ABSTRACT
The Night Parrot Pezoporus occidentalis is a critically endangered, elusive, nocturnal bird that is difficult to study in the field. Much is unknown about its biology, including how it navigates at night. A previous investigation of the eye and brain revealed that it likely has poor nocturnal vision, but its other sensory abilities, and overall skull anatomy, have not yet been investigated. This osteological study, based on micro-CT-scans of the holotype, describes and compares the anatomy of the Night Parrot skull, and identifies its possible ecological adaptations. Specialised ear anatomy suggests that the Night Parrot has enhanced acoustic abilities, in contrast with its diurnal congener P. wallicus and the only other nocturnal parrot, the Kakapo Strigops habroptila. The Night Parrot’s uniquely enlarged exoccipital bones may amplify sound, and bilateral ear asymmetry, not identified in any other parrot species, likely enhances its directional hearing, as in many species of owl. Enlarged exoccipitals displace the zygomatic processes and constrain orbit size, suggesting an evolutionary trade-off between hearing and vision. The auditory abilities of the species require deeper investigation, but our anatomical observations indicate that the potential impacts of noise disturbance may warrant consideration in Night Parrot conservation strategies as a precaution.
Introduction
The endemic Australian Night Parrot P. occidentalis is one of only two strictly nocturnal parrots in the world, along with the Kakapo Strigops habroptila of New Zealand. It is one of three species in the ground-dwelling parrot genus Pezoporus, its diurnal sister-group taxa being the Eastern Ground Parrot, P. wallicus, and the Western Ground Parrot, P. flaviventris. The Night Parrot inhabits arid inland Australia, while the two ground-parrot species live in mesic subcoastal habitats. The nocturnal and diurnal Pezoporus lineages are estimated to have last shared a common ancestor around 3 million years ago (Joseph et al. Citation2011).
In describing the Night Parrot, Gould (Citation1861) considered it sufficiently distinctive to warrant a new genus, naming it Geopsittacus occidentalis, but this was soon challenged by naturalists who argued for its inclusion in Pezoporus (Finsch Citation1867; Murie Citation1868). The genus name Geopsittacus remains a valid taxonomic option, although Pezoporus is generally favoured (Joseph et al. Citation2011).
The biology of P. occidentalis is poorly understood due to its elusiveness, small and geographically diffuse population, remote habitat, and nocturnality. Apparently reasonably common until the late 19th Century, the species declined thereafter. Following the last collection of a live specimen for a museum in 1912, multiple expeditions over eight decades produced no records verified by material evidence (Olsen Citation2018). Sporadic incidental sightings fed hope that it persisted (Horton et al. Citation2021; Leseberg et al. Citation2021), its survival eventually confirmed by two dead specimens from Queensland in 1990 (Boles et al. Citation1994) and 2006 (McDougall et al. Citation2009). A living population was finally discovered in south-west Queensland in 2013 (Pyke and Ehrlich Citation2014).
The last decade has seen research into its distribution, diet, behaviour, reproduction and habitat (e.g. Murphy et al., Citation2017a; Murphy et al., Citation2017b). Renewed interest coupled with improved knowledge and technology, such as acoustic monitoring, has facilitated collaborations between scientists, Traditional Owners and Indigenous ranger groups, leading to the discovery of further populations in Western Australia (Hamilton et al. Citation2017; Jackett et al. Citation2017; Leseberg et al. Citation2021). A record from northern South Australia was later ruled to be based on false evidence (Olsen and Menkhorst Citation2020). The species is Critically Endangered under IUCN criteria because the number of mature individuals is estimated to be 40–500 (best estimate, 200), the population is severely fragmented, and is likely declining (BirdLife International Citation2022). The species is more secure than once feared, but it remains poorly understood with an uncertain future. It is one of 22 bird species prioritised for recovery in the national Threatened Species Action Plan (DCCEEW Citation2022).
Despite recent advances in knowledge, Night Parrot anatomy and sensory ecology remain poorly understood. Its visual capabilities were recently investigated via CT-imaging the skull of a mummified specimen (Iwaniuk et al. Citation2020). The eyes, optic nerve foramina and optic lobes of the brain were shown to be small compared to related species, and the authors inferred that low-acuity night vision may make the Night Parrot vulnerable to hazards like fence strike. In the Kakapo, olfaction is thought to be highly developed (Hagelin Citation2004), but it remains to be determined what, if any, specialist adaptations the Night Parrot has that might compensate for poor night vision. How it navigates is fundamental to understand given that a GPS-tagged individual travelled nearly 10 km from its roost sites to feed, covering ~3,000 ha over five tracking nights (Murphy et al., Citation2017b).
Here, we further describe and compare the cranial anatomy of the species, based on CT-imaging the holotype. We aim to improve understanding of its adaptations, and contribute towards refining conservation strategies for the Night Parrot.
Materials & methods
Pezoporus occidentalis holotype
NHMUK 1868.1.27.30 is a preserved skin in the Ornithology collection of the Natural History Museum, Tring, UK. Described by Gould (Citation1861), it is believed to have been shot ~13 km south-east of Mount Farmer during the 1854 Austin expedition to the Murchison region of Western Australia (Wilson Citation1937). It preserves a partial, osteologically mature skeleton, of which the cranium is described here.
The cranium has minor damage in places, but retains intact delicate structures such as the palatines and scleral rings. The skin is mounted on a metal rod, which has artificially enlarged the foramen magnum and penetrates the right frontal bone just medial of the orbital rim. There is also some damage to the wall of the right posterior cranium.
Comparative specimens
The skull was compared with those of parrots representing all Australian groups of Psittaciformes, including Cacatuidae, Platycercini, Pezoporini, Loriini, Melopsittacini, Cyclopsittini, Polytelini and Psittaculini, as well as with the only other specialist nocturnal parrot, S. habroptila (see specimen list in Supp. Info.). Physical specimens were compared where possible, supplemented with 3D models from CT-scans on MorphoSource. No skeletal specimens of P. occidentalis exist. A 3D model of the previously scanned juvenile P. occidentalis skull (QM O.29055; http://n2t.net/ark:/87602/m4/M115798) shows signs of osteological immaturity, and has some damage presumably due to vehicle strike, so was used for comparison of certain features only. No skeletal specimens of the endangered P. flaviventris are available, but its anatomy is presumed to be nearly identical with closely related P. wallicus, until recently regarded as conspecific (Murphy et al. Citation2011).
Physical specimens were examined and measured by ERS, and photographed using a Canon EOS 5D Mark II with a 100 mm lens at an aperture of F4.5, mounted on a motorised focus stacking rig. Stacked images were created using the software package Zerene Stacker v. T2021-02-16-2045. Photographic plates were made using the Adobe packages Photoshop CC 2019 and Illustrator CC 2019.
CT-scanning & modelling
The P. occidentalis holotype was scanned using a Nikon XT H 225 ST (Nikon Metrology, Leuven, Belgium) at the X-ray micro-CT laboratory, Imaging and Analysis Centre, Natural History Museum, London, UK, using the following parameters: voltage 120 kV; current 833 µA; 2254 projections; and a 1 mm copper filter. A full body scan had a resultant resolution of 56 microns (µm).
Reconstructed datasets were segmented and visualised by AMC using Mimics v. 19.0 (Materialise, Belgium). Digital 3D models of the specimen were created from micro computed tomography (µCT) data. Scan data and the resultant 3D models are available from MorphoSource at https://www.morphosource.org/projects/000433563?locale=en.
Measurements
Physical specimens were measured using digital callipers and rounded to the nearest 0.1 mm. CT-scanned specimens were measured onscreen using the digital measuring tools in Mimics v. 19.0. Angles of key structures (e.g. divergence of mandibular rami) were measured with a protractor on the on-screen images used in the photographic plates.
Terminology
Anatomical terminology follows Baumel et al. (Citation1993) unless otherwise stated. Nomenclature for genera and species follows the IOC World Bird List 13.1, and names of parrot families, subfamilies and tribes follows Joseph et al. (Citation2012).
Statistical comparisons
We compared internal and external scleral ring diameter for NHMUK 1868.1.27.30 with published data on parrots and nocturnal birds (Choiniere et al. Citation2021), to infer visual function in P. occidentalis. A bivariate linear model (ordinary least squares regression) was produced using the statistical package PAST v.3 (Hammer et al. Citation2001) (see Hall Citation2008; Choiniere et al. Citation2021).
Results
Description & comparisons
Cranial vault
Approximate locations of the main bones of the neurocranium are identified in (), with reference to Thompson (Citation1899), Baumel et al. (Citation1993) and Mayr and Manegold (Citation2020). Boundaries between skull plates in adult birds are obscured by extensive fusion, with plate margins only clear in very young birds (Mayr and Manegold Citation2020), and so the interpretation in () should be considered a guide only. Major cranial features are labelled in ().
Figure 1. Cranium of the Night Parrot P. occidentalis holotype (NHMUK 1868.1.27.30, https://doi.org/10.17602/M2/M488551). a, bones of the neurocranium; b, major cranial features; c, right lateral view; d, left lateral view; e, dorsal view; f, anterior view; g, posterior view; h, ventral view.
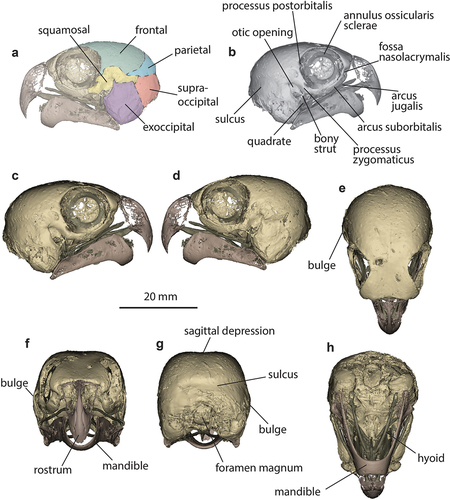
Overall size and shape of the cranium (https://doi.org/10.17602/M2/M434579) conforms with most psittaculids, with a relatively short, bulbous cranial vault that is widest posteriorly, tapering towards narrower frontals, and a relatively small rostrum and mandible. It is small compared to crania of most cacatuids, which are typically large with a proportionally much larger rostrum and mandible, and are widest just posterior to the orbits, tapering posteriorly. Only the Cockatiel, Nymphicus hollandicus, has a cranium that could be mistaken for that of P. occidentalis based on size and gross morphology. It is distinguished by its proportionally broader cranium, broader frontals, more widely flared lacrymals, untapered cranial vault, much smaller exoccipitals, larger otic openings that are placed further posteriorly and lower on the cranium, relatively larger orbits, and posteriorly forked arcus suborbitalis. The cranium of P. occidentalis is dissimilar to that of the only other strictly nocturnal parrot, Strigops habroptila, which has a much larger, more cockatoo-like skull, with an elongate rather than bulbous cranial vault, broad frontals, and a proportionally much larger rostrum.
The holotype cranium is within a similar size range to those of most members of the Platycercini, Loriini and Polytelini, but is larger than in other pezoporines, consistent with Gould’s (Citation1861) observation of the specimen’s ‘thick bluffy head’. All species of Neophema examined, and Neopsephotus bourkii, are much smaller than P. occidentalis and could not be mistaken for it despite similar gross cranial morphology (). In the Pezoporini, only P. wallicus approaches P. occidentalis in cranial size.
Figure 2. Crania of pezoporine parrots in lateral (top row), dorsal (middle), and ventral (bottom row) views (scaled to same height/length). Left to right: P. occidentalis holotype, NHMUK 1881.1.17.71 (https://doi.org/10.17602/M2/M434579); P. wallicus, ANWC 23818; Neopsephotus bourkii, SAM B49119; Neophema splendida, SAM B32903; Neophema petrophila, SAM B55096; Neophema elegans, SAM B51230; Neophema chrysostoma, SAM B3110.
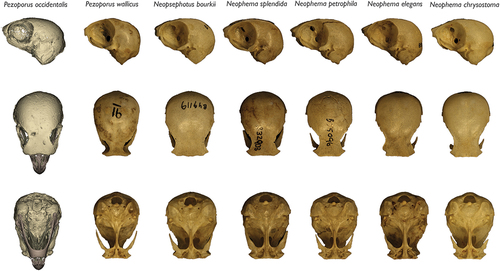
In posterior view, a sagittal depression separates left and right dorsal prominences (), matching the other scanned specimen (QM O.29055). In all other compared psittaculines, the cranial vault is smoothly rounded. The foramen magnum is 5.3 mm wide and 8.5 mm long, but the length is inaccurate because the posterior margin has been enlarged by the metal mounting rod. The condylus occipitalis has been broken off. Due to the damaged foramen magnum and missing spinal column, the angle at which the cranium articulates with the neck could not be determined.
One of the most distinctive features of the cranium is the enlarged exoccipitals (), which form the external wall of the tympanic cavities. These occupy roughly one-third of cranial length, more than in any species examined, and are posteriorly convex (quarter of cranial length and posteriorly concave in P. wallicus; ). The exoccipitals are somewhat enlarged in all pezoporines relative to members of other psittaculid clades, especially in Neopsephotus bourkii and Neophema splendida, but in none are they so exaggerated ().
The other particularly notable feature is bilateral asymmetry not observed in any other parrot species. One asymmetrical feature is a broad but shallow sulcus encircling the right side of the neurocranium, beginning just posterior to the right ear, traversing the posterior part of the cranium through the exoccipital, supraoccipital and parietal regions, terminating just past the midline (; https://doi.org/10.17602/M2/M488551). The corresponding left side of the cranium is convex posterior to the ear, although the continuation of the right-sided sulcus is visible in left lateral view where the parietal and supra-occipital meet (). The previously scanned specimen, QM O.29055, lacks a corresponding sulcus and its significance in the holotype is unclear. It appears to be endogenous and not due to post-mortem damage during preparation, because the indented bone is neither creased nor fractured. There is some overt damage to the right posterior cranium below the sulcus, visible in CT-imagery as a portion of the thin bony wall of the skull having been pushed inwards into the cranial cavity, but this appears separate from, not contiguous with, the sulcus, which displays no similar breakage. In some cacatuids (e.g. Callocephalon fimbriatum, Lophochroa leadbeateri, Cacatua galerita), and in Neophema elegans, both sides of the cranium bear deep, elongate depressions in the equivalent location, interpreted as fossae for large mandibular adductor muscles (see Tokita et al. Citation2013; Carril et al. Citation2015). Other cacatuids and psittaculids bear a short, shallow depression confined to the area immediately posterior to the orbit, and the latero-posterior neurocranium is essentially convex and smooth. If the sulcus in the P. occidentalis holotype is not pathological or the result of damage, it could be the insertion site for an enlarged right mandibular adductor muscle (Carril et al. Citation2015).
Otic region
There is also marked asymmetry of the left and right ears. Immediately posterior to the right ear opening, the exoccipital bulges laterally in posterior, dorsal and anterior views while the left does not (see ). In anterior view, the rim of the right ear opening arches so far laterally that it protrudes beyond the arcus suborbitalis, whereas the flatter opening of the left ear is completely obscured. Similarly, in dorsal view, the rim of the right ear opening arches laterally and is medially recurved, forming a pocket that is visible in dorsal view, while the flatter left rim cannot be seen in this view and no pocket is visible (). Ear symmetry of QM O.29055 could not be determined due to damage to its left side. However, the rim of its intact right ear arches laterally as in the holotype.
Despite the latero-medial asymmetry of the ears, antero-posterior placement of their openings appears equal. As in all psittaculids, the openings are narrower and placed markedly further anteriorly than in members of the Cacatuidae, Nestoriidae and Strigopidae, which have large, round, laterally-directed ear openings near the posterior of the skull. However, in P. occidentalis they are positioned considerably further anteriorly than in any other member of the Psittaculidae, sitting about one-third of the way along the skull. They are also more dorso-ventrally elongate and placed higher on the skull than in other psittaculids, including other pezoporine species in which the dorsal-most point of the ear opening is at most level with, or below, the level of the processus zygomaticus (). The ear openings are a simple, straight slit entirely confined to the lateral surfaces of the cranium, and unlike all other psittaculine species examined, do not extend under the ventral surface of the skull. Therefore, uniquely to P. occidentalis, both tympanic cavities are completely concealed in lateral or ventral views. The angle at which the tympanic cavities open differs slightly between left and right. In right lateral view, the rim of the rim of the right ear is about parallel with the anterior margin of the processus zygomaticus (). By contrast, in left lateral view, the left rim diverges from the anterior rim of the processus zygomaticus by approximately 15° (), more closely approaching the rim of the orbit at its ventral end than does its right counterpart: the gap between these structures is roughly half that on the right side. This effectively means that the laterally-arched right ear is orientated more forwards, while the flatter left ear tilts slightly upwards.
A narrow strut of bone connects the base of the exoccipital to the posterior rim of the orbit, bridging the gap between the otic rim and the arcus suborbitalis where they approach most closely, effectively enclosing the quadrate. Measuring diagonally from the ventral end of this strut of bone to the ventral-most point of the exoccipital, so avoiding measuring the damaged posterior region of the right exoccipital, the left exoccipital (9.2 mm) is slightly larger than the right (8.6 mm). Similarly, measuring from the ventral-most point of the exoccipitals to the dorsal-most point of the ear slits, the left dimension (13 mm) is slightly greater than the right (12 mm). See Supp. Info. for a figure illustrating where measurements were taken. Internal volumes of the left and right tympanic cavities could not be compared due to the damaged right-posterior cranial wall, but these linear differences suggest there may be volumetric differences.
Orbital region
The orbit of the P. occidentalis holotype is relatively small compared to other pezoporine taxa (), occupying less than half the length of the cranium, excluding the rostrum. The right orbit is 12.8 mm in diameter (similar to QM O.29055, 11.7 mm; Iwaniuk et al. Citation2020) and 12.3 mm high. Compared to other pezoporines, orbit size in P. occidentalis is constrained by elevation of the processus zygomaticus, which is concomitant with the enlarged exoccipitals and associated elevation of the ear openings ().
Atypically for a psittaculid, the orbit in P. occidentalis is completely enclosed ventrally by a robust, completely ossified arcus suborbitalis () (see Carril et al. Citation2015). In this species it incorporates the processus preorbitalis anteriorly and the processus zygomaticus of the squamosal posteriorly. In QM O.29055, the better-preserved right arcus suborbitalis is complete, but with a distinct suture visible between the processus zygomaticus and processus postorbitalis. This may be due to incomplete ossification in a younger individual; the damaged left arcus suborbitalis is fractured at this point, demonstrating the weakness of the suture in that specimen. A complete arcus suborbitalis, while common to all cacatuid species examined, was seen in only two other pstittaculids: Melopsittacus undulatus (Melopsittacini) and Cyclopsitta diophthalma (Loriini). In all other psittaculids, the processus preorbitalis and the processus zygomaticus approach each other, but do not touch or fuse (e.g. ), with the space between these bones presumably bridged by connective tissue in vivo.
The composition of the arcus suborbitalis in P. occidentalis differs from all examined taxa in which it is completely ossified, by incorporating the zygomatic process but not the processus postorbitalis posteriorly. In some taxa, only the processus postorbitalis is incorporated posteriorly, and the processus zygomaticus is free, e.g. Strigops habroptila (Strigopidae); Zanda funerea and Probosciger aterrimus (Cacatuidae); Cyclopsitta diophthalma (Psittaculidae). More commonly, both the processus postorbitalis and the processus zygomaticus are incorporated posteriorly, e.g. Nymphicus hollandicus, Callocephalon fimbriatum, Eolophus roseicapilla, Lophochroa leadbeateri and Cacatua galerita (Cacatuidae); and Melopsittacus undulatus (Loriinae). Although the complete arcus suborbitalis of P. occidentalis mimics that of species of cacatuid, and the psittaculids Melopsittacus and Cyclopsitta, its structural composition is more similar to the incomplete sub-orbital structures seen in most members of the Psittaculidae: in nearly all psittaculids, the processus preorbitalis and processus zygomaticus approach each other beneath the orbit, but are not fused. Neophema splendida is the pezoporine with the most similar sub-orbital structure to P. occidentalis, although the processes are not fused. In P. wallicus, the processus preorbitalis is very thin and delicate, unlike the robust process of P. occidentalis and N. splendida (see ). Anteriorly, a deep and broad fossa nasolacrymalis punctuates the processus preorbitalis ().
The optic foramen of the holotype, inside the orbital cavity near the cranial midline, has an area of 1.9 mm2, slightly smaller than in QM O.29055 (2.5 mm2; Iwaniuk et al. Citation2020).
The frontals are relatively broad (9.8 mm at their narrowest point), and are marked by a continuation of the sagittal depression of the neurocranium, lending them a V-shaped anterior margin in dorsal view (). Their breadth means that they overhang the orbits significantly. The frontals and lacrimals flare widely laterally, just posterior to the rostrum (). Proportions of the frontals conform with most pezoporine taxa, but are most similar to those of the species of Neophema (). The condition differs markedly from that of P. wallicus, in which the frontals are about half the width (5.8 mm, ANWC 23818) and constricted above the orbits. At their narrowest point, the frontals of P. occidentalis are proportionally narrower than in Neopsephotus bourkii (), Strigops habroptila and members of the Cacatuidae.
The orbits retain an ossified annulus ossicularis sclerae (scleral ring, often termed ‘sclerotic ring’; see Franz-Odendaal Citation2020) (https://doi.org/10.17602/M2/M434572). The better-preserved right ring is 9.3 mm wide and 9.7 mm high (external), and 6.9 wide and 6.7 mm high (internal). Fragments of a ring are retained in the left orbit of the published CT mesh model of P. occidentalis specimen QM O.29055. The incompleteness in that specimen may be due to an incompletely ossified young individual resulting in poor visibility on CT imagery due to thresholding, or it may have been manually removed to more clearly visualise the orbit.
Comparison with scleral ring measurements published by Choiniere et al. (Citation2021) show that the relative dimensions of the internal and external diameters in P. occidentalis are unremarkable among nocturnal birds, although in absolute size it has the third smallest scleral ring of any nocturnal bird (). However, compared to other parrots with smaller or comparably sized scleral rings, P. occidentalis has a wider internal diameter than would be expected for its size (), indicating a relatively large cornea (see Hall Citation2008).
Figure 3. Comparison of scleral ring internal and external diameter of the Night Parrot P. occidentalis holotype (solid square) with other parrots (open squares) and other nocturnal birds (crosses) (data for other species from Choiniere et al. Citation2021). Ordinary least squares regression; Slope = 0.56, intercept = 1.32. See Supp. Info. for taxon list.
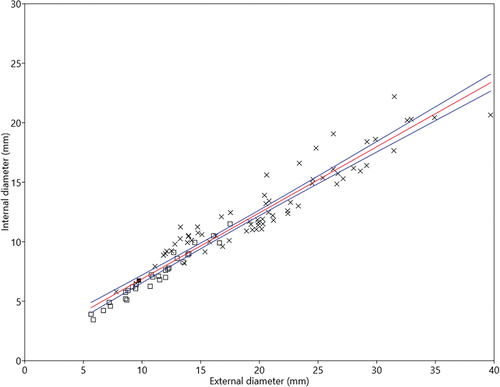
Rostrum & mandible
Typical of all psittaculids besides the Psittaculini, P. occidentalis has a small rostrum (https://doi.org/10.17602/M2/M434569) and mandible (https://doi.org/10.17602/M2/M434554) relative to cranial size. The bony rostrum (without the rhamphotheca) is narrow and antero-posteriorly flattened, as in other pezoporines. The tip is broad and rounded. The bony nares are very large and elongate and occupy about half of the dorso-ventral height of the rostrum (), proportionally more than in nearly all compared taxa, including P. wallicus (about one-third of rostral height). Only Nymphicus hollandicus (Cacatuidae) and Neophema petrophila (Pezoporini) have comparably-sized nares. Their large size in P. occidentalis is not apparent in the holotype skin because they are thickly covered by the bulging, fleshy nostrils noted as one of the defining features of the species (see Gould Citation1861, Citation1865). The nares are separated by a very thin and delicate os nasale. In anterior view, the pars symphysialis of the mandible is deep and U-shaped, and is as deep as it is wide. The left and right rami of the mandible diverge at about 40°.
Quadrate & palatal elements
The articulated quadrates (https://doi.org/10.17602/M2/M434566) are partly obscured in lateral view by the mandible and the strut of bone connecting the otic rim to the arcus suborbitalis (). When segmented from the CT data (), their shape is revealed as dorso-ventrally elongate, with a long, thin processus orbitalis quadrati that is longer than the processus oticalis and orientated vertically. Elongation of the processus orbitalis quadrati is shared with some pezoporines (e.g. Neophema elegans, N. chrysostoma, N. petrophila), but not others (e.g. P. wallicus, Neopsephotus bourkii, Neophema splendida). The processus orbitalis quadrati has a single articular head, as in other pezoporines and a few non-pezoporine species (Alisterus scapularis, Aprosmictus erythropterus, Polytelis alexandrae in the Polytelini, and Trichoglossus moluccanus in the Loriini). As in most compared taxa, a large pneumatic foramen perforates the proximo-medial surface of the processus orbitalis quadrati. Although left and right quadrates seem identical, there appear to be differences in the way they articulate with the cranium, relating to the asymmetries of the neurocranium and ears. The head of the left processus orbitalis quadrati meets an obvious facet in the squamosal bone posterior to the arcus suborbitalis, while the right appears to articulate with the squamosal bone mesad of the arcus suborbitalis, and is partially obscured by a sheet of bone that forms the medial wall of the tympanic cavity.
Figure 4. Palatal bones of the P. occidentalis holotype (NHMUK.1868.1.27.30). a, Palate (https://doi.org/10.17602/M2/M434563), hyoid (https://doi.org/10.17602/M2/M434557), and jugal bars (https://doi.org/10.17602/M2/M434560) in dorsal view; b, Left and right quadrates (https://doi.org/10.17602/M2/M434566) in anterior view; c, Left and right quadrates in posterior view; d, left quadrate in medial view.
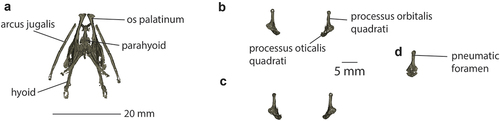
With the beak closed, the arcus jugalis articulates with the quadrate about antero-posteriorly level with the articular head of the processus orbitalis quadrati and runs beneath the arcus suborbitalis. The palatines (https://doi.org/10.17602/M2/M434563) are flat and plate-like posteriorly, and elongate and narrow anteriorly. The parahyoid (https://doi.org/10.17602/M2/M434557) is approximately diamond-shaped with anterior and posterior processes of about equal length, and in ventral view a narrow ridge extends from just posterior of the anterior process onto the ventral side of the posterior process ().
Discussion
Here, we consider the potential adaptive significance of some unique cranial features of P. occidentalis, and implications for conservation.
Orbits, vision & feeding
Both P. occidentalis and S. habroptila have a complete arcus suborbitalis enclosing the orbit, but despite proximity to the eye this is unlikely to represent a nocturnal visual adaptation in either species. This structure has evolved independently across multiple, mainly diurnal, parrot lineages (Carril et al. Citation2015), and does not necessarily correlate well with eye size or relative development of the visual system (Tokita et al. Citation2007). Rather, it serves as the upper attachment site for the pseudomasseter, a jaw-closing muscle unique to the Psittaciformes that is functionally analogous to the masseter muscle of mammals, and facilitates a powerful bite force without distorting the eye (Tokita et al. Citation2013). However, P. occidentalis is reported to inflict a particularly weak bite when handled (pers. comm., S.A. Murphy and A.H. Burbidge, 30 June 2022), so its jaw biomechanics and feeding adaptations, and function of the particularly robust arcus suborbitalis, remain to be investigated. If the unusual, right-sided sulcus on the cranial vault of the holotype is functional and not pathological, and is an attachment site for enlarged jaw musculature, it may indicate an asymmetrical, lateral motion of the jaws.
The complete, robust arcus suborbitalis is likely to negatively influence vision in P. occidentalis by constraining the maximum size of the eye: enlarged exoccipitals in this species displace the zygomatic processes upwards and forwards compared to other pezoporines (), producing relatively smaller orbits. This likely represents an evolutionary trade-off between hearing and vision. Absolute orbit size in P. occidentalis is not particularly small for an Australian psittaculine parrot, but the eye is smaller than in P. wallicus, and considerably smaller relative to inferred brain volume (Iwaniuk et al. Citation2020).
Ossified scleral rings are highly exaggerated in some nocturnal birds, but they are also present in diurnal birds, so any functional role related to night vision remains speculative (Franz-Odendaal Citation2020). In P. occidentalis, internal diameter of the scleral ring is greater than in most parrots, and wider than in many nocturnal birds relative to its external diameter, despite small absolute size (). Tight correlation between scleral ring internal diameter and cornea diameter (Hall Citation2008) indicates that P. occidentalis likely has an enlarged cornea relative to overall eye size. This would maximise the amount of light entering the eye, and increase nocturnal visual sensitivity, though not acuity (Hall and Ross Citation2007). Assuming corneal diameter approximates scleral ring internal dimensions (6.9 mm), cornea size would closely match those of the smallest volant nocturnal birds, hypothesised to be at the lower limits of size allowing visually-dependent nocturnal flight (Hall and Ross Citation2007).
The functional significance of the sagittal depression in the Night Parrot cranium requires consideration in relation to vision. It may relate to enlarged wulsts in the posterior brain, which in some species are correlated with binocular/stereoscopic vision, convergent orbits, and better nocturnal vision (Corfield et al. Citation2011; Wylie et al. Citation2015). Although P. occidentalis has larger wulsts than diurnal P. wallicus, in which they barely protrude from the underlying telencephalon (see Supp. Info.) and which also lacks a sagittal depression, there is no other indication that P. occidentalis could have enhanced nocturnal vision related to stereopsis. We did not quantify eye orientation, but P. occidentalis does not appear to have orbits that are any more forward-facing than other pezoporines (see ), unlike Strigops habroptila (Corfield et al. Citation2011), and so the function of its enlarged wulsts is unclear.
If P. occidentalis lacks enhanced stereoscopic vision, then the wulsts may have been co-opted for the processing of stimuli from senses other than vision, such as touch from rictal bristles (see Wylie et al. Citation2015), or from the oral cavity. Murie (Citation1868) noted that the tongue of P. occidentalis bore unusual longitudinal ridges arranged in a fan, and that two enlarged organs in the mouth could either be the parotid (i.e. salivary) glands or tactile organs.
Hearing
Nocturnal calling plays a major communication role in this species, and listening surveys have proved successful in detecting Night Parrot populations (e.g. Jackett et al. Citation2017; WWF Australia Citation2017; Leseberg et al. Citation2020). However, nothing is known about its acoustic sensory ecology. The uniquely enlarged exoccipitals, which occupy more of the skull than in any other parrot species examined, may act as resonance chambers, amplifying the volume of sound transmitted to the inner ears, while asymmetries of the ear regions suggest that directional hearing may be more important than previously realised. Asymmetrical ears characterise some bird taxa, especially nocturnal ones, but this has not previously been noted in any species of parrot. In many species of owl, the left and right ears are markedly offset dorso-ventrally and antero-posteriorly, producing a dramatically distorted cranium (e.g. Norberg Citation1977, Citation1978; Gutiérrez-Ibáñez et al. Citation2011). This assists owls to pinpoint prey in a vertical plane while in flight, because of the difference in time the sound waves take to reach the left and right ears (Norberg Citation1978). The degree of ear asymmetry in P. occidentalis is less exaggerated than in owls, but may nevertheless enhance their ability to locate sound compared to other parrots. Unlike the dorso-ventral asymmetry of owl ears, in P. occidentalis the main asymmetries are latero-medial, with the right ear opening arching to the side, the left being more or less flat. This may assist this ground-dwelling species to pinpoint sound in the horizontal plane, whether from conspecifics or from predators. Slight differences in the length and angle of the ear slits, which result in the right ear facing forwards and the left tilting slightly upwards, may also assist with pinpointing sound in the vertical plane. Crucially, in this ground-dwelling bird, the ears appear optimised to collect sound at ground level rather than on the wing, as the ear slits face forwards and upwards, but not downwards ().
The specialised ear anatomy of the P. occidentalis holotype contrasts with apparently unremarkable anatomy of the diurnal P. wallicus and nocturnal S. habroptila. Enhanced directional hearing could help explain the extreme elusiveness of the Night Parrot, which may be very efficient at detecting the direction of approaching humans. Secretiveness has long been recognised as its defining quality: a traditional Dreaming story of the Martu people from the Western Deserts region narrates the Night Parrot’s refusal to associate with other animals, evading all attempts at capture (as told by Jeffrey Stewart-Ullala Boss, translated by Rita Cutter, cited in Jones Citation2019).
Noise pollution is not specifically identified as a threat to the Night Parrot (BirdLife International Citation2022), but in light of the likely importance of acoustics to this Critically Endangered species, and its known use of relatively low-frequency calls of around 2 kHz (Jacket et al. Citation2017; Leseberg et al. Citation2019), similar to frequencies used by species known to be excluded by noise pollution (Francis et al. Citation2011), noise disturbance in known or potential Night Parrot habitat should be minimised as a precaution, until more is learnt about its acoustic ecology.
Conclusions
The few similarities between the crania of the Night Parrot and Kakapo suggest that they solved the challenges of nocturnal life in very different ways. Other sensory capabilities of the Night Parrot remain to be investigated, but our study sheds light on its visual and auditory ecology. A relatively large scleral ring, and therefore cornea, could partly compensate for small eye size, but overall, nocturnal vision has likely been traded off against hearing specialisations. Its acoustic ecology, including relevant neuroanatomy, should be further investigated, and may lead to better understanding of how Night Parrots interact with each other and their environment.
Surprisingly, and despite similarities in external morphology, ground-dwelling lifestyle and largely granivorous diet, the cranial morphology of the Night Parrot is no more similar to its congener P. wallicus than to species of Neophema or Neopsephotus. This lends support to the view that the Night Parrot could reasonably remain in Geopsittacus Gould, Citation1861, but reversion to Geopsittacus would require additional supporting evidence from the postcranial skeleton and genetic data.
Supplemental Material
Download MS Word (619.1 KB)Acknowledgments
Thanks to: Vincent Fernandez, Mark Adams and Hein Van Grouw of the UK’s Natural History Museum for information about the holotype and for CT-scanning the specimen under challenging pandemic circumstances; Maya Penck, Philippa Horton and Ben Parslow for access and technical assistance at the South Australian Museum; Leo Joseph and Chris Wilson at ANWC for facilitating specimen loans; and colleagues in the Flinders Palaeontology Lab and Weisbecker Lab for their support and interest. We also thank Steve Murphy, two anonymous reviewers, and the editors for outstandingly constructive criticism.
Disclosure statement
No potential conflict of interest was reported by the authors.
Data availability statement
Image data and 3D models are available via MorphoSource.org, including the following segmented models derived from CT data: entire skull and jaw (https://doi.org/10.17602/M2/M488551); cranium (https://doi.org/10.17602/M2/M434579); rostrum (https://doi.org/10.17602/M2/M434569); mandible (https://doi.org/10.17602/M2/M434554); jugal (https://doi.org/10.17602/M2/M434560); palatal elements (https://doi.org/10.17602/M2/M434563); quadrate bones (https://doi.org/10.17602/M2/M434566); scleral rings (https://doi.org/10.17602/M2/M434572); and hyoid (https://doi.org/10.17602/M2/M434557).
Supplementary material
Supplemental data for this article can be accessed at https://doi.org/10.1080/01584197.2023.2181185.
Additional information
Funding
References
- Baumel, J., King, A., Breazile, J., Evans, H., and Vanden Berge, J. (1993). ‘Handbook of Avian Anatomy: Nomina Anatomica Avium,’ 2nd edn. (Nuttall Ornithology Club: Cambridge, Massachusetts.)
- BirdLife International. (2022). ‘Pezoporus occidentalis’. pp. e.T22685237A211825128. (The IUCN Red List of Threatened Species.) doi:10.2305/IUCN.UK.2022-1.RLTS.T22685237A211825128.en
- Boles, W. E., Longmore, N. W., and Thompson, M. C. (1994). A recent specimen of the Night Parrot, Geopsittacus occidentalis. Emu 94(1), 37–40.
- Carril, J., Degrange, F. J., and Tambussi, C. P. (2015). Jaw myology and bite force of the monk parakeet (Aves, Psittaciformes). Journal of Anatomy 227(1), 34–44. doi:10.1111/joa.12330
- Choiniere, J. N., Neenan, J. M., Schmitz, L., Ford, D. P., Chapelle, K. E. J., Balanoff, A. M., Sipla, J. S., et al. (2021). Evolution of vision and hearing modalities in theropod dinosaurs. Science 372(6542), 610–613. doi:10.1126/science.abe7941
- Corfield, J. R., Gsell, A. C., Brunton, D., Heesy, C. P., Hall, M. I., Acosta, M. L., and Iwaniuk, A. N. (2011). Anatomical specializations for nocturnality in a critically endangered parrot, the Kakapo (Strigops habroptila). PLoS ONE 6(8), e22945. doi:10.1371/journal.pone.0022945
- DCCEEW. (2022). ‘Threatened Species Action Plan 2022–2032 – Towards Zero Extinctions.’ (Department of Climate Change, Energy, the Environment and Water, Canberra). Available at https://www.dcceew.gov.au/environment/biodiversity/threatened/publications/action-plan-2022-2032
- Finsch, O. (1867). (122.). 2. Pezoporus occidentalis, (Gould). - Der kurzzehige Erdsittich. In ‘Die Papageien, monographisch bearbeit’. pp. 151–155. (E.J. Brill: Leiden.)
- Francis, C. D., Ortega, C. P., Cruz, A., Rands, S. A., and Rands, S. A. (2011). Noise pollution filters bird communities based on vocal frequency. PLoS ONE 6(11), e27052. doi:10.1371/journal.pone.0027052
- Franz-Odendaal, T. A. (2020). Skeletons of the eye: An evolutionary and developmental perspective. The Anatomical Record 303(1), 100–109. doi:10.1002/ar.24043
- Gould, J. (1861). On a new genus and species of parrakeet from Western Australia. Proceedings of the Zoological Society of London 29, 87–101. doi:10.1111/j.1469-7998.1861.tb00374.x
- Gould, J. (1865). Genus Pezoporus, Illiger. ‘Handbook to the Birds of Australia: Vol. II. pp. 86–89. (London). https://www.biodiversitylibrary.org/item/49435#page/9/mode/1up [Verified 23 October 2018].
- Gutiérrez-Ibáñez, C., Iwaniuk, A. N., and Wylie, D. R. (2011). Relative size of auditory pathways in symmetrically and asymmetrically eared owls. Brain, Behavior and Evolution 78(4), 286–301. doi:10.1159/000330359
- Hagelin, J. C. (2004). Observations on the olfactory ability of the Kakapo Strigops habroptilus, the critically endangered parrot of New Zealand. Ibis 146, 161–164. doi:10.1111/j.1474-919X.2004.00212.x
- Hall, M. I. (2008). The anatomical relationships between the avian eye, orbit and sclerotic ring: Implications for inferring activity patterns in extinct birds. Journal of Anatomy 212(6), 781–794. doi:10.1111/j.1469-7580.2008.00897.x
- Hall, M. I., and Ross, C. F. (2007). Eye shape and activity pattern in birds. Journal of Zoology 271(4), 437–444. doi:10.1111/j.1469-7998.2006.00227.x
- Hamilton, N. A., Onus, M., Withnell, B., and Withnell, K. (2017). Recent sightings of the Night Parrot Pezoporus occidentalis from Matuwa (Lorna Glen) and Millrose Station in Western Australia. Australian Field Ornithology 34, 71.
- Hammer, Ø., Harper, D. A. T., and Ryan, P. D. (2001). PAST: Paleontological statistics software package for education and data analysis. Palaeontologia Electronica 4(1), 1–9.
- Horton, P., Black, A., Reid, J., and McAllan, I. (2021). Records of the Night Parrot Pezoporus occidentalis in South Australia, including its ‘rediscovery’ in 1979 and a review of its habitat use. South Australian Ornithologist 45(2), 85–117.
- Iwaniuk, A. N., Keirnan, A. R., Janetzki, H., Mardon, K., Murphy, S., Leseberg, N. P., and Weisbecker, V. (2020). The endocast of the Night Parrot (Pezoporus occidentalis) reveals insights into its sensory ecology and the evolution of nocturnality in birds. Scientific Reports 10(1). doi:10.1038/s41598-020-65156-0
- Jackett, N., Greatwich, B., Swann, G., and Boyle, A. (2017). A nesting record and vocalisations of the Night Parrot Pezoporus occidentalis from the East Murchison, Western Australia. Australian Field Ornithology 34, 144–150. doi:10.20938/afo34144150
- Jones, A. (2019, February 5). ‘Back from the dead (No. 2).’ Science on the Run. (ABC). Available at https://www.abc.net.au/radio/programs/the-chase/the-chase-back-from-the-dead/10758038
- Joseph, L., Toon, A., Schirtzinger, E. E., and Wright, T. F. (2011). Molecular systematics of two enigmatic genera Psittacella and Pezoporus illuminate the ecological radiation of Australo-Papuan parrots (Aves: Psittaciformes). Molecular Phylogenetics and Evolution 59(3), 675–684. doi:10.1016/j.ympev.2011.03.017
- Joseph, L., Toon, A., Schirtzinger, E. E., Wright, T. F., and Schodde, R. (2012). A revised nomenclature and classification for family-group taxa of parrots (Psittaciformes). Zootaxa 3205, 26–40. doi:10.11646/zootaxa.3205.1.2
- Leseberg, N. P., McAllan, I. A. W., Murphy, S. A., Burbidge, A. H., Joseph, L., Parker, S. A., Jackett, N. A., et al. (2021). Using anecdotal reports to clarify the distribution and status of a near mythical species: Australia’s Night Parrot (Pezoporus occidentalis). Emu - Austral Ornithology 121(3), 239–249. doi:10.1080/01584197.2021.1927760
- Leseberg, N. P., Murphy, S. A., Jackett, N. A., Greatwich, B. R., Brown, J., Hamilton, N., Joseph, L., et al. (2019). Descriptions of known vocalisations of the Night Parrot Pezoporus occidentalis. Australian Field Ornithology 36, 79–88. doi:10.20938/afo36079088
- Leseberg, N. P., Venables, W. N., Murphy, S. A., Watson, J. E. M., and Zamora-Gutierrez, V. (2020). Using intrinsic and contextual information associated with automated signal detections to improve call recognizer performance: A case study using the cryptic and critically endangered Night Parrot Pezoporus occidentalis. Methods in Ecology and Evolution 11, 1520–1530. doi:10.1111/2041-210X.13475
- Mayr, G., and Manegold, A. (2020). On the comparative morphology of the juvenile avian skull: An assessment of squamosal shape across avian higher-level taxa. The Anatomical Record 304(4), 845–859. doi:10.1002/ar.24504
- McDougall, A., Porter, G., Mostert, M., Cupitt, R., Cupitt, S., Joseph, L., Murphy, S., et al. (2009). Another piece in an Australian ornithological puzzle – A second Night Parrot is found dead in Queensland. Emu - Austral Ornithology 109(3), 198. doi:10.1071/MU08018
- Murie, J. (1868). On the nocturnal Ground-Parrakeet (Geopsittacus occidentalis, Gould). Proceedings of the Zoological Society of London 27, 158–165.
- Murphy, S. A., Austin, J. J., Murphy, R. K., Silcock, J., Joseph, L., Garnett, S. T., Leseberg, N. P., et al. (2017a). Observations on breeding Night Parrots (Pezoporus occidentalis) in western Queensland. Emu - Austral Ornithology 117(2), 107–113. doi:10.1080/01584197.2017.1292404
- Murphy, S. A., Joseph, L., Burbidge, A. H., and Austin, J. (2011). A cryptic and critically endangered species revealed by mitochondrial DNA analyses: The Western Ground Parrot. Conservation Genetics 12(2), 595–600. doi:10.1007/s10592-010-0161-1
- Murphy, S. A., Silcock, J., Murphy, R., Reid, J., and Austin, J. J. (2017b). Movements and habitat use of the Night Parrot Pezoporus occidentalis in south-western Queensland. Austral Ecology 42(7), 858–868. doi:10.1111/aec.12508
- Norberg, R. A. (1977). Occurrence and independent evolution of bilateral ear asymmetry in owls and implications on owl taxonomy. Philosophical Transactions of the Royal Society B: Biological Sciences 280(973), 375–408.
- Norberg, R. A. (1978). Skull asymmetry, ear structure and function, and auditory localization in Tengmalm’s Owl, Aegolius funereus (Linné). Philosophical Transactions of the Royal Society B: Biological Sciences 282(991), 325–410.
- Olsen, P. (2018). ‘Night Parrot.’ (CSIRO Publishing: Victoria.)
- Olsen, P., and Menkhorst, P. (2020). A recent investigation highlights the importance of honesty in ornithology and conservation. Emu - Austral Ornithology 120(2), 178–180. doi:10.1080/01584197.2020.1758570
- Pyke, G., and Ehrlich, P. (2014). Conservation and the Holy Grail: The story of the Night Parrot. Pacific Conservation Biology 20(2), 221–226. doi:10.1071/PC140221
- Thompson, D. W. (1899). On characteristic points in the cranial osteology of the parrots. Proceedings of the Zoological Society of London 67(1), 9–46. doi:10.1111/j.1469-7998.1899.tb06842.x
- Tokita, M., Kiyoshi, T., and Armstrong, K. N. (2007). Evolution of craniofacial novelty in parrots through developmental modularity and heterochrony. Evolution & Development 9(6), 590–601. doi:10.1111/j.1525-142X.2007.00199.x
- Tokita, M., Nakayama, T., Schneider, R. A., and Agata, K. (2013). Molecular and cellular changes associated with the evolution of novel jaw muscles in parrots. Proceedings of the Royal Society B: Biological Sciences 280(1752), 20122319. doi:10.1098/rspb.2012.2319
- Wilson, H. (1937). Notes on the Night Parrot, with references to recent occurrences. Emu - Austral Ornithology 37(2), 79–87. doi:10.1071/MU937079
- WWF Australia. (2017, October 13). ‘Paruku rangers’ night parrot discovery.’ Available at https://www.wwf.org.au/news/news/2017/paruku-rangers-night-parrot-discovery [Verified 29 November 2022].
- Wylie, D. R., Gutiérrez-Ibáñez, C., and Iwaniuk, A. N. (2015). Integrating brain, behavior, and phylogeny to understand the evolution of sensory systems in birds. Frontiers in Neuroscience 9, doi:10.3389/fnins.2015.00281