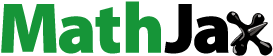
ABSTRACT
Quantifying nest success for seabirds breeding in remote offshore islands can be logistically challenging, especially for species with protracted breeding phenologies. Thus, any monitoring program must be robust to these challenges, but few studies have reported evaluations of survey design, monitoring frequency, and methods for estimating nesting success for such species. We explored whether the current monitoring program for Red-tailed Tropicbirds (Phaethon rubricauda) on Raine Island, Great Barrier Reef, Australia, enabled accurate estimates of nesting success. We use three different approaches to produce the first estimates of nesting success from data collected by this monitoring program. Annual nesting success for Red-tailed Tropicbirds was low at Raine Island, estimated as between 24.3% and 30.6%, indicating that further study is warranted to identify possible causes. However, our study illustrates possible pitfalls of the monitoring program, and we propose three critical considerations (maximising per-nest observations, standardising timing of visits, and using automated technologies) that would improve the ability of monitoring programs to estimate nesting success for seabirds with protracted breeding phenologies. Use of such modifications in monitoring programs will improve our ability to diagnose the causes of population declines for seabirds in the Great Barrier Reef.
Introduction
Seabirds are globally threatened, with almost one-third of species facing imminent extinction (Croxall et al. Citation2012; BirdLife International Citation2018). Seabirds are upper trophic-level predators, and metrics of their reproduction and population status can reflect a changing marine environment (Congdon et al. Citation2007; Parsons et al. Citation2008). Accurate monitoring of seabirds is difficult, as many species are long-lived, have extended nesting periods, and breed on difficult-to-access offshore islands (Bird et al. Citation2021b; Woodworth et al. Citation2021).
Monitoring abundance alone may not give a full picture of the status of a population, since impacts from low reproductive output may take decades to become apparent in the numbers of nesting adults. A useful additional approach to understanding the health of seabird populations is evaluating nesting success. Nesting success, or the probability that a nest survives to fledge at least one offspring (Rotella et al. Citation2004), is a frequently used metric to evaluate the status of avian populations (Johnson Citation1979; Manolis et al. Citation2000; Jehle et al. Citation2004; Rotella et al. Citation2004). Nesting success is a sensitive indicator of environmental or temporal factors affecting a population and can be used to evaluate the impact of threats, management, or conservation actions (Manolis et al. Citation2000; Jehle et al. Citation2004).
The Great Barrier Reef (GBR) hosts breeding populations of 20 seabird species (Congdon Citation2019), with many populations declining despite the recognition of seabirds as important indicators in the Reef 2050 Long-term Sustainability Plan (Australian Government Citation2021). To address declines, the Reef 2050 Objectives and Goals aim to expand the monitoring focus on seabirds to reproductive success (DAWE Citation2021). Specifically, the Reef 2050 Objectives and Goals target measures of fecundity, recruitment, and adult and chick survivorship rates as future indicators of the health of seabird populations (DAWE Citation2021).
Raine Island is the largest shelf edge vegetated cay (27 hectares) in the Northern GBR, situated ~100 km offshore from Cape Grenville (DES Citation2021), and harbours the most significant seabird breeding colony in the GBR, with populations of 17 of the recorded 24 seabird species that breed on Queensland islands (Warham Citation1961; King Citation1993; Claridge and WBM Oceanics Australia Citation1997; Cornelius Citation2010; DES Citation2017). Raine Island has the only major breeding population of Red-tailed Tropicbirds (Phaethon rubricauda) in the GBR (Serventy et al. Citation1971; Tets van and Fullagar Citation1984; Marchant and Higgins Citation1990). The continued health of the Raine Island tropicbird population is key to ensuring the persistence of this species in the GBR as a whole.
The Queensland Government Department of Environment and Science (DES) has been conducting monitoring of active nests of Red-tailed Tropicbirds at Raine Island since 1995, and under current methods since 2015, to determine breeding population numbers. Since 2016, artificial nest boxes have also been implemented as a trial to mitigate possible disturbances to the nesting population. Accurately estimating the nesting success of Red-tailed Tropicbirds on Raine Island directly aligns with the strategic directions outlined in the Reef 2050 Objectives and Goals. It is therefore important to test the efficacy of current monitoring and identify whether accurate and reliable estimates of nesting success can be calculated from surveys that vary in their timing and number each year.
The challenges with measuring nesting success of offshore birds include the remote nature of their habitat and the logistical complexity of visiting these sites. Additionally, Red-tailed Tropicbirds have an extended nesting period of 105–145 days (Fleet Citation1974; Diamond Citation1975b; Marchant and Higgins Citation1990; Schreiber and Schreiber Citation1993; Vanderwerf and Young Citation2014), making it highly impractical to survey the entirety of their nesting period from on-the-ground surveys alone.
The current monitoring schedule of Red-tailed Tropicbirds on Raine Island is three surveys a year, two summer surveys and one winter survey, with further opportunistic monitoring occurring where possible. To date, there has been no evaluation of the current monitoring schedule and methods, or the data it produces, to assess if the current program is suitable for determining nesting success for Red-tailed Tropicbirds. Therefore, this offers the perfect opportunity to investigate the suitability of a critically important monitoring program for tracking the health of an important GBR seabird and quantify the challenges of logistical constraints for robust nest survival estimates.
Here, we aimed to:
compare the suitability and performance of different analytical approaches for estimating nesting success from current monitoring programs,
identify whether the current monitoring program creates any sampling bias that would reduce the reliability of nesting success estimates,
suggest methodological improvements for monitoring the reproductive health of offshore seabird populations using nesting success estimates and identifying factors influencing the nesting success of a population, and
produce first estimates of nesting success for the most important population of Red-tailed Tropicbirds in the GBR.
Methods
Field surveys
Multi-purpose survey trips to Raine Island (coordinates: 11° 35’ 26.26” S, 144° 2’ 6.66” E) were conducted by DES between 2008 and 2021, totalling between one and five surveys per year, at least 1 month apart (supplementary material S1). Between 2008 and 2021 there were 36 visits to Raine Island where detailed nest surveys were conducted (in surveys from 2011 to 2014 life stage of the offspring was not recorded). Monitoring of Red-tailed Tropicbird nests followed Raine Island standard monitoring procedures (supplementary material S2). Surveyors recorded the GPS location and the contents of all accessible nests on the island, which included the number of adults, the presence/absence of an egg or offspring, and the life stage of the offspring recorded as either a newly hatched chick, a young chick, or an adolescent (an offspring of fledging age), according to observations of their plumage (Fleet Citation1974). Surveys after 2015 included the monitoring of ten nest boxes deployed on Raine Island in 2016 by DES.
Red-tailed Tropicbird nesting phenology
We visualised the average total nesting attempts across each month to determine peak nesting times. We also explored occupancy rates of nest boxes, but there was insufficient data to calculate nesting success of Red-tailed Tropicbirds that used artificial nest boxes; therefore, we were unable to make comparisons between artificial and natural nests.
Data preparation
Defining unique nesting attempts
We defined three terms for this study, a nest observation (a single observation of a nest), a nesting attempt (all observations of a nest within one breeding attempt), and the nest period (the length of a typical Red-tailed Tropicbird nesting cycle from incubation to fledging). Previous studies on the biology of Red-tailed Tropicbirds were used to determine critical periods for calculating unique nesting attempts. Red-tailed Tropicbirds have an average incubation of 45-days (Schreiber and Schreiber Citation1993), and a fledging age between 65 and 100 days (Fleet Citation1974; Diamond Citation1975b; Marchant and Higgins Citation1990; Schreiber and Schreiber Citation1993; Vanderwerf and Young Citation2014). We therefore used a maximum nesting period of 145 days for this study.
Using the maximum nesting period, a nest observation was included in the previous nesting attempt if it did not exceed the maximum interval since the first observation (145 days) and was not identified as a ‘replacement egg’ (the observed life stage indicated that the offspring had died and been replaced by a new nesting attempt).
Determining nest age and designating nest outcomes
The age of the initial observation for a nesting attempt was determined as the minimum age (days) of the offspring’s observed life stage (incubation, chick, young, adolescent) as defined by Fleet (Citation1974). The age of the nest for additional observations within the same nesting attempt was calculated by adding the total interval time (number of days) since the first observation. The minimum age for each life stage was assigned when determining nest ages because this ensured that all relevant observations were captured within each nest period.
Each nest period was assigned a nest outcome according to the following conditions:
Successful: final observation of a nesting attempt is an adolescent bird of fledging age (nest age of 105–145 days).
Failed: a dead chick is found, or the nest is empty after a previous observation of a live chick within the same nesting period but before fledging age, or a subsequent observation indicates a replacement egg.
Unknown: all other observations.
Exploring the influence of survey design on the detectability of nest outcomes
A potential source of bias that may cause an overestimation of nesting success was identified in the Raine Island survey design. Specifically, to designate a nest period as ‘successful’ or ‘unknown’, only a single observation is required. However, a failed nest requires at least two observations, one confirming the presence of an egg or chick, and a following observation confirming its absence prior to a plausible fledging date. To explore the impact of this potential bias, a multinomial regression using the ‘nnet’ package (Venables and Ripley Citation2002) run through RStudio (V.4.0.3) was used to determine how the probability of detecting a successful or unknown nest differed from the probability of detecting a failed nest, and how this was affected by the number of observations per nesting attempt and the interval length between surveys. From this analysis, the odds ratio for each variable was calculated by exponentiating the coefficients. The effect size of the variables was calculated as the odds ratio minus one. The significance of the effect size was determined using a two-tailed Wald z-test with a 0.05 significance level.
The results of this analysis indicated whether having different numbers of observations led to overestimating nesting success and if standardising observation number was required before further analysis (i.e. by removing any nest periods that had only a single observation).
Nesting success
We calculated ‘hatching success’ (total hatched eggs divided by total confirmed nesting attempts) and ‘fledging success’ (total successful nesting attempts divided by total hatched eggs) of the population. We also calculated three proxies for ‘nesting success’: the apparent success, the Mayfield success, and the Mayfield success implemented in Program MARK.
Apparent nesting success
Apparent nesting success is the ratio of successful nesting attempts to total observed nesting attempts. Apparent nesting success generally overestimates success due to the positive bias inherent from the fact that nests that survive for longer periods are more likely to be included under the current protocol and also more likely to succeed (Mayfield Citation1961, Citation1975; Jehle et al. Citation2004).
Mayfield method
The Mayfield method is an alternative measure of nesting success that addresses the bias of the apparent method (Mayfield Citation1961, Citation1975). The Mayfield method records the number of days that a nest survives (i.e. is not recorded as ‘failed’), termed ‘exposure days’, and calculates the ratio of ‘total number of recorded failed nests’ to ‘the total sum of exposure days’. This is the probability that a nest survives from one survey day to the next: ‘daily survival rate’ (DSR):
The DSR considers each day that a nesting attempt survived, rather than only considering successful nests, and therefore reduces the bias of the apparent method. The DSR is then used to calculate the nesting success, by exponentiating the DSR across the nesting period for the species:
To calculate the Mayfield nesting success, several parameters were defined. The total number of failed nests was determined using the nest outcome rules defined above. Exposure days for each nesting attempt were determined as the total interval (number of days) from the first to last observation. A 125-day nesting period was used: the midpoint of the fledging age for Red-Tailed Tropicbirds (Fleet Citation1974; Diamond Citation1975b; Marchant and Higgins Citation1990; Schreiber and Schreiber Citation1993; Vanderwerf and Young Citation2014).
The Mayfield method assumes that nest outcomes occur at the mid-point between the penultimate and final observation when the exact date is unknown (Mayfield Citation1961, Citation1975). We set interval times (number of days between two observations within a nesting attempt) as 40% of the actual interval for the final two nest observations, as this method is preferred when intervals exceed 15-days (Miller and Johnson Citation1978; Jehle et al. Citation2004) and prevent overestimating exposure days and inflating DSR (Miller and Johnson Citation1978; Johnson Citation1979).
We excluded unknown fate nest outcomes (~70% of the full dataset) from our Mayfield calculations. Excluding unknowns avoids the strong positive bias that occurs when greater than 40% of the data are unknown because exposure days are overinflated without adding any additional failures (Manolis et al. Citation2000). Excluding unknowns also allowed for a more direct comparison with our other estimates of nesting success which do not allow the inclusion of unknown fate data.
MARK analysis
Finally, we calculated the Mayfield method through program MARK (White and Burnham Citation1999) using the RStudio package ‘Rmark’ (Laake Citation2013). MARK analysis is advantageous as it removes the assumption of the traditional Mayfield method that nesting success is constant between nesting attempts (Dinsmore et al. Citation2002), and provides a standard error for the Mayfield estimator (Rotella Citation2021).
Data for MARK analysis was organised following Laake and Rexstad (Citation2021), setting the number of occasions (the number of sampling days) as the number of unique dates (survey dates), and specifying the intervals between unique dates using the ‘time.intervals’ argument of Dinsmore et al. (Citation2002).
To test the constant survival assumption of the Mayfield method, MARK requires characterisation of the relevant covariates that may influence nesting success. We evaluated three grouping variables, and all combinations of these grouping variables (six grouping scenarios in total). The three grouping variables evaluated were:
Nesting time: whether the nesting attempt was recorded in the first, second, or final third (by number of days) of the calendar year. To evaluate if success was constant across the year.
Life stage: whether the nesting attempt was first recorded pre- or post-hatching. To evaluate if success changes after hatching.
Year: which year the nesting attempt was first recorded. To evaluate if success varied between years.
Each grouping variable in turn was evaluated against each of seven other models:
Linear time: success over time (days). To evaluate whether success followed a linear trend through the calendar year.
Quadratic time: time variable squared. To evaluate whether success followed a quadratic trend throughout the calendar year.
Distance (m) to the nearest active nest: to evaluate intraspecific competition.
Number of active nests within five metres: a second variable for intraspecific competition.
The interaction of 3 and 4.
The interaction of the relevant grouping variable with 3 and 4.
Null model: maintaining the constant survival assumption.
For each grouping scenario, we determined whether the result was reliable by inspecting the real estimates (DSR), the standard error of the real estimates, the upper and lower confidence intervals, and the sample sizes of the groups produced by MARK for the grouping variable in question. If a grouping variable resulted in unrealistic results, e.g. 100% survival, no/low standard error, overly high or low confidence intervals, or small sample sizes, it was considered unreliable and dropped from consideration.
Overall model comparisons were performed by using the Akaike Information Criterion corrected for small sample sizes (AICc) to rank competing models (Burnham and Anderson Citation2007). Beta estimates of the top-performing model were back-transformed to real estimates. These real estimates (DSR) were used in EquationEquation 2(2)
(2) to produce the nesting success across the nesting period. Nesting success was calculated for each group and/or covariate and as an overall nesting success across all groups/covariates in the model.
Results
Red-tailed Tropicbird nesting phenology
A total of 1,779 nesting attempts were documented. The inconsistency in data collection over time (Supplementary material S1) makes it difficult to determine nesting phenology accurately, though the highest average nesting appears to occur in the middle of the calendar year ().
Exploring the influence of survey design
A multinomial regression analysis on all nests confirmed that both successes (P-value = 0.004×10−1) and unknowns (P-value = <0.001×10−5) were significantly more likely to be detected than failures (). The probability of detecting a success compared to a failure was significantly negatively affected by the number of observations per nesting attempt (P-value = 0.023). The probability of detecting a success compared to a failure decreased by 45% with one extra observation per nesting attempt and increased by 0.6% with each extra interval day. The probability of detecting an unknown compared to a failure was significantly negatively affected by the number of observations (P-value = 0.002×10−5) and significantly positively affected by the interval time between surveys (P-value = 0.002) (). The probability of detecting an unknown fate compared to a failure decreased by 82% with an extra observation per nesting attempt and increased by 1.5% per interval. These results indicate that the Raine Island monitoring program is strongly biased towards detecting successful and unknown nests, due to the significant effect of the number of observations on the probability of detecting a successful nest compared to a failed nest.
Table 1. Multinomial regression model comparing the log odds of detecting a successful or unknown fate Red-tailed Tropicbird nest with those of detecting a failed nest. Sign of coefficients indicates direction of the relationship. The effect size represents the change in odds for that coefficient as compared to the odds of detecting the intercept (failures).
Therefore, we standardised observation number in our data set by excluding nesting attempts that had only a single observation and also excluded all unknowns (). Further, we included only surveys from 2015 to 2019 inclusive because these had a minimum of 4 surveys within the year (supplementary material S1). All subsequent analyses used this final data set ().
Table 2. Sample sizes across years, and the outcome of nesting attempts (F = Failed, S = Successful, U = Unknown) for Red-tailed Tropicbirds breeding on Raine Island. Sample sizes and nesting attempts shown for: (i) The full data set, (ii) A data set including only nesting attempts for which more than one observation was recorded, and (iii) The final data used to calculate estimates of nesting success.
Nesting success
Our final dataset resulted in hatching success of 39.3%, fledging success of 77.8%, apparent nesting success of 30.6%, and Mayfield nesting success across the 125-day nesting period of 24.3% ().
Table 3. Nesting success estimates for Red-tailed Tropicbirds breeding on Raine Island, derived from the full, reduced, and final dataset. ANS = apparent nest success (the ratio of successful to total nests), Mayfield = nest survival probability across breeding period, MARK = Mayfield nest success across breeding period calculated using top-ranked model in MARK. Final dataset includes optimal years, nests with >1 observation.
The Program MARK analysis gave a nest survival probability across the 125-day period of 30.1% (±6.7%; ), calculated from the final dataset and the top-ranked model (). The model with the life stage grouping variable was the best-performing model (MARK Nest Model, Parameters = 2, AICc = 145.02, Weight = 0.56; ). Survival estimates from incubation to fledging and chick to fledging were 10.0% (95% CI: 4.7–17.6%) and 91.8% (95% CI: 71.1–97.9%), respectively.
Table 4. Nest survival models for Red-tailed Tropcbirds breeding on Rain Island. Results show the most parsimonious, competing nest survival models from MARK, ranked by AICc. The model that grouped nests by life stage was the top-performing model (S(~group)). Table includes beta and real estimates from the top-performing model (life stage). Real estimates were calculated using EquationEquation (1)(1)
(1) . EquationEquation (2)
(2)
(2) can be used to calculate the nest survival probability (nest success) using the real estimates as the daily survival rate.
Of the six grouping scenarios, all except the life stage and nesting time models were disregarded due to issues with low sample size (Supplementary material S3). In the grouping scenario for nesting time, the quadratic time model was the highest ranked but was outperformed by the life-stage model when this was set as the grouping variable. Therefore, the life-stage model was taken as the overall best-performing model and used to determine the final results for nesting success according to MARK analysis ().
Discussion
Nesting success
Our three methods for estimating nest survival indicated that nesting success of Red-tailed Tropicbirds at Raine Island was between 20% and 40%. We also estimated hatching success of 39.3%, and a fledging success of 77.8%. Our Mark analysis also shows that nesting success increases after the incubation period () suggesting that most nest losses occur during the incubation stage. Of the three methods, the estimate using Program MARK is expected to be the most reliable as it incorporates the variability of the data into the calculations (Rotella Citation2021) and addresses the assumption of constant survival of the standard Mayfield estimator (Dinsmore et al. Citation2002).
Our estimates indicate that nesting success of Red-tailed Tropicbirds at Raine Island is restricted and that most losses occur during the incubation stage. Our estimates are similar to other Red-tailed Tropicbird colonies where predation is recorded. On Kure Atoll, North-western Hawaiian Islands, apparent nesting success was reported as 38% and 17% in 1964 and 1965, respectively (Fleet Citation1974). On Easter Island, Chile, it was 37% and 26% in 2014 and 2015, respectively (Flores et al. Citation2017). Red-tailed Tropicbirds at Midway Atoll achieved 38% and 41% success in 1989 and 1991, respectively, before rats were eradicated (Tyler Citation1991), although this estimate was similar (41%) in 1997 and 2008 after rats were eradicated (Laniawe Citation2008). Our estimate is also similar to that of Christmas Island, Australia, which had a comparatively low apparent nesting success of 33.3% between 1984 and 1986, despite fairly low levels of predation (Sommerfeld et al. Citation2015). Where predators are controlled or absent Red-tailed Tropicbirds typically achieve a higher nesting success, ranging from 42% to 85% (Prys-Jones and Peet Citation1980; Schreiber Citation1994; Felis et al. Citation2019; Vanderwerf Citation2021). There are no invasive predators on Raine Island, though there are potential avian species that could predate eggs or chicks. A predation event by a Buff-banded Rail (Hypotaenidia philippensis) on a Red-tailed Tropicbird egg was captured in a camera survey at Raine Island in 2020 (Robertson pers. obs.).
In addition to predation, further research is required on the impact of inbreeding depression on egg viability (Egerton et al. Citation2022), and heat stress (Morrell and Aquilani Citation2000). Red-tailed Tropicbirds also co-inhabit the island with the world’s largest remaining green turtle (Chelonia mydas) nesting population, which may damage or disturb tropicbird nests during peak breeding times for turtles (Stewart et al. Citation2021).
Assessing the suitability of the current monitoring program
We found that a difference in the methodology used to detect a successful compared to a failed nest created substantial bias in nest estimates derived from the original, complete dataset. Nesting attempts with a single observation constitute 74.4% of this dataset and can only produce successes or unknowns, as two observations are required to demonstrate nest failure. This bias is exasperated by generally large intervals between the survey times, generally caused by inconsistent and opportunistic survey dates. With large intervals between surveys, the probability of recording a successful or unknown fate nest increases (), as we are less likely to capture a second observation for a nesting attempt that is required to confirm a failed nest. In this way, the probability of detecting each nest outcome is directly related to the monitoring program.
The final dataset (used to produce our estimates of nesting success) standardises the number of observations per nesting attempt by removing nesting attempts with only a single observation, thereby decreasing the number of successful nests without changing numbers of failed nests (which can only be detected with multiple observations).
Each year in the final data has a similar number of surveys, yet there is heterogeneity between years in the proportion of nesting attempts with a single observation. Single observations comprised approximately 70% of the data in years 2015 and 2016, with 2017, 2018, and 2019 having upwards of 90%. This difference is caused by the later years having larger intervals between surveys, and also likely the survey date. Years 2015 and 2016 appear to capture peak laying periods better than the later years (supplementary material S1), so increasing sampling, particularly in these periods, would reduce data loss.
The MARK analysis () suggests that the true mean estimates of the nesting success likely fall between 17.7% and 41.5% (the 95% confidence intervals). These wide confidence intervals are a result of the small sample size, which has important implications for management. The highest mean estimate is comparable to that achieved by several stable nesting populations (Laniawe Citation2008; Sommerfeld et al. Citation2015), while the lowest mean estimate indicates levels likely below stability. These results demonstrate the importance of improving the monitoring program to increase the precision of these estimates. Our MARK analysis also allowed us to estimate separate survival rates for nests found at the incubation stage (4.7–17.6%) and those found after the incubation stage (71.1–97.9%). The range within these estimates shows that a large amount of variation is unaccounted for in the model and thus suggests the current monitoring program is not sufficient to capture sources of variation in the data.
The monitoring program had adverse consequences for understanding causes of poor nesting success. Potentially important grouping variables were dropped from the MARK analysis due to issues from small sample sizes (supplementary material S3), that may have helped explain the variation in our survival estimates. Small sample sizes occurred chiefly because of (i) a lack of repeated visits during a nesting attempt and insufficient visits within certain years, (ii) an inability to determine nest fate (a large number of nests with unknown fates had to be removed from analysis), and (iii) the need to group nests by stage/year or other factors to provide management-relevant analyses further reduced effective samples sizes. As a result, it was not possible to examine interannual and intra-annual variation in nest success, which we believe would have explained much of the variation in our estimates. For example, nesting phenology could explain changes in breeding success caused by the non-linear change in breeding numbers within a year (; King Citation1986; Taplin and Blaber Citation1993; Batianoff and Cornelius Citation2005), and annual variability in nesting success could be caused by fluctuations in the number of breeding turtles visiting the island between years (Dunstan and Robertson Citation2017, Citation2019), or changes in food availability caused by ENSO events (Schreiber Citation1994).
Assumptions of the current monitoring program
The existing monitoring program necessitated making several key assumptions including: (1) failure date is 40% between the penultimate and last observation, (2) age of nests at discovery is the median age of the observed life stage, and (3) birds observed at an age old enough to fledge will definitely fledge. The first two assumptions directly influence the Mayfield estimate as they affect the number of exposure days contributed to the Mayfield equation (EquationEquation 1(1)
(1) ), and the third assumption may overestimate the number of successful nests. With more observations per nesting attempt, the estimated failure date shifts closer to the true failure date, reducing the influence of the second assumption while also reducing the number of nests with an unknown fate. Maximising the number of observations per nesting attempt is critical but is challenged by physical access to remote breeding sites.
Implications for monitoring design
Survey trips to Raine Island are limited by cost and logistics and this has important consequences for collecting reliable and useful nesting information on seabirds, such as Red-tailed Tropicbirds. Additionally, the extended breeding period of Red-tailed Tropicbirds (Fleet Citation1974; Diamond Citation1975b; Marchant and Higgins Citation1990; Schreiber and Schreiber Citation1993; Vanderwerf and Young Citation2014) makes it challenging to capture the entirety of a nesting period from on-the-ground surveys alone. These limitations cause a large proportion of data collected to be unusable for estimating nesting success, which undermines the cost and effort to conduct these surveys. Although we have determined a snapshot of breeding success for Raine Island Red-tailed Tropicbirds (from 2015 to 2019), we are unable to identify changes in nesting success as it occurs, restricting the capacity for adaptive management. These limitations are especially important for conservation management at Raine Island because there are not yet sufficient data for a statistical comparison of nesting success between artificial or natural nest sites, despite these being installed in 2016.
Suggestions for improving monitoring
We suggest several improvements for monitoring programs that apply not only to Red-tailed Tropicbirds but other seabirds that breed on remote islands with protracted breeding phenologies: (i) maximising per-nest observations, (ii) standardising timing of visits, and (iii) use of the automated technologies. Ideally, a survey should begin with a random subset of nests at the incubation stage, with a minimum of one additional visit before the end of the species’ nesting period. For an egg laid prior to first visit, uncertainty estimation of laying date can be reduced as described for other species by a variety of methods, e.g. egg floatation, candling, and accurate egg mass measurement (Ackerman and Eagles-Smith Citation2010; Aldará et al. Citation2019). Determining the ideal method should consider the trade-off between data accuracy and increased disturbance of the nesting site.
Automated technologies
Camera surveys can be used to monitor nesting success in long-lived seabirds where frequent physical surveys are not possible (Southwell and Emmerson Citation2015; Merkel et al. Citation2016; Black Citation2018; Hinke et al. Citation2018; Edney and Wood Citation2021). Cameras could potentially deliver daily observations for the entirety of a nesting period, eliminating biases caused by a differing number of nest observations, whilst also providing highly accurate information on nest failure and fledging dates. A camera survey would also drastically reduce the number of unknown fates in a dataset. A fixed-point camera survey that records one photo per day per nest, would likely produce data sufficient to reliably calculate the nesting success of the species, and determine the changes in success over time and between artificial and natural nests. To determine specific causes of breeding failure, such as predation, more frequent observations, which could be achieved via time-lapse or motion-triggered cameras, would be more appropriate (Edney and Wood Citation2021). The applicability of camera surveys will be dependent on the aims of the monitoring program and the available funding, as camera surveys can have significant up-front costs (Bird et al. Citation2021a).
Increasing the number of observations will require either an increase in site visits to maintain equipment, or an increase in cost to purchase long-term equipment (Black Citation2018). Survey design will also need to consider the required sample size, which will be influenced by the number of nests visible in the field of view and failure rate of the chosen equipment (Hereward et al. Citation2021). As an alternative/complement to cameras, inexpensive sensors (i.e. temperature or light sensors) placed in the nest could be used to infer incubation and abandonment dates.
Implications of this study for monitoring seabirds in the GBR
Some examples of species that would benefit from the recommendations presented in this study are Brown Boobies (Sula leucogaster) and Lesser Frigatebirds (Fregata ariel). These species both have winter and summer breeding populations in the Swain Reefs, another remote priority monitoring location in the GBR (DES Citation2013; Bunce Citation2015; Woodworth et al. Citation2021). The Swain reefs lie 120–250 km offshore from St. Lawrence and are the most remote coral cays in the GBR World Heritage Area (DES Citation2013).
Brown Boobies and Lesser Frigate birds have fledging periods of 96–119 (Marchant and Higgins Citation1990) and 140 days (Diamond Citation1975a), respectively. The protracted breeding cycles of the species and remote breeding location would make it difficult to obtain accurate nesting success estimates. On-ground monitoring alone would likely create unstandardised observations and increase the detection of ‘unknown fate’ nests, which this study has shown will decrease the reliability of estimates and the ability to identify causes of nesting failures.
These populations are two examples where the recommendations in this study could vastly improve nesting success estimates and better direct conservation action. The GBR has over 900 continental islands and coral cays (GBRMPA Citation2022), and hosts breeding populations of over 20 seabird species (Congdon Citation2019) of which several are experiencing declines (Woodworth et al. Citation2021). Our recommendations are likely to be applicable to a wide range of remote locations in the GBR that harbour populations of seabirds with protracted breeding chronology.
Conclusion
The current monitoring program at Raine Island is resulting in data that are insufficient for robust estimates of nesting success. Our study highlights three critical considerations that could improve this situation. Monitoring efforts for seabirds are set to increase their focus on reproductive success as part of the Reef 2050 Long-term Sustainability Plan (Australian Government Citation2021). Robust monitoring protocols are thus needed to detect important changes in key metrics such as nesting success and causes of nest failure and to distinguish on-island impacts on nesting success from at-sea impacts. As seabird populations continue to decline around the world (Croxall et al. Citation2012; BirdLife International Citation2018; Woodworth et al. Citation2021), evaluation and improvement of monitoring programs are essential if we are to diagnose the causes of declines, and the success or otherwise of conservation interventions.
Supplemental Material
Download MS Word (105.7 KB)Acknowledgments
The authors would like to acknowledge the Queensland Department of Environment Science (DES), in particular staff from the Queensland Parks and Wildlife Service and Partnerships branch, for providing the field data and supporting this research project. We would also like to acknowledge Jeremy Bird and Rosalie Willacy from the University of Queensland for contributing to a recommendations report presented to the Department of Environment and Science associated with this research. We would also like to acknowledge the Traditional Owners of Raine Island, where the surveys were conducted, the Wuthathi Nation and Meriam Nation (Ugar, Mer, Erub) People, and the Traditional Owners of the land on which the University of Queensland is situated, the Turrbal and Jagera people.
Disclosure statement
No potential conflict of interest was reported by the author(s).
Data availability statement
Raw data generated by the Queensland Parks and Wildlife Service and Partnerships and derived data supporting the findings of this study are openly available on Mendeley Data at https://doi.org/10.17632/dwr79my39k.1.
Supplementary data
Supplemental data for this article can be accessed at https://doi.org/10.1080/01584197.2023.2269189.
References
- Ackerman, J. T., and Eagles-Smith, C. A. (2010). Accuracy of egg flotation throughout incubation to determine embryo age and incubation day in waterbird nests. The Condor 112(3), 438–446. doi:10.1525/cond.2010.090070
- Aldará, J., Hammer, S., Thorup, K., and Snell, K. R. (2019). Determining hatch dates for skuas: An egg density calibration curve. Seabird Journal 32(32), 84–95. doi:10.61350/sbj.32.84
- Australian Government (2021). ’Reef 2050 Long-Term Sustainability Plan.’ (Commonwealth of Australia, Department of Agriculture, Water and the Environment: Canberra.)
- Batianoff, G. N., and Cornelius, N. J. J. (2005). Birds of Raine Island: Population trends, breeding behaviour and nesting habitats. Proceedings of the Royal Society of Queensland 112(2), 1–29. https://search.informit.org/doi/abs/10.3316/ielapa.176416379085803
- Bird, J. P., Fuller, R. A., Pascoe, P. P., and Shaw, J. D. S. (2021a). Trialling camera traps to determine occupancy and breeding in burrowing seabirds. Remote Sensing in Ecology and Conservation. doi:10.1002/rse2.235
- BirdLife International (2018). ‘State of the world’s birds: Taking the pulse of the planet.’ Available at https://www.birdlife.org/papers-reports/state-of-the-worlds-birds/
- Bird, J. P., Woodworth, B. K., Fuller, R. A., and Shaw, J. D. S. (2021b). Uncertainty in population estimates: A meta-analysis for petrels. Ecological Solutions and Evidence 2(3), e12077. doi:10.1002/2688-8319.12077
- Black, C. E. (2018). Spying on seabirds: A review of time-lapse photography capabilities and limitations. Seabird Journal 31, 1–14. http://www.seabirdgroup.org.uk/seabird-31-1
- Bunce, A. (2015). Foraging behaviour of a declining population of Brown Boobies (Sula leucogaster) breeding in the Swain Reefs, Great Barrier Reef. Emu-Austral Ornithology 115(4), 368–372. doi:10.1071/MU14037
- Burnham, K. P., and Anderson, D. R. (2007). ‘Model Selection and Multimodel Inference: A Practical Information-Theoretic Approach,’ 2nd edn. (Springer: New York). doi:10.1007/b97636
- Claridge, G., and WBM Oceanics Australia. (1997). ‘Guidelines for managing visitation to seabird breeding islands.’ (Report for the Great Barrier Reef Marine Park Authority and Environment Australia-Biodiversity Group.) Available at https://www.gbrmpa.gov.au/__data/assets/pdf_file/0004/4765/gbrmpa_GuidelinesManagingVisitationSeabirdBreedingIslands.pdf
- Congdon, B. C. (2019). Seabirds In ’The Great Barrier Reef: Biology, Environment and Management.’ (Eds P. Hutchins, M. Kingsford, and O. Hoegh-Guldberg.) 2nd ed. pp. 419. (CSIRO Publishing: Melbourne.)
- Congdon, B. C., Erwin, C. A., Peck, D. R., Baker, G. B., Double, M. C., and O’Neill, P. (2007). Chapter 14: Vulnerability of seabirds on the Great Barrier Reef to climate change. In ’Climate Change and the Great Barrier Reef.’ (Eds J. Johnson and P. Marshall.) pp. 428. (Great Barrier Reef Marine Park Authority: Canberra.)
- Cornelius, J. (2010). Red-tailed Tropicbird - a 15 year monitoring program at Raine Island. Unpublished report, Queensland Parks and Wildlife Service and the Australian Bird and Bat Banding Schemes.
- Croxall, J. P., Butchart, S. H. M., Lascelles, B. E. N., Stattersfield, A. J., Sullivan, B. E. N., and Symes, A., Taylor, P.H.I.L. (2012). Seabird conservation status, threats and priority actions: A global assessment. Bird Conservation International 22(1), 1–34. doi:10.1017/S0959270912000020
- DAWE (2021). ‘Reef 2050 objectives and goals 2021–2025.’ (Deparment of Agriculture, Water and the Environment, Commonwealth of Australia.) https://www.agriculture.gov.au/sites/default/files/documents/reef-2050-objectives-goals-2021-2025.pdf [Verified 15 September 2022].
- DES (2013). ‘Swain Reefs National Park and adjoining state Marine Park management statement 2013.’ (Department of Environment and Science, Queensland Government.) Available at https://parks.des.qld.gov.au/management/plans-strategies/planning-documents Verified 24 May 2022].
- DES (2017). ’Raine Island Recovery Project.’ (Department of Environment and Science, Queensland Government.) https://parks.des.qld.gov.au/raineisland
- DES (2021). ’Raine Island National Park (Scientific). Management Statement.’ (Department of Environment and Science: Queensland Government.) https://parks.des.qld.gov.au/__data/assets/pdf_file/0032/166946/raine-island.pdf
- Diamond, A. W. (1975a). Biology and behaviour of frigatebirds fregata spp. On Aldabra Atoll. Ibis 117(3), 302–323. doi:10.1111/j.1474-919X.1975.tb04219.x
- Diamond, A. W. (1975b). The biology of Tropicbirds at Aldabra Atoll, Indian Ocean. The Auk 92(1), 16–39. doi:10.2307/4084415
- Dinsmore, S. J., White, G. C., and Knopf, F. L. (2002). Advanced techniques for modeling avian nest survival. Ecology 83(12), 3476–3488. doi:10.1890/0012-9658(2002)083[3476:ATFMAN]2.0.CO;2
- Dunstan, A., and Robertson, K. (2017). ‘Raine Island recovery project: 2016-17 season technical report to the Raine Island scientific advisory committee and Raine Island reference group.’ (Brisbane: Department of National Parks, Sport and Racing, Queensland Government.) Available at https://www.researchgate.net/publication/343688802_Raine_Island_Recovery_Project_2017-18_Technical_Report
- Dunstan, A., and Robertson, K. (2019). ‘Raine Island recovery project: 2018-19 season technical report to the Raine Island scientific advisory committee and Raine Island reference group.’ (Brisbane: Department of National Parks, Sport and Racing, Queensland Government.) Available at https://www.researchgate.net/publication/343688311_Raine_Island_Recovery_Project_2018-19_Technical_Report
- Edney, A. J., and Wood, M. J. (2021). Applications of digital imaging and analysis in seabird monitoring and research. Ibis 163(2), 317–337. doi:10.1111/ibi.12871
- Egerton, Z., Townsend, K., and Potvin, D. (2022). A review of the status and biology of an enigmatic pelagic species, the Red-tailed Tropicbird Phaethon rubricauda. Corella 46, 80–88.
- Felis, J., Kelsey, E., Adams, J., Stenske, J., and White, L. (2019). ‘Population estimates for selected breeding seabirds at Kīlauea point national Wildlife Refuge, Kauaʻi, in 2019.’ US Geological Survey Data Series 1130. (US Geological Survey: Reston, USA.) Available at https://pubs.er.usgs.gov/publication/ds1130
- Fleet, R. R. (1974). The Red-tailed Tropicbird on Kure Atoll. Ornithological Monographs 16 (16), 1–64. http://www.jstor.com/stable/40166698
- Flores, M., Lazo, P., Campbell, G., and Simeone, A. (2017). Breeding status of the Red-tailed Tropicbird (Phaethon rubricauda) and threats to its conservation on easter Island (Rapa Nui). Pacific Science 71(2), 149–160. doi:10.2984/71.2.4
- GBRMPA (2022). ‘Visiting islands and cays.’ Available at https://www.gbrmpa.gov.au/access-and-use/responsible-reef-practices/visiting-islands-and-cays [Verified 24 May 2022].
- Hereward, H. F. R., Facey, R. J., Sargent, A. J., Roda, S., Couldwell, M. L., and Renshaw, E. L., Shaw, K.H., et al. (2021). Raspberry Pi nest cameras: An affordable tool for remote behavioral and conservation monitoring of bird nests. Ecology and Evolution 21(11), 14585–14597. doi:10.1002/ece3.8127
- Hinke, J. T., Barbosa, A., Emmerson, L. M., Hart, T., Juáres, M. A., Korczak‐Abshire, M., and Milinevsky, G., et al. (2018). Estimating nest‐level phenology and reproductive success of colonial seabirds using time‐lapse cameras. Methods in Ecology and Evolution 9(8), 1853–1863. doi:10.1111/2041-210X.13015
- Jehle, G., Yackel Adams, A. A., Savidge, J. A., and Skagen, S. K. (2004). Nest survival estimation: A review of alternatives to the Mayfield estimator. The Condor 106(3), 472–484. doi:10.1093/condor/106.3.472
- Johnson, D. H. (1979). Estimating nest success: The Mayfield method and an alternative. The Auk 96(4), 651–661. doi:10.1093/auk/96.4.651
- King, B. R. (1986). Seabird islands no. 43/1. Raine Island, Great Barrier Reef, Queensland. Corella 10(3), 73–77.
- King, B. R. (1993). The status of Queensland seabirds. Corella 17(3), 65–92.
- Laake, J. (2013). ‘RMark: An R interface for analysis of capture-recapture data with MARK.’ AFSC Processed Rep. 2013-01, Alaska Fish. Sci. Cent., NOAA, Natl. Mar. Fish. Serv.
- Laake, J., and Rexstad, E. (2021). Appendix C – an alternative approach to building linear models in MARK. In ’Program MARK - “A Gentle Introduction”.’ (Eds Evan G. Cooch and Gary C. White.) 19th ed. http://www.phidot.org/software/mark/docs/book//
- Laniawe, L. (2008). Survivorship, productivity, and foraging range of the Red-tailed Tropicbird (Phaethon rubricauda) at Midway Atoll National Wildlife Refuge. MSc Thesis, University of East Anglia, Norwich, England.
- Manolis, J. C., Andersen, D. E., and Cuthbert, F. J. (2000). Uncertain nest fates in songbird studies and variation in Mayfield estimation. The Auk 117(3), 615–626. doi:10.1093/auk/117.3.615
- Marchant, S., and Higgins, P. J. (1990). ’Handbook of Australian, New Zealand and Antarctic Birds: Volume 1 – Ratites to Ducks.’ (Oxford University Press: Melbourne.)
- Mayfield, H. (1961). Nest success calculated from exposure. The Wilson Bulletin 73(3), 255–261. https://sora.unm.edu/sites/default/files/journals/wilson/v073n03/p0255-p0261.pdf
- Mayfield, H. (1975). Suggestions for calculating nest success. The Wilson Bulletin 87(4), 456–466. https://www.jstor.org/stable/4160682
- Merkel, F. R., Johansen, K. L., and Kristensen, A. J. (2016). Use of time‐lapse photography and digital image analysis to estimate breeding success of a cliff‐nesting seabird. Journal of Field Ornithology 87(1), 84–95. doi:10.1111/jofo.12143
- Miller, H., and Johnson, D. H. (1978). Interpreting the results of nesting studies. The Journal of Wildlife Management 42(3), 471–476. doi:10.2307/3800806
- Morrell, T. E., and Aquilani, S. M. (2000). Nest-site characteristics of Red-tailed Tropicbirds on Rose Atoll, American Samoa. Journal of Field Ornithology 71(3), 455–459. doi:10.1648/0273-8570-71.3.455
- Parsons, M., Mitchell, I., Butler, A., Ratcliffe, N., Frederiksen, M., Foster, S., and Reid, J. B., et al. (2008). Seabirds as indicators of the marine environment. ICES Journal of Marine Science 65(8), 1520–1526. doi:10.1093/icesjms/fsn155
- Prys-Jones, R., and Peet, C. (1980). Breeding periodicity, nesting success and nest site selection among Red-tailed Tropicbirds Phaethon Rubricauda and White-tailed Tropicbirds P. Lepturus on Aldabra Atoll. Ibis 122(1), 76–81. doi:10.1111/j.1474-919X.1980.tb00873.x
- Rotella, J. (2021). Chapter 17: Nest survival models. In ’Program MARK - “A Gentle Introduction”.’ (Eds E. G. Cooch and G. C. White.) 19th ed. http://www.phidot.org/software/mark/docs/book/
- Rotella, J. J., Dinsmore, S. J., and Shaffer, T. L. (2004). Modeling nest-survival data: A comparison of recently developed methods that can be implemented in MARK and SAS. Animal Biodiversity and Conservation 27(1), 187–205. https://raco.cat/index.php/ABC/article/view/57047
- Schreiber, E. A. (1994). El nino-southern oscillation effects on provisioning and growth in Red-tailed Tropicbirds. Colonial Waterbirds 17(2), 105–215. doi:10.2307/1521289
- Schreiber, E. A., and Schreiber, R. W. (1993). Red-tailed tropicbirds. In The Birds of North America. Life Histories for the 21st Century. (The Academy of Natural Sciences of Philadelphia.)
- Serventy, D. L., Serventy, V., and Warham, J. (1971). ‘Handbook of Australian Sea-Birds.’ (Eds A. H. Hart and A. W. Reed.) pp. 158–160. (Sydney.)
- Sommerfeld, J., Stokes, T., and Baker, G. B. (2015). Breeding success, mate-fidelity and nest-site fidelity in Red-tailed Tropicbirds (Phaethon rubricauda) on Christmas Island, Indian Ocean. Emu - Austral Ornithology 115(3), 214–222. doi:10.1071/MU14016
- Southwell, C., and Emmerson, L. (2015). Remotely-operating camera network expands Antarctic seabird observations of key breeding parameters for ecosystem monitoring and management. Journal for Nature Conservation 23, 1–8. doi:10.1016/j.jnc.2014.11.002
- Stewart, D. A., Clarke, R. H., Baker, G. B., and Garnett, S. T. (2021). Herald Petrel Pterodroma heraldica. In ’The Action Plan for Australian Birds 2020.’ (Eds S. T. Garnett and G. B. Baker.) pp. 172–174. (CSIRO Publishing: Melbourne.)
- Taplin, A., and Blaber, S. J. M. (1993). Seabird breeding populations studies at Raine Island. In ’Raine Island and Environs, Great Barrier Reef: Quest to Preserve a Fragile Outpost of Nature.’ (Eds. H. K. Zevering and E. C. Zevering.) pp. 51–56. (Townsville: Raine Island Corporation in cooperation with the Great Barrier Reef Marine Park Authority.)
- Tets van, G. F., and Fullagar, P. J. (1984). Status of seabirds breeding in Australia. In ‘Status and Conservation of the World’s Seabirds Proceedings of the ICBP Seabird Conservation Symposium 1982, Cambridge, UK.’ (Eds J. P. Croxall, P. G. H. Evans and R. W Schreiber.) pp. 562–567.
- Tyler, W. B. (1991). A tropical seabird nesting at a temperate latitude: the ecology of Red-tailed Tropicbirds (Phaethon rubricauda) at Midway Atoll. MSc Thesis, University of California, Santa Cruz, USA.
- Vanderwerf, E. A. (2021). Status and monitoring methods of a Red-tailed Tropicbird colony on O’ahu, Hawaiʻi. Marine Ornithology 49, 215–222. http://www.marineornithology.org/content/get.cgi?rn=1426
- Vanderwerf, E. A., and Young, L. C. (2014). Breeding biology of Red-tailed Tropicbirds Phaethon Rubricauda and response to predator control on O’Ahu, HawaiʻI. Marine Ornithology 42(1), 69–72. http://www.marineornithology.org/content/get.cgi?rn=1065
- Venables, W., and Ripley, B. (2002). ’Modern Applied Statistics with S,’ 4th edn. (Springer: New York LLC.)
- Warham, J. (1961). The birds of Raine island, Pandora cay and Murray Island sandbank, North Queensland. Emu - Austral Ornithology 61(2), 77–93. doi:10.1071/MU961077
- White, G. C., and Burnham, K. P. (1999). Program mark: Survival estimation from populations of marked animals. Bird Study 46(sup1), S120–S139. doi:10.1080/00063659909477239
- Woodworth, B. K., Fuller, R. A., Hemson, G., McDougall, A., Congdon, B. C., and Low, M. (2021). Trends in seabird breeding populations across the Great Barrier Reef. Conservation Biology 35(3), 846–858. doi:10.1111/cobi.13630