Abstract
The risk and the size of an outbreak of avian influenza virus (AIV) could be restricted by vaccination of poultry. A vaccine used for rapid intervention during an AIV outbreak should be safe, highly effective after a single administration and suitable for mass application. In the case of AIV, aerosol vaccination using live virus is not desirable because of its zoonotic potential and because of the risk for virus reassortment. The rational design of novel mucosal-inactivated vaccines against AIV requires a comprehensive knowledge of the structure and function of the lung-associated immune system in birds in order to target vaccines appropriately and to design efficient mucosal adjuvants. This review addresses our current understanding of the induction of respiratory immune responses in the chicken. Furthermore, possible mucosal vaccination strategies for AIV are highlighted.
1. Introduction
Several important poultry pathogens, including avian influenza virus (AIV), infectious bronchitis virus (IBV) and Newcastle disease virus (NDV), enter the host through the mucosal tissues of the respiratory tract (RT) (Villegas Citation1998) and subsequently disseminate towards other organs in the body. Therefore, animal health significantly depends on the control of infection already in the lung tissue by the RT immune system. The rational design of novel mucosal vaccines against AIV requires a comprehensive knowledge of the structure and function of the lung-associated immune system in birds in order to target vaccines appropriately and to design efficient mucosal adjuvants. At the moment, there is very limited knowledge of the lung-associated immune system in the chicken and in poultry, in general, which might be a consequence of the unique and complex anatomy and function of the avian lung.
2. Avian influenza virus
Influenza virus is a member of the orthomyxoviruses, a family of single-stranded (ss)-RNA viruses. Three types of influenza virus exist: A, B and C. Aquatic birds are the natural reservoir for influenza A (Baigent and McCauley Citation2003; Muramoto et al. Citation2006). However, influenza A viruses can infect a wide range of mammalian and avian species. Influenza A viruses are characterized by their two surface antigens (Ag), haemagglutinin (HA; H1–16) and neuraminidase (NA; N1–9). All HA and NA subtypes have been isolated from avian species, however H3, H5, H7 and H9 infections are most common in domestic poultry (Swayne Citation2008).
Influenza A enters the host cell by binding of HA to sialic acids (SA) attached to galactose (Gal; SAα-2,3Gal or SAα-2,6Gal-linkages) (Gambaryan et al. Citation1995). Human influenza viruses prefer SAα-2,6Gal-linkages, whereas avian viruses prefer SAα-2,3Gal (Baigent and McCauley Citation2003). Both SAα-2,3Gal- and SAα-2,6Gal-linked receptors are widely expressed within different tissues, including the RT of poultry (Kuchipudi et al. Citation2009; Pillai and Lee Citation2010; Yu et al. Citation2011). This suggests that poultry can be infected with a wide range of influenza viruses and also probably act as a ‘mixing vessel’.
Following fusion, influenza virus mainly enters the host cell via not only clathrin-dependent mechanisms, but also clathrin-independent and caveolin-independent mechanisms (Lakadamyali et al. Citation2004), with the characteristics of macropinocytosis (de Vries et al. Citation2011). Once internalized, these pathways are all equally efficient for virus fusion (Lakadamyali et al. Citation2004). For productive infections, influenza viruses need to have their HA0 cleaved into HA1 and HA2 segments. Low-pathogenic (LP) AIV HA proteins are cleaved by trypsin-like enzymes located primarily in the RT or produced by some types of bacteria. Highly-pathogenic (HP) AIV possess multibasic cleavage sites and can therefore be cleaved by multiple enzymes (Swayne Citation2008), thereby facilitating systemic replication (Webster and Rott Citation1987; Munster et al. Citation2010).
In chicken, infection with HPAIV causes severe depletion, haemorrhages, oedema, cutaneous ischaemia and mortality rates that can reach up to 100% (Muramoto et al. Citation2006). The H5N1 HPAIV has had a great impact on the poultry industry in recent years. Moreover, it is a zoonotic infection, with high mortality rates after transmission to humans. In the Netherlands, the last HPAIV outbreak was an H7N7 outbreak in 2003. Although control measures were implemented, 255 poultry flocks became infected at that time and 1381 commercial flocks were depopulated (Stegeman et al. Citation2004; Elbers et al. Citation2005). In infected poultry flocks, clinical signs included increased mortality, swollen head and wattles, respiratory problems and decreased egg production (Elbers et al. Citation2005). During the epidemic, 89 people involved in handling of infected poultry became infected, resulting in one death (Fouchier et al. Citation2004).
Not only HPAIV infections pose a threat to the poultry industry and to man, but LPAIV strains as well. LPAIV strains are most prevalent and can cause significant economic losses. Furthermore, they can contribute to, or mutate into more pathogenic strains (Marcus et al. Citation2007). LPAIV infections can cause subclinical to mild symptoms, primarily in the RT. Mortality rates are low, but can significantly increase in the case of secondary infections (de Wit et al. Citation2004; Swayne Citation2008).
As AIV enters the host via the RT and from there spreads towards other organs, a vaccine should already halt infection at the mucosal tissues of the RT, the portal of entry. For the design of new vaccines, it is important to gain knowledge on how the RT innate immune system handles viral (vaccine) antigens. Knowledge on early immune responses after infection or vaccination is crucial for the rational design of novel mucosal vaccines. Furthermore, it is important to know if uptake of a vaccine antigen locally in the lung results in induction of both local and systemic humoral responses.
3. Immune system of the avian RT
3.1. Morphology of the avian lung
The chicken RT differs significantly from mammalian RT in both morphology and airflow as well as immunologically. The mammalian lung has bidirectional airflow, whereas the avian lung has a unidirectional airflow () (Reese et al. Citation2006). Chicken lungs are located dorsally that the ribs incise the lungs (Duncker Citation1972). The lungs are small when compared to mammals, and do not significantly change in volume during respiration. Nine air sacs are present, which function in ventilation, but do not contribute to the gas exchange (). These adaptations of the avian lung facilitate rapid gas exchange needed for sustained flight (Farmer Citation2006; Maina Citation2006). Furthermore, air sacs could provide birds with the stability and agility needed in flight (Farmer Citation2006).
Figure 1. Anatomy of the avian lung. A: Schematic drawing of air flow in the avian lung during inspiration (left picture) and expiration (right picture) (adapted from Kothlow and Kaspers 2008, with permission from Elsevier). B: Respiratory system of chickens (Clav. AS = clavicular air sac; Cran. Th. AS = cranial thoracic air sac; Caud. Th. AS = caudal thoracic air sac; Abd. AS = abdominal air sac). During inhalation, the air sacs expand and air is drawn from the outside through the trachea and primary bronchi, partly towards the caudal air sacs and partly towards the paleopulmonic parabronchi (Fedde Citation1998).
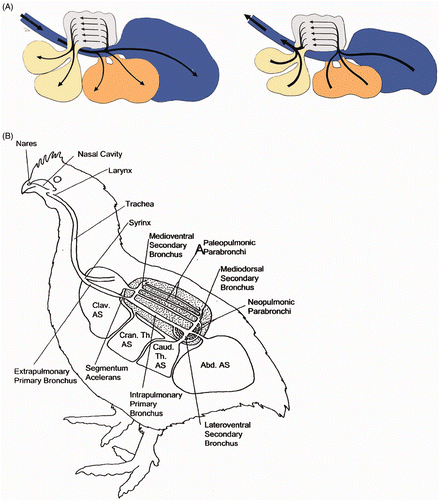
Birds can breathe through their nares or mouth and air first arrives in the oronasal cavity. The oronasal cavity is separated from the trachea by the larynx, which opens into the trachea via the glottis. At the syrinx, the trachea bifurcates into two primary bronchi (Powell Citation2000). The primary bronchus enters the lung ventrally and passes to the dorsolateral surface, ending up in the abdominal air sacs (Duncker Citation1972). The secondary bronchi originate from the primary bronchus. Gas exchange occurs at the tertiary bronchi. The blood–gas barrier of the avian lung is much thinner than that of mammals with the same body mass, while the respiratory surface is greater. These features are thought to predispose birds to pulmonary injury (Reese et al. Citation2006).
The lymphoid tissue of the RT can roughly be divided into an upper and a lower part. The lymphoid tissue of the upper part of the RT includes paraocular (including the Harderian gland (HG)) and nasal lymphoid structures and lymphoid accumulations in the pharynx and larynx. The avian lung exhibits both highly organized lymphoid structures and diffusely-distributed lymphoid and myeloid cells. The organized bronchus-associated lymphoid tissue (BALT) structures develop at the junctions of the primary bronchus and the caudal secondary bronchi () (Bienenstock et al. Citation1973; Van Alstine and Arp Citation1988). In contrast to the mammalian lung, where BALT is inducible, it is constitutively present in the chicken lung (Bienenstock et al. Citation1973). It is believed that BALT is a normal lymphoid structure in poultry, compensating for the lack of a lymph node system (Fagerland and Arp Citation1993a).
Figure 2. Schematic drawing of a chicken lung indicating locations of BALT. PB = primary bronchus; 1 = medioventral secondary bronchi; 2 = mediodorsal secondary bronchi; 3 = lateroventral secondary bronchi; A–D = openings into the air sacs. BALT nodules are indicated as orange dots (Reese et al. Citation2006).
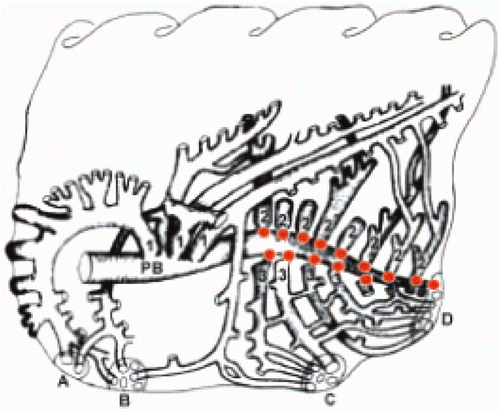
BALT development follows a similar time course in specified pathogen-free (SPF) and conventional chickens, though slight differences are observed with regards to germinal centre (GC) development (Fagerland and Arp Citation1993a). The development is also similar in broiler and layer chickens and in turkeys. In one-day-old chickens, no immunoglobulin (Ig)-producing cells of any isotype are present (Fagerland and Arp Citation1993a) and B cells are not found in chicken lung until the second week after hatch (Jeurissen, Janse, Koch, et al. Citation1989). During the following weeks, increasing numbers of CD45+ cells accumulate and develop into organized structures with T cells in the centre and B cells in the periphery (Jeurissen et al. Citation1994). These organized BALT structures are not observed prior to the third to fourth week after hatching and GC are first seen in 3-week-old chickens (Fagerland and Arp Citation1990; Fagerland and Arp Citation1993a; Reese et al. Citation2006). Fully developed BALT, with B cells in the central area covered by a CD4+ cell cap, is found in birds that are 6 weeks of age and older (Fagerland and Arp Citation1990), and at that time mature antibody-secreting cells (ASC) of all isotypes are seen under or within BALT epithelium and in non-BALT areas (Jeurissen, JanseKoch, et al. Citation1989; Fagerland and Arp Citation1993a). By this time, nearly every bronchial opening is surrounded by the lymphoid tissue (Fagerland and Arp Citation1993b). As there is no local production of Ig in the chicken BALT until the first week after hatch and IgA is only produced after the second week, in young chickens Ig against respiratory pathogens must be serum derived. The Ig could be derived either from maternal sources, or be produced elsewhere (Fagerland and Arp Citation1993a).
Mature BALT consists of lymphocyte aggregates covered by a distinct layer of epithelium, harbouring considerable numbers of lymphocytes (). This epithelium is referred to as follicle-associated epithelium (FAE). In mammalian mucosa-associated lymphoid tissues (MALT), the epithelial layer contains specialized M-cells, but these are not observed in chicken BALT (Fagerland and Arp Citation1993b). In FAE, cells are present that display some characteristics of M-cells, however particle uptake by these cells was not observed (Fagerland and Arp Citation1990; Fagerland and Arp Citation1993b). In 6–8-week-old birds, GC are found in most nodules and occasionally ASC are found under the FAE (Jeurissen, Janse, Kok, et al. Citation1989; Fagerland and Arp Citation1993a).
3.2. The phagocyte system of the RT
Following RT infection or vaccination, the antigen (Ag) needs to be taken up and presented to cells of the adaptive immune system. A variety of antigen-presenting cells (APC) is present in rodent and human RT including dendritic cells (DC), macrophages (MPh) and B cells. RT-DC are considered to be attractive targets for mucosal vaccination strategies, since a large network of phagocytes, including DC is located beneath the epithelium of several mucosal tissues, such as the airway and the intestines and they are potent activators of naive T cells.
In the mouse lung, DC are found in the large conducting airways, the lung parenchyma and in the alveolar space (de Heer et al. Citation2005); both conventional (c)DC and plasmacytoid (p)DC are present. cDC can be divided in trachea and large airway epithelial DC expressing CD11c, langerin and CD103, submucosal DC expressing CD11b and CD11c and interstitial DC that can be either CD11b+ or CD11b- (GeurtsvanKessel et al. Citation2008). In alveolar space, a population of alveolar MPh is also present. In the RT, tracheal DC have a rapid turnover rate (2–3 days), while interstitial DC are long lived (Holt et al. Citation1994; von Garnier et al. Citation2005).
The chicken RT contains few free-residing MPh (‘avian respiratory phagocytes’; ARP) compared to mammals and MPh are absent from the surface of the air capillaries (Maina Citation2002). In avian lung, MPh are present in the lining of the atria and infundibulae (Maina Citation2002) and are also abundantly present in the connective tissue (Klika et al. Citation1996). Phagocytic cells are strategically localized at the start of the gas-exchange area to clear the air of inhaled particles, before it reaches the thin and vulnerable air capillaries (Kothlow Citation2008). Most diffusely distributed leukocytes in interstitial lung tissue belong to the monocyte/MPh and DC phenotype, because of their expression of MHC-II, and their positive staining using the antibodies (Ab) CVI-ChNl-68.1 and −74.2 (Jeurissen, Jeurissen, Janse, Koch, et al. Citation1989; Jeurissen, Janse, Kok, et al. Citation1989). Adjacent to the muscle fibers that form the parabronchi, the lumen is bordered by 74.3 stained cells, and these cells are expected to act as mammalian alveolar MPh (Jeurissen et al. Citation1994). Epithelial cells lining the atrial muscles, the atrial floor and the infundibulae have been shown to be phagocytic and may therefore play a role in the defence of the thin, extensive and highly vulnerable tissue barrier (Nganpiep and Maina Citation2002). It is unclear if phagocytosed material is presented to the local immune system in the BALT, or in the follicles present in the interstitium between parabronchi or if it is transported to the spleen or a combination of these option depending on the antigen. In chicken, BALT is constitutively present and is thought to compensate for the lack of dLN. When chickens were inoculated with fluorescent beads that were coated with LPS as a bacterial Ag, bead + cells were present in BALT areas (de Geus, Jansen, et al. Citation2012). This could indicate that following the uptake of Ag, APC migrate mainly locally towards the BALT area. Moreover, bead + cells were rarely present in blood and spleen, suggesting that local induction of RT immune responses might dominate over the systemic induction.
Ag-bearing DC in the mammalian RT mainly migrate towards mediastinal lymph nodes (MLN), where they interact with naïve T cells (Legge and Braciale Citation2003; Hammad and Lambrecht Citation2007; GeurtsvanKessel et al. Citation2008; Bakocevic et al. Citation2010; Lambrecht and Hammad Citation2010). In chicken, it is not known which cell type is responsible for priming of naive T cells and whether a functional distinction between MPh and DC in priming capacity exists. In mouse, the endosomal compartment of DC has a near neutral pH (Savina et al. Citation2006; Savina et al. Citation2009), while MPh have much lower phagosomal pH (Yates and Russell Citation2005). In mouse, the decreased phagosomal acidification in DC reduces Ag proteolysis to a level that allows processing but does not fully destroy antigenic peptides. This contributes to the high Ag-presenting capacity of DC (Mantegazza et al. Citation2008; Ramachandra et al. Citation2009). MPh have much higher levels of proteolysis than DC, which limits their efficiency as APC (Delamarre et al. Citation2005). Recently, lung phagocytic cells were identified in chicken that decreased the pH of their endosomal compartment following the uptake of a bead (CD11 + , KUL01+ and CD40+ phagocytes) and cells that did not (MHC II+ and CD80+ phagocytes) (de Geus, Jansen, et al. Citation2012), suggesting that in chicken MPh also are functionally distinct from DC. Whether the capacity to present antigen differs between these cell populations needs further investigation. These findings can facilitate the specific targeting of DC using a mucosal vaccine.
3.3. Humoral immune responses in the RT
The mucosal tissue of the nose is first to come into contact with aerosols or micro-organisms during inhalation. Large particles and micro-organisms can be removed mechanically by the mucus, but invasive pathogens need to be controlled by the immune system. A major characteristic of organized chicken nasal-associated lymphoid tissue (NALT) is the formation of circumscribed B cell areas occasionally displaying GC, covered by a CD4+ T cell cap. In both organized NALT and in the epithelium, the dominant isotype is IgY, whereas IgM+ cells are less frequent and IgA+ cells are relatively rare (Ohshima and Hiramatsu Citation2000). IgY-containing cells are more frequently observed than the other isotypes, so in contrast to the importance of IgA in the mammalian mucosal immune system, IgY may be more important at avian mucosal surfaces, at least in the nasal cavity (Ohshima and Hiramatsu Citation2000).
The major eye-associated lymphoid tissues are located in the HG and in the conjunctiva of the lower eyelid. Secretions of the HG drain into the upper RT, providing local protection by secretory Ig (Dohms et al. Citation1981). Almost all tear Ig is produced locally in the HG, because removal of the HG abrogated IgM and IgA and reduced IgY in tears (Scott et al. Citation1993; Jeurissen et al. Citation1994). The HG is organized in two histologically-distinct compartments, a head and a body. The head of the gland resembles a secondary lymphoid organ, with lymphoid accumulation and GC. The majority of B cells are IgA+, although many IgY+ cells are found as well (Jeurissen et al. Citation1994). The plasma cell-filled body resembles a tertiary lymphoid structure (Olah et al. Citation1996). Discrepancies exist regarding Ig expression in HG. Olah et al. (Citation1996) found that the ASC produce mainly IgA and IgM, whereas Jeurissen et al. (Citation1994) showed the presence of a considerable number of IgY-producing cells. Locally produced Ig of all isotypes appears in tears together with IgY transudated from serum (Toro et al. Citation1993), thus protecting the upper RT. The ASC are present in comparable numbers in chickens raised under SPF and under conventional conditions (Bang and Bang Citation1968). In steady state situations, all Ig isotypes are produced, but after local immunization with tetanus toxoid vaccine, IgA and IgY were produced in high concentrations, with only trace amounts of IgM (Shirama et al. Citation1996). The ASC can migrate to the epithelium, where they make contact with cells resembling M cells. These cells are probably involved in Ig-secretion (Gallego and Glick Citation1988).
There is no constitutive lymphoid tissue present in trachea. However, the tracheal mucosa is highly responsive to infection and reacts with extensive lymphocyte infiltration followed by proliferation (Gaunson et al. Citation2000; Gaunson et al. Citation2006; Matthijs et al. Citation2009). Tracheal lesions characteristic for Mycoplasma infections predominantly consist of proliferating B cells (Gaunson et al. Citation2006) and this is similar to IBV infection (Kotani et al. Citation2000). The cells are mainly located in the lamina propria and submucosa (Kotani et al. Citation2000). Responses in trachea are age-dependent as B cells were not detected in the trachea of Mycoplasma gallisepticum-infected SPF layer-type chicken until 3 weeks post infection (Gaunson et al. Citation2006).
In interstitial lung tissue, IgM+ cells are found from 5 days after hatch (Jeurissen, Janse, Kok, et al. Citation1989), while IgA+ and IgY+ cells are not found at that time (Jeurissen, Janse, Kok, Citation1989b). In older birds, B cells are found throughout the parenchyma (Reese et al. Citation2006). In BALT and other regions of the lung, IgM-producing cells predominate over IgY-producing cells and IgA-producing cells (Fagerland and Arp Citation1993a). ASC are not present in the gas-exchange areas of the lung (Fagerland and Arp Citation1993a).
4. Induction of influenza-specific immunity
Induction of an antiviral adaptive immune response requires uptake of AIV by immature respiratory DC. In mice, both pDC and cDC are necessary for the induction of an adaptive response following influenza infection (GeurtsvanKessel et al. Citation2008). In mammals, Ag-bearing DC migrate to the MLN where they interact with T cells. It has been shown that following influenza infection, the langerin + CD103 + CD11b – cDC that migrated to the MLN presented Ag ex vivo to both CD4+ and CD8+ T cells (GeurtsvanKessel et al. Citation2008).
In AIV infections, humoral immune responses contribute to virus neutralization through binding to viral Ag. In humans, all currently used vaccines depend on the induction of neutralizing antibody responses (Letvin Citation2007). Humoral memory, i.e. secretion of specific neutralising antibodies over long periods in the apparent absence of the Ag provides the host with a first line of defence against reinfection (reviewed in Dorner and Radbruch Citation2007).
In influenza-infected mice, virus-specific ASC are detected in various organs, such as lung, MLN, bone marrow (BM) and spleen (Owens et al. Citation1981; Jones and Ada Citation1986; Joo et al. Citation2008; Wolf et al. Citation2011). In humans, HA-specific IgA in nasal washes is considered to be an important mediator in protection from influenza infection (Clements et al. Citation1986). Also in chicken, Ig are involved in protection against AIV as birds that are protected by vaccination have high serum titres of influenza-specific Ig (Kumar et al. Citation2007; Maas et al. Citation2009; Nayak et al. Citation2009). The role of respiratory Ig in AIV-specific immunity has previously been investigated after vaccination at the level of secreted Ig in tears and serum and ASC in HG. It is known that Ig are involved in protection against AIV as birds that are protected by vaccination have high serum titres of influenza-specific Ig (Kumar et al. Citation2007; Maas et al. Citation2009; Nayak et al. Citation2009). Van Ginkel et al. (Citation2008) studied mucosal and systemic humoral immune responses following ocular influenza vaccination with a H5-expressing replication competent adenovirus (RCA)-free human E1/E3-defective Ad5 vector. High frequencies of H5-specific IgA ASC observed in HG- and H5-specific Ig were found in serum and tears. In influenza-infected chickens, IgM responses predominate during primary infection. Antiviral IgA and IgY responses were induced both locally in the lung and systemically in the spleen. Responses in the lung preceded responses in the spleen, indicating that respiratory immune responses were not only induced in the spleen but also locally in the lung. The AIV-specific ASC responses in lung significantly correlated to responses in bone marrow (BM), suggesting also a role for BM in respiratory immunity (de Geus, Rebel, et al. Citation2012).
In vivo, most ASC that are generated in a given immune reaction disappear from the secondary lymphoid organs within a few days to weeks. In a secondary immune response in mice, 10–20% of the ASC that are generated survive in the BM for more than 2 weeks. The persisting ASC can survive in vivo for long periods and can be detected in BM for at least the next 60 days (reviewed by Radbruch et al. Citation2006). Also in chickens, the BM could be a survival niche for long-living ASC. However, when chickens were infected with LP H7N1 AIV, serum responses and also AIV-specific ASC numbers in all organs, including BM, declined very rapidly and could not be detected at 3 weeks post primary and 6 weeks post secondary infection (de Geus, Rebel, et al. 2012). This might suggest that H7N1 infection generates short-lived ASC. Generation of short-lived ASC has been described in a mouse vesicular stomatitis virus model, where ASC were transferred into naïve mice. In the presence of Ag, high numbers of ASC are maintained; while in the absence of Ag, ASC numbers decline rapidly (Ochsenbein et al. Citation2000).
In mice, CD8+ cytotoxic T cells (CTL) also make an important contribution in influenza-specific immunity (Kim et al. Citation2011). In chickens, the role of influenza-specific CTL is less clear. Pulmonary CD8+ cells were shown to be important in protection against AIV (Seo et al. Citation2002) and cells isolated from lungs of AIV-infected chickens secrete IFN-γ after ex vivo restimulation (Rauw et al. Citation2011). Furthermore, in a recent study, MHC I-inbred chickens were infected with AIV and cells isolated from lung were stimulated ex vivo with several predicted CD8 + T cell epitopes. These epitopes where predicted, based on anchor residues described for the different MHC I types of the MHC I-inbred chickens. This way, novel influenza-specific CD8 + T cell epitopes were identified in MHC I-inbred chickens (Reemers et al. Citation2012).
In conclusion, both humoral and cellular responses play a role in protection from influenza infection. Neutralizing Ab are crucial, as chickens with measurable HI titres after vaccination were protected from clinical disease following AIV infection (Kumar et al. Citation2007; Maas et al. Citation2009). Furthermore, passive immunization with AIV protein-specific antiserum protected birds from challenge infection (Shahzad et al. Citation2008).
5. Vaccination strategies
The risk and the size of an outbreak of AIV could be restricted by vaccination of poultry. Currently licensed vaccines for use in chicken are adjuvanted whole-inactivated virus (WIV) or split virus, inactivated reverse-genetics virus for H5N1 and H5N3, and fowl pox and NDV vector vaccines (reviewed by van den Berg et al. Citation2008). Possible vaccination strategies are rapid intervention (e.g. emergency vaccination of poultry in the area around an outbreak), preventive vaccination of specific categories of poultry that are more at risk for a new introduction of AIV (e.g. free range layers in areas with many wild ducks and geese) and general preventive vaccinations of poultry in area's in which AIV is endemic. A vaccine used for rapid intervention during an AIV outbreak should be safe, highly effective after a single administration and be suitable for mass application. A vaccine that could be applied by spray or aerosol would be suitable for mass application, which is regularly performed, e.g. IBV (De Wit et al. Citation2010), NDV, avian metapneumovirus and most Mycoplasma galliseptum vaccines (Ley Citation2003). In the case of AIV, aerosol vaccination using live virus is not desirable because of its zoonotic potential and because of the risk for virus reassortment. Mucosal vaccination via the respiratory route has several advantages: it induces both local and systemic immune responses (Atmar et al. Citation2007; Tseng et al. Citation2009; Worrall et al. Citation2009), it could halt infection already at portal of entry (Yoshikawa et al. Citation2004) and it is suitable for mass application.
5.1. Mucosal application of inactivated vaccines
Intranasally (i.n.) applied whole inactivated AIV is poorly immunogenic (Hagenaars et al. Citation2008; Worrall et al. Citation2009; de Geus et al. Citation2011) and this is also described in chicken for i.n. applied inactivated NDV (Tseng et al. Citation2009). To enhance the immunogenicity of whole-inactivated AIV it needs to be adjuvanted. In the field of mucosal vaccination advances have been made in the development of mucosal adjuvants that protect the Ag from degradation and can increase nasal residence time, such as poly(lactic-co-glycolic acid) (PLGA) and chitosan (and its derivative trimethyl chitosan; TMC) (Slutter et al. Citation2010; Keijzer et al. Citation2011).
The local induction of RT tract immune responses in chicken makes it attractive to apply a vaccine to the RT. However, when different adjuvants were tested in an aerosolized vaccine using WIV, none of the adjuvanted vaccines induced AIV-specific Ig after single vaccination, measured 1 and 3 weeks after vaccination by aerosol, in contrast to the intramuscularly applied vaccine. The aerosolized vaccine did enter the chickens’ RT as cholera toxin B subunit (CT-B)-specific serum Ig were detected after 1 week in chickens vaccinated with the CT-B-adjuvanted vaccine (de Geus et al. Citation2011). Also using an i.n. vaccination strategy, no AIV-specific Ig was found until 3 weeks after the third vaccination (de Geus Citation2012). Although the respiratory route is ideal for mass application, for the use of whole inactivated virus further modifications of a vaccine are needed, for example with regard to retention time and to efficiently induce an immune response in the tolerizing environment of the RT. Van Eck (Citation1990) vaccinated SPF chickens repeatedly with inactivated NDV in PD, a carbomer adjuvant. Only low HI titers were induced after oculonasal vaccination and several booster vaccinations did not result in increased titers. Furthermore, very high doses of antigen were required to induce protection from challenge infection. Following i.n. application, approximately 70–80% of the applied volume is swallowed and thus ends up in the gastrointestinal tract (Van Eck 2011, personal communication). This could affect immunogenicity of WIV, while leaving immunogenicity of CT-B intact. As no AIV-specific Ig was induced both after aerosol vaccination and after repeated i.n. vaccination, future research for rapid intervention strategies or mucosal vaccination strategies should focus on alternatives such as the use of live vector or subunit vaccines. Other approaches could include enhancing retention time of the WIV vaccine and increasing Ag uptake by targeting RT-DC subsets.
5.2. Alternative vaccination strategies suitable for mucosal application
Adjuvanted inactivated AIV did not induce detectable levels of serum Ab after both aerosol and i.n. applications (de Geus et al. Citation2011). An alternative approach for mass vaccination of chickens could be the use of live vector vaccines. Vector vaccines can be compatible with Differentiating Infected from Vaccinated Animals (DIVA) strategies. The currently licensed vector vaccines for influenza vaccination in chicken are recombinant fowl pox virus and NDV vector vaccine. The recombinant fowl pox vaccine induced good protection from heterologous challenge (Swayne et al. Citation2007), but can interfere with preexisting immunity against fowl pox. HA-expressing NDV vector vaccines can be applied by spray or in drinking water and have shown to protect against challenge infection in chicken (Swayne et al. Citation2003; Nayak et al. Citation2009; Schroer et al. Citation2011). Similar to the fowl pox vaccine, the NDV vector vaccine can also interfere with preexisting NDV immunity induced by NDV vaccination. Furthermore, the presence of maternal Ab can interfere with vaccine efficacy. Other vectors that are currently being tested for mucosal vaccination strategies include adenovirus Ad5, infectious laryngotracheitis (ILT) recombinants and baculovirus. Mucosally applied AIV protein-expressing Ad5 induces high levels of AIV-specific Ig and also HI titers against the whole influenza virus in mouse (Price et al. Citation2010), ferrets (Roy et al. Citation2011) and chicken (van Ginkel et al. Citation2008). Coarse spray vaccination of chickens did not induce any adverse effects and slightly elevated H5-specific IgA was found in tears (Toro et al. Citation2010). Ocular (Veits et al. Citation2003; Pavlova et al. Citation2009) or tracheal (Luschow et al. Citation2001) vaccination with HA-expressing ILT induces HA-specific Ig and protects from challenge infection. Vaccination with H7-expressing baculovirus induced H5-specific Ig after intramuscular vaccination, but responses after i.n. vaccination were much lower (He et al. Citation2008).
In summary, a vector vaccine has to be chosen with care because interference with existing vaccination programs or maternal antibodies against the viral vectors (in the case of, for instance, NDV and fowl pox) could interfere with the induction of protective Ig responses. Furthermore, the choice of the vector is important because a viral vector might reassort and could pose a health threat. Although rarely a problem, at least for the NDV vector the possibility of occurrence of reassortment is under debate (Collins et al. Citation2008; Han et al. Citation2008).
Challenges in respiratory vaccination include the mucociliary clearance mechanisms in the nasal cavity and the trachea, resulting in a low residence time and the low permeability of the mucosa (Ugwoke et al. Citation2001; Illum Citation2003). Mucosal residence time can be improved by using particles with mucoadhesive properties (Smart Citation2005; Jaganathan and Vyas Citation2006). These particles are generally inert, but provide persistent Ag exposure and could facilitate the uptake by APC (reviewed by O'Hagan and Valiante Citation2003). Further advantages of mucoadhesive particles include the possibility to crosslink the Ag on the particle surface and the possibility to include immunomodulatory agents such as TLR ligands, thereby increasing recognition and immunogenicity (reviewed by O'Hagan and Valiante 2003; Sharma et al. Citation2009). Enhancing the residence time in the RT of a vaccine would prolong its exposure to RT-DC, resulting in increased uptake and Ag-presentation. As respiratory administration of inactivated AIV alone does not result in upregulation of MHC II and CD80 expression by RT-DC (de Geus, Jansen, et al. 2012), it will be necessary to co-adjuvant with or co-administer a mucoadhesive particle. Particles that are currently tested in mucosal vaccines include PLGA spheres or chitosan and its derivative TMC.
PLGA spheres consist of biocompatible copolymers and are approved for use in humans. A major problem in the use of PLGA spheres is the protein instability of incorporated Ag (van de Weert et al. Citation2000). This problem could be solved by adsorbing Ag onto the surface of the particle (Kazzaz et al. Citation2000). In mice, PLGA particles are poorly taken up by DC and do not induce subsequent maturation. Furthermore, nasal administration of OVA-PLGA spheres did not induce specific Ig responses, even after tertiary vaccination (Slutter et al. Citation2010). In turkeys, PLGA particles are taken up by MPh in vitro, but oculonasal vaccination with live avian metapneumovirus (aMPV) was far superior to vaccination with aMPV F protein-loaded PLGA as live aMPV vaccination completely protected birds from clinical disease and only 50% of aMPV F protein-PLGA vaccinated birds were protected from challenge infection (Liman et al. Citation2007).
TMC is a chitosan derivative that is more stable at neutral pH than chitosan itself and has shown promising results in i.n. vaccines in mice (Hagenaars et al. Citation2009; Slutter et al. Citation2010). Chitosan and TMC have mucoadhesive properties and have the ability to cross transepithelial barriers. Besides its mucoadhesive properties, chitosan can also act as an adjuvant (Ghendon et al. Citation2008; Ghendon et al. Citation2009). TMC significantly increases nasal residence time of co-administered OVA and high levels of IgG and sIgA were induced after nasal administration (Slutter et al. Citation2010). Both adjuvants should therefore be able to overcome the challenges posed by nasal administration. Chitosan has shown promising results in i.n. split virus vaccines in mice (Bacon et al. Citation2000), split virus and protein vaccines in humans (Read et al. Citation2005; Sui, Chen, Fang, et al. Citation2010; Sui, Chen, Wu, et al. Citation2010) and split virus and DNA vaccines in poultry (Tischer et al. Citation2002; Worrall et al. Citation2009; Huang et al. Citation2010; Rauw et al. Citation2010). However, in our study vaccination with chitosan-adjuvanted inactivated AIV and TMC inactivated AIV particles did not induce AIV-specific serum Ig (de Geus et al. Citation2011). It was published that chitosan can work synergistically with secondary adjuvants such as CT-B (Moschos et al. Citation2004). It would therefore be worthwhile to co-adjuvant a chitosan- or TMC-based AIV vaccine for use in chicken.
In summary, mucoadhesive particles are promising vaccine components for use in an AIV vaccine for rapid intervention strategy, but studies are needed to properly co-adjuvant these particles and to prevent Ag degradation.
6. Concluding remarks
Respiratory immune responses in the chicken are locally induced. This would be advantageous for mucosal vaccination strategies. Possible mucosal vaccination strategies for general preventive vaccination include the use of adjuvanted inactivated AIV and the use of vector viruses. Furthermore, vaccination strategies could include the use of particles that increase mucosal residence time or the specific targeting of vaccine Ag to RT-DC subsets. Targeting or enhanced recruitment of RT-DC subsets could be achieved by adding a specific adjuvant, making use of particulate Ag-delivery systems that promote phagocytosis or by the targeting of receptors expressed by RT-DC, such as glycan-recognizing receptors. For respiratory application, particles should be designed in such a way that they can also reach the lung tissue itself to be taken up by RT-DC. Particles in size ranging from 1 to 3.7 µm can be expected to distribute throughout the RT (Hayter and Besch Citation1974; Hayter Citation1977; Corbanie et al. Citation2006; Tell et al. Citation2006). When a vaccine is designed for a rapid intervention strategy, a possible approach could be the activation of the innate immune system by, for instance, CT-B (Matsuo et al. Citation2000), resulting in a partial protection until the adaptive immune system is activated.
References
- Atmar , RL , Keitel , WA , Cate , TR , Munoz , FM , Ruben , F and Couch , RB . 2007 . A dose-response evaluation of inactivated influenza vaccine given intranasally and intramuscularly to healthy young adults . Vaccine , 25 ( 29 ) : 5367 – 5373 .
- Bacon , A , Makin , J , Sizer , PJ , Jabbal-Gill , I , Hinchcliffe , M , Illum , L , Chatfield , S and Roberts , M . 2000 . Carbohydrate biopolymers enhance antibody responses to mucosally delivered vaccine antigens . Infect Immun , 68 ( 10 ) : 5764 – 5770 .
- Baigent , SJ and McCauley , JW . 2003 . Influenza type A in humans, mammals and birds: determinants of virus virulence, host-range and interspecies transmission . Bioessays , 25 ( 7 ) : 657 – 671 .
- Bakocevic , N , Worbs , T , Davalos-Misslitz , A and Forster , R . 2010 . T cell-dendritic cell interaction dynamics during the induction of respiratory tolerance and immunity . J Immunol , 184 ( 3 ) : 1317 – 1327 .
- Bang , BG and Bang , FB . 1968 . Localized lymphoid tissues and plasma cells in paraocular and paranasal organ systems in chickens . Am J Pathol , 53 ( 5 ) : 735 – 751 .
- Bienenstock , J , Johnston , N and Perey , DY . 1973 . Bronchial lymphoid tissue. I. Morphologic characteristics . Lab Invest , 28 ( 6 ) : 686 – 692 .
- Clements , ML , Betts , RF , Tierney , EL and Murphy , BR . 1986 . Serum and nasal wash antibodies associated with resistance to experimental challenge with influenza A wild-type virus . J Clin Microbiol , 24 ( 1 ) : 157 – 160 .
- Collins , PL , Bukreyev , A and Murphy , BR . 2008 . What are the risks – hypothetical and observed – of recombination involving live vaccines and vaccine vectors based on nonsegmented negative-strain RNA viruses? . J Virol , 82 ( 19 ) : 9805 – 9806 .
- Corbanie , EA , Matthijs , MG , van Eck , JH , Remon , JP , Landman , WJ and Vervaet , C . 2006 . Deposition of differently sized airborne microspheres in the respiratory tract of chickens . Avian Pathol , 35 ( 6 ) : 475 – 485 .
- De Geus ED. 2012. Respiratory immune responses in the chicken; towards development of mucosal avian influenza virus vaccines [thesis]. Utrecht University, Faculty of Veterinary Medicine
- De Geus , ED , Jansen , CA and Vervelde , L . 2012 . Uptake of particulate antigens in a nonmammalian lung: phenotypic and functional characterization of avian respiratory phagocytes using bacterial or viral antigens . J Immunol , 188 ( 9 ) : 4516 – 4526 .
- De Geus , ED , Rebel , JM and Vervelde , L . 2012 . Kinetics of the avian influenza-specific humoral responses in lung are indicative of local antibody production . Dev Comp Immunol , 36 ( 2 ) : 317 – 322 .
- De Geus , ED , van Haarlem , DA , Poetri , ON , de Wit , JJ and Vervelde , L . 2011 . A lack of antibody formation against inactivated influenza virus after aerosol vaccination in presence or absence of adjuvantia . Vet Immunol Immunopathol , 143 ( 1–2 ) : 143 – 147 .
- De Heer , HJ , Hammad , H , Kool , M and Lambrecht , BN . 2005 . Dendritic cell subsets and immune regulation in the lung . Semin Immunol , 17 ( 4 ) : 295 – 303 .
- De Vries , E , Tscherne , DM , Wienholts , MJ , Cobos-Jimenez , V , Scholte , F , Garcia-Sastre , A , Rottier , PJ and de Haan , CA . 2011 . Dissection of the influenza A virus endocytic routes reveals macropinocytosis as an alternative entry pathway . PLoS Pathog , 7 ( 3 ) : e1001329
- De Wit , JJ , Koch , G , Fabri , TH and Elbers , AR . 2004 . A cross-sectional serological survey of the Dutch commercial poultry population for the presence of low pathogenic avian influenza virus infections . Avian Pathol , 33 ( 6 ) : 565 – 570 .
- De Wit , JJ , Swart , WA and Fabri , TH . 2010 . Efficacy of infectious bronchitis virus vaccinations in the field: association between the alpha-IBV IgM response, protection and vaccine application parameters . Avian Pathol , 39 ( 2 ) : 123 – 131 .
- Delamarre , L , Pack , M , Chang , H , Mellman , I and Trombetta , ES . 2005 . Differential lysosomal proteolysis in antigen-presenting cells determines antigen fate . Science , 307 ( 5715 ) : 1630 – 1634 .
- Dohms , JE , Lee , KP and Rosenberger , JK . 1981 . Plasma cell changes in the gland of Harder following infectious bursal disease virus infection of the chicken . Avian Dis , 25 ( 3 ) : 683 – 695 .
- Dorner , T and Radbruch , A . 2007 . Antibodies and B cell memory in viral immunity . Immunity , 27 ( 3 ) : 384 – 392 .
- Duncker , HR . 1972 . Structure of avian lungs . Respir Physiol , 14 ( 1 ) : 44 – 63 .
- Elbers , AR , Koch , G and Bouma , A . 2005 . Performance of clinical signs in poultry for the detection of outbreaks during the avian influenza A (H7N7) epidemic in The Netherlands in (2003) . Avian Pathol , 34 ( 3 ) : 181 – 187 .
- Fagerland , JA and Arp , LH . 1993a . Distribution and quantitation of plasma cells, T lymphocyte subsets, and B lymphocytes in bronchus-associated lymphoid tissue of chickens: age-related differences . Reg Immunol , 5 ( 1 ) : 28 – 36 .
- Fagerland , JA and Arp , LH . 1993b . Structure and development of bronchus-associated lymphoid tissue in conventionally reared broiler chickens . Avian Dis , 37 ( 1 ) : 10 – 18 .
- Fagerland , JA and Arp , LH . 1990 . A morphologic study of bronchus-associated lymphoid tissue in turkeys . Am J Anat , 189 ( 1 ) : 24 – 34 .
- Farmer , CG . 2006 . On the origin of avian air sacs . Respir Physiol Neurobiol , 154 ( 1–2 ) : 89 – 106 .
- Fedde , MR . 1998 . Relationship of structure and function of the avian respiratory system to disease susceptibility . Poult Sci , 77 ( 8 ) : 1130 – 1138 .
- Fouchier , RA , Schneeberger , PM , Rozendaal , FW , Broekman , JM , Kemink , SA , Munster , V , Kuiken , T , Rimmelzwaan , GF , Schutten , M Van Doornum , GJ . 2004 . Avian influenza A virus (H7N7) associated with human conjunctivitis and a fatal case of acute respiratory distress syndrome . Proc Natl Acad Sci USA , 101 ( 5 ) : 1356 – 1361 .
- Gallego , M and Glick , B . 1988 . The proliferative capacity of the cells of the avian Harderian gland . Dev Comp Immunol , 12 ( 1 ) : 157 – 166 .
- Gambaryan , AS , Piskarev , VE , Yamskov , IA , Sakharov , AM , Tuzikov , AB , Bovin , NV , Nifant'ev , NE and Matrosovich , MN . 1995 . Human influenza virus recognition of sialyloligosaccharides . FEBS Lett , 366 ( 1 ) : 57 – 60 .
- Gaunson , JE , Philip , CJ , Whithear , KG and Browning , GF . 2006 . The cellular immune response in the tracheal mucosa to Mycoplasma gallisepticum in vaccinated and unvaccinated chickens in the acute and chronic stages of disease . Vaccine , 24 ( 14 ) : 2627 – 2633 .
- Gaunson , JE , Philip , CJ , Whithear , KG and Browning , GF . 2000 . Lymphocytic infiltration in the chicken trachea in response to Mycoplasma gallisepticum infection . Microbiology , 146 ( Pt 5 ) : 1223 – 1229 .
- GeurtsvanKessel , CH , Willart , MA , van Rijt , LS , Muskens , F , Kool , M , Baas , C , Thielemans , K , Bennett , C , Clausen , BE Hoogsteden , HC . 2008 . Clearance of influenza virus from the lung depends on migratory langerin+CD11b but not plasmacytoid dendritic cells . J Exp Med , 205 ( 7 ) : 1621 – 1634 .
- Ghendon , Y , Markushin , S , Krivtsov , G and Akopova , I . 2008 . Chitosan as an adjuvant for parenterally administered inactivated influenza vaccines . Arch. Virol. , 153 ( 5 ) : 831 – 837 .
- Ghendon , Y , Markushin , S , Vasiliev , Y , Akopova , I , Koptiaeva , I , Krivtsov , G , Borisova , O , Ahmatova , N , Kurbatova , E Mazurina , S . 2009 . Evaluation of properties of chitosan as an adjuvant for inactivated influenza vaccines administered parenterally . J Med Virol , 81 ( 3 ) : 494 – 506 .
- Hagenaars , N , Mastrobattista , E , Glansbeek , H , Heldens , J , van den Bosch , H , Schijns , V , Betbeder , D , Vromans , H and Jiskoot , W . 2008 . Head-to-head comparison of four nonadjuvanted inactivated cell culture-derived influenza vaccines: Effect of composition, spatial organization and immunization route on the immunogenicity in a murine challenge model . Vaccine , 26 ( 51 ) : 6555 – 6563 .
- Hagenaars , N , Mastrobattista , E , Verheul , RJ , Mooren , I , Glansbeek , HL , Heldens , JG , van den Bosch , H and Jiskoot , W . 2009 . Physicochemical and immunological characterization of N,N,N-trimethyl chitosan-coated whole inactivated influenza virus vaccine for intranasal administration . Pharm Res , 26 ( 6 ) : 1353 – 1364 .
- Hammad , H and Lambrecht , BN . 2007 . Lung dendritic cell migration . Adv Immunol , 92 : 265 – 278 .
- Han , GZ , Liu , XP and Li , SS . 2008 . Caution about Newcastle disease virus-based live attenuated vaccine . J Virol , 82 ( 13 ) : 6782
- Hayter , RB . 1977 . A technique for the exposure and detection of inhaled aerosols in poultry . Poult Sci , 56 ( 3 ) : 949 – 952 .
- Hayter , RB and Besch , EL . 1974 . Airborne-particle deposition in the respiratory tract of chickens . Poult Sci , 53 ( 4 ) : 1507 – 1511 .
- He , F , Ho , Y , Yu , L and Kwang , J . 2008 . WSSV ie1 promoter is more efficient than CMV promoter to express H5 hemagglutinin from influenza virus in baculovirus as a chicken vaccine . BMC Microbiol , 8 : 238
- Holt , PG , Haining , S , Nelson , DJ and Sedgwick , JD . 1994 . Origin and steady-state turnover of class II MHC-bearing dendritic cells in the epithelium of the conducting airways . J Immunol , 153 ( 1 ) : 256 – 261 .
- Huang , JL , Yin , YX , Pan , ZM , Zhang , G , Zhu , AP , Liu , XF and Jiao , XA . 2010. Intranasal immunization with chitosan/pCAGGS-flaA nanoparticles inhibits Campylobacter jejuni in a White Leghorn model. J Biomed. Biotechnol. 2010:589476
- Illum , L . 2003 . Nasal drug delivery–possibilities, problems and solutions . J Control Release , 87 ( 1–3 ) : 187 – 198 .
- Jaganathan , KS and Vyas , SP . 2006 . Strong systemic and mucosal immune responses to surface-modified PLGA microspheres containing recombinant hepatitis B antigen administered intranasally . Vaccine , 24 ( 19 ) : 4201 – 4211 .
- Jeurissen , SH , Janse , EM , Koch , G and De Boer , GF . 1989 . Postnatal development of mucosa-associated lymphoid tissues in chickens . Cell Tissue Res , 258 ( 1 ) : 119 – 124 .
- Jeurissen , SH , Janse , EM , Kok , GL and De Boer , GF . 1989 . Distribution and function of non-lymphoid cells positive for monoclonal antibody CVI-ChNL-68.2 in healthy chickens and those infected with Marek's disease virus . Vet Immunol Immunopathol , 22 ( 2 ) : 123 – 133 .
- Jeurissen , SHM , Vervelde , L and Janse , EM . 1994 . Structure and function of lymphoid tissues of the chicken . Poult Sci Rev , 5 : 183 – 207 .
- Jones , PD and Ada , GL . 1986 . Influenza virus-specific antibody-secreting cells in the murine lung during primary influenza virus infection . J Virol , 60 ( 2 ) : 614 – 619 .
- Joo , HM , He , Y and Sangster , MY . 2008 . Broad dispersion and lung localization of virus-specific memory B cells induced by influenza pneumonia . Proc Natl Acad Sci USA , 105 ( 9 ) : 3485 – 3490 .
- Kazzaz , J , Neidleman , J , Singh , M , Ott , G and O'Hagan , DT . 2000 . Novel anionic microparticles are a potent adjuvant for the induction of cytotoxic T lymphocytes against recombinant p55 gag from HIV-1 . J Control Release , 67 ( 2–3 ) : 347 – 356 .
- Keijzer , C , Slutter , B , van der Zee , R , Jiskoot , W , van Eden , W and Broere , F . 2011 . PLGA, PLGA-TMC and TMC-TPP nanoparticles differentially modulate the outcome of nasal vaccination by inducing tolerance or enhancing humoral immunity . PLoS One , 6 ( 11 ) : e26684
- Kim , TS , Sun , J and Braciale , TJ . 2011 . T cell responses during influenza infection: getting and keeping control . Trends Immunol , 32 ( 5 ) : 225 – 231 .
- Klika , E , Scheuermann , DW , De Groodt-Lasseel , MH , Bazantova , I and Switka , A . 1996 . Pulmonary macrophages in birds (barn owl, Tyto tyto alba), domestic fowl (Gallus gallus f . domestica), quail (Coturnix coturnix), and pigeons (Columbia livia). Anat Rec , 246 ( 1 ) : 87 – 97 .
- Kotani , T , Wada , S , Tsukamoto , Y , Kuwamura , M , Yamate , J and Sakuma , S . 2000 . Kinetics of lymphocytic subsets in chicken tracheal lesions infected with infectious bronchitis virus . J Vet Med Sci , 62 ( 4 ) : 397 – 401 .
- Kothlow , S . 2008 . Avian Immunology , London : Academic Press. . Chapter 14. The avian respiratory immune system; p. 273–288
- Kothlow , S and Kaspers , B . 2008 . The avian respiratory immune system, , 1st ed. , Vol. 273 , San Diego, CA : Academic Press .
- Kuchipudi , SV , Nelli , R , White , GA , Bain , M , Chang , KC and Dunham , S . 2009 . Differences in influenza virus receptors in chickens and ducks: implications for interspecies transmission . J Mol Genet Med , 3 ( 1 ) : 143 – 151 .
- Kumar , M , Chu , HJ , Rodenberg , J , Krauss , S and Webster , RG . 2007 . Association of serologic and protective responses of avian influenza vaccines in chickens . Avian Dis , 51 ( 1 Suppl ) : 481 – 483 .
- Lakadamyali , M , Rust , MJ and Zhuang , X . 2004 . Endocytosis of influenza viruses . Microbes Infect , 6 ( 10 ) : 929 – 936 .
- Lambrecht , BN and Hammad , H . 2010 . The role of dendritic and epithelial cells as master regulators of allergic airway inflammation . Lancet , 376 ( 9743 ) : 835 – 843 .
- Legge , KL and Braciale , TJ . 2003 . Accelerated migration of respiratory dendritic cells to the regional lymph nodes is limited to the early phase of pulmonary infection . Immunity , 18 ( 2 ) : 265 – 277 .
- Letvin , NL . 2007 . Correlates of immune protection and the development of a human immunodeficiency virus vaccine . Immunity , 27 ( 3 ) : 366 – 369 .
- Ley , DH . 2003 . “ ‘Mycoplasmosis’ ” . In Diseases of poultry , 11th , Edited by: Saif , YM , Barnes , HJ , Glission , JR , Fadly , AM , McDougald , LR and Swayne , DE . 722 – 744 . Iowa : Iowa State University Press .
- Liman , M , Peiser , L , Zimmer , G , Propsting , M , Naim , HY and Rautenschlein , S . 2007 . A genetically engineered prime-boost vaccination strategy for oculonasal delivery with poly(D,L-lactic-co-glycolic acid) microparticles against infection of turkeys with avian Metapneumovirus . Vaccine , 25 ( 46 ) : 7914 – 7926 .
- Luschow , D , Werner , O , Mettenleiter , TC and Fuchs , W . 2001 . Protection of chickens from lethal avian influenza A virus infection by live-virus vaccination with infectious laryngotracheitis virus recombinants expressing the hemagglutinin (H5) gene . Vaccine , 19 ( 30 ) : 4249 – 4259 .
- Maas , R , Tacken , M , van Zoelen , D and Oei , H . 2009 . Dose response effects of avian influenza (H7N7) vaccination of chickens: serology, clinical protection and reduction of virus excretion . Vaccine , 27 ( 27 ) : 3592 – 3597 .
- Maina , JN . 2002 . Some recent advances on the study and understanding of the functional design of the avian lung: morphological and morphometric perspectives . Biol Rev Camb Philos Soc , 77 ( 1 ) : 97 – 152 .
- Maina , JN . 2006 . Development, structure, and function of a novel respiratory organ, the lung-air sac system of birds: to go where no other vertebrate has gone . Biol Rev Camb Philos Soc , 81 ( 4 ) : 545 – 579 .
- Mantegazza , AR , Savina , A , Vermeulen , M , Perez , L , Geffner , J , Hermine , O , Rosenzweig , SD , Faure , F and Amigorena , S . 2008 . NADPH oxidase controls phagosomal pH and antigen cross-presentation in human dendritic cells . Blood , 112 ( 12 ) : 4712 – 4722 .
- Marcus , PI , Girshick , T , van der Heide , L and Sekellick , MJ . 2007 . Super-sentinel chickens and detection of low-pathogenicity influenza virus . Emerg Infect Dis , 13 ( 10 ) : 1608 – 1610 .
- Matsuo , K , Yoshikawa , T , Asanuma , H , Iwasaki , T , Hagiwara , Y , Chen , Z , Kadowaki , SE , Tsujimoto , H , Kurata , T and Tamura , SI . 2000 . Induction of innate immunity by nasal influenza vaccine administered in combination with an adjuvant (cholera toxin) . Vaccine , 18 ( 24 ) : 2713 – 2722 .
- Matthijs , MG , Ariaans , MP , Dwars , RM , van Eck , JH , Bouma , A , Stegeman , A and Vervelde , L . 2009 . Course of infection and immune responses in the respiratory tract of IBV infected broilers after superinfection with E. coli . Vet Immunol Immunopathol , 127 ( 1–2 ) : 77 – 84 .
- Moschos , SA , Bramwell , VW , Somavarapu , S and Alpar , HO . 2004 . Adjuvant synergy: the effects of nasal coadministration of adjuvants . Immunol Cell Biol , 82 ( 6 ) : 628 – 637 .
- Munster , VJ , Schrauwen , EJ , de Wit , E , van den Brand , JM , Bestebroer , TM , Herfst , S , Rimmelzwaan , GF , Osterhaus , AD and Fouchier , RA . 2010 . Insertion of a multibasic cleavage motif into the hemagglutinin of a low-pathogenic avian influenza H6N1 virus induces a highly pathogenic phenotype . J Virol , 84 ( 16 ) : 7953 – 7960 .
- Muramoto , Y , Ozaki , H , Takada , A , Park , CH , Sunden , Y , Umemura , T , Kawaoka , Y , Matsuda , H and Kida , H . 2006 . Highly pathogenic H5N1 influenza virus causes coagulopathy in chickens . Microbiol Immunol , 50 ( 1 ) : 73 – 81 .
- Nayak , B , Rout , SN , Kumar , S , Khalil , MS , Fouda , MM , Ahmed , LE , Earhart , KC , Perez , DR , Collins , PL and Samal , SK . 2009 . Immunization of chickens with Newcastle disease virus expressing H5 hemagglutinin protects against highly pathogenic H5N1 avian influenza viruses . PloS One , 4 ( 8 ) : e6509
- Nganpiep , LN and Maina , JN . 2002 . Composite cellular defence stratagem in the avian respiratory system: functional morphology of the free (surface) macrophages and specialized pulmonary epithelia . J Anat , 200 ( 5 ) : 499 – 516 .
- Ochsenbein , AF , Pinschewer , DD , Sierro , S , Horvath , E , Hengartner , H and Zinkernagel , RM . 2000 . Protective long-term antibody memory by antigen-driven and T help-dependent differentiation of long-lived memory B cells to short-lived plasma cells independent of secondary lymphoid organs . Proc Natl Acad Sci USA , 97 ( 24 ) : 13263 – 13268 .
- O'Hagan , DT and Valiante , NM . 2003 . Recent advances in the discovery and delivery of vaccine adjuvants . Nat Rev Drug Discov , 2 ( 9 ) : 727 – 735 .
- Ohshima , K and Hiramatsu , K . 2000 . Distribution of T-cell subsets and immunoglobulin-containing cells in nasal-associated lymphoid tissue (NALT) of chickens . Histol Histopathol , 15 ( 3 ) : 713 – 720 .
- Olah , I , Kupper , A and Kittner , Z . 1996 . The lymphoid substance of the chicken's harderian gland is organized in two histologically distinct compartments . Microscopy Res Tech , 34 ( 2 ) : 166 – 176 .
- Owens , SL , Osebold , JW and Zee , YC . 1981 . Dynamics of B-lymphocytes in the lungs of mice exposed to aerosolized influenza virus . Infect Immun , 33 ( 1 ) : 231 – 238 .
- Pavlova , SP , Veits , J , Mettenleiter , TC and Fuchs , W . 2009 . Live vaccination with an H5-hemagglutinin-expressing infectious laryngotracheitis virus recombinant protects chickens against different highly pathogenic avian influenza viruses of the H5 subtype . Vaccine , 27 ( 37 ) : 5085 – 5090 .
- Pillai , SP and Lee , CW . 2010 . Species and age related differences in the type and distribution of influenza virus receptors in different tissues of chickens, ducks and turkeys . Virol J , 75 : 5 – 12 .
- Powell , FL . 2000 . “ ‘Respiration’ ” . In Sturkie's avian physiology , 5th , Edited by: Whittow , GC . 233 – 264 . London : Academic Press .
- Price , GE , Soboleski , MR , Lo , CY , Misplon , JA , Quirion , MR , Houser , KV , Pearce , MB , Pappas , C , Tumpey , TM and Epstein , SL . 2010 . Single-dose mucosal immunization with a candidate universal influenza vaccine provides rapid protection from virulent H5N1, H3N2 and H1N1 viruses . PLoS One , 5 ( 10 ) : e13162
- Radbruch , A , Muehlinghaus , G , Luger , EO , Inamine , A , Smith , KG , Dorner , T and Hiepe , F . 2006 . Competence and competition: the challenge of becoming a long-lived plasma cell . Nat Rev Immunol , 6 ( 10 ) : 741 – 750 .
- Ramachandra , L , Simmons , D and Harding , CV . 2009 . MHC molecules and microbial antigen processing in phagosomes . Curr Opin Immunol , 21 ( 1 ) : 98 – 104 .
- Rauw , F , Anbari , S , van den Berg , T and Lambrecht , B . 2011 . Measurement of systemic and local respiratory cell-mediated immunity after influenza infection in chickens . Vet Immunol Immunopathol , 143 ( 1–2 ) : 27 – 37 .
- Rauw , F , Gardin , Y , Palya , V , Anbari , S , Gonze , M , Lemaire , S , van den Berg , T and Lambrecht , B . 2010 . The positive adjuvant effect of chitosan on antigen-specific cell-mediated immunity after chickens vaccination with live Newcastle disease vaccine . Vet Immunol Immunopathol , 134 ( 3–4 ) : 249 – 258 .
- Read , RC , Naylor , SC , Potter , CW , Bond , J , Jabbal-Gill , I , Fisher , A , Illum , L and Jennings , R . 2005 . Effective nasal influenza vaccine delivery using chitosan . Vaccine , 23 ( 35 ) : 4367 – 4374 .
- Reemers , SS , van Haarlem , DA , Sijts , AJ , Vervelde , L and Jansen , CA . 2012 . Identification of novel avian influenza virus derived CD8+ T-cell epitopes . PLoS One , 7 ( 2 ) : e31953
- Reese , S , Dalamani , G and Kaspers , B . 2006 . The avian lung-associated immune system: a review . Vet Res , 37 : 311 – 324 .
- Roy , CJ , Ault , A , Sivasubramani , SK , Gorres , JP , Wei , CJ , Andersen , H , Gall , J , Roederer , M and Rao , SS . 2011 . Aerosolized adenovirus-vectored vaccine as an alternative vaccine delivery method . Respir Res , 12 ( 1 ) : 153
- Savina , A , Jancic , C , Hugues , S , Guermonprez , P , Vargas , P , Moura , IC , Lennon-Dumenil , AM , Seabra , MC , Raposo , G and Amigorena , S . 2006 . NOX2 controls phagosomal pH to regulate antigen processing during crosspresentation by dendritic cells . Cell , 126 ( 1 ) : 205 – 218 .
- Savina , A , Peres , A , Cebrian , I , Carmo , N , Moita , C , Hacohen , N , Moita , LF and Amigorena , S . 2009 . The small GTPase Rac2 controls phagosomal alkalinization and antigen crosspresentation selectively in CD8(+) dendritic cells . Immunity , 30 ( 4 ) : 544 – 555 .
- Schroer , D , Veits , J , Keil , G , Romer-Oberdorfer , A , Weber , S and Mettenleiter , TC . 2011 . Efficacy of Newcastle disease virus recombinant expressing avian influenza virus H6 hemagglutinin against Newcastle disease and low pathogenic avian influenza in chickens and turkeys . Avian Dis , 55 ( 2 ) : 201 – 211 .
- Scott , TR , Savage , ML and Olah , I . 1993 . Plasma cells of the chicken Harderian gland . Poult Sci , 72 ( 7 ) : 1273 – 1279 .
- Seo , SH , Peiris , M and Webster , RG . 2002 . Protective cross-reactive cellular immunity to lethal A/Goose/Guangdong/1/96-like H5N1 influenza virus is correlated with the proportion of pulmonary CD8(+) T cells expressing gamma interferon . J Virol , 76 ( 10 ) : 4886 – 4890 .
- Shahzad , MI , Naeem , K , Mukhtar , M and Khanum , A . 2008 . Passive immunization against highly pathogenic Avian Influenza Virus (AIV) strain H7N3 with antiserum generated from viral polypeptides protect poultry birds from lethal viral infection . Virol J , 5 : 144
- Sharma , S , Mukkur , TK , Benson , HA and Chen , Y . 2009 . Pharmaceutical aspects of intranasal delivery of vaccines using particulate systems . J Pharm Sci , 98 ( 3 ) : 812 – 843 .
- Shirama , K , Satoh , T , Kitamura , T and Yamada , J . 1996 . The avian Harderian gland: morphology and immunology . Microsc Res Tech , 34 ( 1 ) : 16 – 27 .
- Slutter , B , Bal , S , Keijzer , C , Mallants , R , Hagenaars , N , Que , I , Kaijzel , E , van Eden , W , Augustijns , P Lowik , C . 2010 . Nasal vaccination with N-trimethyl chitosan and PLGA based nanoparticles: nanoparticle characteristics determine quality and strength of the antibody response in mice against the encapsulated antigen . Vaccine , 28 ( 38 ) : 6282 – 6291 .
- Smart , JD . 2005 . The basics and underlying mechanisms of mucoadhesion . Adv Drug Deliv Rev , 57 ( 11 ) : 1556 – 1568 .
- Stegeman , A , Bouma , A , Elbers , AR , de Jong , MC , Nodelijk , G , de Klerk , F , Koch , G and van Boven , M . 2004 . Avian influenza A virus (H7N7) epidemic in The Netherlands in (2003): course of the epidemic and effectiveness of control measures . J Infect Dis , 190 ( 12 ) : 2088 – 2095 .
- Sui , Z , Chen , Q , Fang , F , Zheng , M and Chen , Z . 2010 . Cross-protection against influenza virus infection by intranasal administration of M1-based vaccine with chitosan as an adjuvant . Vaccine , 28 ( 48 ) : 7690 – 7698 .
- Sui , Z , Chen , Q , Wu , R , Zhang , H , Zheng , M , Wang , H and Chen , Z . 2010 . Cross-protection against influenza virus infection by intranasal administration of M2-based vaccine with chitosan as an adjuvant . Arch Virol , 155 ( 4 ) : 535 – 544 .
- Swayne , DE , ed. 2008 . Avian influenza, 1st ed , Iowa : Blackwell Publishing .
- Swayne , DE , Avellaneda , G , Mickle , TR , Pritchard , N , Cruz , J and Bublot , M . 2007 . Improvements to the hemagglutination inhibition test for serological assessment of recombinant fowlpox-H5-avian-influenza vaccination in chickens and its use along with an agar gel immunodiffusion test for differentiating infected from noninfected vaccinated animals . Avian Dis , 51 ( 3 ) : 697 – 704 .
- Swayne , DE , Suarez , DL , Schultz-Cherry , S , Tumpey , TM , King , DJ , Nakaya , T , Palese , P and Garcia-Sastre , A . 2003 . Recombinant paramyxovirus type 1-avian influenza-H7 virus as a vaccine for protection of chickens against influenza and Newcastle disease . Avian Dis , 47 ( 3 Suppl ) : 1047 – 1050 .
- Tell , LA , Smiley-Jewell , S , Hinds , D , Stephens , KE , Teague , SV , Plopper , CG and Pinkerton , KE . 2006 . An aerosolized fluorescent microsphere technique for evaluating particle deposition in the avian respiratory tract . Avian Dis , 50 ( 2 ) : 238 – 244 .
- Tischer , BK , Schumacher , D , Beer , M , Beyer , J , Teifke , JP , Osterrieder , K , Wink , K , Zelnik , V , Fehler , F and Osterrieder , N . 2002 . A DNA vaccine containing an infectious Marek's disease virus genome can confer protection against tumorigenic Marek's disease in chickens . J Gen Virol , 83 ( Pt 10 ) : 2367 – 2376 .
- Toro , H , Lavaud , P , Vallejos , P and Ferreira , A . 1993 . Transfer of IgG from serum to lachrymal fluid in chickens . Avian Dis , 37 ( 1 ) : 60 – 66 .
- Toro , H , van Ginkel , FW , Tang , DC , Schemera , B , Rodning , S and Newton , J . 2010 . Avian influenza vaccination in chickens and pigs with replication-competent adenovirus-free human recombinant adenovirus 5 . Avian Dis , 54 ( 1 Suppl ) : 224 – 231 .
- Tseng , LP , Chiou , CJ , Chen , CC , Deng , MC , Chung , TW , Huang , YY and Liu , DZ . 2009 . Effect of lipopolysaccharide on intranasal administration of liposomal Newcastle disease virus vaccine to SPF chickens . Vet Immunol. Immunopathol , 131 ( 3-4 ) : 285 – 289 .
- Ugwoke , MI , Verbeke , N and Kinget , R . 2001 . The biopharmaceutical aspects of nasal mucoadhesive drug delivery . J Pharm Pharmacol , 53 ( 1 ) : 3 – 21 .
- Van Alstine , WG and Arp , LH . 1988 . Histologic evaluation of lung and bronchus-associated lymphoid tissue in young turkeys infected with Bordetella avium . Am J Vet Res , 49 ( 6 ) : 835 – 839 .
- Van de Weert , M , Hennink , WE and Jiskoot , W . 2000 . Protein instability in poly(lactic-co-glycolic acid) microparticles . Pharm Res , 17 ( 10 ) : 1159 – 1167 .
- Van den Berg , T , Lambrecht , B , Marche , S , Steensels , M , Van Borm , S and Bublot , M . 2008 . Influenza vaccines and vaccination strategies in birds . Comp Immunol Microbiol Infect Dis , 31 ( 2–3 ) : 121 – 165 .
- Van Eck , JH . 1990 . Vaccination of fowl with inactivated Newcastle disease vaccine by the respiratory route . Avian Pathol , 19 ( 2 ) : 313 – 330 .
- Van Ginkel , FW , Toro , H , Tang , DC and Gulley , SL . 2008 . Induction of mucosal immunity in the avian Harderian gland with a replication-deficient Ad5 vector expressing avian influenza H5 hemagglutinin . Dev Comp Immunol , 33 ( 1 ) : 28 – 34 .
- Veits , J , Luschow , D , Kindermann , K , Werner , O , Teifke , JP , Mettenleiter , TC and Fuchs , W . 2003 . Deletion of the non-essential UL0 gene of infectious laryngotracheitis (ILT) virus leads to attenuation in chickens, and UL0 mutants expressing influenza virus haemagglutinin (H7) protect against ILT and fowl plague . J Gen Virol , 84 ( 12 ) : 3343 – 3352 .
- Villegas , P . 1998 . Viral diseases of the respiratory system . Poult Sci , 77 ( 8 ) : 1143 – 1145 .
- Von Garnier , C , Filgueira , L , Wikstrom , M , Smith , M , Thomas , JA , Strickland , DH , Holt , PG and Stumbles , PA . 2005 . Anatomical location determines the distribution and function of dendritic cells and other APCs in the respiratory tract . J Immunol , 175 ( 3 ) : 1609 – 1618 .
- Webster , RG and Rott , R . 1987 . Influenza virus A pathogenicity: the pivotal role of hemagglutinin . Cell , 50 ( 5 ) : 665 – 666 .
- Wolf , AI , Mozdzanowska , KJ , Quinn , W 3rd , Metzgar , M , Williams , KL , Caton , AJ , Meffre , E , Bram , RJ , Erickson , LD Allman , D . 2011 . Protective antiviral antibody responses in a mouse model of influenza virus infection require TACI . J Clin Invest , 121 ( 10 ) : 3954 – 3964 .
- Worrall , EE and Sudarisman Priadi , A . 2009 . Sialivac: an intranasal homologous inactivated split virus vaccine containing bacterial sialidase for the control of avian influenza in poultry . Vaccine , 27 ( 31 ) : 4161 – 4168 .
- Yates , RM and Russell , DG . 2005 . Phagosome maturation proceeds independently of stimulation of toll-like receptors 2 and 4 . Immunity , 23 ( 4 ) : 409 – 417 .
- Yoshikawa , T , Matsuo , K , Matsuo , K , Suzuki , Y , Nomoto , A , Tamura , S , Kurata , T and Sata , T . 2004 . Total viral genome copies and virus-Ig complexes after infection with influenza virus in the nasal secretions of immunized mice . J Gen Virol , 85 ( Pt 8 ) : 2339 – 2346 .
- Yu , JE , Yoon , H , Lee , HJ , Lee , JH , Chang , BJ , Song , CS and Nahm , SS . 2011 . Expression patterns of influenza virus receptors in the respiratory tracts of four species of poultry . Vet Sci , 12 ( 1 ) : 7 – 13 .