ABSTRACT
The Plains-wanderer (Pedionomidae: Pedionomus torquatus) is a species of Australian shorebird that exclusively inhabits open grasslands. The reported presence of this species in the fossil deposits of Naracoorte Caves suggests that it once inhabited a wooded environment. It was therefore important to assess whether the fossils are indeed conspecific with P. torquatus before inferences on the past palaeoecological preferences of the species are made. This study reassessed the identity of fossils identified as P. torquatus using both qualitative observations and morphometric analyses to test for proportional differences of fossil specimens which might indicate taxonomic distinction. Measurements were collected from fossils attributed to Pedionomus from Blanche Cave (45–15 ka) and Victoria Fossil Cave (>400–220 ka), and compared with modern skeletal specimens through univariate and principal component analyses. Fossil elements were significantly larger than the modern specimens, although marked overlap was consistently observed, precluding unambiguous differentiation on size. No qualitative traits distinguishing the two groups could be identified. The Naracoorte fossil Pedionomus are therefore considered to represent a population of P. torquatus, wherein birds were slightly larger than extant ones. The Naracoorte deposits sampled a forest and woodland-dominated environment over the last 400,000 years, one which would be deemed unsuitable for modern Plains-wanderers, indicating that P. torquatus may have formerly occupied a much broader range of habitats than its present distribution suggests. It is inferred that the Plains-wanderer underwent a marked contraction in occupied habitat during the Holocene.
Introduction
Pedionomus torquatus (Plains-wanderer) is a small species of shorebird (Charadriiformes) endemic to central and eastern Australia. It is classified as critically endangered under the Environmental Protection and Biodiversity Conservation Act 1999 (Department of the Environment Citation2015), is one of the most evolutionarily distinct birds in Australia (Jetz et al. Citation2014), and is listed among the 20 Australian endemic birds most likely to become extinct in the next 20 years (Geyle et al. Citation2018). Initially viewed as closely allied with Turnicidae (buttonquails) (Gadow Citation1891; Bock and McEvey Citation1969), it has more recently been reassessed as a member of Scolopaci most closely related to Thinocoridae (seedsnipes) among extant taxa (Olson and Steadman Citation1981), as confirmed by numerous molecular studies (Van Tuinen et al. Citation2004; Baker et al. Citation2007; Gibson and Baker Citation2012; Kuhl et al. Citation2021). Pedionomus torquatus is the sole extant representative of Pedionomidae, a group which also includes a single extinct species (De Pietri et al. Citation2015) – Oligonomus milleri. A second fossil relative of the group has also been discovered from the Miocene of New Zealand (De Pietri et al. Citation2016).
Historical sightings of P. torquatus have been recorded in Victoria, New South Wales, South Australia, and Queensland (see Baker-Gabb Citation1990; Forshaw and Knight Citation2023). The taxon has undergone a range contraction in recent decades and persists primarily in strongholds in the Riverina of south-western New South Wales and north-central Victoria (Bennett Citation1983; Baker-Gabb et al. Citation1990). The species now exclusively inhabits native grassland environments devoid of woodland (Harrington et al. Citation1988; Baker-Gabb et al. Citation1990; Marchant and Higgins Citation1993; Baker‐Gabb et al. Citation2016; Antos and Schultz Citation2020; Forshaw and Knight Citation2023) – depending on open grasslands with low grass cover to roost, and higher grass cover for foraging (Antos and Schultz Citation2020; Nugent et al. Citation2022). Pedionomus torquatus has also been shown to avoid areas with high levels of litter cover, or invasive flora (Nugent et al. Citation2022). Increased density of grassland environments due to inter-annual fluctuations in precipitation have previously caused significant declines in P. torquatus populations (Baker‐Gabb et al. Citation2016).
Like Pedionomus, thinocorids are a group of terrestrial shorebirds adapted primarily to open environments (Fjeldså Citation1996). The close relationship between these taxa has led to the suggestion that such a lifestyle is ancestral to the two groups (Fjeldså Citation1996). This, however, is contradicted by the fossil record: Hakawai melvillei – the putative sister taxon to Pedionomidae – shows adaptations for wading likely ancestral to Charadriiformes (De Pietri et al. Citation2016), while the only other known fossil species of pedionomid was derived from a wooded environment (De Pietri et al. Citation2015). This indicates that the specialisation to grasslands of P. torquatus is a more recent adaptation, possibly arising during the Miocene or Pliocene (De Pietri et al. Citation2015, Citation2016).
Reports of fossils of Pedionomus are few, limited to a single element from the Nullarbor Plain (Shute Citation2019), a small number of isolated specimens from across Victoria (Vickers Rich and McEvey Citation1980; Baird Citation1991), and specimens from the Naracoorte Caves World Heritage Area and nearby deposits in south-eastern South Australia (Van Tets and Smith Citation1974; Reed and Bourne Citation2000, Citation2009). The latter occurrence is perplexing in light of the apparent habitat sensitivity of P. torquatus. Indeed, palaeoecological reconstructions of the Naracoorte area have often interpreted it as a heterogeneous environment dominated by open forest and woodland (e.g. Brown and Wells Citation2000; Fraser and Wells Citation2006), which would be inconsistent with the presence of an obligate grassland specialist such as P. torquatus. This calls into question the identification of the Naracoorte fossil pedionomids as P. torquatus, and indeed possible size differences with modern individuals suggest these may represent a distinct taxon (Laslett Citation2006). This study therefore seeks to address whether the Naracoorte fossil Pedionomus may be distinguished from modern P. torquatus at the species level by quantitatively comparing fossil appendicular elements attributed to Pedionomus from Blanche Cave and Victoria Fossil Cave with those of modern specimens of P. torquatus.
Methods
Data collection
Fossil bones of Pedionomus from the Main Fossil Chamber excavation, Victoria Fossil Cave (VFC) were identified via comparison to a reference specimen of Pedionomus torquatus (SAMA B45368) and illustrations from Bock and McEvey (Citation1969). The identity of additional Pedionomus specimens from Blanche Cave housed at the South Australian Museum were verified using the same reference specimen. A total of 39 specimens from VFC and 105 specimens from Blanche Cave were sampled (see Table S1).
Measurements of all humeri, ulnae, carpometacarpi, coracoids, femora, tibiotarsi, and tarsometatarsi (see ) from this sample were collected using digital callipers (resolution = 0.01 mm, rounded to the nearest 0.05 mm). Specimens were discarded from the dataset if at least two measurements could not be collected.
Table 1. A list of measurements collected in this study.
Measurements were also collected from all available modern Pedionomus torquatus skeletal specimens housed at the South Australian Museum, Australian Museum, and Museums Victoria. A total of 23 specimens were sampled, although some elements were not represented in all specimens. One juvenile specimen (SAMA B47080) – as evidenced by incomplete ossification and pitted bone surface – was subsequently removed from the dataset. Additionally, comparative measurements were collected from two species of Charadriidae: one skeleton of the Double-banded Plover (Charadrius bicinctus) and three of the Red-kneed Dotterel (Erythrogonys cinctus). See Table S2 for further details.
Abbreviations
Institutions: AM – Australian Museum, Sydney, New South Wales; NMV – Museums Victoria, Melbourne, Victoria; SAMA – South Australian Museum, Adelaide, South Australia
Data analysis
All statistical analyses were conducted in R software (R Core Team Citation2022). Summary statistics were calculated for each variable using the package pastecs (Grosjean and Ibanez Citation2018), and Shapiro–Wilk tests were used to assess the normality of these variables. Welch’s t-tests were then conducted to test for significant difference in mean values between fossil and modern Pedionomus where data were found to adhere to normality, and Mann–Whitney U tests were used where data was found to significantly deviate from normality.
Individual principal component analyses (PCA) were conducted for each element from all usable specimens after log10 transformation of all values. Imputation of missing values was carried out using the package mice (Van Buuren and Groothuis-Oushoorn Citation2011), and used only data from the relevant taxon group (i.e. treating fossil and modern Pedionomus separately). The PCA plots were created using the packages ggplot2 (Wickham Citation2016), ggfortify (Tang et al. Citation2016) and RColorBrewer (Neuwirth Citation2022). Permutational multivariate analyses of variance (PERMANOVA) were subsequently conducted using the package PERMANOVA (Vicente-Gonzalez and Vicente-Villardon Citation2021) to test for significant difference between fossil and modern Pedionomus within individual elements. Analyses were not conducted for ulnae or femora due to an insufficient sample of fossil specimens (see Table S1).
A PCA was conducted on the combined data of all elements following log10 transformation. Due to the isolated nature of the fossil elements, mean untransformed values of each variable were used to create a single fossil ‘individual’ to enable comparison with modern individuals of Pedionomus torquatus. Five modern specimens were also removed from the analysis due to a lack of elements resulting in much missing data. To minimise the effects of highly variable measurements, an additional PCA was also conducted using only variables with a coefficient of variation less than 0.08.
Results
Univariate analyses
Mean values were greater in fossil Pedionomus than modern Pedionomus torquatus for all variables (), bar four. Fossil and modern Pedionomus were equal in humerus distal depth and tarsometatarsus total length, while modern specimens were larger in femur distal depth (for which only one fossil specimen was sampled) and tibiotarsus distal depth (which did not differ significantly: t = −1.8961, df = 34.970, p = 0.06623). Further results of univariate analyses are reported alongside multivariate results below.
Table 2. Mean (in mm), standard deviation, and sample size (n) of all variables measured from Naracoorte fossil Pedionomus, modern Pedionomus torquatus, and Erythrogonys cinctus. Abbreviations follow .
Humerus
A PCA was conducted to visualise variation in humerus data (). The principal component (PC) 1 accounted for 72.38% of variation in the data, and PC2 accounted for 10.71%. There was marked overlap between all groups, with Charadrius bicinctus and Erythrogonys cinctus plotting in the region of overlap between fossil and modern Pedionomus. There was marked overlap between fossil and modern Pedionomus, with fossil specimens tending to occupy lower PC1 values. A PERMANOVA revealed significant difference between fossil and modern Pedionomus humeri (F = 14.273, p = 9.990 × 10−4). Visual inspection of the biplot indicated that these differences were primarily driven by the larger size of fossil elements, which was largely corroborated by Mann–Whitney U tests demonstrating that fossil specimens had significantly greater total length (W = 466, p = 8.299 × 10−3) and proximal width (W = 616.5, p = 1.453 × 10−5), while t-tests demonstrated fossil specimens had significantly greater proximal length (t = 3.3885, df = 24.768, p = 2.353 × 10−3), shaft width (t = 4.8155, df = 25.539, p = 5.709 × 10−5), and distal width (t = 2.9293, df = 22.695, p = 7.605 × 10−3) (). Fossil and modern Pedionomus therefore appeared to differ only in average size. It is also notable that there was no distinction between fossil humeri from VFC and Blanche Cave, with all VFC fossils plotting within the Blanche Cave morphospace (Figure S2).
Figure 1. Principal component analyses of forelimb element measurement data from Naracoorte fossil Pedionomus (squares), modern Pedionomus torquatus (dots), Charadrius bicinctus (diamond), and Erythrogonys cinctus (triangles) specimens. A – Humerus; B – Carpometacarpus; C – Coracoid. Abbreviations on biplot follow .
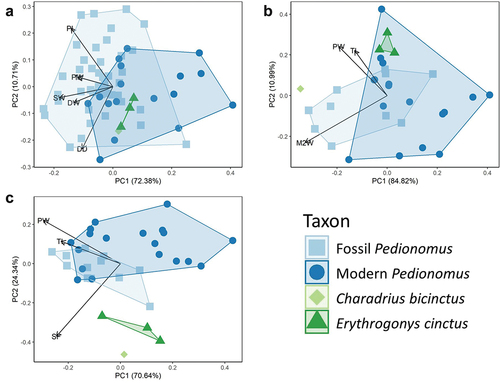
Carpometacarpus
In the PCA conducted on carpometacarpus data (), the PC1 accounted for 84.82% of the variation in the data, and PC2 for 10.99%. Erythrogonys cinctus fully overlapped with both fossil and modern Pedionomus, while Charadrius bicinctus was distinct from all other groups. There was marked overlap between fossil and modern Pedionomus. A PERMANOVA revealed significant difference between fossil and modern specimens (F = 5.940, p = 9.990 × 10−3), with visual inspection of the biplot indicating that this was once more a result of moderately larger size in the fossil individuals. A Mann–Whitney U test revealed that the difference in total length between fossil and modern Pedionomus was marginally non-significant (W = 102, p = 0.05081), while t-tests further demonstrated significantly greater proximal width (t = 2.4447, df = 20.472, p = 0.02365) and metacarpal II width (t = 2.7123, df = 14.655, p = 0.01632) in fossil specimens ().
Coracoid
In the PCA conducted on coracoid data (), PC1 accounted for 70.64% of variation in the data, and PC2 for 24.34%. There was marked segregation between Pedionomus, Charadrius bicinctus, and Erythrogonys cinctus along PC2. There was partial overlap between fossil and modern Pedionomus, with visual inspection of the biplot indicating that differences were primarily driven by sternal facet width. The t-tests corroborated this, showing significantly greater sternal facet width in fossil specimens (t = 4.0764, df = 21.246, p = 5.308 × 10−4) (), while total length and proximal width were not found to differ significantly. A PERMANOVA showed significant difference between fossil and modern Pedionomus data (F = 5.726, p = 0.01199).
Tibiotarsus
In the PCA conducted on tibiotarsus data (), PC1 accounted for 59.24% of the variation in the data, and PC2 accounted for 16.28%. There was marked segregation between Pedionomus, Charadrius bicinctus, and Erythrogonys cinctus, with Pedionomus and the charadriids separating along PC2. Visual inspection of the biplot indicated this was primarily driven by greater tibiotarsus total length in the charadriids. There was marked overlap between fossil and modern Pedionomus, though a PERMANOVA demonstrated significant difference between these groups (F = 12.446, p = 9.990 × 10−4). Visual inspection of the biplot indicated that the difference between fossil and modern Pedionomus was driven by greater width values in fossil specimens. Mann–Whitney U tests showed significantly greater proximal width in fossil specimens (W = 380, p = 5.885 × 10−5) and distal width (W = 238.5, p = 0.0314), while a t-test showed significantly greater shaft width (t = 6.1608, df = 36.734, p = 3.915 × 10−7).
Figure 2. Principal component analysis of hindlimb element data from Naracoorte fossil Pedionomus (squares), modern Pedionomus torquatus (dots), Charadrius bicinctus (diamond), and Erythrogonys cinctus (triangles) specimens (legend as in ). A – Tibiotarsus; B – Tarsometatarsus. Abbreviations on biplot follow .
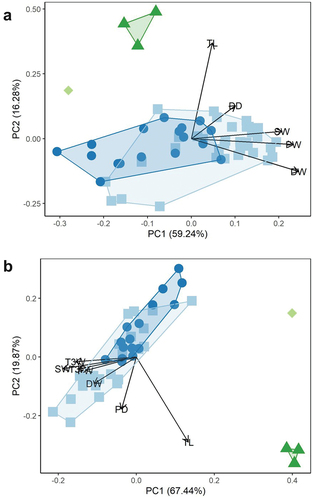
Tarsometatarsus
In the PCA conducted on tarsometatarsus data (), the PC1 accounted for 67.44% of the variation in the data, and PC2 for 19.87%. There was marked segregation between Pedionomus, Charadrius bicinctus, and Erythrogonys cinctus, with Pedionomus and the charadriids separating primarily along PC1 – a difference largely driven by greater total length in E. cinctus. There was marked overlap between fossil and modern Pedionomus, with most modern specimens plotting within the morphospace of fossil specimens. Many fossils, however, occupied lower PC1 and PC2 values than any modern individuals. A PERMANOVA showed significant difference between fossil and modern Pedionomus (F = 8.607, p = 1.998 × 10−3). Inspection of the biplot showed that this difference was primarily driven by greater width and depth values in the fossil specimens, with t-tests showing significantly greater proximal depth (t = 6.0585, df = 28.998, p = 1.357 × 10−6), shaft width (t = 3.9007, df = 34.362, p = 4.247 × 10−4), distal width (t = 2.0996, df = 34.036, p = 0.04326), and T3 depth (t = 2.437, df = 29.938, p = 0.02097) in fossil specimens (see ).
Whole skeleton
The ‘individual’ created from mean data generally had larger values for each element than the mean values of extant skeletons (). A PCA was conducted on skeleton wide data with the averaged fossil Pedionomus skeleton (). The PC1 accounted for 38.78% of variation in the data, and PC2 accounted for 35.36%. There was marked separation on PC2 between Pedionomus and the charadriids. Modern Pedionomus were broadly distributed along PC1 but occupied a relatively narrow range of values on PC2. There was no separation between fossil and modern Pedionomus, however, with the averaged fossil specimen occupying the low PC1 region of the modern P. torquatus morphospace. The averaged fossil Pedionomus therefore displayed moderately lower PC1 values than most modern P. torquatus.
Figure 3. Principal component analysis of linear measurements of the appendicular skeleton of Naracoorte fossil Pedionomus (square), modern Pedionomus torquatus (dots), Charadrius bicinctus (diamond), and Erythrogonys cinctus (triangles) (legend as in ). Due to a lack of complete specimens, fossil Pedionomus data represents the mean of all fossil specimens. A – whole dataset; B – measurements of Pedionomus specimens with low (<0.08) coefficients of variation. Biplot data indicate negative PC1 values in Figure A and positive PC2 values in Figure B are primarily driven by large size of width values.
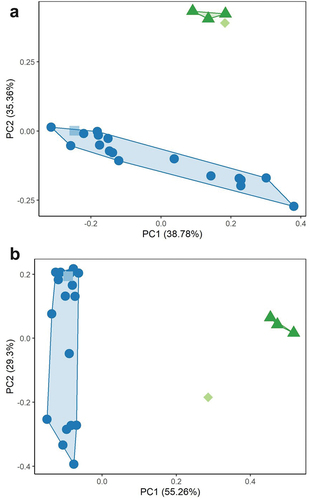
An additional PCA was conducted using a reduced dataset including only measurements with low (<0.08) coefficients of variation (). A total of 14 measurements were found to meet the threshold for this analysis and were therefore included. These were humerus total length, proximal width, and distal width, carpometacarpus total length and proximal width, femur total length, tibiotarsus total length, distal width, and distal depth, and tarsometatarsus total length, proximal width, proximal depth, distal width, and trochlea metatarsi III (T3) width. The PC1 accounted for 55.26% of the variation in the data, and PC2 for 29.30%. There was no separation between fossil and modern Pedionomus, with the averaged fossil specimen occupying low PC1 values within the modern P. torquatus morphospace. This suggests that there were no proportional differences between the modern and fossil specimens of Pedionomus.
Discussion
Morphology
All multivariate analyses conducted showed marked overlap between the morphospace of Naracoorte fossil Pedionomus and that of modern P. torquatus (), indicating that the groups are not readily distinguishable from one another. As such, these data do not support a species level distinction between the Naracoorte fossil Pedionomus and modern P. torquatus. Despite PERMANOVAs demonstrating significant differences between the groups, these appeared to largely be driven by a difference in size () – fossil specimens being generally larger than modern ones. In this size-dimorphic species, females are markedly larger than males (Olson and Steadman Citation1981; Székely et al. Citation2000), and the smaller Naracoorte fossils – presumed males – wholly overlapped the range of larger (female) modern specimens. Furthermore, slight size differences may be accounted for by increased body mass in cooler climates, resulting from both geographic and temporal variation (see Wooller et al. Citation1985; Gardner et al. Citation2019). This alone is therefore insufficient morphological evidence to justify taxonomic distinction.
Ratios of width and depth values divided by length of the humerus and tarsometatarsus (see Table S3) also showed significant difference between fossil and modern specimens only in shaft width (both elements) and tarsometatarsus proximal depth – all of which nevertheless showed extensive overlap (Figure S1) – indicating that any minor proportional variations observed are inadequate to justify taxonomic distinction, and therefore may be accounted for by variations over time or between populations. Furthermore, no qualitative traits which unambiguously differentiated the Naracoorte Pedionomus from modern P. torquatus were observed. Morphological data therefore do not support the suggestion (Laslett Citation2006) that the greater size of fossil Pedionomus may indicate taxonomic distinction. The Naracoorte fossil Pedionomus appear to represent a conspecific population of P. torquatus which were moderately larger than modern individuals.
The few fossils from the older VFC deposits (>400–220 ka) limited effective morphometric comparisons to those from Blanche Cave (45–15 ka) to the humerus, the only element with sample size >10 from both localities. No size differences were observed between fossil humeri from VFC and Blanche Cave, despite the two deposits sampling different periods of the Late Pleistocene (Moriarty et al. Citation2000; Grün et al. Citation2001; Darrénougué et al. Citation2009; St Pierre et al. Citation2012; Arnold et al. Citation2022). The deposits in VFC sample at least two glacial–interglacial cycles, while those from Blanche Cave date mainly to the cool-climate last glacial period (Marine Isotopic Stages (MIS) 4–2), highlighting the possibility that the Pedionomus from these deposits may differ if size was temperature related. However, the complete overlap in humerus morphospace indicates no such distinction (Figure S2). This strongly suggests that all identified Pedionomus from Naracoorte are conspecific, and that no apparent morphological change occurred in the taxon between deposition of these sites.
Ecology
The palaeoecology of the Naracoorte area as inferred from the fossil fauna has been a key interest in the literature. Such studies have been undertaken for Blanche Cave (Laslett Citation2006), Main Fossil Chamber (Wells et al. Citation1984; Moriarty et al. Citation2000) and Grant Hall Chamber (Fraser and Wells Citation2006; Macken et al. Citation2012) in Victoria Fossil Cave, Cathedral Cave (Brown and Wells Citation2000; Prideaux et al. Citation2007), Wet Cave (McDowell Citation2001), and Robertson Cave (McDowell Citation2001; Tavasci Citation2018). Macken and Reed (Citation2013) also provided a comprehensive list of the small mammal fauna from Blanche Cave, Robertson Cave, and Wet Cave. These analyses frequently show the fauna to be dominated by taxa characteristic of open sclerophyll forest or woodland, including a number of arboreal taxa such as koalas, possums, and phascogales (e.g. Brown and Wells Citation2000; Macken et al. Citation2012; Macken and Reed Citation2013). The large mammal fauna also includes an abundance of browsing herbivores (e.g. sthenurine kangaroos, Zygomaturus trilobus) (Wells et al. Citation1984; Moriarty et al. Citation2000; Brown and Wells Citation2000; Laslett Citation2006; Fraser and Wells Citation2006; Prideaux et al. Citation2007), indicating the presence of substantial mid-level vegetation cover. Plant microfossils from Robertson Cave equally demonstrate the presence of trees and shrubs around the cave entrance (Atkins et al. Citation2022). It is notable, however, that taxa characteristic of mallee or heathland – primarily murine rodents – have also consistently been identified (e.g. Laslett Citation2006; Fraser and Wells Citation2006; Prideaux et al. Citation2007; Macken and Reed Citation2013). These often dominate in relative abundance of specimens (e.g. Prideaux et al. Citation2007; Macken et al. Citation2012), indicating a degree of environmental heterogeneity. A proportion of the fauna further indicates the presence of some grassland habitat (Wells et al. Citation1984; Laslett Citation2006; Prideaux et al. Citation2007), although the presence of large grazing herbivores such as some macropodids is also consistent with grassy woodland (Brown and Wells Citation2000; Macken et al. Citation2012). The avifauna from the site remains poorly described, however the few birds that have been reported are generally consistent with a forest or woodland habitat (see Van Tets and Smith Citation1974; Laslett Citation2006), in addition to some wetland taxa (wading Charadriiformes, Rallidae). Indeed, other reported taxa typical of open environments (e.g. Little Buttonquail, Turnix velox; Stubble Quail, Coturnix pectoralis) may also be found in open grassy woodlands or shrublands today (Marchant and Higgins Citation1993; Forshaw and Knight Citation2023), and thus remain consistent with a forest and open woodland dominated environment. The Naracoorte palaeoenvironment is therefore likely best summarised as a mosaic habitat dominated by open sclerophyll forest and grassy woodland.
The above interpretation does, however, ignore change over the 500,000 years represented in the Naracoorte deposits (Ayliffe et al. Citation1998; Grün et al. Citation2001; Prideaux et al. Citation2007). Indeed, while earlier studies failed to detect changes over time within individual deposits (Moriarty et al. Citation2000; Grün et al. Citation2001) more recent analyses have demonstrated temporal variation within and between the faunas of Blanche Cave (Laslett Citation2006), Cathedral Cave (Prideaux et al. Citation2007), and Grant Hall (Macken et al. Citation2012). These together show repeated shifts over time in the relative prominences of wooded and more open environments, with wooded environments generally dominating during wetter phases, while more open environments such as heathland or grassland generally dominate during dryer phases (Prideaux et al. Citation2007; Macken et al. Citation2012). Notably, however, woodland and forest taxa persist to an extent in the area even during more grassland-dominated phases (see Laslett Citation2006; Prideaux et al. Citation2007; Macken et al. Citation2012), and the taxonomic composition of the fauna appears to have remained generally stable across time (Macken and Reed Citation2014), highlighting that wooded environments remained in the area to some extent even when less abundant.
The presence of Pedionomus torquatus in the Naracoorte deposits is thus perplexing. Modern individuals survive exclusively in sparse open grassland environments (Bennett Citation1983; Harrington et al. Citation1988; Baker-Gabb et al. Citation1990; Marchant and Higgins Citation1993; Baker‐Gabb et al. Citation2016; Antos and Schultz Citation2020; Forshaw and Knight Citation2023), and have not been found to live in areas with any form of woodland (Harrington et al. Citation1988; Baker-Gabb et al. Citation1990; Marchant and Higgins Citation1993; Forshaw and Knight Citation2023). Indeed, the habitat specialisation of modern P. torquatus is such that variations in grass cover within a single habitat may have a significant influence on population levels (Baker‐Gabb et al. Citation2016). Survival of P. torquatus in a predominantly wooded environment such as Naracoorte would therefore appear to contrast sharply with all modern data on the taxon. While one possible explanation may be that they inhabited pockets of grassland interspersed within the forest and woodland, this too appears improbable as modern P. torquatus require large areas of open grassland (approximately 9 hectares per individual) (Baker-Gabb et al. Citation1990), and thus sufficient expanses to support a viable population are unlikely to have been present at Naracoorte. Furthermore, while the available data are insufficient to effectively describe variation in the Pedionomus population through time, it is notable that the taxon appears to be consistently present in every major depth interval in the deposits (see Table S1), and thus persisted through several key phases of aridification and increased precipitation (e.g. Ayliffe et al. Citation1998; De Deckker et al. Citation2021). The Naracoorte fossil P. torquatus population therefore appears to have been relatively resilient to environmental variations, which differs markedly from trends seen in modern individuals (see Baker‐Gabb et al. Citation2016).
Another explanation for the presence of P. torquatus in the Naracoorte deposits may be accumulation via owl pellets. Indeed, it has been argued that much of the small mammal fauna in the Naracoorte deposits was likely accumulated primarily by roosting owls (McDowell Citation2001; Laslett Citation2006; Fraser and Wells Citation2006; Macken et al. Citation2012; Tavasci Citation2018), with the same likely holding true for the avifauna (evidenced by acid etching observed on P. torquatus specimens from Blanche Cave). It is therefore possible that remains were transported from more distant grasslands to the site by owls, as has been suggested for some anachronistic mammal faunal elements at the site (e.g. Fraser and Wells Citation2006; Tavasci Citation2018). It should be noted, however, that P. torquatus are relatively abundant in the Naracoorte deposits, with 105 specimens recorded herein from Blanche Cave alone (see Table S1). Such a number of specimens seems unlikely to have originated from distant environments, as the main predator – the Australian Masked Owl (Tyto novaehollandiae) – today occupies home ranges of only 1000–1500 ha, and preferentially hunts in open woodland environments (Kavanagh and Murray Citation1996; McNabb et al. Citation2003). The Eastern Barn Owl (Tyto javanica) has also been reported from Blanche Cave (Laslett Citation2006; Reed and Bourne Citation2009), although it appears to be less common (pers. obs. authors). Little literature exists on the home range of this taxon; however, Western Barn Owls (Tyto alba) in Iran have an average hunting radius of 2.5 km (Cain et al. Citation2023) – comparable with the range of T. novaehollandiae – indicating that such ranges may be common for large owls, and therefore that the Naracoorte P. torquatus were likely present in the nearby environment. Furthermore, a small number of juvenile specimens were identified from the deposits, indicating that P. torquatus were likely breeding in the immediate area. Pedionomus torquatus is also a generally sedentary species, as well a reluctant flier (Marchant and Higgins Citation1993; Forshaw and Knight Citation2023) – highlighting that the Naracoorte specimens were unlikely to be migrant individuals. This, therefore, suggests that the P. torquatus at Naracoorte were likely permanent inhabitants of the local area, and thus occupied a habitat distinct from that which the species is limited to today.
The presence of Pedionomus torquatus specimens in the Naracoorte deposits, therefore, may indicate that the species has undergone a shift or contraction in its fundamental niche (that a species is adapted to or could occupy) during the late Quaternary. While the limited fossil record of the taxon renders it unclear whether the species also inhabited open grassland during the Pleistocene, it is apparent that the Naracoorte population likely occupied a predominately wooded area for in excess of 200–400 thousand years (Moriarty et al. Citation2000; Grün et al. Citation2001; Darrénougué et al. Citation2009; St Pierre et al. Citation2012; Arnold et al. Citation2022). Other isolated elements of P. torquatus have been reported from Leaena’s Breath Cave on the Nullarbor Plain (Shute Citation2019), and several sites in Victoria – including Morwell (Vickers Rich and McEvey Citation1980) and Clogg’s Cave (Baird Citation1991). While the Laeana’s Breath deposits indicate the presence of grassland in the area during the interval in which P. torquatus was preserved (Shute Citation2019), the vegetation at Morwell during the Plio-Pleistocene appears to have been dominated by shrubs and open sclerophyll forest (Kershaw et al. Citation1991), while the Clogg’s Cave deposits preserve a heterogeneous fauna indicative of both forest and grassland habitats (McDowell et al. Citation2022). Pedionomus torquatus therefore appears to have inhabited a broad range of environments during most of the late Quaternary. A shift in environmental preference is also corroborated by the presence of the sister species – Oligonomus milleri – in deposits representing a wooded environment likely pre-dating the spread of grasslands across the continent (De Pietri et al. Citation2015), indicating that Pedionomidae may have ancestrally inhabited woodlands and forests. This, therefore, suggests that the dependence of P. torquatus exclusively on open grassland may be a recent phenomenon – likely occurring during the late Quaternary, contrary to the Neogene age suggested by De Pietri et al. (Citation2015). Causes for this shift are unclear, but may relate to changes in the faunal composition of the area following the last glacial maximum (see Macken and Reed Citation2014). Indeed, it has been suggested that modern P. torquatus inhabit open grassland primarily due to improved predator detection (Baker-Gabb et al. Citation1990), and may avoid wooded environments due to aerial predators hunting from trees (Harrington et al. Citation1988), although both hypotheses remain untested. Furthermore, Llewellyn (Citation1975) argued that P. torquatus is likely highly susceptible to predation by foxes and cats, although there are few empirical data to support this.
Implications for conservation
An accurate understanding of the past distribution of a taxon is vital to effective conservation efforts. A key example of this may be seen in the critically endangered Mountain Pygmy-possum (Burramys parvus), which today exclusively inhabits alpine areas above the snow line in Victoria and New South Wales (see Mansergh and Broome Citation1994; Broome et al. Citation2012). The species has been deemed highly susceptible to climate change due to an inability to adapt to warmer climates brought on with the rising snow line (Broome et al. Citation2012; Archer et al. Citation2019). However, the fossil record of the taxon indicates that species of Burramys inhabited wet, lowland forests for much of its evolutionary history (Archer et al. Citation1991, Citation2019; Broome et al. Citation2012), suggesting that reintroduction of B. parvus to non-alpine areas may be a viable means of conservation in the face of increasing global temperatures (Broome et al. Citation2012; Archer et al. Citation2019). This has led to the construction of a breeding facility at low altitudes at Secret Creek, Lithgow, New South Wales to test the viability of this approach (Archer et al. Citation2019), and the translocated individuals have already bred (Australian Ecosystems Foundation Inc Citation2022). Such an approach may therefore also be applicable to a number of other species inhabiting a range of different environments.
Additional evidence of the importance of fossil data to the translocation of threatened species may be derived from the Takahe (Porphyrio hochstetteri), a flightless rail from New Zealand. Prior to human arrival, Takahe appear to have occupied a far greater range of environments than the alpine tussock grasslands to which they are exclusive today (Beauchamp and Worthy Citation1988). This has encouraged translocation of the species to lowland islands in which the vegetations differs markedly from that of their modern habitat (Jamieson Citation2004), with high levels of overall success (Miskelly and Powlesland Citation2013). Fossil and past distributions have also been used to suggest potential sites for translocation in a number of other Australasian tetrapods, including the Kakapo (Strigops habroptila) (Lentini et al. Citation2018), Tuatara (Sphenodon punctatus) (Jarvie et al. Citation2021), and Eastern Quoll (Dasyurus viverrinus) (Barlow et al. Citation2021).
The indication from Naracoorte fossil specimens that Pedionomus torquatus previously inhabited a broader range of environments than observed today suggests that translocation to sites including open grassy woodland or sclerophyll forest may merit consideration as a means of conserving the species. Indeed, reintroduction of captive-bred individuals forms a key component of the national recovery plan for Plains-wanderers (Commonwealth of Australia Citation2016), and the loss of native grassland environments constitutes a major threat to the species (Commonwealth of Australia Citation2016; Forshaw and Knight Citation2023). Before such an attempt is made, however, it would be beneficial to clarify why modern Plains-wanderers are seemingly dependent on open grasslands, and if indeed its fundamental niche has altered from the adaptive capacity to inhabit wooded environments. A clearer understanding of the past distribution of Pedionomus torquatus through examination of other late Quaternary avifaunal deposits across the country may help clarify the timing, extent of, and perhaps reason for the probable ecological changes inferred herein. Furthermore, one might look to analogues such as buttonquails (Turnix) – which may inhabit both wooded (e.g. Painted Buttonquail, T. varius; Chestnut-backed Buttonquail, T. castanotus), and more open (e.g. Little Buttonquail, T. velox; Red-backed Buttonquail, T. maculosus) habitats (Marchant and Higgins Citation1993; Forshaw and Knight Citation2023) – to determine if any physiological or genetic factors not detectable from skeletal data alone may determine habitat choice.
Conclusion
Despite moderate differences in average size, morphometric analyses cannot unambiguously differentiate the Naracoorte fossil Pedionomus from modern specimens of P. torquatus. This corroborates referral of the fossil specimens from the Naracoorte area to P. torquatus, as has generally been assumed (Van Tets and Smith Citation1974; Reed and Bourne Citation2000, Citation2009). The Naracoorte palaeoenvironment does, however, appear to represent a principally wooded environment (e.g. Wells et al. Citation1984; Fraser and Wells Citation2006), differing sharply from the open grassland habitat occupied by modern P. torquatus. The Plains-wanderer, therefore, appears to have undergone a potentially substantial degree of shift or contraction in preferred habitat or fundamental niche during the Holocene, with wooded environments likely representing the ancestral habitat of Pedionomidae. It is hoped that future discoveries of pedionomids outside of the Naracoorte area may provide additional data on the pre-Holocene habitat preferences of the taxon. Additionally, the discovery that the Plains-wanderer previously occupied a much greater range of habitats than observed in the modern day suggests that the when contemplating the possible translocation of the species, sites not solely vegetated in grassland may merit consideration, with grassland–woodland mosaic environments a possibility to increase its resilience to future climate change.
Supplemental Material
Download MS Excel (33.6 KB)Supplemental Material
Download MS Word (395.3 KB)Acknowledgments
We thank Mary-Anne Binnie and Maya Penck for access to specimens at the SAMA, Karen Roberts and Kylea Clarke for access to those at NMV, and Jacqueline Nguyen for providing measurements of specimens at the AM. Similarly, Warren Handley provided valuable help with imputation in R and Diana Fusco provided feedback on a draft of this manuscript. We also thank Leo Joseph and two anonymous reviewers for their insightful comments which improved the quality of this manuscript.
Disclosure statement
No potential conflict of interest was reported by the author(s).
Supplementary material
Supplemental data for this article can be accessed at https://doi.org/10.1080/01584197.2023.2240346.
Additional information
Funding
References
- Antos, M., and Schultz, N. L. (2020). Climate-mediated changes to grassland structure determine habitat suitability for the critically endangered Plains-wanderer (Pedionomus torquatus). Emu - Austral Ornithology 120(1), 2–10. doi:10.1080/01584197.2019.1641415
- Archer, M., Bates, H., Hand, S. J., Evans, T., Broome, L., McAllan, B., and Geiser, F., et al. (2019). The Burramys project: A conservationist’s reach should exceed history’s grasp, or what is the fossil record for? Philosophical Transactions of the Royal Society B: Biological Sciences 374, 20190221. doi:10.1098/rstb.2019.0221
- Archer, M., Hand, S. J., and Godthelp, H. (1991). Back to the future: The contribution of palaeontology to the conservation of Australian forest faunas. In ‘Conservation of Australia’s Forest Fauna’. (Ed. D. Lunney.) pp. 67–80. (Royal Zoological Society of New South Wales: Sydney.) doi:10.7882/RZSNSW.1991.006
- Arnold, L. J., Demuro, M., Power, R., Duval, M., Guilarte, V., Weij, R., and Woodhead, J., et al. (2022). Examining sediment infill dynamics at Naracoorte cave megafauna sites using multiple luminescence dating signals. Quaternary Geochronology 70, 101301. doi:10.1016/j.quageo.2022.101301
- Atkins, R. A., Hill, R. S., Hill, K. E., Munroe, S. E., and Reed, E. H. (2022). Preservation quality of plant macrofossils through a Quaternary cave sediment sequence at Naracoorte, South Australia: Implications for vegetation reconstruction. Review of Palaeobotany and Palynology 299, 104607. doi:10.1016/j.revpalbo.2022.104607
- Australian Ecosystems Foundation Inc. (2022). ‘Saving the Mountain Pygmy-possum (Burramys parvus).’ Available at https://www.ausecosystems.org.au/mountain-pygmy-possum [Verified 27 April 2023].
- Ayliffe, L. K., Marianelli, P. C., Moriarty, K. C., Wells, R. T., McCulloch, M. T., Mortimer, G. E., and Hellstrom, J. C. (1998). 500 ka precipitation record from southeastern Australia: Evidence for interglacial relative aridity. Geology 26(2), 147–150. doi:10.1130/0091-7613(1998)026<0147:KPRFSA>2.3.CO;2
- Baird, R. F. (1991). Avian fossils from the quaternary of Australia. In ‘Vertebrate Palaeontology of Australia’. (Eds P. Vickers Rich, R. F. Monahan, R. F. Baird, and T. H. Rich.) pp. 809–870. (Pioneer Design Studio: Melbourne.)
- Baker‐Gabb, D. J., Antos, M., and Brown, G. (2016). Recent decline of the critically endangered Plains‐wanderer (Pedionomus torquatus), and the application of a simple method for assessing its cause: Major changes in grassland structure. Ecological Management & Restoration 17(3), 235–242. doi:10.1111/emr.12221
- Baker-Gabb, D. J. (1990). An annotated list of records of Plains-wanderers’ Pedionomus torquatus’, 1980-89. Australian Bird Watcher 13(8), 249–252.
- Baker-Gabb, D. J., Benshemesh, J. S., and Maher, P. N. (1990). A revision of the distribution, status and management of the Plains-wanderer Pedionomus torquatus. Emu - Austral Ornithology 90(3), 161–168. doi:10.1071/MU9900161
- Baker, A. J., Pereira, S. L., and Paton, T. A. (2007). Phylogenetic relationships and divergence times of Charadriiformes genera: Multigene evidence for the Cretaceous origin of at least 14 clades of shorebirds. Biology Letters 3(2), 205–210. doi:10.1098/rsbl.2006.0606
- Barlow, M. M., Johnson, C. N., McDowell, M. C., Fielding, M. W., Amin, R. J., and Brewster, R. (2021). Species distribution models for conservation: Identifying translocation sites for Eastern Quolls under climate change. Global Ecology and Conservation 29, e01735. doi:10.1016/j.gecco.2021.e01735
- Beauchamp, A. J., and Worthy, T. H. (1988). Decline in distribution of the Takahe Porphyrio (= Notornis) mantelli: A re-examination. Journal of the Royal Society of New Zealand 18(1), 103–118. doi:10.1080/03036758.1988.10421698
- Bennett, S. (1983). A review of the distribution, status and biology of the Plains-wanderer Pedionomus torquatus, Gould. Emu - Austral Ornithology 83(1), 1–11. doi:10.1071/MU9830001
- Bock, W. J., and McEvey, A. (1969). Osteology of Pedionomus torquatus (Aves: Pedionomidae) and its allies. Proceedings of the Royal Society of Victoria 82, 187–232.
- Broome, L., Archer, M., Bates, H., Shi, H., Geiser, F., McAllan, B., and Heinze, D., et al. (2012). A brief review of the life history of, and threats to, Burramys parvus with a prehistory-based proposal for ensuring that it has a future. In ‘Wildlife and Climate Change: Towards Robust Conservation Strategies for Australian Fauna’. (Eds D. Lunney and P. Hutchings.) pp. 114–126. (Royal Zoological Society of New South Wales: Mosman, New South Wales.) doi:10.7882/FS.2012.018
- Brown, S. P., and Wells, R. T. (2000). A middle Pleistocene vertebrate fossil assemblage from Cathedral Cave, Naracoorte, South Australia. Transactions of the Royal Society of South Australia 124, 91–104.
- Cain, S., Solomon, T., Leshem, Y., Toledo, S., Arnon, E., Roulin, A., and Spiegel, O. (2023). Movement predictability of individual Barn Owls facilitates estimation of home range size and survival. Movement Ecology 11(1), 10. doi:10.1186/s40462-022-00366-x
- Commonwealth of Australia. (2016). ‘National Recovery Plan for the Plains-Wanderer (Pedionomus torquatus).’ (Commonwealth of Australia: Canberra.)
- Darrénougué, N., De Deckker, P., Fitzsimmons, K. E., Norman, M. D., Reed, E. H., van der Kaars, S., and Fallon, S. (2009). A late Pleistocene record of aeolian sedimentation in Blanche Cave, Naracoorte, South Australia. Quaternary Science Reviews 28, 2600–2615. doi:10.1016/j.quascirev.2009.05.021
- De Deckker, P., Van Der Kaars, S., Haberle, S., Hua, Q., and Stuut, J.-B. (2021). The pollen record from marine core MD03-2607 from offshore Kangaroo Island spanning the last 125 ka; implications for vegetation changes across the Murray-Darling basin. Australian Journal of Earth Sciences 68(7), 928–951. doi:10.1080/08120099.2021.1896578
- Department of the Environment. (2015). ‘Conservation Advice Pedionomus torquatus.’ (Plains-wanderer. Department of the Environment: Canberra.)
- De Pietri, V. L., Camens, A. B., Worthy, T. H., and Collinson, M. (2015). A Plains‐wanderer (Pedionomidae) that did not wander plains: A new species from the Oligocene of South Australia. Ibis 157(1), 68–74. doi:10.1111/ibi.12215
- De Pietri, V. L., Scofield, R. P., Tennyson, A. J. D., Hand, S. J., and Worthy, T. H. (2016). Wading a lost southern connection: Miocene fossils from New Zealand reveal a new lineage of shorebirds (Charadriiformes) linking Gondwanan avifaunas. Journal of Systematic Palaeontology 14(7), 603–616. doi:10.1080/14772019.2015.1087064
- Fjeldså, J. (1996). Family Thinocoridae (seedsnipes). In ‘Handbook of the Birds of the World. Vol. 3’. Hoatzin to Auks’ (Eds J. del Hoyo, A. Elliott, and J. Sargatal.) pp. 538–545. (Lynx Edicions: Barcelona, Spain.)
- Forshaw, J. M., and Knight, F. (2023). ‘Quail, Buttonquail and Plains-Wanderer in Australia and New Zealand.’ (CSIRO Publishing: Collingwood, Victoria.)
- Fraser, R. A., and Wells, R. T. (2006). Palaeontological excavation and taphonomic investigation of the late Pleistocene fossil deposit in Grant Hall, Victoria Fossil Cave, Naracoorte, South Australia. Alcheringa: An Australasian Journal of Palaeontology 30(sup1), 147–161. doi:10.1080/03115510609506860
- Gadow, H. (1891). Notes on the structure of Pedionomus torquatus, with regard to its systematic position. Records of the Australian Museum 1(10), 205–211. doi:10.3853/j.0067-1975.1.1891.1259
- Gardner, J. L., Amano, T., Peters, A., Sutherland, W. J., Mackey, B., Joseph, L., and Stein, J., et al. (2019). Australian songbird body size tracks climate variation: 82 species over 50 years. Proceedings of the Royal Society B: Biological Sciences 286, 20192258. doi:10.1098/rspb.2019.2258
- Geyle, H. M., Woinarski, J. C., Baker, G. B., Dickman, C. R., Dutson, G., Fisher, D. O., and Ford, H., et al. (2018). Quantifying extinction risk and forecasting the number of impending Australian bird and mammal extinctions. Pacific Conservation Biology 24(2), 157–167. doi:10.1071/PC18006
- Gibson, R., and Baker, A. (2012). Multiple gene sequences resolve phylogenetic relationships in the shorebird suborder Scolopaci (Aves: Charadriiformes). Molecular Phylogenetics and Evolution 64(1), 66–72. doi:10.1016/j.ympev.2012.03.008
- Grosjean, P., and Ibanez, F. (2018). ‘pastecs: Package for analysis of space-time ecological series.’ Available at https://CRAN.R-project.org/package=pastecs.
- Grün, R., Moriarty, K., and Wells, R. (2001). Electron spin resonance dating of the fossil deposits in the Naracoorte Caves, South Australia. Journal of Quaternary Science 16(1), 49–59. doi:10.1002/1099-1417(200101)16:1%3C49:AID-JQS570%3E3.0.CO;2-%23
- Harrington, G. N., Maher, P. N., and Baker-Gabb, D. J. (1988). The biology of the Plains-wanderer Pedionomus torquatus on the Riverine Plain of New South Wales during and after drought. Corella 12(1), 7–13.
- Jamieson, I. G. (2004). No evidence that dietary nutrient deficiency is related to poor reproductive success of translocated Takahe. Biological Conservation 115(1), 165–170. doi:10.1016/S0006-3207(03)00216-7
- Jarvie, S., Worthy, T. H., Saltré, F., Scofield, R. P., Seddon, P. J., and Cree, A. (2021). Using Holocene fossils to model the future: distribution of climate suitability for Tuatara, the last rhynchocephalian. Journal of Biogeography 48(6), 1489–1502. doi:10.1111/jbi.14092
- Jetz, W., Thomas, G. H., Joy, J. B., Redding, D. W., Hartmann, K., and Mooers, A. O. (2014). Global distribution and conservation of evolutionary distinctness in birds. Current Biology 24(9), 919–930. doi:10.1016/j.cub.2014.03.011
- Kavanagh, R. P., and Murray, M. (1996). Home range, habitat and behaviour of the Masked Owl Tyto novaehollandiae near Newcastle, New South Wales. Emu - Austral Ornithology 96(4), 250–257. doi:10.1071/MU9960250
- Kershaw, A. P., D’Costa, D. M., McEwen Mason, J. R. C., and Wagstaff, B. E. (1991). Palynological evidence for Quaternary vegetation and environments of mainland southeastern Australia. Quaternary Science Reviews 10(5), 391–404. doi:10.1016/0277-3791(91)90003-D
- Kuhl, H., Frankl-Vilches, C., Bakker, A., Mayr, G., Nikolaus, G., Boerno, S. T., and Klages, S., et al. (2021). An unbiased molecular approach using 3′-UTRs resolves the avian family-level tree of life. Molecular Biology and Evolution 38(1), 108–127. doi:10.1093/molbev/msaa191
- Laslett, T. M. (2006). A palaeoecological study of a Quaternary vertebrate fossil deposit in Blanche Cave, Naracoorte, South Australia. BSc (Hons) Thesis, Flinders University, Adelaide.
- Lentini, P. E., Stirnemann, I. A., Stojanovic, D., Worthy, T. H., and Stein, J. A. (2018). Using fossil records to inform reintroduction of the Kakapo as a refugee species. Biological Conservation 217, 157–165. doi:10.1016/j.biocon.2017.10.027
- Llewellyn, L. C. (1975). Recent observations on the Plains-wanderer, with a review of its past and present status. Emu - Austral Ornithology 75(3), 137–142. doi:10.1071/MU9750137
- Macken, A. C., Prideaux, G. J., and Reed, E. H. (2012). Variation and pattern in the responses of mammal faunas to late Pleistocene climatic change in southeastern South Australia. Journal of Quaternary Science 27(4), 415–424. doi:10.1002/jqs.1563
- Macken, A. C., and Reed, E. H. (2013). Late Quaternary small mammal faunas of the Naracoorte Caves World Heritage Area. Transactions of the Royal Society of South Australia 137(1), 53–67. doi:10.1080/3721426.2013.10887171
- Macken, A. C., and Reed, E. H. (2014). Postglacial reorganization of a small‐mammal paleocommunity in southern Australia reveals thresholds of change. Ecological Monographs 84(4), 563–577. doi:10.1890/13-0713.1
- Mansergh, I. M., and Broome, L. (1994). ‘The Mountain Pygmy-Possum of the Australian Alps.’ (New South Wales University Press: Kensington, New South Wales.)
- Marchant, S., and Higgins, P. J. (1993). ‘Handbook of Australian, New Zealand & Antarctic Birds: Volume 2 Raptors to Lapwings.’ (Oxford University Press: Melbourne, Victoria.)
- McDowell, M. C. (2001). The analysis of late Quaternary fossil mammal faunas from Robertson Cave (5U17, 18, 19) & Wet Cave (5U10, 11) in the Naracoorte World Heritage Area, South Australia. MSc Thesis, Flinders University, Adelaide.
- McDowell, M. C., David, B., Mullett, R., Fresløv, J., Delannoy, J. J., Mialanes, J., and Thomas, C., et al. (2022). Interpreting the mammal deposits of Cloggs Cave (SE Australia), GunaiKurnai Aboriginal Country, through community‐led partnership research. People and Nature 4(6), 1629–1643. doi:10.1002/pan3.10410
- McNabb, E., McNabb, J., and Barker, K. (2003). Post-nesting home range, habitat use and diet of a female Masked Owl Tyto novaehollandiae in western Victoria. Corella 27(4), 109–117.
- Miskelly, C. M., and Powlesland, R. (2013). Conservation translocations of New Zealand birds, 1863–2012. Notornis 60(1), 3–28.
- Moriarty, K. C., McCulloch, M. T., Wells, R. T., and McDowell, M. C. (2000). Mid-Pleistocene cave fills, megafaunal remains and climate change at Naracoorte, South Australia: Towards a predictive model using U-Th dating of speleothems. Palaeogeography, Palaeoclimatology, Palaeoecology 159(1–2), 113–143. doi:10.1016/S0031-0182(00)00036-5
- Neuwirth, E. (2022). ‘RColorBrewer: ColorBrewer Palettes.’ Available at https://CRAN.R-project.org/package=RColorBrewer.
- Nugent, D. T., Baker‐Gabb, D. J., Green, P., Ostendorf, B., Dawlings, F., Clarke, R. H., and Morgan, J. W. (2022). Multi‐scale habitat selection by a cryptic, critically endangered grassland bird—the Plains‐wanderer (Pedionomus torquatus): Implications for habitat management and conservation. Austral Ecology 47(3), 698–712. doi:10.1111/aec.13157
- Olson, S. L., and Steadman, D. W. (1981). The relationships of the Pedionomidae (Aves, Charadriiformes). Smithsonian Contributions to Zoology 337, 1–25. doi:10.5479/si.00810282.337
- Prideaux, G. J., Roberts, R. G., Megirian, D., Westaway, K. E., Hellstrom, J. C., and Olley, J. M. (2007). Mammalian responses to Pleistocene climate change in southeastern Australia. Geology 35(1), 33–36. doi:10.1130/G23070A.1
- R Core Team. (2022). ‘R: A language and environment for statistical computing.’ Available at https://www.R-project.org/.
- Reed, E. H., and Bourne, S. J. (2000). Pleistocene fossil vertebrate sites of the South East region of South Australia. Transactions of the Royal Society of South Australia 124(2), 61–90.
- Reed, E. H., and Bourne, S. J. (2009). Pleistocene fossil vertebrate sites of the South East region of South Australia II. Transactions of the Royal Society of South Australia 133(1), 30–40. doi:10.1080/03721426.2009.10887108
- Shute, E. (2019). Early and Middle Pleistocene non-passerine bird fossils from the Thylacoleo Caves, Nullarbor Plain. Ph.D. Thesis, Flinders University, Adelaide.
- St Pierre, E., Zhao, J.-X., Feng, Y.-X., and Reed, E. (2012). U-series dating of soda straw stalactites from excavated deposits: Method development and application to Blanche Cave, Naracoorte, South Australia. Journal of Archaeological Science 39(4), 922–930. doi:10.1016/j.jas.2011.10.027
- Székely, T., Reynolds, J. D., and Figuerola, J. (2000). Sexual size dimorphism in shorebirds, gulls, and alcids: The influence of sexual and natural selection. Evolution 54(4), 1404–1413. doi:10.1111/j.0014-3820.2000.tb00572.x
- Tang, Y., Horikoshi, M., and Li, W. (2016). ggfortify: Unified interface to visualize statistical result of popular R packages. The R Journal 8(2), 478–489. doi:10.32614/RJ-2016-060
- Tavasci, P. R. (2018). Palaeoecology and preliminary taphonomy of the Robertson Cave entrance chamber fauna, Naracoorte. BSc (Hons) Thesis, Univeristy of Adelaide, Adelaide.
- Van Buuren, S., and Groothuis-Oushoorn, K. (2011). mice: Multivariate imputation by chained equations in R. Journal of Statistical Software 45(3), 1–67. doi:10.18637/jss.v045.i03
- Van Tets, G. F., and Smith, M. J. (1974). Small fossil vertebrates from Victoria Fossil Cave, Naracoorte, South Australia: III. Birds (Aves). Transactions of the Royal Society of South Australia 98(4), 225–227.
- Van Tuinen, M., Waterhouse, D., and Dyke G, J. (2004). Avian molecular systematics on the rebound: A fresh look at modern shorebird phylogenetic relationships. Journal of Avian Biology 35(3), 191–194. doi:10.1111/j.0908-8857.2004.03362.x
- Vicente-Gonzalez, L., and Vicente-Villardon, J. (2021). ‘PERMANOVA: Multivariate analysis of variance based on distances and permutations.’ Available at https://CRAN.R-project.org/package=PERMANOVA.
- Vickers Rich, P., and McEvey, A. (1980). A fossil plain wanderer (Aves: Pedionomidae) from fire-hole deposits, Morwell, southeastern Victoria, Australia. Contributions in Science/Natural History Museum of Los Angeles County 330, 251–255. doi:10.5962/p.226848
- Wells, R. T., Moriarty, K., and Williams, D. (1984). The fossil vertebrate deposits of Victoria Fossil Cave Naracoorte: An introduction to the geology and fauna. The Australian Zoologist 21(4), 305–333.
- Wickham, H. (2016). ‘ggplot2: Elegant graphics for data analysis.’ Available at https://ggplot2.tidyverse.org.
- Wooller, R. D., Saunders, D. A., Bradley, J. S., and de Rebeira, C. P. (1985). Geographical variation in size of an Australian honeyeater (Aves: Meliphagidae): An example of Bergmann’s rule. Biological Journal of the Linnean Society 25(4), 355–363. doi:10.1111/j.1095-8312.1985.tb00401.x