ABSTRACT
There have been few empirical studies of the sensitivity of birds to the effect of air pollutants. In late 2019 and early 2020 the Australian Capital Territory (ACT) and much of south-eastern Australia were affected by extreme wildfire events and smoke extended to surrounding areas. Prior to this event, GPS transmitters had been fitted to a sample of Little Eagles Hieraaetus morphnoides in the ACT as part of a study of their movement behaviour. Three of these birds carried transmitters in the breeding season during the fires and in the previous breeding season. This offered opportunistic analysis of data from both periods to test for effects of smoke on the birds’ flight behaviour. The effects of particulate matter in the air of ≤2.5 microns in diameter (PM2.5) and covariates on flight status were investigated with a binomial generalised linear model with logistic link. The birds were more likely to fly when there were low levels of PM2.5 and the odds of flying decreased as density of PM2.5 increased at a rate of 0.202% per ug/m3. None of the sample birds died during or after smoke exposure, although their respiratory system might have been affected.
Introduction
In late 2019 and early 2020, there were extensive wildfires in the Australian Capital Territory (ACT) and surrounding New South Wales (NSW). These were part of a series of mega fires that burned around 5.8 million hectares of forest in south-east Australia (Boer and Resco de Dios Citation2020). Smoke from these wildfires extended into regions within eastern states far beyond fire-affected areas. Air quality in the ACT was severely impacted due to wildfire smoke from November 2019 to February 2020 (Environment Protection Authority Citation2020, Citation2021).
Exposure to high levels of particulate matter (PM) in smoke causes the most severe effects in terms of the overall health in humans (Jalaludin and Cowie Citation2012). Smoke from these fires affected large numbers of people, causing excess respiratory problems, cardiovascular problems, and deaths (Borchers Arriagada et al. Citation2020). It has been estimated that more than three billion vertebrates including 180 million birds were killed in these fires (WWF Australia Citation2020) and many animals probably suffer health effects from exposure to smoke (Engstrom Citation2010). Birds are significantly affected even after short duration of exposure to aerosolised particulates (Brown et al. Citation1997), and such health effects may impact on bird behaviours, including movement (Sanderfoot and Gardner Citation2021). Few studies have measured the sensitivity of wild birds to the effect of pollutants (Sanderfoot and Holloway Citation2017) and the lack of robust air pollution measurements has limited meta-analysis in such studies (Sanderfoot et al. Citation2021).
Air pollution was measured in our study area, providing an opportunity to assess Little Eagle (Hieraaetus morphnoides) movement behaviour during periods of measured smoke particulate levels. Standard methods for the assessment of the impact of air pollution on human health include monitoring of particulate matter of ≤2.5 microns (Cascio Citation2018; Borchers Arriagada et al. Citation2020) and the same levels are used in wildlife health (Lee et al. Citation2017; Sanderfoot and Gardner Citation2021). In the ACT, the daily maximum particulate matter level of 25 µg/m3 is the recognised standard by the National Environment Protection (Ambient Air Quality) Measure (AAQ NEPM) for particles of ≤2.5 microns (PM2.5) and this is monitored by the ACT Government Environment Protection Authority.
There has been a strong geographical bias towards the northern hemisphere on the impact of air pollution on terrestrial birds (95%) with no such studies in Australia (Barton et al. Citation2023). We had been studying the Little Eagle’s breeding ecology in the ACT (Rae et al. Citation2018; Rae Citation2021) and had fitted GPS transmitters to birds to record their movements, covering the period when the wildfire smoke affected the area and in the previous breeding season for a comparable period. Together with the records for particulate matter (Environment Protection Authority Citation2020, Citation2021), this presented an opportunity to assess the effect of smoke particles on Little Eagle flight behaviour during exposure to wildfire smoke as a measure of the sensitivity of birds to the effect of a pollutant.
Methods
The study area was in the ACT in south-eastern Australia (S. 35° 16' 55, E. 149° 7' 44), which is surrounded by the state of New South Wales (NSW). The study area was in the north of the territory, around the city of Canberra, the capital of Australia, and the habitat is peri urban with woodlands, some of which have been cleared for grazing. Much of the southern part of the territory is forested and there are extensive forests to the south and east in NSW.
Observations were done from vantage point watches over potential nest areas from August 2017 to February 2020 to watch for eagle flight behaviour. The birds were accustomed to human proximity as all nests were within 5 km of the urban edge and most were within 1 km of a sealed road (Rae et al. Citation2018), the closest being 25 m from a busy road. To establish occupancy and breeding success of a pair, all nests were checked with a telescope for freshly added nesting material and the presence of birds on or near the nest. Incubating or brooding birds were accepted as evidence of eggs or chicks in the nest. Young were considered to have fledged once they left the nest for their first flight. The main criteria recorded were: a nesting territory where there was a territorial pair with a nest, whether eggs were laid or young hatched, and number of young reared (Hayhow et al. Citation2017; Steenhof et al. Citation2017).
In 2018, three Little Eagles were fitted with GPS transmitters to study movement and breeding behaviour. All three birds were still carrying the transmitters in the 2019–2020 summer when the wildfire smoke affected the study area. All birds were adult males in breeding territories and the devices were active throughout the 2018–2019 and 2019–2020 breeding seasons. The Little Eagle breeding season in the ACT area is approximately August–February (Taylor and Canberra Ornithologists Group Citation1992). In mid-summer, when the area was affected by smoke, the birds were providing food for chicks, either in the nest or fledged and still dependent upon the adults for food.
The birds were fitted with Solar Argos/GPS backpack style transmitters (PTT-100, Microwave Telemetry Inc., Colombia, USA or GT-22GS-GPS, GeoTrak, North Carolina, USA) attached with a Teflon ribbon harness (Steenhof et al. Citation2006) (Animal ethics permit CEAE16–22, University of Canberra). The weight of each transmitter was 22 g. Transmitters weighed 3.52% or less of eagle body mass. The devices recorded location, time of fix, speed, direction of movement and altitude.
The birds’ movements were assessed by examining flight status at the time of each GPS fix. GPS fixes where speed was 0 km/h were given a ‘not flying’ flight status, and GPS fixes where speed was greater than 0 km/h were given a ‘flying’ flight status. To ensure that only observations occurring during daylight hours were included in the analysis, GPS fixes during civil twilight were removed. The data collection schedule varied with season to spread fixes over available daylight hours. Fixes were recorded daily in Australian Eastern Standard Time (AEST) at 07:00, 09:00, 10:00, 11:00, 13:00, 14:00, 15:00, 17:00 and 18:00 during November (Spring) and 05:00, 07:00, 09:00, 11:00, 13:00, 15:00, 17:00 and 19:00 during the summer months of December, January and February. Some days had fewer records due to GPS error. The full activity of the birds is not presented in this study, and the results do not give a full account of the birds’ movements.
Several covariates were expected to affect flight behaviour and these were tested for effect on probability of flight: study year (2018–2019 summer or 2019–2020 summer), individual (bird ID), breeding status (breeding or not breeding), date (week), temperature (°C), wind speed (km/h) and rainfall during previous hour (mm). Flight activity was expected to be higher during periods when the birds were supplying food for chicks, as with Red Kite Milvus milvus (Pfeiffer and Meyburg Citation2022). The probability of flying was also expected to be higher from late morning to afternoon when thermals are at their greatest for soaring birds, such as Lesser Spotted Eagle Clanga pomarina (Meyburg and Meyburg Citation2020), and when there was more lift from higher wind speeds (Pfeiffer and Meyburg Citation2022). Heavy precipitation was expected to inhibit birds hunting, as with Black Kite Milvus migrans (Sergio Citation2003).
The effects of PM2.5 and covariates on flight status were investigated with a binomial generalised linear model with logistic link, with the response being the binomial variable of flight status. Individual was included as a fixed rather than random effect due to the small number of three individuals GPS-tracked in the study. The three birds were identified as D2, X2 and Z5.
A second binomial generalised linear model was run using only the data from the 2019–2020 study year (i.e. the summer of the wildfires). This additional analysis was included to determine whether PM2.5 affected Little Eagle flight status similarly within and between study years. The model was run as described above, except with the covariate of study year removed.
The model was generated in R Studio (RStudio Team Citation2015, www.r-project.org) using the ‘stats’ package (www.r-project.org). Model validation was performed by using the ‘DHARMa’ package (Hartig Citation2022) to assess residuals, outliers and dispersion (KS test, P = 0.91, Dispersion test, P = 0.61, Outlier test, P = 1.00). To determine the importance of each fixed effect in the model, the drop1 command was used. AIC values from the drop1 output demonstrated that the optimal models for both the full dataset and the subsetted 2019–2020 study year dataset included all fixed effects. Therefore, no fixed effects were dropped from the model. The figures were created using the ‘ggplot2’ package (Wickham Citation2016). Differences in PM2.5, temperature, wind speed and rainfall (during previous hour) between the 2018–2019 and 2019–2020 summers were also evaluated with non-parametric permutation tests, using the ‘coin’ package in R (Hothorn et al. Citation2008). Temperature, wind and rainfall data were those recorded at Canberra Airport (Bureau of Meteorology Citation2022), which was within 20 km of the nest sites of all the tracked birds. Smoke particle density values PM2.5 are those recorded hourly for air quality at Canberra City which was within 15 km of the nests of all birds and 6 km from the airport (Environment Protection Authority Citation2020, Citation2021). The ambient air quality in the ACT is in accordance with the requirements of the National Environment Protection (Ambient Air Quality) Measure (AAQ NEPM) made by the National Environment Protection Council. Data from two other monitoring stations were referred to for maximum levels in the area, Florey and Monash, both of which were within 20 km of the Little Eagle nests.
Results
The air pollution levels dramatically increased in the ACT in late 2019 due to the very high levels of the wildfire smoke. The area experienced 59 days with extreme pollution between 23 November 2019 and 5 February 2020. In early January 2020, the particle pollution was at levels more than 20 times (PM10) or 45 times (PM2.5) the national standards. The nearest wildfire was in the ACT at approximately 27 km south-west of D2’s nest, 27 km from X2, 31 km from Z5, and 28 km from the city air quality monitoring station. The PM2.5 levels ranged 26.0–827.1 µg/m3 and the highest level recorded in the ACT was 1146.5 μg/m3 on 1 January 2020 at Monash, which was approximately 15 km from the nest of D2, 12 km from X2 and 18 km from Z5.
All three Little Eagles remained in their territories during the smoke period and the two that had chicks continued to provide food for them. Both chicks subsequently fledged. There were 5510 GPS records from the three Little Eagles between 1 November and 24 February in 2018–19 and 2019–2020. Individually, there were 1905 from D2, 1905 from X2 and 1700 from Z5. D2 did not have a chick in 2018–2019 but did rear one in 2019–2020. X2 reared a chick in 2018–2019 but not in 2019–2020. Z5 reared a chick in both seasons.
All predictors were retained in the models following the drop1 test as this determined that none could be removed (Supp Table S1; Supp Table S2). However, there was some support for alternative models (∆ AIC < 4; see Burnham et al. Citation2011, for further discussion on ∆ AIC), and the coefficients for supported alternative models are shown in Supp Table S3 and Supp Table S4. In 2018–2019, PM2.5 ranged from 0 to 314 µg/m3. In 2019–2020, PM2.5 ranged from 0 to 1372 µg/m3. On 1 January 2020, PM2.5 ranged from 382.9 to 1372.1 µg/m3, a difference of 1069 µg/m3 and the highest daily difference of PM2.5 recorded over the study period ().
Figure 1. The variation in recorded levels of smoke particle density values PM2.5 as a measure of air quality recorded at Canberra City during the two study periods, 2018–2019 and 2019–2020. The data points represent the hourly PM2.5 values recorded at the time of each GPS fix.
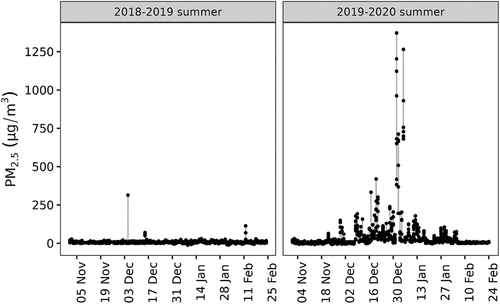
Little Eagle flying behaviour was negatively affected by the wildfire smoke. Both generalised linear models showed that birds were more likely to fly when there were low levels of PM2.5. The analysis of the combined 2018–2019 summer and 2019–2020 summer data showed that the odds of flying decreased by 0.202% per µg/m3 increase in the density of PM2.5 (Supp Table S5; ). This trend was also observed in the 2019–2020 summer-only data, with the odds of flying decreasing 0.185% per µg/m3 increase in density of PM2.5 (Supp Table S6).
Figure 2. The relationship between density of particulate matter (PM2.5) and model-fitted odds of a Little Eagle flying. The box-plots were generated for illustration only with the PM2.5 points grouped into discrete bins at increments of 200 centred on each scaled value. Analysis was done with the value for PM2.5 as a continuous variable.
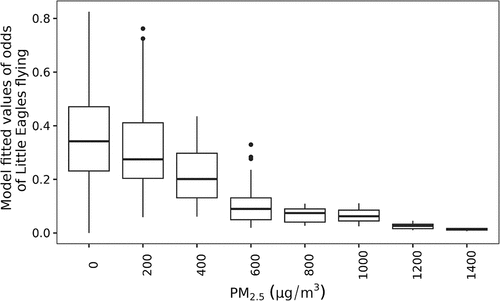
All other terms in the model were highly significant and in the expected directions (Supp Table S1). Temperature and wind speed had positive effects on the odds of Little Eagles flying, whereas rainfall and week had negative effects. There were lower odds of flying in 2019–2020 than in 2018–2019, which fits the hypothesis that the birds flew less in the year when there was intense smoke. Individual Z5 had the highest odds of flying, followed by D2, with X2 having the lowest odds of flying. These trends were observed in the model including all data (Supp Table S5) and the model including only the 2019/2020 summer data (Supp Table S6).
The non-parametric permutation tests showed that in the 2019–2020 summer, PM2.5 was significantly higher (Z = −15.4, P < 0.001), wind speed was significantly higher (Z = −11.073, P < 0.001) and rainfall was significantly lower (Z = 2.343, P = 0.019) than in the 2018–2019 summer. There was no significant difference in temperature between the two summers (Z = −0.992, P = 0.321).
The distance the birds ranged around their territories varied, as measured from the distribution of the GPS records. The maximum range from the nest of D2 was 13.6 km in 2019 (median 2.9 km) and 7.8 km in 2020 (median 0.8 km), X2 ranged up to 34.5 km in 2019 (median 1.1 km) and 49.0 km in 2020 (median 11.1 km), Z5 ranged up to 19.8 km in 2019 (median 0.9 km) and 20.6 km in 2020 (median 0.7 km).
Discussion
This opportunistic study of the effect of particulate matter (PM2.5) in wildfire smoke on the flying behaviour of a bird, the Little Eagle, has shown that the birds were more likely to fly when there were low levels of PM2.5. This finding was observed across two summers, one when there was a wildfire and one when there was not. The odds of flying were less likely during the summer that experienced wildfire, and a decrease in the odds of flying by 0.202% per µg/m3 increase in density of PM2.5. The trend was also observed within the summer that experienced wildfire, where the odds of flying decreased by 0.185% per µg/m3 increase in density of PM2.5. This negative correlation between PM2.5 and the odds of Little Eagles flying both between study years and within the study year affected by wildfires indicates that higher PM2.5 density reduces the odds of Little Eagles flying.
From observations of the birds during the study, it was expected that the eagles were more likely to fly during the late morning and early afternoon than at other times of the day. Eagles are known to soar on thermals for lift, and these begin to form mid-morning when the temperature increases. This was supported by the higher probability of flying when temperature was higher. Heat generated by the fire or smoke might have increased the temperature in the study area and hence lift for the birds’ flight. Any effect of this was not assessed in this post-hoc study, although there was no difference in temperatures between the two summers. The other form of lift in flight used by eagles is wind, so the higher probability of flying in stronger wind was as expected. Observations during the study led to the expectation that the birds seldom flew during rainfall or immediately afterwards, and this was confirmed as the effect of rain on the probability of eagles flying was the strongest of the predictors.
It is not known whether or how the birds were affected physiologically by the wildfire smoke. Nor was it known if the fire and smoke had any effect on potential prey species in the study area, which might have caused an increase in weak animals or carcasses for the eagles to feed upon. No sick or dead animals were found during the study, however, at a research facility in NSW, 32 km east of the Little Eagle study area, nine Smoky Mice Pseudomys fumeus died after exposure to smoke from the same fires in January 2020 that affected the ACT (Peters et al. Citation2021). All the mice had pulmonary lesions consistent with smoke inhalation, like those found in experimental murine exposure to acute wood smoke inhalation (Matthew et al. Citation2001). Dark brown to black particulate matter 0.5–2.5 µm were found associated with severe lesions in the lungs of four mice that were histologically examined. Yet there were no mortalities in parrots (unspecified) which were also housed at the facility, which suggests they had a different sensitivity to smoke inhalation (Peters et al. Citation2021).
In a study of a larger mammal, Bornean Orangutan (Pongo pygmaeus wurmbii), when exposed to wildfire and hazardous levels of PM10, there was no mortality (Erb et al. Citation2018). However, they were less active during and after the smoke period, travelling less and for shorter distances. This reduction in activity is similar to that found in this study where the Little Eagles flew less frequently as the smoke intensity increased.
Although none of the Little Eagles died during the study, it is not known if they suffered respiratory or cardiovascular problems as a result of smoke inhalation. Birds can be affected by smoke inhalation, such as Blue-fronted Amazon parrots Amazona aestiva aestiva which exhibited variable degrees of dyspnoea, breathlessness (Verstappen and Dorrestein Citation2005). Particulate matters can reduce pulmonary function in the Rock Dove Columba livia (Lorz and López Citation1997) and pigeons return home more quickly when in more polluted conditions (Li et al. Citation2016). It is highly possible that poor air quality caused by wildfire can cause respiratory distress and illness in birds (Sanderfoot and Holloway Citation2017). If the Little Eagles had been affected by smoke, that might give reason for the low probability of flight activity when the PM2.5 level was high.
Distance to wildfire and air quality are major factors that influence the number of bird mortalities related to wildfire (Yang et al. Citation2021). However, that study assessed the spatial pattern in a wide grid of units 25 km × 25 km and our study area was farther that 25 km from the nearest fire. There might have been some mortality closer to the fires which our study did not cover.
In conclusion, it is likely that high levels of particulate matter (PM2.5) caused a reduction in flight activity by Little Eagles during a period when the study area was affected by smoke from wildfires. It is also possible that the birds suffered some level of respiratory distress as the levels of PM2.5 were above the AAQ NEPM standard of 25 µg/m3 on 59 days in the latter part of their breeding season. If climate change with associated rising temperatures and dry weather continue, the frequency and intensity of wildfire events is likely to increase, and wildfire smoke would continue to impair air quality in the ACT (Environment Protection Authority Citation2020, Citation2021). Although this outcome might not cause death to Little Eagles, they would be further impacted upon and other birds, especially smaller species, could be impacted upon more severely. The authors recognise the limitations of this opportunistic study and suggest further work on such topics as potential effects of fire and smoke on prey species and heat on thermal lift to birds.
Supplemental Material
Download MS Word (20.2 KB)Supplemental Material
Download MS Word (20.3 KB)Supplemental Material
Download MS Word (20 KB)Supplemental Material
Download MS Word (20.1 KB)Supplemental Material
Download MS Word (18.6 KB)Supplemental Material
Download MS Word (18.8 KB)Acknowledgments
We acknowledge the Ngunnawal People as the Traditional Custodians of the land on which this study is undertaken and pay our respects to Elders past, present and future. We are grateful to landowners and managers who allowed access to nest sites and hunting areas. This study was part of a wider study by the Little Eagle Research Group and we thank other members of the group for help with fieldwork and comments on this report; these being Jacqui Stol (CSIRO Land and Water, Black Mountain), Michael Mulvaney (Rtd. Conservation Research Unit, ACT Government), David Roberts (Ginninderry Joint Venture), Penny Olsen (Research School of Biology, Australian National University) and Don Fletcher.
Disclosure statement
No potential conflict of interest was reported by the author(s).
Supplementary material
Supplemental data for this article can be accessed at https://doi.org/10.1080/01584197.2023.2267609
References
- Barton, M. G., Henderson, I., Border, J. A., and Siriwardena, G. (2023). A review of the impacts of air pollution on terrestrial birds. Science of the Total Environment 873, 162136. doi:10.1016/j.scitotenv.2023.162136
- Boer, M. M., and Resco de Dios, V. (2020). Unprecedented burn area of Australian mega forest fires. Nature Climate Change 10, 170. doi:10.1038/s41558-020-0716-1
- Borchers Arriagada, N., Palmer, A. J., Bowman, D. M. J. S., Morgan, G. G., Jalaludin, B. B., and Johnston, F. H. (2020). Unprecedented smoke-related health burden associated with the 2019–20 bushfires in eastern Australia. Medical Journal of Australia 213, 282–283. doi:10.5694/mja2.50545
- Brown, R. E., Brain, J. D., and Wang, N. (1997). The avian respiratory system: A unique model for studies of respiratory toxicosis and for monitoring air quality. Environmental Health Perspectives 105, 188–200. doi:10.1289/ehp.97105188
- Bureau of Meteorology - Australian Government (2022). ‘Climate data online.’ Available at http://www.bom.gov.au/climate/data/.
- Burnham, K. P., Anderson, D. R., and Huyvaert, K. P. (2011). AIC model selection and multimodel inference in behavioural ecology: Some background, observations, and comparisons. Behavioral Ecology and Sociobiology 65, 23–25.
- Cascio, W. E. (2018). Wildland fire smoke and human health. Science of the Total Environment 624, 586–595. doi:10.1016/j.scitotenv.2017.12.086
- Engstrom, R. T. (2010). First-order fire effects on animals: Review and recommendations. Fire Ecology 6, 115–130. doi:10.4996/fireecology.0601115
- Environment Protection Authority (2020). ACT air quality report 2019. Canberra: ACT Government.
- Environment Protection Authority (2021). ACT air quality report 2020. Canberra: ACT Government.
- Erb, W. M., Barrow, E. J., Hofner, A. N., Utami-Atmoko, S. S., and Vogel, E. R. (2018). Wildfire smoke impacts activity and energetics of wild Bornean orangutans/631/158/856/631/158/672/631/158/2465/631/601/18/631/601/1737 article. Scientific Reports 8, 1–8. doi:10.1038/s41598-018-25847-1
- Hartig, F. (2022). ‘Dharma: Residual diagnostics for hierarchical multi-level/mixed) regression models.’ Available at http://florianhartig.github.io/DHARMa/.
- Hayhow, D. B., Benn, S., Stevenson, A., Stirling-Aird, P. K., and Eaton, M. A. (2017). Status of Golden Eagle Aquila chrysaetos in Britain in 2015. Bird Study 64, 281–294. doi:10.1080/00063657.2017.1366972
- Hothorn, T., Hornik, K., Wiel, M. A., and Zeileis, A. (2008). Implementing a class of permutation tests: The coin package. Journal of Statistical Software 28, 1–23. doi:10.18637/jss.v028.i08
- Jalaludin, B., and Cowie, C. (2012). ‘Health risk assessment – preliminary work to identify concentration-response functions.’ Available at https://www.nepc.gov.au/sites/default/files/2022-09/health-report.pdf.
- Lee, B. P. Y. H., Davies, Z. G., and Struebig, M. J. (2017). Smoke pollution disrupted biodiversity during the 2015 El Niño fires in Southeast Asia. Environmental Research Letters 12, 094022. doi:10.1088/1748-9326/aa87ed
- Li, Z., Courchamp, F., and Blumstein, D. T. (2016). Pigeons home faster through polluted air. Scientific Reports 6, 1–6. doi:10.1038/srep18989
- Lorz, C., and López, J. (1997). Incidence of air pollution in the pulmonary surfactant system of the pigeon (Columba livia). The Anatomical Record 249, 206–212. doi:10.1002/(SICI)1097-0185(199710)249:2<206:AID-AR7>3.0.CO;2-V
- Matthew, E., Warden, G., and Dedman, J. (2001). A murine model of smoke inhalation. American Journal of Physiology - Lung Cellular and Molecular Physiology 280, 716–723. doi:10.1152/ajplung.2001.280.4.L716
- Meyburg, B.-U., and Meyburg, C. (2020). Minimum distances and shutdown times for wind turbines to protect the lesser spotted Eagle (Clanga pomarina) – recommendations based on GPS telemetry results. Berichte zum Vogelschutz 57, 113–136.
- Peters, A., Hume, S., Raidal, S., Crawley, L., and Gowland, D. (2021). Mortality associated with bushfire smoke inhalation in a captive population of the smoky mouse (Pseudomys fumeus), a threatened Australian rodent. Journal of Wildlife Diseases 57, 199–204. doi:10.7589/JWD-D-20-00026
- Pfeiffer, T., and Meyburg, B. U. (2022). Flight altitudes and flight activities of adult Red Kites (Milvus milvus) in the breeding area as determined by GPS telemetry. Journal of Ornithology 163, 867–879. doi:10.1007/s10336-022-01994-1
- Rae, S. (2021). ‘The breeding ecology and movements of the Little Eagle in the ACT and nearby NSW, 2017–2020 with reference to the Ginninderry development area.’ Available at https://ginninderry.com/wp-content/uploads/2021/08/LE-report-2017-2020-Ginninderry70.pdf.
- Rae, S., Fletcher, D., Mulvaney, M., Davies, M., Roberts, D., and Olsen, P. (2018). Notes on the breeding ecology of Little Eagles in the ACT in 2017/2018. Canberra Bird Notes 43, 186–193. Available at https://www.researchgate.net/publication/325896593_Notes_on_the_breeding_ecology_of_Little_Eagles_in_the_ACT_in_20172018.
- RStudio Team. (2015). ‘Rstudio: Integrated development for R.’ Available at http://www.rstudio.com/.
- Sanderfoot, O. V., Bassing, S. B., Brusa, J. L., Emmet, R. L., Gillman, S. J., Swift, K., and Gardner, B. (2021). A review of the effects of wildfire smoke on the health and behavior of wildlife. Environmental Research Letters 16, 123003. doi:10.1088/1748-9326/ac30f6
- Sanderfoot, O. V., and Gardner, B. (2021). Wildfire smoke affects detection of birds in Washington State. Ornithological Applications 123, 1–14. doi:10.1093/ornithapp/duab028
- Sanderfoot, O. V., and Holloway, T. (2017). Air pollution impacts on avian species via inhalation exposure and associated outcomes. Environmental Research Letters 12, 083002. doi:10.1088/1748-9326/aa8051
- Sergio, F. (2003). From individual behaviour to population pattern: Weather-dependent foraging and breeding performance in Black Kites. Animal Behaviour 66, 1109–1117. doi:10.1006/anbe.2003.2303
- Steenhof, K., Bates, K. K., Fuller, M. R., Kochert, M. N., Mckinley, J. O., and Lukacs, P. M. (2006). Effects of radiomarking on Prairie Falcons: Attachment failures provide insights about survival. Wildlife Society Bulletin 34, 116–126. doi:10.2193/0091-7648(2006)34[116:EOROPF]2.0.CO;2
- Steenhof, K., Kochert, M. N., McIntyre, C. L., and Brown, J. L. (2017). Coming to terms about describing Golden Eagle reproduction. Journal of Raptor Research 51, 378–390. doi:10.3356/JRR-16-46.1
- Taylor, M., Canberra Ornithologists Group, and Australia. National Capital Planning Authority (1992). 'Birds of the Australian Capital Territory : An Atlas/McComas Taylor and Canberra Ornithologists Group.' (Canberra Ornithologists Group and the National Capital Planning Authority: Canberra.)
- Verstappen, F. A. L. M., and Dorrestein, G. M. (2005). Aspergillosis in Amazon parrots after corticosteroid therapy for smoke-inhalation injury. Journal of Avian Medicine and Surgery 19, 138–141. doi:10.1647/2002-029
- Wickham, H. (2016). ‘ggplot2: Elegant Graphics for data analysis.’ Available at https://ggplot2.tidyverse.org.
- WWF Australia (2020). ‘Australia’s 2019–2020 bushfires: The wildlife toll.’ Available at https://assets.wwf.org.au/image/upload/Animals_Impacted_Interim_Report_24072020_final?_a=ATO2Ba20.
- Yang, D., Yang, A., Yang, J., Xu, R., and Qiu, H. (2021). Unprecedented migratory bird die-off: A citizen-based analysis on the spatiotemporal patterns of mass mortality events in the western United States. GeoHealth 5, e2021GH000395. doi:10.1029/2021GH000395