Abstract
Genetic variation in TAS2R38 bitterness taste receptor could alter the efficacy of molecular sensing, hence may be associated with cancer risk. Thus, we performed a meta-analysis to verify the association between the risk of gastrointestinal (GI) neoplasm and TAS2R38 genetic variation. Studies with TAS2R38 diplotype distribution and GI neoplasm phenotypes were searched from PubMed, EMBASE and SCOPUS, and five articles including eight studies were finally selected. The association between diplotype and neoplasm risk was estimated with summarized odds ratios (ORs) and 95% confidence intervals (CIs), applying of fixed- or random-effects models. The findings suggested TAS2R38 diplotype was not associated with GI neoplasms susceptibility [AVI vs. PAV: OR = 1.03 (95%CI: 0.97–1.09), AVI/PAV vs. PAV/PAV: OR = 1.05, (95%CI: 0.94–1.17), AVI/* vs. PAV/PAV: OR = 1.04 (95%CI: 0.94–1.16)]. Because of the presence of heterogeneity under the two genetic models (AVI/AVI vs. PAV/PAV and AVI/AVI vs. PAV/*), further subgroup analyses by ethnicity and neoplasm type were performed. However, results failed to show the neoplasm risk was altered by diplotype. In conclusion, the meta-analysis indicates that TAS2R38 diplotype minimally modified the GI neoplasm risk. Given the limited study size and resources, further well-designed and larger studies are required to validate the true effect of TAS2R38 polymorphisms on neoplasm risk.
Introduction
The gastrointestinal (GI) system is not simply an organ for digestion but also a comprehensive sensory tissue (Citation1). Molecular sensing in the GI system differentiates between a variety of externally/internally derived molecules and regulates the subsequent digestive process, including secretion, absorption and transportation. Furthermore, chemosensing in the GI system also recognizes ingested pernicious or non-nutritive compounds, such as drugs and/or food-borne toxins (Citation2) and initiates elimination or neutralization responses, including vomiting or excretion, in the alimentary track to promote survival in response to external/internal stimuli. For these reasons, the GI system, with its molecular sensing, acts as a secondary nutritional gatekeeper along with oral taste perception (Citation3).
Among the major human taste categories, bitterness is considered critical in food preference and rejection, and therefore, bitterness can influence dietary intake and nutritional status (Citation4 − 7). Furthermore, an array of toxins, including phytochemicals and milieu molecules, generally present with bitterness (Citation8). Therefore, lingual and GI perception of bitterness are critical warning systems for survival and disease etiology (Citation9). Type 2 bitter-taste receptors (T2Rs, TAS2Rs), a family of G protein-coupled receptors (GCPRs), mediate the bitterness perception. T2R chemosensing proteins are known to have 25 isoform types and are expressed in multiple tissues, including the oral cavity and GI tract (Citation10). Human TAS2R genes are located on chromosomes 5, 7, and 12 and are highly polymorphic (Citation11). Among the identified genetic variants, the diplotype of TAS2R38, consisting of three single-nucleotide polymorphisms (SNPs) [A49P (145G > C, rs713598), V262A (785T > C, rs1726866) and I296V (886A > G, rs10246939)], has been well described for its functional alterations (Citation12). Observational and experimental studies have reported that the AVI haplotype showed differential phenotypic and molecular characteristics compared with those of the PAV haplotype (Citation12,Citation13). This evidence suggests that structural changes in T2R38 induced by those genetic variations are decisive factors in bitterness sensitivity and recognition of harmful molecules. Therefore, they might regulate subsequent metabolism and disease susceptibility.
GI cancer is the leading cause of death worldwide (Citation14). The cause of cancer is multifactorial; therefore, multiple studies have been conducted to understand the mechanisms of cancer development and progression, utilizing various approaches that consider dietary, genetic and environmental factors (Citation6,Citation15,Citation16). Recently, an increasing number of studies have been conducted to examine whether TAS2R38 genetic variation is responsible for GI disorders, especially cancer. Researchers have reported that the AVI haplotype or AVI homo-diplotype is associated with the incidence of gastric cancer (GC) and colorectal cancer (CRC) (Citation3,Citation17,Citation18). However, the association between TAS2R38 genetic variation and GI neoplasms is still controversial (Citation3,Citation17). Furthermore, the trend toward the risk-modifying effect of the TAS2R38 diplotype is variable. The potential reason for such inconsistency surrounding TAS2R38 genetic variation and neoplasm incidence is assumed to be lack of power due to small sample sizes, but much remains unconfirmed. Therefore, to obtain a more comprehensive understanding of the role of the T2R38 bitterness receptor in cancer etiology, we performed a meta-analysis to evaluate the association between TAS2R38 diplotype genetic variation and the risk of GI neoplasm.
Methods
Data Sources, Search Strategy, and Selection Criteria
shows a summary of the literature search procedure applied in our study. Articles in 3 electronic literature databases (PubMed, EMBASE and SCOPUS) published in English and prior to September 2017 were examined. The following keywords were used to obtain potential articles regarding the association between TAS2R38 diplotype and neoplasm risk, independently and then combined: (1) (tas2r38 or t2r38 or tas2r or bitter taste receptor or bitterness receptor or phenylthiocarbamide gene or 6-n-propylthiouracil or prop), (2) (SNP or polymorphism or mutation or genotype or variation or variant), and (3) (cancer or tumor or carcinoma or neoplasm). The relevance of the studies was evaluated by screening the title, abstract and full text. Only human studies were considered for inclusion, and abstracts, conference proceedings and unpublished reports were excluded from the article pool. Manual searching for the references for original and review articles was also performed. Only articles meeting the following inclusion criteria were considered for this meta-analysis: (1) studies assessing the association between neoplasm incidence and the TAS2R38 diplotype/haplotype and (2) studies describing the results as raw data and considering the TAS2R38 diplotype and neoplasm phenotype in any human populations.
Figure 1. A flow chart depicting the literature search and study selection for the present meta-analysis.
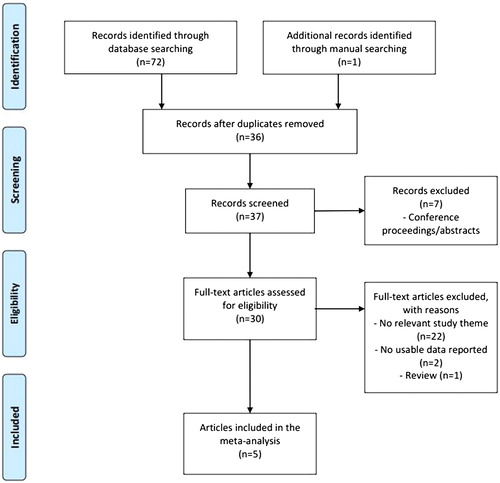
In total, 73 items were obtained using the search terms listed above. After removing the duplicates (n = 36), 37 items were evaluated for their relevance to TAS2R38 diplotype related-neoplasm risk. Twenty-nine items were excluded due to inappropriate format or irrelevance (n = 7 and 22). The remaining eight articles were carefully reviewed, and among these, one review and two original articles that did not contain a usable dataset were eliminated. After these steps, five original articles were analyzed for this unadjusted pooled meta-analysis.
Data Extraction
The following key information, which is required for meta-analysis, was retrieved from each study: the first author’s name, publication year, study location (country), ethnicity of study subjects, neoplasm type, number of cases and controls, and distribution of TAS2R38 diplotype. Two independent researchers extracted the data and achieved a consensus on all retrieved information.
Statistical Analyses
The association between the TAS2R38 genetic variants and GI neoplasm incidence was estimated using odds ratios (ORs) with corresponding 95% confidence intervals (CIs). To present the study specific results, the determined association is exhibited with forest plots. Five genotypic models were applied to assess the association between genetic variation and neoplasm risk: (1) haplotype comparison (AVI vs. PAV), (2) heterozygote comparison (PAV/AVI vs. PAV/PAV), (3) recessive homozygote comparison (AVI/AVI vs. PAV/PAV), (4) dominant model comparison (AVI/*vs. PAV/PAV), and (5) recessive model comparison (AVI/AVI vs. PAV/*). These genetic models were applied in the pooled population as well as in groups stratified by ethnicity (Caucasian and non-Caucasian), or neoplasm type [GI cancer only excluding adenomatous polyps (AP), AP only, CRC including AP, or CRC excluding AP)]. Prior to the analyses, we intended to confirm that the genotypic data were in Hardy-Weinberg equilibrium (HWE). However, a few articles did not show the distribution of genotypes in each locus for the TAS2R38 diplotype. Therefore, we could not assess whether the data were in HWE; however, all the original articles reported that the genotype distributions of the controls were in HWE. Other haplotypes of TAS2R38, in addition to PAV and AVI, were evident in each article. However, their association with neoplasm risk was not generally computed due to the rarity. For this reason, only the PAV and AVI diplotypes were considered in this study. We estimated unadjusted OR due to the studies without a published OR value. Heterogeneity was evaluated using a Q-statistic and I2 test. For the summary OR estimation, a random effects model was applied when P < 0.05 from the heterogeneity of Q-statistics and I2 > 50%; otherwise, a fixed model was used. In the case of heterogeneity, subgroup/stratified analyses by ethnicity and neoplasm type were also performed to explore the source of the heterogeneity. Sensitivity analysis was also conducted by omitting each study to ascertain the effect of an individual study. Publication bias was examined using a funnel plot, and its skewedness was evaluated using Egger’s linear regression test (P < 0.05).
The statistical analyses for this study were performed with STATA software (version 14, Stata Corporation, College Station, TX). Two-sided P values <0.05 were considered statistically distinctive.
Results
The Details of the Analyzed Studies
Using the search terms and procedures described above, 5 articles with 3,060 cases and 4,908 controls were selected for this meta-analysis. Of these articles, Schembre et al. (Citation17) reported the distribution of the TAS2R38 diplotype in three ethnic groups, and Carrai et al. (3) separately presented this information in two different nationalities. For this reason, these two articles were considered three and two independent studies, respectively, in this meta-analysis. Therefore, the total number of studies included was eight.
presents the major characteristics of the studies included in this meta-analysis. The studies were all designed for case-control investigations. Specifically, three and four studies were conducted in Caucasian (Citation3,Citation17) and Asian populations (Citation17–20), respectively. A single study was performed in a Polynesian (native Hawaiian) population (Citation17). Three of the studies focused on the incidence of CRC and the effect of TAS2R38 genetic variants (Citation3,Citation18). Another three studies investigated AP, a major antecedent of CRC (Citation17). One study by Choi et al. reported the influence of TAS2R38 genetic variants on GC (Citation19). Yamaki’s Japanese study reported the association between the TAS2R38 diplotype and GI cancer (Citation20). However, the study was not stratified by neoplasm type because the number of cases for each cancer type was limited [e.g., GC (n = 18) or CRC (n = 21)]. The prevalence of the PAV haplotype ranged from 0.38 to 0.66 and was more frequently evident in non-Caucasian groups (n = 5). Therefore, PAV was considered a reference haplotype in this meta-analysis.
Table 1. Summary of the key characteristics of the studies included in this meta-analysis.
The Association Between Neoplasm Risk and TAS2R38 Genetic Variants
When the effect of the TAS2R38 haplo-/diplotype was analyzed, none of the genetic analysis models indicated that the TAS2R38 diplo- or haplotype was associated with a risk for GI neoplasm. The presence of the AVI haplotype and the AVI/PAV diplotype did not modify the risk for GI neoplasms compared with the reference PAV haplotype or PAV/PAV diplotype groups (AVI vs. PAV: OR = 1.03, 95% CI = 0.97–1.09, AVI/PAV vs. PAV/PAV: OR = 1.05, 95% CI = 0.94–1.17, AVI/* vs. PAV/PAV: OR = 1.04, 95% CI = 0.94–1.16) (). Stratified analyses were conducted to investigate the potential association between the TAS2R38 haplo-/diplotype and neoplasia, considering ethnicity and neoplasm type. However, no meaningful genetic effect was observed on neoplasm incidence using any of the genetic models. The results and details of all the analyses are presented in Supporting Information Table S1.
Figure 2. Odds ratios (ORs) and 95% confidence intervals (CIs) from analyzed studies comparing gastrointestinal neoplasms and the TAS2R38 haplotype (AVI vs. PAV) using a fixed effect model. The summarized OR is exhibited using a diamond. The center and both terminals of the diamond indicate the OR and 95% CI, respectively.
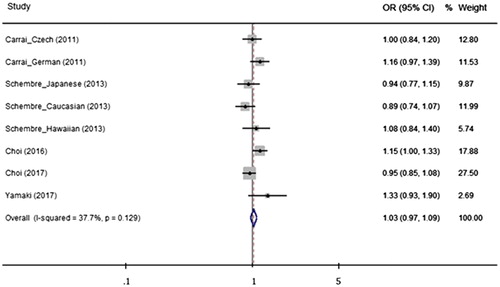
Figure 3. Odds ratios (ORs) and 95% confidence intervals (CIs) from studies comparing gastrointestinal neoplasms and the TAS2R38 diplotype (AVI/PAV vs. PAV/PAV) using a fixed effect model. The summarized OR is exhibited using a diamond. The center and both terminals of the diamond indicate the OR and 95% CI, respectively.

Figure 4. Odds ratios (ORs) and 95% confidence intervals (CIs) from studies comparing gastrointestinal neoplasms and the TAS2R38 diplotype (AVI/* vs. PAV/PAV) using a fixed effect model. The summarized OR is exhibited using a diamond. The center and both terminals of the diamond indicate the OR and 95% CI, respectively
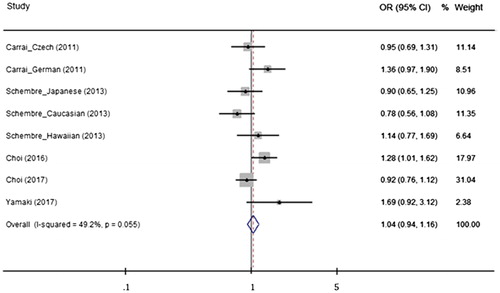
Heterogeneity and Subgroup Analysis
When the risk-modifying effect of the AVI/AVI diplotype was compared with the PAV homo- or hetero-diplotype (PAV/PAV or PAV/*), heterogeneity was detected (, P for heterogeneity = 0.006 and I2 = 65% for AVI/AVI vs. PAV/PAV, and p for heterogeneity = 0.002 and I2 = 69% for AVI/AVI vs. PAV/*). To identify the cause of heterogeneity, subgroup analyses based on ethnicity and neoplasm type were performed. We conducted subsequent analyses in Caucasians and non-Caucasians, separately, but the results showed that the TAS2R38 AVI/AVI diplotype was not associated with a risk for GI neoplasms. Additional subgroup analyses were performed in studies of GI cancer only (excluding AP), AP only, CRC (including AP), or CRC (excluding AP). However, the AVI/AVI homo-recessive diplotype was not associated with a risk for GI neoplasms, and after these stratified analyses, heterogeneity was still detected.
Table 2. Stratified analyses of the effect of the TAS2R38 diplotype (AVI/AVI vs. PAV/PAV or PAV/*) on the risk of gastrointestinal neoplasms.
Publication Bias Assessment and Sensitivity Test
To estimate the presence of publication bias, funnel plot analysis and Egger’s test were applied. The plots and the P values of the tests provided evidence that no selection or publication bias existed in the meta-analysis (see Supporting Information Table S1 and Figure S1 for the P values and funnel plots). Sensitivity analysis, conducted by omitting individual studies, was performed to evaluate the effect of each study on the pooled OR under all the genetic models. However, the results did not reveal a significant change in the final pooled OR for TAS2R38 genetic variants in the risk for GI neoplasms (data not shown).
Discussion
T2R38 is a type of GCPR chemosensing enzyme universally expressed in multiple tissues, including the GI tract and oral cavity. Therefore, genetic variation in TAS2R38 was thought to be associated with disease risk by modifying dietary intake. The true effect of TAS2R38 genetic variation on the risk for GI neoplasms, independent of dietary intake, has also been a major academic interest, but the findings are inconclusive. Such inconsistency may stem from issues with statistical power and heterogeneity. We therefore examined the risk-modifying effect of the TAS2R38 genetic variant on neoplasm susceptibility using a meta-analysis technique. However, the results from 8 studies with a total of 7968 participants suggest that TAS2R38 genetic variation is not associated with susceptibility to GI neoplasms. Additional subgroup analyses were performed, but they showed that TAS2R38 genetic variants had no modifying effect on the risk for neoplasms.
Epidemiological studies in multiple ethnicities were conducted to examine the association between the T2R38 bitterness receptor and cancer with genetic variation as a modifying factor. Increased risk for colorectal and GI cancer associated with the TAS2R38 AVI/AVI diplotype was observed in Germans and Japanese (Citation3,Citation20). A Korean study also showed that PAV/AVI increased the risk for GC (Citation19). The pathogenic role of T2Rs was also observed in an in vitro study with pancreatic cancer cells. T2R38 was involved in pancreatic cancer progression by mediating the activation of key transcription factors (Citation21,Citation22). However, how T2R38 regulates the development and progression of other types of neoplasms, including breast and GI cancer, has not been clearly elucidated (Citation23). To conjecture the potential association between T2R38 and GI cancer, the study of respiratory diseases could be referred. The epithelial expression of T2Rs appeared to be associated with the quorum sensing of bacterial compounds, regulating nitric oxide production and innate immunity (Citation24,). This could also explain why the TAS2R38 diplotype showed differential efficacy in bactericidal activity and mucociliary clearance (Citation25,Citation26). In line with this, one putative hypothesis is suggested. The structural changes in T2R38 due to genetic variation might alter the efficacy of the alimentary tract in sensing potential carcinogenic/harmful compounds. This may lead to inappropriate protective responses against those stimuli and may increase the risk for GI cancer (Citation3). Additionally, the regulatory role of bitterness perception and genetic variation in other aspects of physiological metabolism may also be linked to cancer predisposition (Citation1,Citation27,Citation28). For instance, thyrocyte-expressed T2R proteins have been observed (Citation29,Citation30), and bitterness sensitivity and TAS2R38 genetic variation are known to be associated with energy and glucose metabolism. The decisive factors and markers in this mechanism, including glycemic index and load, insulin, insulin resistance, insulin-like growth factor (IGF) and IGF-binding protein, play regulatory roles in cell proliferation and apoptosis and hence may modify cancer risk (Citation31,Citation32). These ideas may support the hypothesis that bitterness intensity and the TAS2R38 variant may be related to the mechanism of carcinogenesis, regardless of dietary modification.
Earlier studies predicted that carriers of the TAS2R38 AVI/AVI diplotype or AVI haplotype were at greater risk for cancer compared with those with the PAV/PAV diplotype (Citation3,Citation33). However, in Koreans, the AVI/AVI diplotype was observed not to increase the risk but rather to protect against CRC (Citation18). Experimental evidence may explain the contrasting findings between these studies. Although individuals with the AVI haplotype were less sensitive to chemicals containing a thiourea (N-C=S) moiety, they were still responsive to other bitterness molecules (Citation13). Additionally, independent expression of the AVI transcript was evident (Citation13). This may suggest that the structural changes caused by genetic variation do not simply impair function; these changes may improve sensing for other unknown compounds and hence may initiate appropriate anti-carcinogenic mechanisms. This may also explain why TAS2R38, as well as other TAS2R bitterness receptor-encoding genes, show a high degree of polymorphism (Citation11). The perception of bitterness from a variety of natural/artificial substances is mainly mediated by 25 types of T2R bitterness receptors. Deorphanization of T2Rs has led to identification of precise agonists for each isoform. However, single molecules can activate multiple T2Rs, and one T2R can have a wide spectrum of chemical ligands (Citation33,Citation34). Therefore, the genetic diversity in TAS2Rs may provide a benefit in recognition of multiple pernicious chemical compounds in terms of survival and natural selection in human evolution (Citation11).
Studies have shown the significance of bitterness receptor genetic variation in disease etiology; however, controversies remain. In Czech populations, AVI/AVI was not associated with a risk for CRC. The same null association of AVI/AVI was also observed in native Hawaiian and Japanese- and Caucasian-American populations. The statistical results of the present meta-analysis also suggest that TAS2R38 genetic variation only has a limited association with susceptibility to GI neoplasms across multiple ethnic populations and neoplasm types. A few ideas may be proposed to explain this minimal role of bitterness-related genetic variation in neoplasm risk. First, the distribution of the TAS2R38 haplo-/diplotype varies among ethnicities. The PAV haplotype was dominant in East Asians but not in populations of Caucasian origin. Furthermore, the AAI haplotype was barely evident in Caucasians and East Asians but is often present in populations of African origin (Citation35). This genetic diversity may affect or hinder the pathogenic role of the TAS2R38 variant, and therefore, it did not appear in the summarized OR in the meta-analysis. Second, as alluded above, the risk-modifying effect of the TAS2R38 variant was different depending on the characteristics of the subjects, ethnicities and neoplasm type. Therefore, differential risk-modifying effects may be nullified in the meta-analysis procedure. To test such ambiguity, as well as to seek the source of heterogeneity, we conducted subgroup analyses. However, the analyses gave us a limited association between the TAS2R38 genetic variant and the risk for GI neoplasms. Further studies should be performed to verify these hypotheses and the true role of T2R38 in GI carcinogenesis.
This meta-analysis provides more comprehensive power to assess the effect of TAS2R38 genetic variation in neoplasm susceptibility; however, potential limitations may exist. First, this meta-analysis was conducted in a population of 3060 patients and 4908 controls. The size of this study is relatively large compared with previous studies, but we cannot dismiss the possibility that a bigger sample size may provide a better understanding of the associations between the genetic polymorphisms and disease. Second, a degree of heterogeneity was observed in the analyses using recessive homozygote and recessive genetic model comparison. Additional stratified analyses by ethnicity and type of neoplasm, as well as sensitivity tests, were conducted, yet those did not answer this issue. The limited number of subjects and different clinical, genetic and environmental characteristics of subjects may act as confounding factors responsible for these results. Last, the number of studies available was limited, especially for stratified analysis, and thus, each subgroup may not appropriately represent the full population characteristics. In addition, most of the studies focused on colorectal neoplasm, but numbers of studies with other GI neoplasm were limited. For these reasons, the current findings should be carefully interpreted.
In summary, using a meta-analysis, we investigated the association between genetic variation in the TAS2R38 bitterness taste receptor and the risk for GI neoplasms. The results indicate that bitterness-related genetic variation does not regulate GI neoplasm susceptibility. Nevertheless, the epidemiological investigation of TAS2R38-related disease etiology, especially for cancer, is still in its infancy. Additional well-designed and larger studies in populations with various genetic and ethnic backgrounds are required.
Author Contributions
JHC designed the study. JHC and JK screened and retrieved the literature and data. JHC analyzed the data and drafted the manuscript. JK provided critical review and undertook responsibility for the overall study content. All authors read and approved the final manuscript.
Discloure Statement
None of the authors has a conflict of interest.
Supplemental Material
Download PDF (114.7 KB)Additional information
Funding
References
- Rozengurt E: Taste receptors in the gastrointestinal tract. I. Bitter taste receptors and alpha-gustducin in the mammalian gut. Am J Physiol Gastrointest Liver Physiol 291, G171–G177, 2006.
- Sternini C, Anselmi L, and Rozengurt E: Enteroendocrine cells: A site of 'taste' in gastrointestinal chemosensing. Curr Opin Endocrinol Diabetes Obes 15, 73–78, 2008.
- Carrai M, Steinke V, Vodicka P, Pardini B, Rahner N, et al.: Association between TAS2R38 gene polymorphisms and colorectal cancer risk: a case-control study in two independent populations of Caucasian origin. PLoS One 6, e20464, 2011.
- Basson MD, Bartoshuk LM, Dichello SZ, Panzini L, Weiffenbach JM, et al.: Association between 6-n-propylthiouracil (PROP) bitterness and colonic neoplasms. Dig Dis Sci 50, 483–489, 2005.
- Sandell M, Hoppu U, Mikkila V, Mononen N, Kahonen M, et al.: Genetic variation in the hTAS2R38 taste receptor and food consumption among Finnish adults. Genes Nutr 9, 433, 2014.
- Choi JH, Lee J, Choi IJ, Kim YW, Ryu KW, et al.: Variations in TAS1R taste receptor gene family modify food intake and gastric cancer risk in a Korean population. Mol Nutr Food Res 60, 2433–2445, 2016.
- Choi JH, Lee J, Yang S, and Kim J: Genetic variations in taste perception modify alcohol drinking behavior in Koreans. Appetite 113, 178–186, 2017.
- Ames BN, Profet M, and Gold LS: Nature’s chemicals and synthetic chemicals: Comparative toxicology. Proc Natl Acad Sci USA 87, 7782–7786, 1990.
- Lucock M, Ng X, Boyd L, Skinner V, Wai R, et al.: TAS2R38 bitter taste genetics, dietary vitamin C, and both natural and synthetic dietary folic acid predict folate status, a key micronutrient in the pathoaetiology of adenomatous polyps. Food Funct 2, 457–465, 2011.
- Lu P, Zhang CH, Lifshitz LM, and ZhuGe R: Extraoral bitter taste receptors in health and disease. J Gen Physiol 149, 181–197, 2017.
- Kim U, Wooding S, Ricci D, Jorde LB, and Drayna D: Worldwide haplotype diversity and coding sequence variation at human bitter taste receptor loci. Hum Mutat 26, 199–204, 2005.
- Kim UK, Jorgenson E, Coon H, Leppert M, Risch N, et al.: Positional cloning of the human quantitative trait locus underlying taste sensitivity to phenylthiocarbamide. Science 299, 1221–1225, 2003.
- Bufe B, Breslin PA, Kuhn C, Reed DR, Tharp CD, et al.: The molecular basis of individual differences in phenylthiocarbamide and propylthiouracil bitterness perception. Curr Biol 15, 322–327, 2005.
- Siegel RL, Miller KD, and Jemal A: Cancer statistics, 2015. CA Cancer J Clin 65, 5–29, 2015.
- Sacerdote C, Guarrera S, Smith GD, Grioni S, Krogh V, et al.: Lactase persistence and bitter taste response: Instrumental variables and mendelian randomization in epidemiologic studies of dietary factors and cancer risk. Am J Epidemiol 166, 576–581, 2007.
- Gong J, Hutter CM, Newcomb PA, Ulrich CM, Bien SA, et al.: Genome-wide interaction analyses between genetic variants and alcohol consumption and smoking for risk of colorectal cancer. PLoS Genet 12, e1006296, 2016.
- Schembre SM, Cheng I, Wilkens LR, Albright CL, and Marchand Le L: Variations in bitter-taste receptor genes, dietary intake, and colorectal adenoma risk. Nutr Cancer 65, 982–990, 2013.
- Choi JH, Lee J, Oh JH, Chang HJ, Sohn DK, et al.: Variations in the bitterness perception-related genes TAS2R38 and CA6 modify the risk for colorectal cancer in Koreans. Oncotarget 8, 21253–21265, 2017.
- Choi JH, Lee J, Choi IJ, Kim YW, Ryu KW, et al.: Genetic variation in the TAS2R38 bitter taste receptor and gastric cancer risk in Koreans. Sci Rep 6, 26904, 2016.
- Yamaki M, Saito H, Isono K, Goto T, Shirakawa H, et al.: Genotyping analysis of bitter-taste receptor genes TAS2R38 and TAS2R46 in Japanese patients with gastrointestinal cancers. J Nutr Sci Vitaminol 63, 148–154, 2017.
- Singh N, Chakraborty R, Bhullar RP, and Chelikani P: Differential expression of bitter taste receptors in non-cancerous breast epithelial and breast cancer cells. Biochem Biophys Res Commun 446, 499–503, 2014.
- Gaida MM, Mayer C, Dapunt U, Stegmaier S, Schirmacher P, et al.: Expression of the bitter receptor T2R38 in pancreatic cancer: Localization in lipid droplets and activation by a bacteria-derived quorum-sensing molecule. Oncotarget 7, 12623–12632, 2016.
- Shaik FA, Singh N, Arakawa M, Duan K, Bhullar RP, and Chelikani P: Bitter taste receptors: Extraoral roles in pathophysiology. Int J Biochem Cell Biol 77, 197–204, 2016.
- Lee RJ, Xiong G, Kofonow JM, Chen B, Lysenko A, et al.: T2R38 taste receptor polymorphisms underlie susceptibility to upper respiratory infection. J Clin Invest 122, 4145–4159, 2012.
- Lee RJ, Kofonow JM, Rosen PL, Siebert AP, Chen B, et al.: Bitter and sweet taste receptors regulate human upper respiratory innate immunity. J Clin Invest 124, 1393–1405, 2014.
- Lee RJ and Cohen NA: Taste receptors in innate immunity. Cell Mol Life Sci 72, 217–236, 2015.
- Dotson CD, Zhang L, Xu H, Shin YK, Vigues S, et al.: Bitter taste receptors influence glucose homeostasis. PLoS One 3, e3974, 2008.
- Keller M, Liu X, Wohland T, Rohde K, Gast MT, et al.: TAS2R38 and its influence on smoking behavior and glucose homeostasis in the German Sorbs. PLoS One 8, e80512, 2013.
- Clark AA, Dotson CD, Elson AE, Voigt A, Boehm U, et al.: TAS2R bitter taste receptors regulate thyroid function. FASEB J 29, 164–172, 2015.
- Choi JH, Lee J, Yang S, Lee EK, Hwangbo Y, et al.: Genetic variations in TAS2R3 and TAS2R4 bitterness receptors modify papillary carcinoma risk and thyroid function in Korean females. Sci Rep 8, 15004, 2018.
- Sandhu MS, Dunger DB, and Giovannucci EL: Insulin, insulin-like growth factor-I (IGF-I), IGF binding proteins, their biologic interactions, and colorectal cancer. J Natl Cancer Inst 94, 972–980, 2002.
- Turati F, Galeone C, Gandini S, Augustin LS, Jenkins DJ, et al.: High glycemic index and glycemic load are associated with moderately increased cancer risk. Mol Nutr Food Res 59, 1384–1394, 2015.
- Meyerhof W, Batram C, Kuhn C, Brockhoff A, Chudoba E, et al.: The molecular receptive ranges of human TAS2R bitter taste receptors. Chem Senses 35, 157–170, 2010.
- Fujikura K: Multiple loss-of-function variants of taste receptors in modern humans. Sci Rep 5, 12349, 2015.
- Risso DS, Mezzavilla M, Pagani L, Robino A, Morini G, et al.: Global diversity in the TAS2R38 bitter taste receptor: Revisiting a classic evolutionary PROPosal. Sci Rep 6, 25506, 2016.