg of sorbent (mass ratio IL/MGO: 26), 8 min vortexing, acetate buffer pH = 4, and for desorption 3 min vortexing of HNO3 (1 mL). The limits of detection, quantification, preconcentration factor and extraction recovery were found at 0.57, 1.88 µg L−1, 21 and 84%. Relative standard deviation (RSD) was 6.5% (n = 3).
Introduction
Heavy and hazardous metal ions are spread widely in the environment from different sources such as industry, mining, and coal-fired power plants. Heavy metal ions that are also widely found in the earth’s crust bring serious effects on environment and human health. They are nonbiodegradable in nature and over the last few years, determination of trace amounts of these ions in natural polluted sources has received large attention.[Citation1]
Mercury is known as one of the most critical contaminants in air, soil, and water, and is transferred to organisms by inhalation and oral intake, and may retain and accumulate in human tissues, especially in the brain and kidneys. Cutaneous absorption of Hg+2 could occur by cosmetics such as skin-lightening products[Citation2] and lipstick[Citation3] containing mercury salts. Some types of light bulbs,[Citation4] pharmaceuticals, and batteries[Citation5] are other sources of mercury contamination. Because of the adverse effect of this hazardous compound on living organisms, and human health, determination of trace amounts of mercury is a very important subject for analytical scientists and environmentalists. Monitoring of low mercury concentrations demands highly sensitive detection methods. The mercury determination step is relatively simple, and it has been performed by different methods including inductively coupled plasma mass spectroscopy (ICP-MS),[Citation6] cold vapor atomic fluorescence spectroscopy (CVAFS),[Citation7] cold vapor atomic absorption spectroscopy (CV-AAS),[Citation8–Citation14] ICP-MS,[Citation15] and cold vapor/hydride generation atomic absorption spectroscopy (CV/HG-AAS).[Citation16–Citation19] Even with the high selectivity and sensitivity of above-mentioned analytical techniques, preconcentration of hazardous trace elements before their analysis is crucial due to the often very low concentrations in numerous samples (especially water samples). However, most of the sample preparation methods applied to extract the trace amounts of mercury from various samples are costly and time-consuming. One of the most common methods for preconcentration of low levels of compounds is liquid-liquid extraction,[Citation20] which has been limited because of the use of a high amount of organic solvents. These days, scientists tend to utilize miniaturization and simplification of the preconcentration steps, so solid phase microextraction (SPME),[Citation21] dispersive liquid-liquid microextraction (DLLME),[Citation22] single-drop microextraction,[Citation23] cold-induced aggregation microextraction,[Citation24] and electromembrane extraction[Citation25] have been widely used as extraction techniques. All of these techniques have pros and cons that make each of them more preferable than the others for specific analytical problems. Nano materials have attracted lots of attention for numerous technologies due to their special properties and main advantages like high surface-to-volume ratio, easy derivatization procedures, and unique thermal, mechanical or electrical properties. Carbon-based materials that have been investigated as the sorbent materials in sample preparation are widely used in cleanup techniques.[Citation26] A variety of sorbents including carbon nanotubes (CNT) and graphene oxide (GO)[Citation27] have been reported for the removal of metal ions from aqueous solutions in sample pretreatment.[Citation28,Citation29] GO offers a large specific surface area on both sides of the planar sheet including a variety of oxygenated functional groups leading to a strong π-π interaction with aromatic compounds, and has been extensively investigated to remove pollutants from sample solutions.[Citation30] Some problems are reported for using GO as adsorbents in solid-phase extraction (SPE) such as irreversible aggregation, which may decrease the adsorption capacity of the sorbent.[Citation31] GO can be modified with magnetic nanoparticles (MNPs), and used in magnetic solid-phase extraction, which can reduce the equilibrium time due to the fast mass transfer. An external magnetic field can be used to separate the sorbent including analytes from the sample solution very easily.[Citation32,Citation33] To improve the ability of MNPs for selective preconcentration and extraction of analytes, surface modification plays an important role. Room-temperature ionic liquids and organic molten salts are one of the new solvents in sample preparation, with melting points at or below 100°C, and a wide range of combinations of cations and anions. They have unique physicochemical properties including negligible vapor pressure, and good extraction ability for various organic and inorganic compounds. The option for tunable viscosity and ability to be miscible in water and organic solvents attract lots of attention for ILs as extractants in microextraction techniques.[Citation34,Citation35] Metal ions tend to stay in the aqueous phase but their hydrophobicity will be increased by complexation with a suitable ligand. Hydrophobic ILs with hexafluorophosphate or bis [(trifluoromethyl) sulfonyl] imide anions have the ability to extract hydrophobic compounds like complex ions in biphasic solutions.[Citation36] In 2003, Takuzo Aida and coworkers[Citation37,Citation38] discovered the gelatinous soft materials combining single-walled carbon nanotubes and ILs. This soft material that was prepared by mixing ILs and carbon-based materials in a mortar or powerful sonicating is called bucky gel. The potential ability of ILs to bind to the carbon network structures of CNTs via π-cation/π- electronic interaction causes strong connections by physical crosslinking.[Citation39] The hypothesis of the present work was that by using mixtures of IL with magnetic graphene oxide (MGO) making a viscous gel would give the advantages of the IL and the MGO simultaneously. This type of compound has the potential to adsorb a wide range of organic and inorganic analytes from a complex matrix. Development and application of this new generation of sorbents encouraged us to use the bucky gel consisting of MGO and 1-butyl-3-methylimidazolium hexafluorophosphate ([BMim] PF6) as an IL in the field of separation and extraction of heavy metal ions from different matrices. The magnetic bucky gel has been applied for extraction and preconcentration of mercury in complexation with dithizone (DTZ) by magnetic dispersive solid-phase extraction prior to detection by CV-AAS.
This study was performed to investigate the applicability of this new sorbent on different complex matrices. Lipstick with ingredients including waxes, oils, dyes, and pigments and omega-3 supplements with long chain fatty acids is known as difficult matrices. Compatibility of the lipstick and omega-3 capsules with the proposed method was a challenge in this project. To the best of our knowledge there is no earlier report on “magnetic bucky gel based DSPE” for microextraction of mercury from food and cosmetics samples. The parameters influencing the extraction efficiency of the mercury, including pH, extraction time, sorbent amount, MGO/IL ratio, type and volume of eluent, and desorption time, were optimized.
Experimental
Materials and standard solutions
The ionic liquid, ([BMim] PF6) 284.18 g mol−1, 97% was obtained (Sigma-Aldrich, USA). Stock solutions of 1000 mg L−1 mercury were prepared by dissolving an appropriate amount of HgCl2 (Merck, Germany) in ultra-pure water and stored in polyethylene bottles at 4°C. The standard solutions were diluted with ultrapure water to prepare working solutions. Nitric acid (HNO3, 65%), sulfuric acid (H2SO4, 97%), perchloric acid (HCLO4, 70%), acetic acid (HAc, 99.5%), sodium acetate (NaOAc), acetone, acetonitrile (ACN), methanol (MeOH), hydrochloric acid (HCl), sodium hydroxide (NaOH), ammonium hydroxide (NH4OH), sodium borohydrate (NaBH4), tin(II) chloride (SnCl2), DTZ (chelating agent), and hydrogen peroxide were purchased (Merck, Germany). MGO was synthesized in our lab.[Citation40] A 1000 mg L−1 solution of DTZ was prepared by dissolving proper amounts of the reagent in MeOH and was kept and protected from light. Solutions of 0.6% (w/v) NaBH4 in 0.6% (w/v) NaOH and 3.0 mol L−1 HCl were used as the reducing agent and the mercury vapor producer, respectively. A buffer solution of HAc (1 mol L−1) was used to adjust the pH of solutions.
Instrumentation
A GBC 932 plus atomic absorption spectrometer (AAS), (GBC Scientific Equipment, Australia), equipped with a deuterium background corrector, was used for the determination of mercury in samples. A mercury hollow cathode lamp operated at 10.0 mA with the wavelength of 253.7 nm and the spectral band pass of 0.5 nm was used as the radiation source. A T-cell quartz tube (140-mm length and 25-mm i.d.) was placed directly on the burner, which was equipped with a T-cell tube holder. A GBC HG3000 vapor generation system with argon (99.996%) as the carrier gas at a pressure of 1.38 bar was coupled to the AAS system to produce the cold vapor of Hg assisted by NaBH4 (0.6%) in NaOH solution (0.6%) and HCl 3.0 mol L−1. The peak height absorbance of the transient signal was chosen for quantification. A Philips CM120 transmission electron microscope, TEM, (Philips, Netherlands), a Perkin–Elmer Fourier transform infrared (FTIR) spectroscope with KBr disks (USA), a vibrating sample magnetometer (VSM) (AGFM/VSM 3886, Iran), a digital Metrohm pH meter model 692 (Herisau, Switzerland) equipped with a combined glass calomel electrode, and also a IKA vortex shaker, 230V (Sigma-Aldrich, Germany) were used during the experimental process.
Preparation of soft gel
The bucky gel was prepared by mixing IL and MGO in an agate mortar. 400 µL of IL and 15 mg of MGO were ground smoothly for 30 min () until the material got viscous, which means that the IL interacted with the surface of the carbon nanoparticles by π-π interactions.[Citation41] The obtained black bucky gel was kept in a plastic sample tube for further use.
Magnetic gel dispersive extraction procedure
In this study, a magnetic bucky gel based DSPE was combined with CV-AAS to develop a new analytical method for the determination and speciation of ultra-trace amounts of mercury in standard solutions of river water, food, and cosmetics samples. First, 25 mL of solution containing, 50 µg L−1 of mercury standard solution, 25 µL (200 µg L−1) of DTZ (excess amount), and 5 mL of 1 mol L−1 NaOAc (pH = 4) was transferred to a conical tube. Next, 50 mg of the prepared bucky gel was dispersed in the sample solution and vortexed for 8 min in order to extract the complex by IL/MGO. Then, the bucky gel was collected at the bottom of the tube by applying an external magnetic field around the test tube and the upper aqueous phase was decanted carefully. Afterwards, 1 mL of high-purity HNO3 was added followed by 3 min vortexing to desorb the Hg-DTZ complex. Finally, the solid phase was removed from the eluted phase by means of a magnet, and the extracted solution was mixed with solutions of NaBH4 and HCl before guiding to the burner for quantitative analysis by CV-AAS.
Sample preparation procedure for real samples
Sample preparation procedure for milk
The sample preparation was based on a previous work.[Citation42] To precipitate the lipid phase, 6 mL of milk was centrifuged for 10 min at 6000 rpm, the upper phase containing lipids was removed, and 200 µL of a mixture of HCl (37%) and pure glacial HAc (1/1) was added to the aqueous phase to remove all the lipids present in the solution. Then, the solution was centrifuged at 6000 rpm for 10 min. The supernatant was collected and the pH was neutralized by using an appropriate amount of NaOH solution. The final solution was used for determination of mercury content by the proposed methods.
Sample preparation procedure for omega-3 supplements
Digestion of the samples was carried out according to a previous work.[Citation43] One omega-3 soft gel (w = 1.2 g) was put in a volumetric digestion flask and 1 mL ultra-pure water, 2 mL mixture of HNO3: HCLO4 (1:1) and 5 mL H2SO4 were added. The mixture was heated to 200°C for 30 min in an oven. The solution was allowed to cool, filtered, and kept in a propylene tube in 4°C.
Sample preparation procedure for lipstick
The digestion procedure was successfully performed similar to a previous study.[Citation44] First of all, 2 g of weighed dried sample of lipstick (invisible color) and 10 mL of concentrated HNO3 were added to a Teflon vessel and left at room temperature for 4 h. Next, the sample was heated to 85°C overnight. After digestion, the sample was cooled to room temperature, then 1 mL of 30% hydrogen peroxide was added and the sample solution was kept at 85°C for 1 h. The clear supernatant was neutralized by adding concentrated NH3 and transferred to a polypropylene tube for future use.
Sample preparation procedure for river water
The water sample was centrifuged, filtered through cellulose acetate membrane filters (pore size 0.45 µm) and diluted 1:100 with ultra-pure water.
Results and discussion
Characterization of the bucky gel
To investigate the structure and morphology of the produced bucky gel, TEM was used as shown in . The TEM image of the bucky gel shows a hazy MGO surface, which is a strong evidence of IL covering the MGO. A FT-IR spectrum was used to extract useful information to identify the presence of certain functional groups or chemical bonds in the prepared bucky gel (). The strong band at 557 cm−1 corresponding to the Fe–O stretching vibration confirms that MNPs were successfully anchored onto the GO. The stretching vibration of the C-H band at 2968.38, and 2879.78 cm−1, and the vibration of N-H in the imidazole ring at 1468 cm−1 indicate that IL was successfully coating the surface of MGO. The magnetic behavior of the sample was investigated by the M-H curve from VSM analysis at room temperature in a magnetic field strength of 1 T. Figure 1S shows the saturation magnetization for MGO/IL nanocomposite to be estimated to 10.0 emu g−1, which confirmed that the dispersed solution could be easily separated by applying an external magnetic field.
Optimization of parameters influencing the extraction and desorption process
Several factors that influenced the extraction recovery (ER %) of mercury were considered and optimized, including factors effective on extraction and elution. One variable at a time (OVAT) method was used to find the optimum condition of acidity, the amount of sorbent, extraction time and the IL/MGO ratio for extraction procedure, and type of eluent, desorption time, and the eluent volume were studied as main parameters influencing the desorption.
Effect of pH
pH is one of the most important factors for formation of metal-chelate complexes and subsequent extraction from various media. Since DTZ complexes are known to be stable in acidic medium, effects of pH on the complex formation and recovery values of mercury were studied in the range of 3–6 while other parameters were kept constant. , shows that the maximum recovery was obtained at pH = 4, adjusted by NaOAc buffer.
Effect of the amount of sorbent
The effect of the adsorbent amount was studied within the range of 20–60 mg of prepared bucky gel. shows that the highest extraction efficiency was obtained at 50 mg of sorbent. This means that lower amounts of the sorbent cannot provide sufficient extraction recovery of the target complex, and higher levels do not give any significant change. According to the results, 50 mg of bucky gel was selected as the best amount of sorbent.
Effect of the extraction time
Since one of the crucial factors affecting the extraction efficiency is the mass transfer, which is related to the equilibrated time, the extraction was investigated for times between 5 and 10 min at the concentration level 50 µg L−1 using vortex at constant rate. According to the results shown in , extraction recovery increased when the extraction time was up to 8 min and longer times did not have any significant effect on the ER%.
Effect of IL/MGO ratio
To reach the best synergetic effect of IL and MGO, different ratios ranging from 200 to 600 µL of IL were tested while the amount of MGO was constant at 15 mg. It has been proposed that this synergetic effect is the result of the special orientation (cation–π) of the carbon-based nanoparticle with IL in the soft material (bucky gel), which allows the lateral interaction of GO through the π-system.[Citation41] The results in show that the ratio of 26 (400 μL of IL to 15 mg MGO) was the optimum ratio for preparing the bucky gel. Based on previous work,[Citation41] the gel has the ability to trap a limited amount of the IL, which means that when the excess amount of IL was grounded into the MGO, the gel and IL phases separate from each other easily during the extraction process.
Optimization of desorption steps
To obtain the best conditions for desorption, extraction factors were kept at their optimum value, and type and volume of the eluent, and desorption time were tested. One of the most important factors involved in the elution procedure is the choice of suitable solvent to release the analyte from the sorbent. Four eluents including concentrated and diluted HNO3, MeOH, and ACN were examined. Higher signals were achieved by using conc. HNO3 in comparison with the other solvents. This could be because of the breaking of the ligand-Hg binding, and release of Hg ions from the sorbent.[Citation45] Thus, conc.HNO3 was used as the suitable desorption eluent for subsequent experiments (). Although decreasing the total preconcentration factor may be the result of increasing the eluent volume, sufficient amounts of desorption solvent are required for efficient elution of the adsorbed analyte from the surface of the sorbent. By examination of the eluent volume in the range of 0.5–1.5 mL, 1 mL of concentrated HNO3 was chosen as the sufficient volume of eluent to remove the analyte from the sorbent. The effect of desorption time was investigated in the range of 3–9 min. As illustrated in , min vortexing for the desorption step gave higher intensity than 5 min of desorption, while extending this time did not have any significant effect on desorption recovery.
Effect of interfering ions on extraction recovery
The effect of co-existing ions was studied for the extraction of mercury according to the proposed procedure. For this reason, different concentrations of interfering ions were added to 25 mL of 50 µg L−1 of mercury and extraction was performed with the optimized method. The effect of a species was considered significant when its presence caused a change greater than 10% in the analytical response. According to the results summarized in Table S1, relative recovery was about 94–100%, which showed that interference from high levels of contaminant ions in the matrix could be ignored.
Effect of chemical vapor generation condition
In order to find the best hydride generation conditions, two different reducing agents, including NaBH4 and SnCl2 in acidic media (HCl), were tested. NaBH4 is the more common reducing agent, which provides more effective and faster reduction compared to SnCl2.[Citation46] The range 0.5–1% of NaBH4 in 0.6% NaOH was tested, and the better analytical signal was obtained at 0.6% of NaBH4, so this was selected as the optimum reducing agent.
Method validation
In order to assess the figures of merit of the proposed method, optimized experimental conditions were utilized. A linear dynamic range from 1 to 200 µg L−1 of the calibration curve with an appropriate correlation coefficient (R2) of 0.9951 was obtained. The LOD was found to be 0.57 µg L−1, based on Equation (1):
Where Sb is the standard deviation of the blank signal extractions and me is the slope of the calibration curve of the extracted analyte. The LOQ was determined as 1.88 µg L−1 at 10 times the above-mentioned ratio. The experimental preconcentration factor (PF) was calculated by dividing the slope of the extraction calibration curve (me) to the slope of the standard calibration (ms) curve to be 21 (Eqution (2)). The ER was 84% achieved using Eqution (3). The inter-day relative standard deviation (RSD) 6.5% was obtained for three replicated measurements at the concentration of 50 µg L−1 of the analyte. The comparison between the analytical performance of the proposed method with other alternative techniques for extraction and determination of mercury is shown in . As might be seen, the method has generally lower LOD and RSD% with good linearity in comparison to the other reported techniques, and can be used for different kinds of samples with complex matrices. In the case of reference[Citation47] where they reported lower detection limit, it was due to the use of a more sensitive instrument and detector (ICP-MS).
Table 1. Comparison of the presented method with some other reported methods for determination of mercury ions in different samples.
Adsorption capacity
The important factor that determines the amount of sorbent needed for quantitative extraction of a specific target analyte is the adsorbent capacity, this is determined by adding a specific amount of sorbent to different concentrations of the analyte.[Citation48] In the present study, to determine the adsorption capacity of the bucky gel, 25 mL of the sample solution containing the different concentrations of mercury and 50 mg of sorbent were used. The adsorption capacity was calculated by Eqution (4):
Where Qe is the adsorption capacity (mg g−1), Ci and Ce are the initial and equilibrium concentrations of the metal ions (mg L−1), respectively. V (L) is the volume of the aqueous solution and m (g) is the weight of the sorbent. To investigate the partitioning of the analyte between the sample solution and the sorbent at the equilibrium concentration, the Langmuir isotherm model was used. (Equation (5))
Where Kl is the Langmuir isotherm constant (L mg−1) and Qm is the maximum adsorption capacity. The plot of Ce/Qe versus Ce yielded a straight line exhibiting that the adsorption of the target analyte obeyed the Langmuir isotherm.[Citation30] Based on the Equations (4) and (5), maximum Qm was obtained as 286 mg g−1.
Analysis of real samples
To compare the applicability and accuracy of the proposed method for different matrices, river water (collected from Kan River, Tehran, Iran), cow milk, omega-3 capsules, and lipstick (all purchased from local markets) were analyzed representing real samples. All samples were digested and prepared by the procedures in section 2.5, diluted to 25 mL with ultrapure water (except the water sample), and the proposed procedure in Section 2.4 was used for determination of the mercury content (Creal). Then, all real samples were spiked with appropriate amounts of standard mercury solution (Cadded) and the extraction procedure described in Section 2.4 was applied under the optimum conditions to find the concentrations of the analyte after addition of known amount of standard in the real sample (Ctotal). Relative recovery (RR %) was calculated using Equation (6):
Comparison of the extraction ability of the proposed method for different matrixes
It should be noted that analysis in complex matrix composition is the main cause of problems in the determination of organic and inorganic contaminants. Lipstick and omega-3 with high content of fatty acids brought different challenges for the digestion and cleanup steps. The matrix effect can influence the reproducibility in the extraction and analysis method and may cause suppression of signals. As shown in , milk as a source of protein represented a simpler matrix considering the preparation step, and thus gave more acceptable accuracies, as did the river water sample for determination of mercury based on the proposed method.
Table 2. Determination of mercury in different samples using the proposed method.
Conclusion
The magnetic bucky gel was synthesized and used as an adsorbent for the DSPE of mercury in real samples prior to CV-AAS analysis. The proposed microscale dispersive method with nano sorbent, which requires low volumes of solvents with high capacity, makes it useful for trace microextraction of the target analyte in low-fat samples. The results showed that the synthesized bucky gel provides high sensitivity, high extraction efficiency, fast and simple extraction of mercury with good compatibility for water based samples like river water and milk. Shorter extraction time, good sorbent dispersibility, high surface area, convenient magnetic separation by an external magnetic field and high adsorption capacity would be some advantages of the proposed bucky gel in comparison with other reported sorbents. Higher RSD for high fatty acid matrices (lipstick and omega-3), confirmed that an alternative matrix elimination method should be applied for sample cleanup in order to improve precision and offer a matrices effect-free analytical methods.
Nevertheless, bucky gels can introduce as a new generation of sorbents that has the ability to extract trace amounts of organic and inorganic compounds from aqueous matrices.
Conflict of interest
The authors declare that they have no conflict of interest.
Acknowledgments
The authors gratefully acknowledge the kind support of Kharazmi University (Tehran, Iran).
Supplementary material
Supplemental data for this article can be accessed here.
References
- Gautam, R.K.; Sharma, S.K.; Mahiya, S.; Chattopadhyaya, M.C. (2015) CHAPTER 1 contamination of heavy metals in aquatic media: transport, toxicity and technologies for remediation Heavy Metals In Water: Presence, Removal and Safety;The Royal Society of Chemistry
- Hamann, C.R.; Boonchai, W.; Wen, L.; Sakanashi, E.N.; Chu, C.-Y.; Hamann, K.; Hamann, C.P.; Sinniah, K.; Hamann, D. (2014) Spectrometric analysis of mercury content in 549 skin-lightening products: is mercury toxicity a hidden global health hazard? Journal of the American Academy of Dermatology, 70 (2): 281–287. doi:10.1016/j.jaad.2013.09.050.
- Fernández, E.; Vidal, L.; Costa-García, A.; Canals, A. (2016) Mercury determination in urine samples by gold nanostructured screen-printed carbon electrodes after vortex-assisted ionic liquid dispersive liquid–liquid microextraction. Analytica Chimica Acta, 915: 49–55. doi:10.1016/j.aca.2016.02.028.
- Tunsu, C.; Ekberg, C.; Retegan, T. (2014) Characterization and leaching of real fluorescent lamp waste for the recovery of rare earth metals and mercury. Hydrometallurgy, 144: 91–98. doi:10.1016/j.hydromet.2014.01.019.
- Temple, D.M.; McNeese, M.C. (1983) Hazards of battery ingestion. Pediatrics, 71 (1): 100–103.
- Dico, G.M.L.; Galvano, F.; Dugo, G.; D’Ascenzi, C.; Macaluso, A.; Vella, A.; Giangrosso, G.; Cammilleri, G.; Ferrantelli, V. (2018) Toxic metal levels in cocoa powder and chocolate by ICP-MS method after microwave-assisted digestion. Food Chemistry, 245: 1163–1168. doi:10.1016/j.foodchem.2017.11.052.
- Lummas, S.; Ruiz-Jiménez, J.; Luque de Castro, M.D.; Colston, B.; González-Rodríguez, J.; Chen, B.; Corns, W. (2011) Determination of arsenic, mercury and barium in herbarium mount paper using dynamic ultrasound-assisted extraction prior to atomic fluorescence and absorption spectrometry. Analytical Letters, 44 (10): 1842–1852. doi:10.1080/00032719.2010.526265.
- Konshina, D.N.; Open’ko, V.V.; Temerdashev, Z.A.; Gurinov, A.A.; Konshin, V.V. (2016) Synthesis of novel silica-gel-supported thiosemicarbazide and its properties for solid phase extraction of mercury. Separation Science and Technology, 51 (7): 1103–1111. doi:10.1080/01496395.2016.1143005.
- Swami, K.; Judd, C.D.; Orsini, J. (2009) Trace metals analysis of legal and counterfeit cigarette tobacco samples using inductively coupled plasma mass spectrometry and cold vapor atomic absorption spectrometry. Spectroscopy Letters, 42 (8): 479–490. doi:10.1080/00387010903267799.
- Türker, A.R.; Çabuk, D.; Yalçınkaya, Ö. (2013) Preconcentration, speciation, and determination of mercury by solid phase extraction with cold vapor atomic absorption spectrometry. Analytical Letters, 46 (7): 1155–1170. doi:10.1080/00032719.2012.753608.
- Ahmet Sari, M.;. (2009) Removal of mercury(II) from aqueous solution using moss (Drepanocladus revolvens) biomass: equilibrium, thermodynamic and kinetic studies. Journal of Hazardous Materials, 169: 500–507. doi:10.1016/j.jhazmat.2009.06.023.
- MustafaTuzen, A.; Mendil, D.; Soylak, M. (2009) Biosorptive removal of mercury(II) from aqueous solution using lichen (Xanthoparmelia conspersa) biomass: kinetic and equilibrium studies. Journal of Hazardous Materials, 169: 263–270. doi:10.1016/j.jhazmat.2009.03.096.
- MustafaTuzen, I.A.; Demirhan, C.; Soylak, M. (2009) Mercury(II) and methyl mercury determinations in water and fish samples by using solid phase extraction and cold vapour atomic absorption spectrometry combination. Food and Chemical Toxicology, 47: 1648–1652. doi:10.1016/j.fct.2009.04.024.
- MustafaTuzen, O.D.; UluozluaIs, K.; Soylak, M. (2009) Mercury(II) and methyl mercury speciation on Streptococcus pyogenes loaded Dowex Optipore SD-2. Journal of Hazardous Materials, 169: 345–350. doi:10.1016/j.jhazmat.2009.03.100.
- Hight, S.C.; Cheng, J. (2006) Determination of methylmercury and estimation of total mercury in seafood using high performance liquid chromatography (HPLC) and inductively coupled plasma-mass spectrometry (ICP-MS). Method Development And, 567 (2): 160–172.
- Capelo, J.L.; Maduro, C.; Mota, A.M. (2004) Advanced oxidation processes for degradation of organomercurials: determination of inorganic and total mercury in urine by FI-CV-AAS. Journal of Analytical Atomic Spectrometry, 19 (3): 414–416. doi:10.1039/b314905f.
- Pistón, M.; Silva, J.; Pérez-Zambra, R.; Dol, I.; Knochen, M. (2012) Automated method for the determination of total arsenic and selenium in natural and drinking water by HG-AAS. Environmental Geochemistry and Health, 34 (2): 273–278. doi:10.1007/s10653-011-9436-9.
- Torres, D.P.; Borges, D.L.G.; Frescura, V.L.A.; Curtius, A.J. (2009) A simple and fast approach for the determination of inorganic and total mercury in aqueous slurries of biological samples using cold vapor atomic absorption spectrometry and in situoxidation. Journal of Analytical Atomic Spectrometry, 24 (8): 1118–1122. doi:10.1039/b816622f.
- Shah, A.Q.; Kazi, T.G.; Baig, J.A.; Afridi, H.I.; Arain, M.B. (2012) Simultaneously determination of methyl and inorganic mercury in fish species by cold vapour generation atomic absorption spectrometry. Food Chemistry, 134 (4): 2345–2349. doi:10.1016/j.foodchem.2012.03.109.
- Pérez-García, F.; Galán-Vidal, C.A.; Alvarado-Rodríguez, J.G.; Páez-Hernández, M.E.; Andrade-López, N.; Ramírez Silva, M.T. (2013) Selective liquid-liquid extraction of mercury(II) from aqueous solution by N-Alkyldithiophosphate Compounds CH3(CH2)nS2P(OC6H4)2 (n = 0 to 4). Separation Science and Technology, 48 (5): 736–740. doi:10.1080/01496395.2012.716480.
- Dahaghin, Z.; Mousavi, H.Z.; Sajjadi, S.M. (2017) A novel magnetic ion imprinted polymer as a selective magnetic solid phase for separation of trace lead(II) ions from agricultural products, and optimization using a Box–behnken design. Food Chemistry, 237: 275–281. doi:10.1016/j.foodchem.2017.05.118.
- Shokoufi, N.; Abbasgholi Nejad Asbaghi, B. (2015) Rotative liquid-liquid microextraction as a preconcentration method in combination with fiber optic-linear array detection spectrophotometry for the determination of cobalt in pharmaceutical samples. Separation Science and Technology, 50 (15): 2327–2334.
- Pena-Pereira, F.; Lavilla, I.; Bendicho, C.; Vidal, L.; Canals, A. (2009) Speciation of mercury by ionic liquid-based single-drop microextraction combined with high-performance liquid chromatography-photodiode array detection. Talanta, 78 (2): 537–541. doi:10.1016/j.talanta.2008.12.003.
- Baghdadi, M.; Shemirani, F. (2008) Cold-induced aggregation microextraction: A novel sample preparation technique based on ionic liquids. Analytica Chimica Acta, 613 (1): 56–63. doi:10.1016/j.aca.2008.02.057.
- Fashi, A.; Yaftian, M.R.; Zamani, A. (2017) Electromembrane extraction-preconcentration followed by microvolume UV–vis spectrophotometric determination of mercury in water and fish samples. Food Chemistry, 221: 714–720. doi:10.1016/j.foodchem.2016.11.115.
- Zhang, B.-T.; Zheng, X.; Li, H.-F.; Lin, J.M. (2013) Application of carbon-based nanomaterials in sample preparation: A review. Analytica Chimica Acta, 784: 1–17. doi:10.1016/j.aca.2013.03.054.
- Saygili Canlidinç, R.; Kalfa, O.M.; Üstündağ, Z.; Erdoğan, Y. (2017) Graphene oxide modified expanded perlite as a new sorbent for Cu(II) and Pb(II) prior to determination by high-resolution continuum source flame atomic absorption spectrometry. Separation Science and Technology, 52 (13): 2069–2078. doi:10.1080/01496395.2017.1328443.
- Mehdinia, A.; Ramezani, M.; Jabbari, A. (2017) Preconcentration and determination of lead ions in fish and mollusk tissues by nanocomposite of Fe3O4@graphene oxide@polyimide as a solid phase extraction sorbent. Food Chemistry, 237: 1112–1117. doi:10.1016/j.foodchem.2017.06.051.
- Razmi, H.; Musevi, S.J.; Mohammad-Rezaei, R. (2016) Solid phase extraction of mercury(II) using soluble eggshell membrane protein doped with reduced graphene oxide, and its quantitation by anodic stripping voltammetry. Microchimica Acta, 183 (2): 555–562. doi:10.1007/s00604-015-1665-7.
- Wang, P.; Zhou, X.; Zhang, Y.; Wang, L.; Zhi, K.; Jiang, Y. (2016) Synthesis and application of magnetic reduced graphene oxide composites for the removal of bisphenol A in aqueous solution—a mechanistic study. RSC Advances, 6 (104): 102348–102358. doi:10.1039/C6RA23542E.
- Liu, Q.; Shi, J.; Sun, J.; Wang, T.; Zeng, L.; Jiang, G. (2011) Graphene and graphene oxide sheets supported on silica as versatile and high-performance adsorbents for solid-phase extraction. Angew Chem Int Ed Engl, 50 (26): 5913–5917. doi:10.1002/anie.201007138.
- Chen, L.; Wang, T.; Tong, J. (2011) Application of derivatized magnetic materials to the separation and the preconcentration of pollutants in water samples. TrAC Trends in Analytical Chemistry, 30 (7): 1095–1108. doi:10.1016/j.trac.2011.02.013.
- Geng, Y.; Ding, M.; Chen, H.; Li, H.-F.; Lin, J.-M. (2012) Preparation of hydrophilic carbon-functionalized magnetic microspheres coated with chitosan and application in solid-phase extraction of bisphenol A in aqueous samples. Talanta, 89: 189–194. doi:10.1016/j.talanta.2011.12.015.
- Bağda, E.; Tüzen, M. (2017) A simple and sensitive vortex-assisted ionic liquid-dispersive microextraction and spectrophotometric determination of selenium in food samples. Food Chemistry, 232: 98–104. doi:10.1016/j.foodchem.2017.03.104.
- Cacho, J.I.; Campillo, N.; Viñas, P.; Hernández-Córdoba, M. (2016) In situ ionic liquid dispersive liquid–liquid microextraction and direct microvial insert thermal desorption for gas chromatographic determination of bisphenol compounds. Analytical and Bioanalytical Chemistry, 408 (1): 243–249. doi:10.1007/s00216-015-9098-z.
- Hua, Z.; Shuqian, X.; Peisheng, M. (2005) Use of ionic liquids as ‘green’ solvents for extractions. Journal of Chemical Technology & Biotechnology, 80 (10): 1089–1096. doi:10.1002/jctb.1333.
- Takanori, F.; Atsuko, K.; Yohei, Y.; Takuji, A.; Shunsuke, N.; Toshikazu, T.; Tamotsu, I.; Takuzo, A. (2006) Dramatic effect of dispersed carbon nanotubes on the mechanical and electroconductive properties of polymers derived from ionic liquids. Small, 2 (4): 554–560. doi:10.1002/smll.200500404.
- Takanori, F.; Takuzo, A. (2007) Ionic liquids for soft functional materials with carbon nanotubes. Chemistry – A European Journal, 13 (18): 5048–5058. doi:10.1002/chem.200700554.
- Lee, J.; Aida, T. (2011) “Bucky gels” for tailoring electroactive materials and devices: the composites of carbon materials with ionic liquids. Chemical Communications, 47 (24): 6757–6762. doi:10.1039/c1cc00043h.
- Rofouei, M.K.; Jamshidi, S.; Seidi, S.; Saleh, A. (2017) A bucky gel consisting of Fe3O4 nanoparticles, graphene oxide and ionic liquid as an efficient sorbent for extraction of heavy metal ions from water prior to their determination by ICP-OES. Microchimica acta, 184 (9): 3425–3432.
- Polo-Luque, M.L.; Simonet, B.M.; Valcárcel, M. (2013) Ionic liquid combined with carbon nanotubes: A soft material for the preconcentration of {PAHs}. Talanta, 104: 169–172. doi:10.1016/j.talanta.2012.10.025.
- Ping, J.; Wu, J.; Ying, Y. (2012) Determination of trace heavy metals in milk using an ionic liquid and bismuth oxide nanoparticles modified carbon paste electrode. Chinese Science Bulletin, 57 (15): 1781–1787. doi:10.1007/s11434-012-5115-1.
- Acheampong, A.; Darko, G. (2014) Assessment of mercury levels in Omega-3 Food supplements available on the Ghanaian market. Asian Journal of Applied, 2 (5): 658–662.
- Karimi, M.A.; Hatefi-Mehrjardi, A.; Mohammadi, S.Z.; Mohadesi, A.; Mazloum-Ardakani, M.; Nezhad, M.R.H.; Kabir, A.A. (2012) Solid phase extraction of trace amounts of Pb(II) in opium, heroin, lipstick, plants and water samples using modified magnetite nanoparticles prior to its atomic absorption determination. Journal of the Iranian Chemical Society, 9 (2): 171–180. doi:10.1007/s13738-011-0035-6.
- Rofouei, M.K.; Hosseini, S.M.; Khani, H.; Rahimi-Alangi, S. (2012) Highly selective determination of trace quantities of Hg(ii) in water samples by spectrophotometric and inductively coupled plasma-optical emission spectrometry methods after cloud point extraction. Analytical Methods, 4 (3): 759–765. doi:10.1039/c2ay05810c.
- Pohl, P.; Jimenéz Zapata, I.; Voges, E.; Bings, N.H.; Broekaert, J.A.C. (2008) Comparison of the cold vapor generation using NaBH4 and SnCl2 as reducing agents and atomic emission spectrometry for the determination of Hg with a microstrip microwave induced argon plasma exiting from the wafer. Microchimica Acta, 161 (1): 175–184. doi:10.1007/s00604-007-0887-8.
- Jia, X.; Han, Y.; Wei, C.; Duan, T.; Chen, H. (2011) Speciation of mercury in liquid cosmetic samples by ionic liquid based dispersive liquid-liquid microextraction combined with high-performance liquid chromatography-inductively coupled plasma mass spectrometry. Journal of Analytical Atomic Spectrometry, 26 (7): 1380–1386. doi:10.1039/c0ja00121j.
- Jamali, M.R.; Assadi, Y.; Shemirani, F.; Salavati-Niasari, M. (2007) Application of thiophene-2-carbaldehyde-modified mesoporous silica as a new sorbent for separation and preconcentration of palladium prior to inductively coupled plasma atomic emission spectrometric determination. Talanta, 71 (4): 1524–1529. doi:10.1016/j.talanta.2006.07.034.