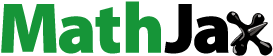
ABSTRACT
The rate at which avian offspring grow can have consequences for survival and reproductive output as an adult and is known to vary widely among and within species. This variation is thought to be an adaptive response to cope with environmental variation. The principal environmental factors affecting growth are food availability and predation risk, predominantly acting as constraints on parental care. Islands pose an interesting system to explore growth rate dynamics, because the characteristic insular features of high population densities and depauperate predator diversity translate into a potentially food limited environment with low predation risk. Insular environments typically produce populations with slower life history strategies and larger body size in small-bodied species, features that are likely to be mediated by growth rate. We describe the nestling growth of an insular population of Silvereyes and how it relates to parental size and parental care. Neither parental size nor parental care explained insular nestling growth rate, even though food acquisition is thought to underpin avian growth rates. This could be due to a mismatch between acquisition and allocation of resources by nestlings. Compared to a small number of mainland nestlings, the island growth curve asymptotes were significantly larger and inflection points much later, but insular growth rates were only marginally slower. This is in line with proposed insular adaptations required to produce larger body size on islands, however understanding the mechanism underlying this pattern will require data on the relationship between food quality and acquisition, and physiological allocation of resources within individuals.
Introduction
Growth rates are an important life history trait that can have long-term consequences for individual fitness (Gebhardt-Henrich and Richner Citation1998; Lindström Citation1999; Monaghan Citation2008) and can vary widely among and within species (Ricklefs Citation1968; Starck and Ricklefs Citation1998). Within species, growth rates can vary between populations under different environmental conditions (Ndithia et al. Citation2017; Sofaer et al. Citation2018), as well as among individuals within a population (Lameris et al. Citation2016). Understanding the causes of differences among individuals within populations provides the opportunity to develop a fine scale understanding of how strategies evolve. In mainland bird populations, higher predation risk is known to favour faster growth (Cheng and Martin Citation2012), but growth is also regulated by food availability (Drent and Daan Citation1980; Emlen et al. Citation1991). A recent meta-analysis showed a trend of slower growth in island species (Sandvig et al. Citation2019), but there are very few detailed studies of within population variation on islands (see Sofaer et al. Citation2018). Whether or not growth strategies in island populations show the same relationship with the key environmental drivers (predation risk and food availability) as that on the mainland remains poorly understood.
The joint effects of food availability and predation risk can affect growth rates by regulating how much energy a nestling receives. For example, in organisms with parental care, both factors can affect offspring provisioning rates, being lower in food-limited environments because of search times (Naef-Daenzer and Keller Citation1999; Tremblay et al. Citation2005), and lower in the presence of predators because of the time spent avoiding predators (Bosque and Bosque Citation1995; Goullaud et al. Citation2018). Furthermore, provisioning rates per nestling vary depending on brood size in food limited environments, being lower on average for larger brood sizes (Tremblay et al. Citation2003; Martin et al. Citation2011). All else being equal, lowered provisioning rates might be expected to lead to slower growth rates. However, several other factors can interact with the key drivers of food availability and predation risk to influence variation in growth rates among individuals. One additional factor is how resources are allocated within an individual (Arendt Citation1997; Sofaer et al. Citation2018; see review in Dmitriew Citation2011). In birds, nestlings in high predation environments may offset lowered provisioning rates by prioritising resource allocation to traits that allow them to increase their chances of survival by escaping predation (e.g. feather growth) (Martin et al. Citation2011). The allocation of resources towards growth or maintenance (e.g. thermoregulation) by nestlings can also be influenced by the amount of time parents spend brooding (Starck and Ricklefs Citation1998). Additionally, growth rates may be slower in small clutches compared to large ones, when adult survival is high, because adults may be prioritising their own survival over parental care (Ghalambor and Martin Citation2001) or show no relationship with clutch size if food is not limited (Tremblay et al. Citation2003; Martin et al. Citation2011). Growth rates can also vary depending on the timing of breeding, where early breeding generally favours faster growth in systems where it is important to match a distinct time of peak food availability (Sedinger and Flint Citation1991). The relative importance of these factors, and their interactions, determine the direction and magnitude of the selective pressures acting upon growth rates in a given population. Where nest predation rates are high and food is not limiting (characteristic of mainland environments), faster growth is favoured, whereas low predation but food limited environments (characteristic of islands) may favour slower growth rate (Palkovacs Citation2003; Sandvig et al. Citation2019). However, detailed descriptions of growth patterns within insular populations, their relationship with environmental drivers, parental behaviours, and intraspecific comparisons between island populations and their mainland relatives are lacking (Sandvig et al. Citation2019).
Here, we characterise the post-natal growth patterns of an island bird subspecies that embodies island evolution in a high density, low predation environment. The Capricorn Silvereye (Zosterops lateralis chlorocephalus) has achieved up to 40% increase in body size in less than 4000 years, compared to the adjacent mainland silvereye subspecies (Clegg et al. Citation2008). On Heron Island, the Capricorn Silvereye population shows high population densities and density dependence (McCallum et al. Citation2000), with population limiting processes being most pronounced in the winter period when reduced overwinter survival has been observed particularly for juveniles (Clegg et al. Citation2008; Sandvig et al. Citation2017). These food-limiting constraints may extend into the breeding season and impact growth rates. Larger adults are generally behaviourally dominant and win more interactions when competing for food (Robinson-Wolrath and Owens Citation2003). In the Heron Island population, nestling predation risk is low (<5%), compared to mainland Australia estimates (58.8%, nestlings = 51; Feeney and Webster unpublished). On Heron Island, nestlings are very occasionally predated by Buff-banded Rails (Galliralus philippensis), Silver Gulls (Chroicocephalus novahollandiae) or Eastern Reef Egrets (Egretta sacra) (Catterall et al. Citation1982) (EMS and SMC Pers. Obs.). These qualities make this population an interesting system for studying intraspecific variation in growth patterns.
In this study, we aim to describe within-population variation in growth rates and their association to parental size and parental care. We employ the use of nonlinear mixed models to estimate parameters that describe the growth trajectories for three morphological traits and determine the relationships between these parameters and parental body size, nestling hatch date, and brood size. We also characterise parental care investment using proxies of food provisioning rates over the nestling period and determine its relationship to growth parameters. According to the scenario presented by an insular, high density, low predation risk environment, we expect that nestling growth rates will be (1) faster in nestlings with larger parents, assuming an allometric scaling of the parents’ ability of acquiring food with their body size; (2) slower with increasing brood size, as per-nestling provisioning would decrease in a food-limited environment; and (3) faster in nestlings with higher parental provisioning rates. We further expect that (4) estimated asymptotic nestling body size will increase with parental provisioning rates, as higher per-nestling resource intake can translate into larger body size. Finally, we present a preliminary comparison of insular growth parameters with a small dataset from a mainland population of Silvereyes, with the expectation that (5) island nestling growth rates will be slower than those of mainland nestlings.
Methods
Study areas
Heron Island (23° 26′ S, 151° 57′ E) is a wooded island of the Capricorn-Bunker Group, covering an area of approximately 16 ha, located 70 km off the eastern Australian Coast on the southern tip of the Great Barrier Reef. The climate is subtropical with a mean annual rainfall of 1034.3 mm (1956–2007), and mean maximum and minimum temperatures of 26.2°C and 20.8°C (1962–2007), respectively (Australian Bureau of Meteorology, www.bom.gov.au). On the island, the Capricorn Silvereye is the only regularly breeding passerine (Kikkawa Citation1976) and over 12,000 individuals have been colour-banded since 1964 (Sandvig et al. Citation2017; SMC unpublished). The breeding season of Silvereye starts in late September (exceptionally early September) and ends in early March (exceptionally early April) (Sandvig et al. Citation2017). A cup-shaped nest is built by both parents in the crook of a branch between 0.5 m and 13 m high, predominantly in Pisonia grandis, the dominant tree species, but they will nest in most other tree, bush and herbaceous species found on the island. Incubation starts the day after the last egg is laid. Parents take turns incubating for a period of 10 to 14 days. Both parents share provisioning of nestlings, feeding them an assortment of insects and pieces of fig, the latter in an increasing proportion as they grow (Catterall et al. Citation1982). The nestling period lasts 10 to 15 days. Clutch size ranges from 1 to 4 eggs (exceptionally six), with a mode of 3 eggs (2015–2016).
Mainland fieldwork was conducted on the western side of Lake Samsonvale (27° 16’ S, 152° 51’ E) in south-east Queensland, the site of a large nest monitoring programme. The habitat is varied, with remnant dry sclerophyll forest dominated by various Eucalyptus species, patchy rainforest communities that primarily reside within some gullies, and large areas of grassland that is undergoing successive rounds of Eucalyptus revegetation by local stakeholders. Invasive species, such as lantana (Lantana camara) are also common in the understory. Silvereyes are an abundant member of the passerine community, and nests are opportunistically found and monitored. Between 2015 and 2020, Silvereye breeding activity has been consistent across years, with nests located between September and December each year. While the subspecies of the mainland Silvereyes associated with nests were not identified, banding records indicate that over 98% (59/60) of Silvereyes captured at this location, for which breeding status (presence of cloacal protuberance or brood patch) was recorded, were Z. l. cornwalli.
Nest searching and nestling measurements
On Heron Island, nest searching was conducted during October and November of 2015 and October to December of 2016. Nests were checked every 3 days during building and egg laying stages, and daily from a day before expected hatch date to determine an accurate hatching date. Nestlings were measured every 2 days between 14:00 and 17:30, beginning on day 2 (hatching day = day zero) until day 8. Nests were not approached after day 8 to prevent premature fledging. Measurements for wing length and tarsus length were taken with callipers and weight was recorded using a 20 g (±0.06 g) spring scale. At Lake Samsonvale, nest searching was conducted between August of 2018 and January of 2019. After being located, nests were checked approximately every 2 days to monitor nest progress and ascertain hatch date. Measurements were taken between 05:00 and 12:00, beginning on day two and measured approximately every 2 days. Measurements were taken following the same methods described for the island population.
Nest provisioning
On Heron Island, nests (n = 23) were recorded with action cameras (GoPro 3 Silver, www.gopro.com) for two to 3 hours between 14:30 and 18:30, on each day that nestlings were measured. Cameras were covered in a camouflage tape and positioned between 0.5 and 1 metre from nests and nesting pair behaviour was monitored to ensure camera placement was not detrimental to nest success (no cases of nest abandonment were recorded). The videos were scored for the number of trips made to the nest by both parents and the amount of time spent at the nest on each visit. Time was recorded starting from the first visit of a parent after installing or changing the battery of the camera. The individual parental identity could not be determined as colour bands could not always be easily seen in the shaded conditions of the nests; therefore, variables represent combined parental visits. Provisioning rates were calculated as the number of nest visits per nestling per hour. To assess if there was a temporal trend in provisioning rates as nestlings increased in age, we determined if the slope estimate in a simple linear model was significantly different from zero. For models describing growth parameters detailed below, we used the mean of the provisioning rate over the number of days recorded (3 or 4 days).
Growth rates
The logistic model is the most widely used equation for growth rates in passerines, and has proven to be a useful tool for interspecific comparisons of growth rates (Starck and Ricklefs Citation1998). We estimated growth curve parameters for weight (g), tarsus length (mm), and wing length (mm), by fitting the morphometric measurements from the nestlings to a logistic model of the form EquationEquation (1)(1)
(1) :
where = weight or length at time t (g or mm), A = asymptotic weight or length (g or mm), K = growth rate constant,
= the inflection point of the growth curve (days), and t = nestling age (days). Following methods using nonlinear mixed models described by Sofaer et al. (Citation2013) we first determine the best random effects structure to account for variation within the population on one or more of the three growth curve parameters; growth rate, inflection point and asymptote (n = 494 nestlings). We built models including single random effects of nest and nestling, as well as nestling nested within nest. If a model failed to converge, if the estimated random effect standard deviation was zero, or if the absolute value of the correlation between two random effects was 0.9 or higher, we concluded that the model was overparameterized, and hence was discarded (Pinheiro and Bates Citation2006). From this exercise we found that for each morphological trait (weight, tarsus and wing), the best random effects structure was one with a nested random effect (nestling within nest) on the asymptotic value and inflection point, with no random effect required for growth rate. This random effect structure was then used in all models testing for fixed effects.
To compare model performance we used an information-theoretic approach based on Akaike’s information criterion (AIC), considering ΔAIC values >2 to be evidence of one model performing better than another (Burnham and Anderson Citation2002). We considered P values <0.05 to show significant differences when comparing parameter means between mainland and island populations. We fit all models using maximum-likelihood using the ‘nlme’ package (Pinheiro et al. Citation2021) in R version 4.0.5 (R Core Team Citation2021).
Insular growth curve variation
To assess predictors of within population variation in the growth curve parameters we built models including fixed effects of parental body size, brood size, and hatch date. Parental body size was quantified using a principal component analysis (PCA) on adult morphometrics. We used the base function princomp and included measurements of weight, wing, tarsus, bill length to the posterior nostril opening, and bill depth and width taken at the anterior nostril opening from a five-year dataset (2012–2016, n = 859). The first Principal Component (PC1) was interpreted as adult body size with comparable loadings for weight, wing, tarsus, bill length, depth and width, and explained 29.5% of the variance. An exploratory model including PC1 for each parent separately was tested for possible differences in the effect between sexes, but no difference was found (results not shown). Given this result, and that nestling provisioning is shared between both parents (Wilson and Kikkawa Citation1988), we used average PC1 for each pair in subsequent models. Brood size was the number nestlings in the nest on each day of measuring. When a nestling died between measuring days, that nestling was subtracted from the brood size. Hatch date was transformed to a numeric vector starting on the first recorded hatching date of the study period. A full model was built with the three variables of average parental PC1, brood size and hatch date as fixed effects on the asymptote and growth rate parameters (n = 363 nestlings), but excluded the inflection point parameter. This is because on inclusion of a third parameter (inflection point), the model failed to converge. Because provisioning rates were only available for a subset of nests with growth measurements (23/62), we examined their effect on response variables of growth curve asymptote and then growth rate, considering provisioning rate as a single fixed effect, and including the same random-effects structure as previous models.
Comparing island and mainland growth parameters
We compared the growth curve parameter estimates for weight and tarsus length of the Heron Island population (N = 496) to that of the mainland population (N = 17). We fit a nonlinear mixed model using the same random effects structure of nestling nested within nest as previous models, and included parameters to estimate the difference in asymptote, inflection point and growth constant between island and mainland populations following methods described by Sofaer et al. (Citation2013).
Results
Nestling growth rate variation
We found a positive relationship between average parental body size and nestling asymptotic weight, and positive but marginally insignificant trends for asymptotic tarsus length and asymptotic wing length ()). There was some indication that later hatching dates were associated with longer asymptotic wing length but this was also marginally insignificant ()). None of the explanatory variables explained a significant portion of variation in the growth rate parameter K ()). The best random-effect model for the Heron Island population shows substantial variation in asymptotic values and the inflection point for all three morphological traits among nestlings and nests. Growth curve parameters for three morphological traits and the associations with fixed effects of parental body size, brood size and hatch date are shown in .
Table 1. List of parameter estimates (±1 SE) for (a) three-parameter logistic model (A = asymptote, I = inflection point, K = growth rate constant) with fixed effects on (b) the asymptote (A), modelled as Asymptotic value ~ mean parental body size + brood size + hatching date (AAd, ABrood, AHatch, respectively); (c) growth rate constant (K) modelled as growth rate value ~ mean parental body size + brood size + hatching date (KAd, Kbrood, Khatch, respectively); (d) provisioning rate, modelled as asymptotic value or growth rate ~ parental provisioning rate (AProv and KProv, respectively). Note that parental body size, brood size and hatching date were modelled together, while provisioning rates were modelled separately because of sample size differences. Values in bold indicate estimates with p-values: ^0.05 > p > 0.1; **p < 0.01; ***p < 0.001. For all other values, p > 0.1.
Nest provisioning and nestling growth
We found a significant increase in visits per nestling per hour as the nestling period progressed (, slope = 1.08 (0.15 SE), t value = 7.08, p = <0.001, n = 77). For the models including only provisioning as a fixed effect, we found a significant positive relationship of mean provisioning rates on asymptotic weight (t128 = 2.932, p = 0.004) and wing length (t128 = 2.557, p = 0.012). We found no effect of mean provisioning rates on growth rates for any of the morphological traits ()).
Figure 1. Number of visits per Silvereye nestling per hour by parents to the nest over the development period. The black line is the model fit from a linear mixed model of visits per nestling per hour as a function of day, with nest as a random factor. Points are jittered along the x axis to improve clarity.
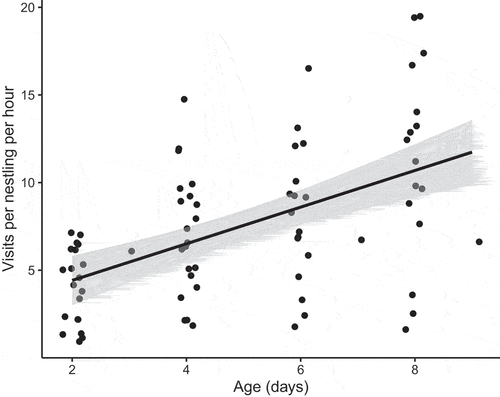
Comparing island and mainland growth parameters
We found a significant difference between estimated asymptotic weight and tarsus length for insular and mainland populations (; ). We also found significant differences in the inflection point for weight and tarsus length (). There were no significant differences between estimated growth rates in weight or tarsus length ().
Figure 2. Growth curves for weight (g), tarsus length (mm) and wing length (mm) of Silvereye nestlings. Lines are the predicted values for the best fit growth curve of each trait. Grey points and lines show logistic growth curves for the mainland Silvereye population.
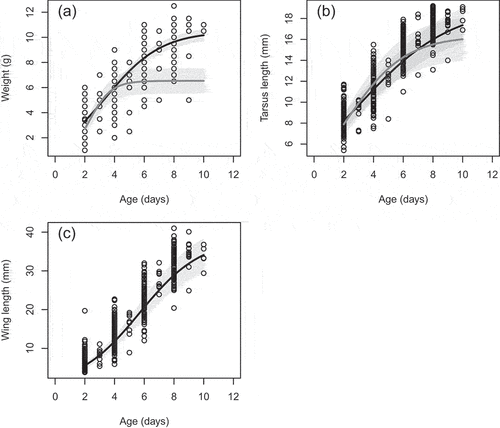
Table 2. List of parameter estimates (±1 SE) from three parameter logistic models testing differences in growth trajectories between island and mainland populations. Values in bold indicate estimates with p-values: ^0.05 > p > 0.1; **p < 0.01; ***p < 0.001. For all other values, p > 0.1.
Discussion
Variation among Silvereye nestlings on Heron Island was mostly explained by consistent variation in the asymptotic value and inflection point of the growth trajectories, but not of the growth rate, comparable to that reported for other altricial passerine populations (e.g. Ricklefs Citation1968; Sofaer et al. Citation2013). We were unable to explain variation in growth rates despite examining several key drivers implicated in other cases. Contrary to expectations, the measured maximum relative growth rates of nestlings were not related to parental body size, brood size or clutch-level parental provisioning rate. However, we did find that the asymptote of some traits was related to parental body size and provisioning rate. The relationship between direct and indirect factors on nestling growth can be complex (Segura and Palacio Citation2022). Simple relationships that explain growth rate variation in Silvereye nestlings may be masked or negated by a suite of non-mutually exclusive conditions or incorrect assumptions that we expand on below.
Quality versus quantity of nestling provisions
The quantity of food provided to nestlings during their growth trajectory was demonstrably important, with increasing rates as nestlings aged. However, provisioning rates did not explain growth rate variation among nests. Other studies have also reported that provisioning rate did not explain nestling weight gain (Sofaer et al. Citation2018) or weight at fledging (though number of larger food items did) (Schwagmeyer and Mock Citation2008). Food acquisition is thought to regulate most within-population growth rate variation (Ricklefs Citation1976; Konarzewski et al. Citation1996; Searcy et al. Citation2004; Killpack and Karasov Citation2012), but provisioning rates may be secondary to the type or quality of food delivered to nestlings (Sofaer et al. Citation2018). Segura et al. (Citation2019) found that the quality of prey items was important in determining if provisioning rates directly influenced growth rates or not. In Heron Island Silvereyes, the amount of fig and insect food provided has measurable effects on a range of reproductive outcomes. Catterall et al. (Citation1982) found that the proportion of figs to arthropods (insects are the main source of protein needed for growth) fed to Silvereye nestlings increased as they grew, and pairs that fledged more offspring, fed nestlings more arthropods, nested in areas with higher fruiting fig tree density and were older. The methods used in our study were not able to consistently identify food types provided to nestlings, but food type rather than provisioning rate may be a more appropriate variable for examining growth rate association in generalist foragers.
Temporal changes in the quantity or quality of food could also help explain why nestlings with longer wings were produced as the breeding season progressed. We do not have parallel information on food availability over the time of this study; however, an earlier study on the island found that arthropod abundance declined as the breeding season progressed (Eguchi Citation1993). Food availability fluctuates with climate conditions, and thus our findings of larger winged nestlings later in the season may be a function of the climatic conditions experienced during the study period and not a general pattern on the island. It is also important to note that our study period did not extend to the end of the potential breeding season (i.e. early April). Thus, it may be that the end of our study period coincided with a peak in food abundance, before a subsequent decline (as documented in Eguchi Citation1993).
The temporal and spatial heterogeneity in the nutritional contents of provisioning adds an additional layer of complexity to the provisioning–growth relationship. Isotope analyses of feathers or faecal material across the nestling growth period would allow quantification of the variability in protein versus plant dietary provisions among and within nestlings (Podlesak et al. Citation2005; Inger and Bearhop Citation2008) to compare to growth parameters.
Behaviour and provisioning
In Heron Island Silvereyes, larger adults tend to be behaviourally dominant, winning more agonistic interactions in competition for food (Robinson-Wolrath and Owens Citation2003), yet this did not translate to faster nestling growth rates for offspring of larger-bodied parents. This could be due to several reasons. First, behavioural dominance and provisioning rates by adults may be uncoupled, if, for example, parents prioritise food intake for themselves to maximise survival. Second, relationships between behavioural dominance, provisioning and growth rates may be dependent on climatic variability within and among breeding seasons. For example, Eguchi (Citation1993) found that when food supply was poor, parental provisioning rates were lower, but dominant pairs raised nestlings in better condition compared to subordinate and intermediate classes. This difference was not detected in times of more abundant food supply. Hence, the environmental conditions during the timing of our study may not have been sufficiently harsh for associations between body size (as a proxy for dominance) and provisioning rates to emerge. A further line of evidence comes from the lack of association between brood size and growth rates for Silvereye nestlings. Under food-limited conditions, growth rates are predicted to decrease with increasing brood size (Martin et al. Citation2011). Hence, our findings suggest that over the two breeding seasons examined, the island was not an exceptionally food-limited environment, i.e. they were ‘good’ years for Silvereye reproduction, therefore food quantity and quality was unlikely to greatly differ among nests. This may go some way to explaining the lack of associations observed.
Acquisition versus allocation of resources in nestlings
An additional explanation for a lack of observed explanations for variation in growth rates could be a mismatch between the nestlings’ acquisition of the food provided by the parents and the actual allocation of these resources towards the traits that we measured. Nestlings are able to prioritise the allocation of food differentially between traits during development (e.g. prioritise development of internal organs over flight feathers) depending on selective pressures (Martin et al. Citation2011). The allocation of resources can also be affected by the microclimate experienced by nestling at the nest (Sauve et al. Citation2021). For example, nestlings in colder conditions may need to allocate more energy to thermoregulation. In the case of insular environments, a lower predation pressure may shift allocation prioritisation from fast growth in flight feathers, typical in high predation environments, to development of internal systems and maintenance. This could produce higher quality fledglings, better able to compete in high-density populations often found on islands. However, it may also be the case that the limited length of the recordings was not able to explain variation in growth rates, as patterns of parental investment can vary throughout the day (Low et al. Citation2008). This requires more detailed investigation of parental care with longer periods of recording.
Island versus mainland population growth curves
The mechanisms that underlie the taxonomically and geographically widespread phenomenon of the ‘island syndrome’, which includes the ‘island rule’ describing a tendency towards medium body size (Clegg and Owens Citation2002) and a shift towards slower life history traits (Covas Citation2012), are still poorly understood. Detailed descriptions of insular growth strategies are important because a shift towards slower growth has been suggested to underpin body size evolution on islands (Palkovacs Citation2003). A phylogenetically controlled meta-analysis of growth rates in birds showed that island birds tend to grow slower than mainland ones, after accounting for a range of confounding factors including latitude, clutch size, adult body size, and nest type (Sandvig et al. Citation2019). Given high adult survival (Sandvig et al. Citation2017), low predation environment (Catterall et al. Citation1982) and strong intraspecific competition (Robinson-Wolrath and Owens Citation2003) in the Heron Island population of Silvereyes, maximising phenotypic quality of nestlings by means of slower nestling growth would be expected. At the embryonic stage, slower growth has been related to increased individual quality and fitness (Ricklefs et al. Citation2017). While we did not find a significant difference in growth rate between island and mainland birds, the trend was in the expected direction – that of slower growth in the island Silvereyes. Additionally, the inflection points for both weight and tarsus length were significantly later for the island population. In combination, the slower growth rate trend, and the later inflection point for weight and tarsus is consistent with a scenario of decreased pressure on nestlings to develop quickly to escape nest predation. In the only other published comparisons that we could find between island and mainland populations in a small passerine (Orange-crowned Warbler, Leiothlypis Sofaer et al. (Citation2013), Sofaer et al. Citation2018) also reported slower growth in the island population. However, the mainland population was located at a much higher latitude than the island, and differences in environmental factors at high latitudes (i.e. higher productivity, longer photoperiod and shorter breeding season), may explain the faster mainland growth rate in that comparison. In our study, data for the island and mainland populations were collected in different years. Differences in environmental conditions between years in terms of climate and food availability can explain variability in growth rates (McCarty Citation2001; Sauve et al. Citation2021), and could potentially be a better predictor than island/mainland differences in this case. Finally, it is important to note that the sample size for the available mainland Silvereye data in this study was small and thus our results may reflect reduced statistical power to detect growth rate differences. Nevertheless, the comparison we include here provides sufficient information to show how the dynamics of growth trajectories from mainland to island can differ, culminating in the significant size differences between island and mainland Silvereyes (Clegg et al. Citation2008).
Conclusions
In contrast to most studies of nestling growth rate variation that have examined populations facing environmental constraints of food limitation and predation risk, our study population experiences negligible predation risk and, in the data collection period at least, possibly minimal food limitation during the breeding season. Our results shed light on the complex relationships driving growth rate variation of nestlings in this type of environment. They also highlight the need for a more detailed examination of parental care, looking into the quality and quantity of food items, incorporating the spatial and temporal variation related to dominance hierarchies, and how these resources are allocated physiologically. The maximum growth rate parameter has often been the focus of interspecific studies of growth trajectories because of its variability, but this parameter showed low within-population variability among Heron Island Silvereyes. The inflection point, which described the age at which the maximum growth rate is reached, may be an equally interesting aspect of growth trajectories that merits further attention, especially in intraspecific studies. Finally, extending this study to compare the drivers of growth variation with a mainland population of Silvereyes at a similar latitude, with the same level of detail as suggested above, would provide a more nuanced understanding of how the selective pressures in local environments shape nestling growth strategies between populations on different evolutionary trajectories and contribute to a mechanistic understanding of key insular adaptations.
Permits
This study was conducted under ethics clearance from Griffith University Ethics Committee (ENV/24/13/AEC and ENV/08/19/AEC), Queensland Government Department of Environment and Heritage Protection Scientific Purposes permit (WITK14627014 to SMC), and (WISP16200615 to WF), Australian Bird and Bat Banding Scheme project licences and personal banding licences to SMC, EMS and WF.
Acknowledgements
We greatly appreciate the assistance provided by the staff at the Heron Island Research Station, especially Scott Latimer for help with field work. We also thank Fiona Robertson for field work contributions. We also thank SeqWater for their continued support of research at Lake Samsonvale and Michael S Webster for his contributions as a co-principal investigator on the Lake Samsonvale project.
Disclosure statement
No potential conflict of interest was reported by the author(s).
Additional information
Funding
References
- Arendt, J. D. 1997. Adaptive intrinsic growth rates: An integration across taxa. The Quarterly Review of Biology 72, 149–177. doi:10.1086/419764
- Bosque, C., and Bosque, M. T. 1995. Nest predation as a selective factor in the evolution of developmental rates in altricial birds. The American Naturalist 145, 234–260. doi:10.1086/285738
- Burnham, K., and Anderson, D. (2002). ‘Model Selection and Multimodel Inference,’ 2nd edn. (Springer-Verlag: New York Berlin Heidelberg.)
- Catterall, C. P., Wyatt, W. S., and Henderson, L. J. 1982. Food resources, territory density and reproductive success of an island Silvereye population Zosterops lateralis. Ibis 124, 405–421. doi:10.1111/j.1474-919X.1982.tb03789.x
- Cheng, Y.-R., and Martin, T. E. 2012. Nest predation risk and growth strategies of passerine species: Grow fast or develop traits to escape risk? The American Naturalist 180, 285–295. doi:10.1086/667214
- Clegg, S. M., and Owens, I. P. F. 2002. The ‘island rule’ in birds: Medium body size and its ecological explanation. Proceedings of the Royal Society B 269, 1359–1365. doi:10.1098/rspb.2002.2024
- Clegg, S. M., Owens, I. P. F., Tavecchia, G., Kikkawa, J., Frentiu, F. D., Frentiu, F. D., Kikkawa, J., et al. 2008. 4000 years of phenotypic change in an island bird: Heterogeneity of selection over three microevolutionary timescales. Evolution; International Journal of Organic Evolution 62, 2393–2410. doi:10.1111/j.1558-5646.2008.00437.x
- Covas, R. 2012. Evolution of reproductive life histories in island birds worldwide. Proceedings of the Royal Society B: Biological Sciences 279, 1531–1537. doi:10.1098/rspb.2011.1785
- Dmitriew, C. M. 2011. The evolution of growth trajectories: What limits growth rate? Biological Reviews 86, 97–116. doi:10.1111/j.1469-185X.2010.00136.x
- Drent, R. H., and Daan, S. (1980). The prudent parent: Energetic adjustments in avian breeding. Ardea 68, 225–252.
- Eguchi, K. 1993. Temporal changes in food resources, parental feeding and breeding success of Heron Island Silvereyes, Zosterops lateralis chlorocephala. Ecological Research 8, 319–327. doi:10.1007/bf02347191
- Emlen, S. T., Wrege, P. H., Demong, N. J., and Hegner, R. E. 1991. Flexible growth rates in nestling white-fronted bee-eaters: A possible adaptation to short-term food shortage. The Condor 93, 591–597. doi:10.2307/1368191
- Gebhardt-Henrich, S., and Richner, H. 1998. Causes of growth variation and its consequences for fitness. In ‘Avian Growth and Development.’ pp. 324–339. (New York: Oxford University Press.)
- Ghalambor, C. K., and Martin, T. E. 2001. Fecundity-survival trade-offs and parental risk-taking in birds. Science 292, 494–497. doi:10.1126/science.1059379
- Goullaud, E. L., De Zwaan, D. R., and Martin, K. 2018. Predation risk-induced adjustments in provisioning behavior for horned lark (Eremophila alpestris) in British Columbia. The Wilson Journal of Ornithology 130, 180–190. doi:10.1676/16-150.1
- Inger, R., and Bearhop, S. 2008. Applications of stable isotope analyses to avian ecology. Ibis 150, 447–461. doi:10.1111/j.1474-919X.2008.00839.x
- Kikkawa, J. (1976). The birds of the great barrier reef. In ‘Biology and Geology of Coral Reefs. Vol. III’. (Eds O. A. Jones and O. A. Jones). (London).
- Killpack, T. L., and Karasov, W. H. 2012. Growth and development of house sparrows (Passer domesticus) in response to chronic food restriction throughout the nestling period. Journal of Experimental Biology 215, 1806–1815. doi:10.1242/jeb.066316
- Konarzewski, M., Kowalczyk, J., Swierubska, T., and Lewonczuk, B. 1996. Effect of short-term feed restriction, realimentation and overfeeding on growth of song thrush (Turdus philomelos) nestlings. Functional Ecology 10, 97–105. doi:10.2307/2390267
- Lameris, T. K., Fijen, T. P. M., Urazaliev, R., Pulikova, G., Donald, P. F., and Kamp, J. 2016. Breeding ecology of the endemic black lark Melanocorypha yeltoniensis on natural steppe and abandoned croplands in post-Soviet Kazakhstan. Biodiversity and Conservation 25, 2381–2400. doi:10.1007/s10531-015-1041-2
- Lindström, J. 1999. Early development and fitness in birds and mammals. Trends in Ecology and Evolution 14, 343–348. doi:10.1016/S0169-5347(99)01639-0
- Low, M., Eggers, S., Arlt, D., and Pärt, T. 2008. Daily patterns of nest visits are correlated with ambient temperature in the Northern Wheatear. Journal of Ornithology 149, 515–519. doi:10.1007/s10336-008-0300-3
- Martin, T. E., Lloyd, P., Bosque, C., Barton, D. C., Biancucci, A. L., Cheng, Y.-R., and Ton, R. 2011. Growth rate variation among passerine species in tropical and temperate sites: An antagonistic interaction between parental food provisioning and nest predation risk. Evolution 65, 1607–1622. doi:10.1111/j.1558-5646.2011.01227.x
- McCallum, H., Kikkawa, J., and Catterall, C. 2000. Density dependence in an island population of Silvereyes. Ecology Letters 3, 95–100. doi:10.1046/j.1461-0248.2000.00120.x
- McCarty, J. P. 2001. Variation in growth of nestling tree swallows across multiple temporal and spatial scales. The Auk 118, 176–190. doi:10.1093/auk/118.1.176
- Monaghan, P. 2008. Early growth conditions, phenotypic development and environmental change. Philosophical Transactions of the Royal Society B: Biological Sciences 363, 1635–1645. doi:10.1098/rstb.2007.0011
- Naef-Daenzer, B., and Keller, L. F. 1999. The foraging performance of great and blue tits (Parus major and P. caeruleus) in relation to caterpillar development, and its consequences for nestling growth and fledging weight. Journal of Animal Ecology 68, 708–718. doi:10.1046/j.1365-2656.1999.00318.x
- Ndithia, H. K., Bakari, S. N., Matson, K. D., Muchai, M., and Tieleman, B. I. 2017. Geographical and temporal variation in environmental conditions affects nestling growth but not immune function in a yearround breeding equatorial lark. Frontiers in Zoology 14, 1–14. doi:10.1186/s12983-017-0213-1
- Palkovacs, E. P. 2003. Explaining adaptive shifts body size on islands: A life history appoach. Oikos 103, 37–44. doi:10.1034/j.1600-0706.2003.12502.x
- Pinheiro, J., and Bates, D. (2006). ‘Mixed-effects Models in S and S-PLUS.’ (New York: Springer Science & Business Media.)
- Pinheiro, J., Bates, D., DebRoy, S., Sarkar, D., and R Core Team. 2021. nlme: Linear and nonlinear mixed effects models. R package version 3.1-152.
- Podlesak, D. W., McWilliams, S. R., and Hatch, K. A. 2005. Stable isotopes in breath, blood, feces and feathers can indicate intra-individual changes in the diet of migratory songbirds. Oecologia 142, 501–510. doi:10.1007/s00442-004-1737-6
- R Core Team. 2021. ‘R: A Language and Environment for Statistical Computing.’ (R Foundation for Statistical Computing: Vienna, Austria.) http://www.r-project.org/
- Ricklefs, R. E. 1968. Patterns of growth in birds. Ibis 110, 419–451. doi:10.1111/j.1474-919X.1968.tb00058.x
- Ricklefs, R. E. 1976. Growth rates of birds in the humid new world tropics. Ibis 118, 179–207. doi:10.1111/j.1474-919X.1976.tb03065.x
- Ricklefs, R. E., Austin, S. H., and Robinson, W. D. 2017. The adaptive significance of variation in avian incubation periods. The Auk 134, 542–550. doi:10.1642/AUK-16-171.1
- Robinson-Wolrath, S. I., and Owens, I. P. F. 2003. Large size in an island-dwelling bird: Intraspecific competition and the dominance hypothesis. Journal of Evolutionary Biology 16, 1106–1114. doi:10.1046/j.1420-9101.2003.00615.x
- Sandvig, E. M., Coulson, T., Kikkawa, J., and Clegg, S. M. 2017. The influence of climatic variation and density on the survival of an insular passerine Zosterops lateralis. PLoS ONE 12, e0176360. doi:10.1371/journal.pone.0176360
- Sandvig, E. M., Coulson, T., and Clegg, S. M. 2019. The effect of insularity on avian growth rates and implications for insular body size evolution. Proceedings of the Royal Society B: Biological Sciences 286, 20181967. doi:10.1098/rspb.2018.1967
- Sauve, D., Friesen, V. L., and Charmantier, A. 2021. The effects of weather on avian growth and implications for adaptation to climate change. Frontiers in Ecology and Evolution 9, 1–20. doi:10.3389/fevo.2021.569741
- Schwagmeyer, P. L., and Mock, D. W. 2008. Parental provisioning and offspring fitness: Size matters. Animal Behaviour 75, 291–298. doi:10.1016/j.anbehav.2007.05.023
- Searcy, W. A., Peters, S., and Nowicki, S. 2004. Effects of early nutrition on growth rate and adult size in song sparrows Melospiza melodia. Journal of Avian Biology 35, 269–279. doi:10.1111/j.0908-8857.2004.03247.x
- Sedinger, J. S., and Flint, P. L. 1991. Growth rate is negatively correlated with hatch date in black brant. Ecology 72, 496–502. doi:10.2307/2937190
- Segura, L. N., Gonzalez, E., Harguindeguy, F. M., Colombo, M. A., and Jauregui, A. 2019. Food delivery rates increased with forest canopy cover and nestling growth improved with arachnids in the masked gnatcatcher Polioptila dumicola. Acta Ornithologica 54, 233–241. doi:10.3161/00016454AO2019.54.2.009
- Segura, L. N., and Palacio, F. X. 2022. Quantifying the relative importance of direct and indirect effects influencing bird nestling growth. Integrative Zoology 17, 408–419. doi:10.1111/1749-4877.12544
- Sofaer, H. R., Chapman, P. L., Sillett, T. S., and Ghalambor, C. K. 2013. Advantages of nonlinear mixed models for fitting avian growth curves. Journal of Avian Biology 44, 469–478. doi:10.1111/j.1600-048X.2013.05719.x
- Sofaer, H. R., Sillett, T. S., Yoon, J., Power, M. L., Morrison, S. A., and Ghalambor, C. K. 2018. Offspring growth and mobility in response to variation in parental care: A comparison between populations. Journal of Avian Biology 49, e01646. doi:10.1111/oik.02629
- Starck, J. M., and Starck, J. M. (Eds.) 1998. ‘Avian Growth and Development: Evolution within the altricial-precocial Spectrum.’ (New York: Oxford University Press.)
- Tremblay, I., Thomas, D. W., Lambrechts, M. M., Blondel, J., and Perret, P. 2003. Variation in blue tit breeding performance across gradients in habitat richness. Ecology 84, 3033–3043. doi:10.1890/02-0663
- Tremblay, I., Thomas, D., Blondel, J., Perret, P., and Lambrechts, M. M. 2005. The effect of habitat quality on foraging patterns, provisioning rate and nestling growth in Corsican blue tits Parus caeruleus. Ibis 147, 17–24. doi:10.1111/j.1474-919x.2004.00312
- Wilson, S. M., and Kikkawa, J. 1988. Post-fledging parental investment in the Capricorn Silvereye. Emu 88, 81–87. doi:10.1071/MU9880081