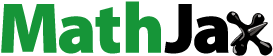
ABSTRACT
The present study modifies the sorption isotherm for simulating the influences of initial pH and temperature variations on the cadmium sorption from contaminated water using waste foundry sand based on Langmuir, Freundlich, and Temkin models. Results proved that the Langmuir expression is able to adopt these effects by relating sorption capacity and affinity constants with pH and temperature of aqueous solution through exponential relationships (determination coefficient = 0.9375). The present model is assumed that the sorption process occurs through acidic functional groups and this is consistent with FTIR outputs. Interaction of cadmium/WFS is found to be exothermic by thermodynamic analysis.
Introduction
Metals represent the common dangerous inorganic contaminants that resulted from many anthropogenic sources and they can cause a serious threat for the components of ecosystem such as human, plants, organisms as well as the water resources.[Citation1,Citation2] Groundwater is considered one of the most important resources that can be contaminated with metal ions spilled from municipal landfills, dumping sites, airports, petrol stations, agriculture, and chemical plants. These metals can migrate with the flowing water through the unsaturated-saturated zones of the soil and this will cause a considerable contamination for large volumes of soil and groundwater; however, these pollutants with time can pollute the surface water resources such as rivers, reservoirs, etc. Permeable reactive barrier (PRB) is an innovative technology that applied within the polluted site to retain the contaminants by the reactive material of the barrier for restricting the migration of dissolved plume.[Citation3,Citation4] The transport of contaminant through the PRB is governed by three major mechanisms, namely advection, dispersion, and sorption within the solid phase. The sorption process plays a significant role in the specifying the extend of the plume front because it will specify the value of retardation factor where this factor can be represented by different sorption isotherms such as Langmuir and Freundlich models. The isotherm models are used to simulate the experimental results for sorption of contaminants measured at certain values of pH and temperature.
Many studies[Citation5–Citation7] have been achieved to fit the isotherm models to experimental datasets measured at different conditions. In this direction, Langmuir isotherm was used to represent a set of laboratory measurements collected at various values of pH and, consequently, the relationship between the adsorption capacity as a function of pH was recognized.[Citation8,Citation9] Adsorption of arsenic from aqueous solutions onto iron oxide can be represented by a modified Langmuir expression.[Citation10] Also, the pH-dependent bio-sorption using marine macro-algae for removal of heavy metals with aid of the functional groups can be formulated by modified Langmuir model.[Citation11] To represent the effect of pH on the uptake of copper onto the calcium alginate and Sphaerotilus natans sorbents, a set of semi-empirical and empirical relationships have been developed. The Langmuir isotherm is considered the origin of these models and they can be used effectively for characterizing the experimental measurements by monitoring of the pH.[Citation12] Further, long-term column tests for reduction of Cr(VI) from contaminated water onto scrap iron were conducted when the pH varied from 2.00 to 7.30. The results signified that the reduction capacity of the iron was depended on the value of initial pH and the maximum capacity is equal to 19.2 mg Cr(VI)/g iron at pH of 2.50; however, this capacity may be decreased due to increase of pH value.[Citation13] Freundlich and Langmuir models were collected in one form and this formula was utilized for simulating the influence of pH on the sorption data. The values of affinity constants were correlated linearly with pH of aqueous solutions. The adsorption measurements resulted from interaction of goethite-coated sand and pure goethite with the arsenic were used for validation of developed formula.[Citation14]
In addition, the Langmuir model was developed to simulate the effect of pH on the interaction between the olive pips and copper-contaminated solution. Results proved that the maximum adsorption capacity is related with initial pH of contaminated water by linear regression, while the relationship between the affinity coefficient and initial pH can be represented by power equation. Further, the combination of this isotherm with solute transport equation was carried out for finding the distribution of contaminant through the column tests under the effect of initial pH.[Citation1] Finally, the Freundlich model was developed, for predicting the adsorption capacity of quinolone by powdered activated carbon at different pH values. This model had excellent prediction capabilities under different laboratory scenarios.[Citation15] Accordingly, an isotherm model for simulating the effects of temperature and initial pH on the uptake of contaminant is required. This s model can be incorporated into the advection-dispersion equation as a sink term for finding the propagation of contaminant plume under these effects.
Hence, the present work is aimed to modify an isotherm model for simulating the effect of temperature and pH of the aqueous solution on the adsorption process followed by further verification using experimental data of cadmium onto waste foundry sand (WFS). The developed model opens up a new methodology for finding the effect of pH and temperature on the retardation factor of the reactive medium and, consequently, the longevity of the PRB by using the available codes of contaminant transport such as COMSOL software.
Material and methods
Preparation of sorbent and contaminant
The WFS was used as sorbent in the batch experiments. This waste was collected from the Nasr Company for Mechanical Industries/Baghdad/Iraq. The bulk density, porosity, hydraulic conductivity, surface area and cation exchange capacity were equal to 1.44 g/cm3, 0.46, 1 × 10−6 cm/s, 5.935 m2/g, 10.94 mEq/100 g, respectively. The particle size of WFS was ranged from 0.075 to 1 mm where the effective and median sizes of 180 and 320 µm, respectively, with coefficient of uniformity equal to 1.94. The chemical composition of WFS is consisted of SiO2, AL2O3, Fe2O3, MgO, and CaO and with proportions of 94.36%, 2.82%, 2.12%, 0.23%, and 0.05%, respectively
Cadmium was chosen for representing the heavy metal contaminant. Aqueous solution contaminated with cadmium in the concentration of 1000 mg/L was prepared by dissolving of Cd(NO3)2 · 4H2O (manufactured by SD Fine-Chem Limited, India) in distilled water; however, this stock solution can be diluted to required concentration.
Sorption tests
Experimental tests were carried out under different values of initial pH (3–6), temperature (30°C, 40°C, and 50°C), and initial cadmium concentration of (10, 50, 100, and 200 mg/L). A series of flasks were taken, each flask filled with 50 mL of cadmium solution, followed by the addition of the 2.5-g dosage of WFS. Subsequently, continuous agitation of the solution in each one was carried out, up to total contact time of 3 h using orbital shaker (Edmund Buhler SM25, German) with an agitation speed of 200 rpm. The previous studies, such as[Citation16], proved that the contact time required to achieve the equilibrium status due to interaction of heavy metal with WFS is not exceeded 1 h and, accordingly, 3 h was chosen in the present study to ensure the reach for the equilibrium. The samples at the end of each tests were filtered and the concentrations of metal ions measured by the atomic absorption spectrophotometer (AAS) (AA-7000 Shimadzu, Japan). The mass of cadmium sorbed on the WFS, qe, calculates by:
where m is the mass of WFS (g) added to the certain volume (V, L) of aqueous solution. Sorption models such as Freundlich, Langmuir and Temkin were used for representing the measured data by linear and non-linear regression methods using Excel 2016.
Finally, sorption mechanisms can be investigated through the Fourier transform infrared (FTIR) analysis using Shimadzu FTIR, 8000 series spectrophotometers by identification of the effective functional groups that play a potential role in the removal of Cd(II) from aqueous solution due to the binding process.
Sorption isotherm models
A brief review of two parameter isotherm models used in the present study can be presented below[Citation17]:
Freundlich model (1906) is the earliest known sorption isotherm expression and it can be written as follows:
where aF is a constant related to the maximum adsorption capacity of the adsorbent and
is the sorption intensity of.[Citation18] This empirical isotherm is applicable for multilayer sorption and heterogeneous surfaces.[Citation19]
Langmuir model (1916) is used for description of uniform energies of sorption onto the sorbent surface and EquationEq. (3)(3)
(3) represents the formula of Langmuir:
where b is the affinity of the contaminant to the sorbent and is the maximum sorption capacity (mg/g).
Temkin model: This model assumes that the adsorption is characterized by a uniform distribution of the binding energies and its formula can be written as follows[Citation20]:
where is the equilibrium binding constant (L/mg) and B is the constant related to the heat of sorption (J/mol).
Results and discussion
Equilibrium study
It is clear from that the removal percentages increased with an increase in the pH values until they equalize up to 4.5. Subsequently, the removal percentages have decreased due to increase of the pH. For more accuracy, each value of the removal percentage plotted in is the average of the two measured values for two tests conducted under the similar operational conditions. This behavior may be resulted from the deprotonation and protonation of the basic and acidic groups of WFS which have a significant effect on the interaction between metal ions and sorbent as a function of the pH. The less removal in comparison to the best value can be attributed to inactivation of binding sites in the basic conditions.[Citation1] The pH of the aqueous solution is tended to rise from acidic state (=3) as the operation progresses but it becomes almost constant at higher pH. This trend is observed, as mentioned earlier, because the cations and protons compete for the surface sites up to a particular point, after which the aqueous medium tends toward a higher pH, due to the removal of protons from the aqueous medium. In contrast, with an initial alkaline pH (pH = 6), the trend progresses downward as the operation proceeds further until the pH becomes practically constant. The presence of Cd(OH)+ ions and Cd(OH)2 for an alkaline pH can be caused the removal of OH groups and resulting in the drop of pH values.
Figure 1. Removal efficiency of cadmium onto WFS as a function of initial cadmium concentration (WFS dosage = 2.5 g/50 mL; agitation speed = 250 rpm).
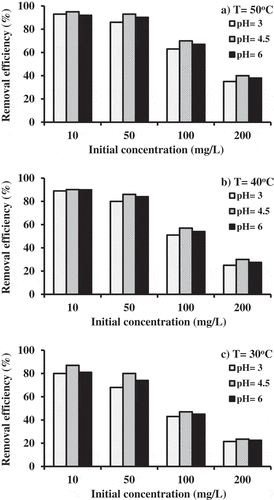
Freundlich, Langmuir and Temkin isotherm models were employed for representing the sorption measurements using linear and nonlinear fitting and the constants of the models are listed in –. Based on coefficient of determination (R2), it is clear that the Freundlich and Temkin models are not described the experimental data in a better way for different conditions under consideration, thereby confirming that fitting of heterogeneous mode of sorption with the Freundlich model, was not supported by present data. Additionally, signified that the Temkin isotherm-binding constant increased from 3.954 L/mg at pH of 3 to value of 6.338 L/mg at pH of 4.5 for the temperature of 50°C, which in turn indicates that the pH has a significant effect on the adsorption activity. However, the Langmuir model was found to exhibit the best fit among all the other two parameter models () suggesting a homogeneous monolayer mode of sorption owing to high R2 range from 0.972 to 0.999 with a minimum value of SSE. Statistical measures proved that the non-linear fitting for Langmuir model gives a closer approximation in comparison with linear regression.
Table 1. Constants of Freundlich isotherm model using linear and non-linear fitting for sorption of cadmium onto WFS.
Table 2. Constants of Langmuir isotherm model using linear and non-linear fitting for sorption of cadmium onto WFS.
Table 3. Constants of Temkin isotherm model using linear and non-linear fitting for sorption of cadmium onto WFS.
Modification of Langmuir model constants
Affinity constant: The activation energy of metal sorption onto the sorbent can be calculated by the relationships[Citation21,Citation22]:
where is the affinity of the sorbate at reference temperature which changes as function of pH (L/mg) and
is the heat of sorption (the difference between the activation energies necessary for desorption and sorption).
To understand the expression related between and pH, the fitted values of parameter (k.) based on nonlinear regression were plotted against pH as shown in . It is clear that the values of
, plotted as power relationship, increases with an increase in the pH values until they equalize up to 4.5. Subsequently, this parameter decreases with a similar type of fitted relationship due to increase of the pH. This behavior may be resulted from the protonation and deprotonation of the acidic and basic groups of WFS which have a significant effect on the interaction between metal ions and sorbent as a function of the pH.[Citation23,Citation24] Hence, the affinity constant (i.e. b) for surfaces of WFS was decreased due to decrease or increase of the pH from the value of 4.5 (which represents the best value for interaction of cadmium and WFS). This reduction in affinity of metal ions for the surface sites at low pH values mainly occurs due to competition between the cations and protons for the binding sites, however, at higher pH (>4.5), the less removal in comparison to the best value can be attributed to inactivation of binding sites in the basic condition. The pH of the aqueous solution is tended to rise from acidic state (= 3) as the operation progresses but it becomes almost constant at higher pH. This trend is observed, as mentioned earlier, because the cations and protons compete for the surface sites up to a particular point, after which the aqueous medium tends toward a higher pH, due to the removal of protons from the aqueous medium. In contrast, with an initial alkaline pH (pH = 6), the trend progresses downward as the operation proceeds further until the pH becomes practically constant. The general expression for describing the value of
can be written as:
EquationEq. (6)(6)
(6) can be substituted into EquationEq. (5)
(5)
(5) and the resultant will be:
Maximum sorption capacity: The effect of initial pH and temperature on the maximum sorption capacity (qmax) were studied depend on the effect of acid function group. Where there is one functional weakly acid group,[Citation11] the qmax can be represented in EquationEq. (8)(8)
(8) :
where and
are the maximum adsorption capacities for protonated (
) and deprotonated (
) forms of functional group, and the equilibrium is described using the following equations:
where is the apparent equilibrium constant, and it is expressed as the difference between entropy change (
), as a function of temperature, and enthalpy change (
) for the described reaction.[Citation25] The relationship between
and temperature was described using the van’t Hoff equation:
From EquationEqs. (11)(11)
(11) and (Equation13
(13)
(13) ), EquationEq. (14)
(14)
(14) can be obtained:
EquationEq. (8)(8)
(8) can be combined with EquationEq. (15)
(15)
(15) and the resultant will be:
From the EquationEqs. (8)(8)
(8) and (Equation16
(16)
(16) ), the modification of Langmuir model be:
where
The experimental data ( as a function of
) were obtained at different values of pH and temperature. These data were fitted by a non-linear regression method to evaluate the adjustable constants (
,
), by minimizing the sum of the squared deviations between experimental and predicted values. displayed the scatter diagram obtained by plotting values of qe predicted by the modified Langmuir model in comparison with experimental work. presents the fitted constant of the developed models using non-linear regression, reported together with the sum of squares error.
Table 4. Non-linear regression analysis for cadmium sorption onto waste foundry sand using modified Langmuir model developed in the present study.
Thermodynamic analysis
The enthalpy change (), the entropy change (
), and Gibbs free energy change (
) are the thermodynamic parameters that calculated for the sorption process from the variation of Langmuir constant (
) with temperature using well-known relations listed below:
where T is the absolute temperature (K), R is the universal gas constant (8.314 × 10−3 kJ mol−1 K−1), and (kc, L/g) is thermodynamic distribution coefficient for the adsorption calculated from the following equation[Citation26,Citation27]:
where was calculated for various temperatures at the equilibrium. Enthalpy change, due to the adsorption of onto WFS within the range of temperature, can be specified from the linear relation between ln
and 1/
using the least square analysis. The magnitudes of
,
, and
for cadmium adsorption onto WFS at different temperatures are listed in . The negative values of
and
means that the process is spontaneous and requires energy from the outside respectively. Hence, the removal of Cd(II) onto WFS is exothermic in nature. Additionally, the negative value of
suggested that the adsorption process involves an associative mechanism.[Citation28]
Table 5. Thermodynamic parameters for cadmium sorption onto WFS.
Fourier-transform infrared (FTIR) analysis
Infrared spectra of WFS sample before and after sorption of Cd(II) were measured within the range of 400–4000 cm−1 (). The band at 721 cm−1 can be assigned to disordered trydymite and the sand spectrum with a band around 1070 cm−1, which is broadened due to the overlap with the 1031 cm−1 band of the bentonite clays. The O-H stretch band is located at 3632 cm−1 in the spectra of bentonite clays.[Citation29] This is likely due to the fact that the green sands were only prepared with about 7% bentonite clay. The bands around 3450 cm−1 indicate the presence of water and the Si-O band around 518 cm−1 was presented in all of the Si-containing materials.
The shifts in the infrared frequencies of bonds (O-H band, H-O-H, O-Si-O, and Si-O band) were responsible for the binding of Cd(II) onto WFS, with the wavenumbers of the functional groups obtained at 3619, 1668, 662, and 522 cm−1, respectively.[Citation30] From FTIR wavenumber values, surface silanol groups (O-H band) are weak acids.[Citation31] The change in the hydroxide group due to the formation of cadmium hydroxide and the change in Si-O due to the adsorption of cadmium as following:
The sorption mechanism led to decrease final pH when compared with control as shown in . Hence, the results of this test are consistent with the assumptions of the developed models described in the earlier section.
Table 6. The initial and final values of pH through the interaction between aqueous solution contaminated with cadmium and WFS.
Conclusions
In comparison with Freundlich and Temkin models, Langmuir isotherm has a significant ability to consider the variations of initial pH and temperature of contaminated water on the sorption of cadmium onto WFS by relating the maximum adsorption capacity and affinity constants with initial pH and temperature through nonlinear (exponential) relationships. The modified model proved there is a good agreement between experimental and predicted values of sorbed cadmium per unit mass of WFS with coefficient of determination (R2) of 0.9375.
The modification of Langmuir model was taken into account the effect of weakly acidic functional groups and the affinity of metal ions regarding the reactive material.
FTIR test certified that the acidic functional groups are accountable for the sorption process and the association-dissociation of the functional groups represent the predominant mechanisms; however, this result is consistent with output the present model.
The nature of cadmium sorption onto WFS is exothermic based on the thermodynamic analysis.
Acknowledgements
We would like to gratefully acknowledge the technical support of Environmental Engineering Department/University of Baghdad during this work.
References
- Faisal, A. A. H. Effect of pH on the Performance of Olive Pips Reactive Barrier through the Migration of Copper-contaminated Groundwater. Desalin. Water Treat. 2016, 57, 4935–4943. DOI: 10.1080/19443994.2014.999132.
- Suddique, M. I. M.; Ahmad, G. M. S. I.; Shah, B. M. N. S.; Ahmad, M. M. S. Kinetic and Equilibrium Studies for Cadmium Biosorption from Contaminated Water Using Cassia Fistula Biomass. Int. J. Environ. Sci. Technol. 2018. DOI: 10.1007/s13762-018-1872-y.
- Mieles, J.; Zhan, H. Analytical Solutions of One-dimensional Multispecies Reactive Transport in a Permeable Reactive Barrier-aquifer System. J. Contam. Hydrol. 2012, 134–135, 54–68. DOI: 10.1016/j.jconhyd.2012.04.002.
- Bartzas, G.; Komnitsas, K. Solid Phase Studies and Geochemical Modelling of Low-cost Permeable Reactive Barriers. J. Hazard. Mater. 2010, 183, 301–308. DOI: 10.1016/j.jhazmat.2010.07.024.
- Faisal, A. A. H.; Abbas, T. R.; Jassam, S. H. Iron Permeable Reactive Barrier for Removal of Lead from Contaminated Groundwater. J. Eng. 2014, 20, 29–46.
- Faisal, A. A. H.; Jasim, H. K. Adsorption-precipitation Mechansims for Cadmium Removal from Polluted Water. Assoc. Arab Univ. J. Eng. Sci. 2018, 25, 110–125.
- Faisal, A. A. H.; Naji, L. N. Simulation of Ammonia Nitrogen Removal from Simulated Wastewater by Sorption onto Waste Foundry Sand Using Artificial Neural Network. Assoc. Arab Univ. J. Eng. Sci. 2019, 26, 28–34. DOI: 10.33261/jaaru.2019.26.1.004.
- Ghosh, M. M.;. Adsorption of Inorganic Arsenic and Organo-arsenical on Hydrous Oxides. In Met. Speciation, Sep. Recover. Proc. Int. Symp.; Patterson, J. W., Passino, R., Eds.; Lewis Publ. Inc.: Chelsea, MI, 1987; Vol. 6, pp 499–518.
- Wankasi, D.; Tarawou, T. Studies on the Effect pH on the Sorption of Pb (II) and Cu (II) Ions from Aqueous Media by Nipa Palm (nypa Fruticans Wurmb). J. Appl. Sci. Environ. Manag. 2008, 12, 87–94.
- Hsia, T. H.; Lo, S. L.; Lin, C. F. As(V) Adsorption on Amorphous Iron Oxide: Triple Layer Modelling. Chemosphere. 1992, 25, 1825–1837. DOI: 10.1016/0045-6535(92)90022-J.
- Yu, Q.; Kaewsarn, P. A. Model for pH Dependent Equilibrium of Heavy Metal Biosorption. Korean J. Chem. Eng. 1999, 16, 753–757. DOI: 10.1007/BF02698347.
- Vegliò, F.;. Modelling of Equilibrium Heavy Metal Biosorption Data at Different pH: a Possible Methodological Approach. Eur. J. Miner. Process. Environ. Prot. 2003, 3, 49–57.
- Gheju, M.; Iovi, A.; Balcu, I. Hexavalent Chromium Reduction with Scrap Iron in Continuous-flow System. Part 1: Effect of Feed Solution pH. J. Hazard. Mater. 2008, 153, 655–662. DOI: 10.1016/j.jhazmat.2007.09.009.
- Jeppu, G. P.; Clement, T. P. A Modified Langmuir-Freundlich Isotherm Model for Simulating pH-dependent Adsorption Effects. J. Contam. Hydrol. 2012, 129–130, 46–53. DOI: 10.1016/j.jconhyd.2011.12.001.
- Fu, H.; Li, X.; Wang, J.; Lin, P.; Chen, C.; Zhang, X.; Suffet, I. H. Activated Carbon Adsorption of Quinolone Antibiotics in Water: Performance, Mechanism, and Modeling. J. Environ. Sci. 2017, 56, 145–152. DOI: 10.1016/j.jes.2016.09.010.
- Faisal, A. A. H.; Ahmed, M. D. Removal of Copper Ions from Contaminated Groundwater Using Waste Foundry Sand as Permeable Reactive Barrier. Int. J. Environ. Sci. Technol. 2015, 12, 2613–2622. DOI: 10.1007/s13762-014-0670-4.
- Hamdaoui, O.; Naffrechoux, E. Modeling of Adsorption Isotherms of Phenol and Chlorophenols onto Granular Activated Carbon. Part I. Two-parameter Models and Equations Allowing Determination of Thermodynamic Parameters. J. Hazard. Mater. 2007, 147(1–2), 381–394. DOI: 10.1016/j.jhazmat.2007.01.021.
- Gheju, M.; Miulescu, A. Sorption Equilibrium of Hexavalent Chromium on Granular Activated Carbon. Chem. Bull. Politehnica Timisoara Univ. 2007, 52, 41–46.
- Ho, Y. S.; Porter, J. F.; McKay, G. Equilibrium Isotherm Studies for the Sorption of Divalent Metal Ions onto Peat: Copper, Nickel and Lead Single Component Systems. Water Air Soil Pollut. 2002, 141, 1–33. DOI: 10.1023/A:1021304828010.
- Vijayaraghavan, K.; Padmesh, T. V. N.; Palanivelu, K.; Velan, M. Biosorption of nickel(II) Ions onto Sargassum Wightii: Application of Two-parameter and Three-parameter Isotherm Models. J. Hazard. Mater. 2006, 133(1–3), 304–308. DOI: 10.1016/j.jhazmat.2005.10.016.
- Thomas, W. J.; Crittenden, B. Adsorption Technology and Design. ISBN: 978-0-7506-1959-2. DOI: 10.1016/B978-075061959-2/50002-1.
- Al-Ghouti, M.; Khraisheh, M. A. M.; Ahmad, M. N. M.; Allen, S. Thermodynamic Behaviour and the Effect of Temperature on the Removal of Dyes from Aqueous Solution Using Modified Diatomite: A Kinetic Study. J. Colloid Interface Sci. 2005, 287, 6–13. DOI: 10.1016/j.jcis.2005.02.002.
- Naushad, M.; AL-Othman, Z. A.; Islam, M. Adsorption of Cadmium Ion Using a New Composite Cation-exchanger Polyaniline Sn(IV) Silicate: Kinetics, Thermodynamic and Isotherm Studies. Int. J. Environ. Sci. Technol. 2013, 10, 567–578. DOI: 10.1007/s13762-013-0189-0.
- Madala, S.; Nadavala, S. K.; Vudagandla, S.; Boddu, V. M.; Abburi, K. Equilibrium, Kinetics and Thermodynamics of cadmium(II) Biosorption onto Composite Chitosan Biosorbent. Arab. J. Chem. 2017, 10, S1883–S1893. DOI: 10.1016/j.arabjc.2013.07.017.
- Aksu, Z.;. Determination of the Equilibrium, Kinetic and Thermodynamic Parameters of the Batch Biosorption of nickel(II) Ions onto Chlorella Vulgaris. Process Biochem. 2002, 38, 89–99. DOI: 10.1016/S0032-9592(02)00051-1.
- Zhu, H.; Tan, X.; Tan, L.; Zhang, H.; Liu, H.; Fang, M.; Hayat, T.; Wang, X. Magnetic Porous Polymers Prepared via High Internal Phase Emulsions for Efficient Removal of Pb2+ and Cd2+. ACS Sustainable Chem. Eng. 2018. DOI: 10.1021/acssuschemeng.7b04868.
- Zhu, H.; Yuan, J.; Tan, X.; Zhang, W.; Fang, M.; Wang, X. Efficient Removal of Pb2+ by Tb-MOFs: Identifying the Adsorption Mechanism through Experimental and Theoretical Investigations. Environ. Sci. Nano. 2019, 6, 261–272. DOI: 10.1039/C8EN01066H.
- Saha, P.; Chowdhury, S. (2011) Insight Into Adsorption Thermodynamics. Thermodynamics. InTechOpen. https://www.intechopen.com/books/thermodynamics/insight-into-adsorption-thermodynamics
- Carnin, R. L. P.; Folgueras, M. V.; Luvizão, R. R.; Correia, S. L.; Da Cunha, C. J.; Dungan, R. S. Use of an Integrated Approach to Characterize the Physicochemical Properties of Foundry Green Sands. Thermochim. Acta. 2012, 543, 150–155. DOI: 10.1016/j.tca.2012.05.018.
- Alkan, M.; Kalay, B.; Doğan, M.; Demirbaş, Ö. Removal of Copper Ions from Aqueous Solutions by Kaolinite and Batch Design. J. Hazard. Mater. 2008, 153, 867–876. DOI: 10.1016/j.jhazmat.2007.09.047.
- Yong, R. N.;. Geoenvironmental Engineering: Contaminated Soils, Pollutant Fate and Mitigation, 1st ed.; CRC Press, 2000. ISBN: 9780849382895 - CAT# 8289. DOI: 10.1088/1751-8113/44/8/085201.